DOI:
10.1039/D3RA01368E
(Review Article)
RSC Adv., 2023,
13, 13655-13682
An updated review of fatty acid residue-tethered heterocyclic compounds: synthetic strategies and biological significance
Received
28th February 2023
, Accepted 21st April 2023
First published on 4th May 2023
Abstract
Heterocyclic compounds have been featured as the key building blocks for the development of biologically active molecules. In addition to being derived from renewable raw materials, fatty acids possess a variety of biological properties. The two bioactive ingredients are being combined by many researchers to produce hybrid molecules that have a number of desirable properties. Biological activities and significance of heterocyclic derivatives of fatty acids have been demonstrated in a new class of heterocyclic compounds called heterocyclic fatty acid hybrid derivatives. The significance of heterocyclic-fatty acid hybrid derivatives has been emphasized in numerous research articles over the past few years. In this review, we emphasize the development of synthetic methods and their biological evaluation for heterocyclic fatty acid derivatives. These reports, combined with the upcoming compilation, are expected to serve as comprehensive foundations and references for synthetic, preparative, and applicable methods in medicinal chemistry.
1. Introduction
Among the monocarboxylic acids, fatty acids (FA) contain long hydrocarbon chains. Saturated or unsaturated oils are generally formed from the cleavage of fats and oils that come from natural sources, like triglycerides or phospholipids.1 The length of the aliphatic tails of the FAs determines the classification: short chain fatty acids (SCFA) have aliphatic tails of five or less carbon atoms, medium chain fatty acids (MCFA) begin with six carbon atoms or more, and long chain fatty acids (LCFA) begin with 14 or more carbon atoms.2,3 In addition to their involvement in membrane function, fatty acids are believed to play a significant role in brain and retinal function.4 Since each fatty acid involves non-physiological conditions, it is hard to evaluate their function in relation to protein function. Nonetheless, many review articles argue that a diet low in saturated fat may decrease the risk of diabetes, heart disease, and death.5,6 Their applications are quite wide in industrial uses such as fuels, surfactants and catalysts.7–9 Furthermore, they can be used as precursors for biologically active compounds through simple transformations; such as the reaction of the carboxylic moiety to produce a stable ester or amide bond.10 These bioactive compounds, branched and cyclic chain FAs, have a variety of biological effects11 such as antiinflammatory,12 antibacterial,13 antioxidant capabilities,14 and have recently been used for cancer treatment.15
On the other hand, heterocyclic rings serve as a primary scaffold for the synthesis of bioactive compounds,16,17 and have a high proclivity for forming complexes with various metals. Some of these complexes have been utilized as antibiotics18 and catalysts in a variety of processes, including the Tsuji–Trost and Mizoroki–Heck reactions.19 Hybridization of fatty acids with heterocyclic rings yields the heterocyclic-fatty acid hybrid derivatives, which results in novel hybrid molecules to broaden the scope of applications compared to the applications of each group separately.12,20
2. Synthesis of five membered heterocycles with one heteroatom
Unsaturated fatty substances were utilized to produce a new C–C bond through radical additions reaction initiated via a metal as catalyst. The methyl oleate 1 was used as the precursor to produce the 2-oxotetrahydrofuran derivative (3) through the reaction with malonic acid and manganese(III) acetate as catalyst under acid condition.21 On the other hand, the reaction of 2-bromopropane methyl ester with 1, using copper as an initiator gave the γ-lactone 6 in 58% yield, in addition to compound 5 that forms as a side product via an addition–elimination reaction22 (Scheme 1).
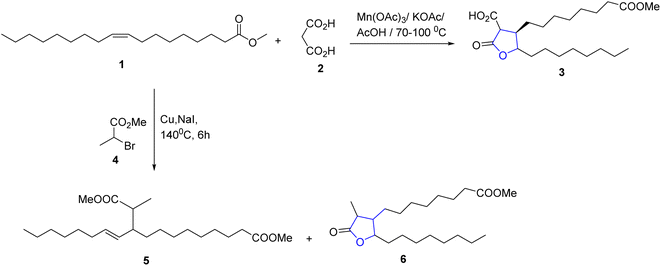 |
| Scheme 1 Synthesis of 2-oxotetrahydrofuran derivative (3) and γ-lactone 6. | |
Hidalgo et al., reported the synthesis of certain novel N-substituted long-chain pyrrole fatty acids via reaction of epoxyoxoene fatty acids 7a and 7b with butylamine and lysine 8a and 8b. Two types of pyrroles were isolated and characterized. 1,2,5-Trisubstituted pyrroles 9a and 9b were the major product while 1,2-disubstituted pyrrole 10a and 10b and short chain aldehydes 11a and 11b were formed in minor23 (Scheme 2).
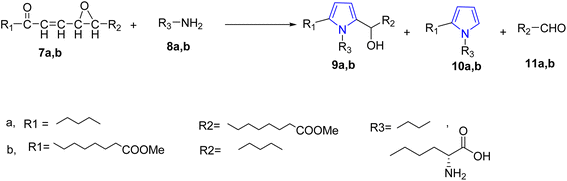 |
| Scheme 2 Preparation of di- and tri-substituted pyrroles. | |
In 2017, a series of 2,5-dimethyl pyrrole 14a–e derivatives were synthesized by Ahmad and co-workers via the reaction of fatty acid 12 with hydrazine hydrate to form fatty acid hydrazides 13a–e which cyclized with acetonyl acetone in presence of ethyl alcohol to afford pyrrole compounds 14a–e. Then, 12 was reacted with methanol to yield the fatty acid esters 15b–e, which later through the reaction with anthranilic acid in the presence of POCl3 furnished the 1,3-benzoxazin-4-one derivatives 16a–e (ref. 24) (Scheme 3).
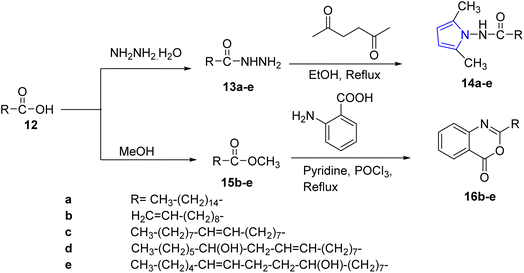 |
| Scheme 3 Synthesis of 2,5-dimethyl pyrroles 14a–e, and synthesis of benzoxazine-4-one derivatives 16a–e. | |
Furthermore, a series of 4,5-dimethoxy tryptamine derivatives with long chain fatty acids were synthesized by Venepally et al.25 The target compounds were obtained via synthetic routes with eight steps sequence. The nitration of 2,3-dimethoxy benzaldehyde 17 as the starting material with fuming nitric acid and glacial acetic acid yielded a mixture of 5-nitro and 6-nitro derivatives. The beseeched compound 19 was obtained via the conversation of 18 by using nitromethane in the presence of 18-crown ether and n-methyl morpholine. Then compound 19 was subjected to cyclization to give 4,5-dimethoxy-1H-indole 20 in the presence of iron and acetic acid. Vilsmeier–Haack reaction was used to yield compound 21 via the introduction of aldehyde moiety to compound 20 (Scheme 4).
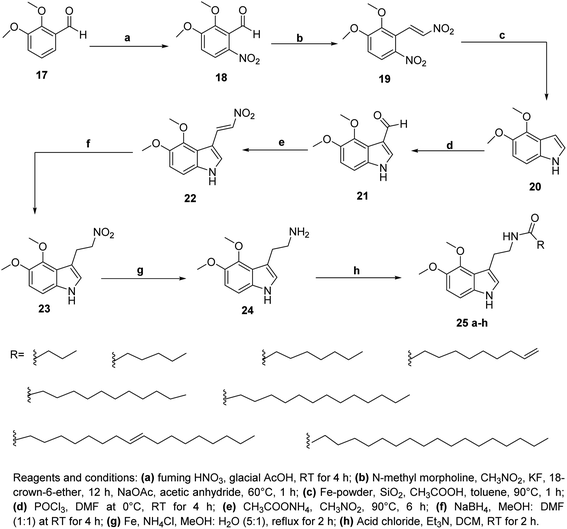 |
| Scheme 4 Synthesis of 4,5-dimethoxy tryptamine derivatives 25a–h with long chain fatty acids from 2,3-dimethoxybenzaldehyde 17. | |
Compound 21 was condensed with nitromethane and ammonium acetate based on the Henry reaction to form the corresponding 4,5-dimethoxy-3-(2-nitrovinyl)-1H-indole 22. The latter compound was reduced to yield 4,5-dimethoxy-3-(2-nitroethyl)-1H-indole 23. Furthermore, compound 23 was refluxed in methanol and water in presence of Fe powder and ammonium chloride to give 2-(4,5-dimethoxy-1H-indol-3-yl) ethylamine 24, which was reacted with fatty acid chlorides in dichloromethane and triethylamine as catalyst giving the corresponding amide derivatives 25a–h (Scheme 4).
3. Synthesis of five membered heterocycles with two heteroatoms
3.1. Synthesis of pyrazole derivative
Compound 26 was treated with hydrazine hydrate in acetic acid/ethanol mixture to yield the pyrazol-5-one 27 bearing the long chain oleyl residue at 3-position. The later compound was subjected to reaction with benzylidene malononitrile in the presence of sodium ethoxide offering the pyrazole derivative 28 (ref. 26) (Scheme 5).
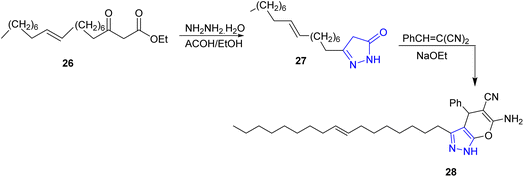 |
| Scheme 5 Synthesis of a pyrazole core containing a pyrano system. | |
Preliminary structural optimization of the series provided compounds 35 and 36 which may lead to the discovery of new inhibitory agents against the cell division cycle 25 (CDC25) phosphatases. Starting from primary amines 29, acylation with acryloyl chloride yielded N-substituted compound 30, which was used as dipolarophile to carry out 1,3-dipolar cycloaddition reaction with α-diazo carbonyl compounds 31 (ref. 27 and 28) in benzene, yielding pyrazoline derivatives 32. The treatment of these derivatives with MnO2 in acetone provided the desired products 33 and 34 which were hydrolyzed by LiOH in THF to introduce free carboxylic group in compounds 35 and 36, respectively29 (Scheme 6).
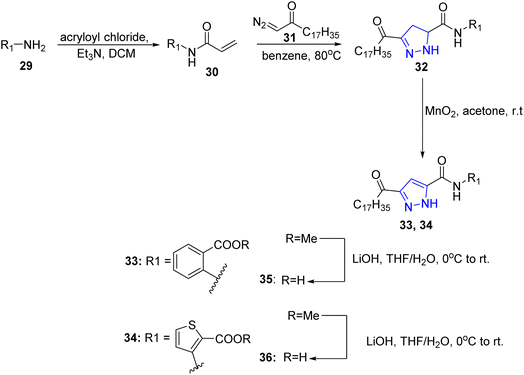 |
| Scheme 6 Preparation of pyrazolo amide derivatives 35 and 36. | |
A series of novel pyrazoline derivatives bearing fatty acid chain were synthesized by Laskar et al. Claisen–Schmidt condensation was used to react the acetophenone 37 with p-chlorobenzaldehyde 38a and furfural 38b in ethanol in presence of sodium hydroxide yielding the products 39a and 39b, respectively. These chalcone compounds were cyclized by various fatty acid hydrazides 40a–c to give the pyrazoline derivatives 41a–c and 42a–c (ref. 30) (Scheme 7).
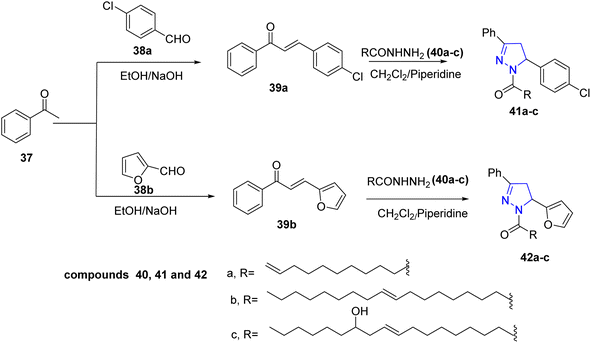 |
| Scheme 7 Synthesis of pyrazoline derivatives bearing fatty acid chain via Claisen–Schmidt condensation. | |
In 2014, Ahmed and coworkers utilized the fatty acid hydrazides 43a–e as the starting material to prepare new long chain fatty acid derivatives of 1,3-disubstituted-1H-pyrazol-5(4H)-one 44a–e and 2-substituted-3H-1,4-phthalazin-1,4-dione 45b–e, through the condensation of hydrazide compound 43a–e with ethylacetoacetate, followed by cyclization to afford pyrazole derivatives 44a–e. Meanwhile, the fatty acid hydrazide 43a–e reacted with phthalic anhydride in ethanol and glacial acetic acid as a catalyst to give phthalazine derivatives 45b–e (ref. 31) (Scheme 8).
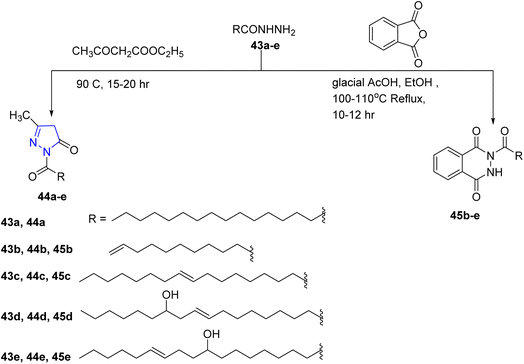 |
| Scheme 8 Synthesis of 1H-pyrazol-5(4H)-one derivatives 44a–e. | |
3.2. Synthesis of 3,5-disubstituted isoxazoles
Kenar et al., synthesized fatty acid esters of disubstituted isoxazoles in two steps starting from aldoximes. Firstly, aldoximes 46 reacted with aqueous sodium hypochlorite and a catalytic amount of triethylamine to form the reactive intermediate 47 which was trapped with methyl 10-undecenoate 48 leading to yield the target compound of the fatty ester isoxazoline derivatives 50 (ref. 32 and 33) (Scheme 9).
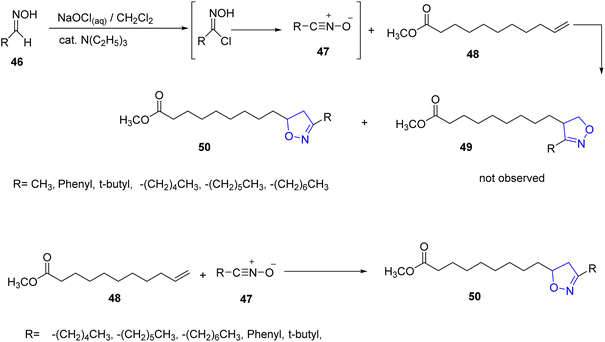 |
| Scheme 9 Synthesis of fatty ester isoxazoline derivatives 50. | |
In addition, the same research group synthesized new isoxazole bearing long chain fatty acid through many steps. It is found that the treatment of N-(propan-2-ylidine) propan-2-amine 51 with an excess of lithium diisopropyl amide (LDA) in THF below −5 °C, gives its corresponding lithiated imine anion 52. Subsequent reaction of the lithiated anion with fatty ester 1 (methyl oleate) which was deprotonated by a second equivalent of LDA to yield the keto enamine 53 which undergoes rearrangement to produce 54. Enamine 54 was then converted into its corresponding ketoxime derivative 55 through the reaction of hydroxylamine hydrochloride at pH 5–6. The latter compound was heated in concentrated sulfuric acid to afford the fatty isoxazole 56 as final product with fairly good yield34 (Scheme 10).
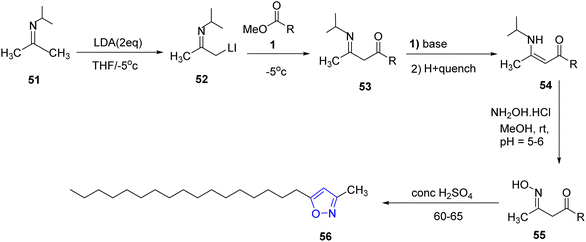 |
| Scheme 10 Preparation of 5-heptadecyl-3-methylisoxazole 56 by using lithium diisopropyl amide. | |
In an attempt to prepare isoxazole with fatty acid, a reaction between three-components; the long chain alkynoic acid 57, Nitrobutane 58 and 1,4-phenylene diisocyanate 59 occurred in tetrahydrofuran (THF) and catalytic amount of Triethylamine to afford 5-(carboxyoctyl)-3-propylisoxazole 60 (Scheme 11).
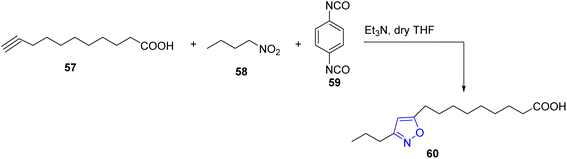 |
| Scheme 11 Synthesis of carboxy isoxazol 60. | |
With the same previous method, the esters of fatty acids 61a–d reacted with nitrobutane 58 and 1,4-phenylene diisocyanate 59 in dry benzene yielded derivatives from fatty acid isoxazole 62a–d (ref. 35) (Scheme 12).
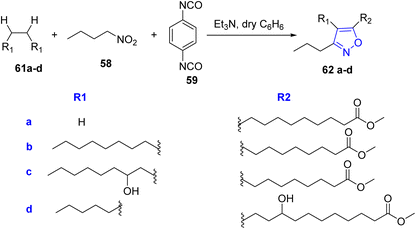 |
| Scheme 12 Synthesis of isoxazole derivatives 62a–d. | |
Anderson et al. have described the preparation of α-keto benzoxazole 65 via acylation of oleoyl chloride 63 with Zn/Cu metalated benzoxazole 64 according to the following scheme36 (Scheme 13).
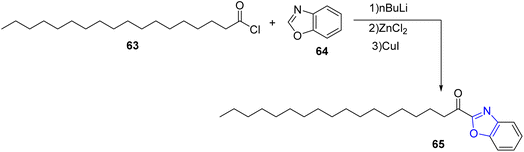 |
| Scheme 13 Synthesis of α-keto benzoxazole 65 via acylation of oleoyl chloride 63. | |
3.3. Synthesis of imidazole, benzoimidazole and thioimidazoline derivatives
Abdul Rauf and co-worker have synthesized imidazole, benzoimidazole and tetrahydrobenzimidazoles bearing long chain fatty acid via a one-pot manner. This reaction started by the formation of the acid chlorides of 66a–d, which then reacted with 1,2-phenylenediamine derivatives 67 in dry dioxane to yield N-acyl-1,2-phenylenediamine derivatives 68a–d. The latter compounds were cyclized by using borontrifluoride and diethylether to afford the target compounds 69a–d. The same method was applied to prepare tetrahydrobenzimidazole 72a–d and imidazole 75a–d using tetrahydrodiamine 70 and ethylenediamine 73, respectively37 (Scheme 14).
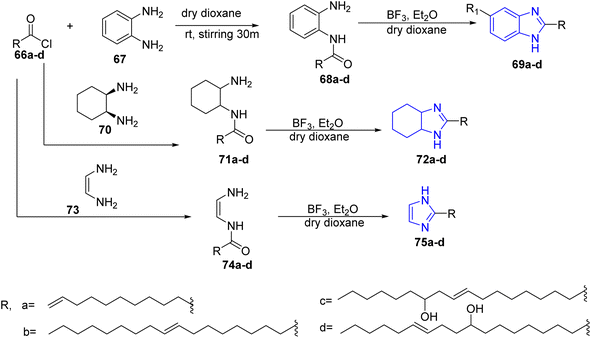 |
| Scheme 14 Formation of imidazole, benzoimidazole and tetrahydrobenzimidazoles bearing long chain fatty acid. | |
The α-hydroxy ketone of oleic acid 77 was achieved in accordance with the method published by Brous and Lefort38 via the oxidation of methyl cis-9,10-epoxyoctadecanoate 76 with dimethyl sulfoxide at 90–100 °C in the presence of boron trifluoride, which was cyclized to obtain the thioimidazoline derivative 78 through the method published by Vandenberghe and Willems procedure39,40 (Scheme 15).
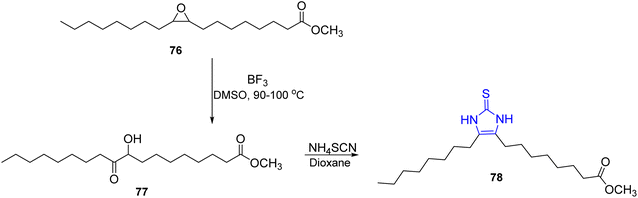 |
| Scheme 15 Synthesis of thioimidazoline derivative 78. | |
On the other hand, Hosamani et al., described a convenient method for the preparation of 2-alkyl substituted benzimidazole derivatives. These target compounds were synthesized from the reaction of derivatives of o-phenylenediamine dihydrochloride 79 and fatty acid derivatives 80 using ethylene glycol as reaction media to furnish the 2-alkyl substituted benzimidazole derivatives 81 (ref. 41) (Scheme 16).
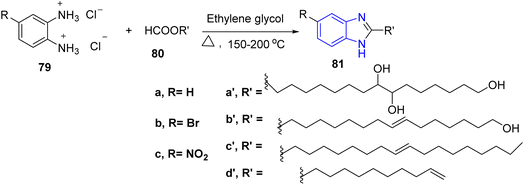 |
| Scheme 16 Synthesis of 2-alkyl substituted benzimidazole derivatives 81. | |
3.4. Synthesis of thiazole, benzothiazole and thiazolidinone derivatives
Many scientific researchers have reported the preparation of thiazolidine derivatives with fatty acid,42–44 therefore, a series of thiazolidine and thiazole appended fatty acid derivatives were synthesized via Gududuru et al. reaction of L-cysteine 82 with numerous aldehydes in ethanol which led to the corresponding acids 83 as diastereomeric mixtures. These mixtures reacted with alkylamines in presence of methylene chloride and EDC/HOBt to afford the corresponding alkylamide thiazolidine 84. Similarly, thiazole fatty acid 89 was prepared via reaction of the methyl D-cysteinate hydrochloride 85 with benzaldehyde to give compound 86 which was converted to the thiazole 87 by NBS, CCl4, then the acidic compound 88 was reacted with alkylamine to afford the target compound of thiazole 89 (ref. 45) (Scheme 17).
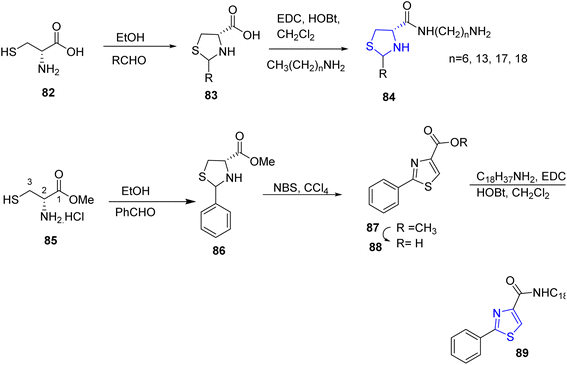 |
| Scheme 17 Synthesis of N-octadecyl-2-phenylthiazole-4-carboxamide 89. | |
In 2008, Abdul Rauf et al. designed and synthesized benzothiazoles bearing long chain fatty acid 93a–e in two steps. First, fatty acid derivatives 90a–e reacted with thionyl chloride to afford the corresponding acid chlorides 91a–e, which reacted with 2-aminothiophenol 92 in toluene to yield the 2-substituted benzothiazoles 93a–e. Alternatively, these target compounds are obtained using microwave by the reaction of fatty acid 90a–e directly with 2-aminothiophenol 92 (ref. 46) (Scheme 18).
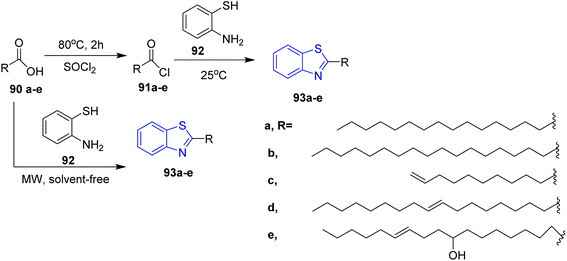 |
| Scheme 18 Synthesis of benzothiazoles bearing long chain fatty acid 93a–e. | |
A novel series of 2,3-disubstituted-4-thiazolidinones was synthesized by Varshney et al.47 This was accomplished by the condensation of fatty acid hydrazides 94a–e with p-chlorobenzaldehyde 95 in dry benzene to afford the key intermediate compounds 96. These intermediates; 96 were conducted on thioglycolic acid (mercapto acetic acid) and thiolactic acid in presence of DMF and catalytic amount of ZnCl2 yielding the thiazolidinones 97a–e and 98b, respectively (Scheme 19).
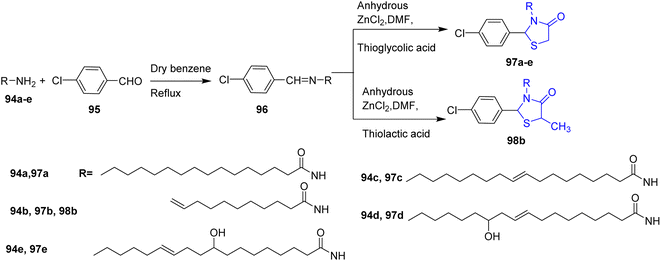 |
| Scheme 19 Synthesis of 2,3-disubstituted-4-thiazolidinones derivatives. | |
4. Synthesis of five membered heterocycles with three heteroatoms
4.1. Synthesis of oxadiazole and thiadiazole derivatives
The fatty acid hydrazide 101 was reacted with chlorosulphonic acid in carbon tetrachloride and neutralized by sodium hydroxide to give sodium salt of α-sulphonated fatty acid hydrazide 102. The later compound has been reacted with carbon disulphide in the presence of potassium hydroxide as a catalyst to give the oxadiazole derivative 103 (ref. 48) (Scheme 20).
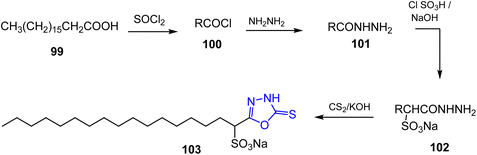 |
| Scheme 20 Synthesis of oxadiazole 103. | |
In another study, Abdul Rauf and collaborators synthesized a series of new 2,5-disubstituted-1,3,4-oxadiazoles by using long-chain alkanoic and alkenoic acids. Fatty acids 104a–f reacted with thionylchloride to afford acid chloride of fatty acids 105a–f, then reacted with hydrazine hydrate in dry dioxane furnishing the corresponding 1,2-diacylhydrazine 106a–f. These derivatives underwent cyclodehydration via using BF3–OEt2 yielding fatty acid of 1,3,4-oxadiazoles 107a–f (ref. 49) (Scheme 21).
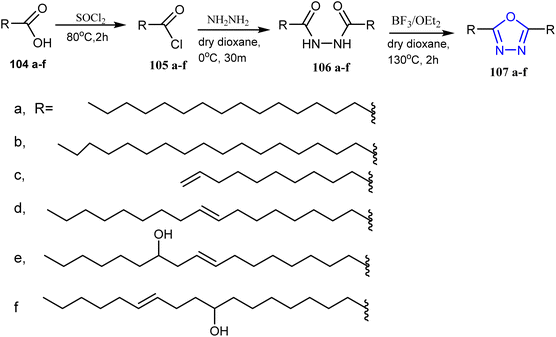 |
| Scheme 21 Synthesis of fatty acid of 1,3,4-oxadiazoles 107a–f. | |
Farshori and co-workers50 reported the synthesis of thiadiazole 110a–c and oxadiazole 112a–c derivatives from long chain fatty acid hydrazides. In this reaction fatty acid hydrazides 108a–c reacted with phenyl isothiocyanate in dry benzene to afford the corresponding thiosemicarbazide derivatives 109a–c which were cyclized using acetic anhydride to give thiadiazoles 110a–c (Scheme 22).
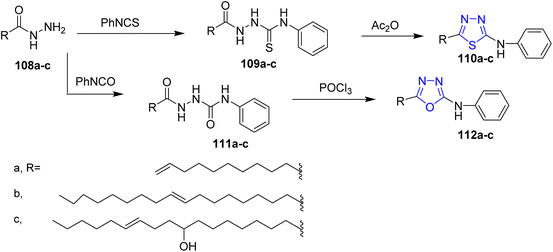 |
| Scheme 22 Synthesis of thiadiazole 110a–c and oxadiazole 112a–c derivatives. | |
Similarly, refluxing the key compounds 108a–c with phenyl isocyanate in presence of dry benzene afforded the semicarbazides 111a–c, then, the treatment of 111a–c with POCl3 furnished oxadiazoles 112a–c (Scheme 23).
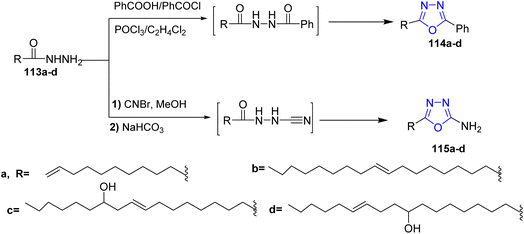 |
| Scheme 23 Preparation of di-substituted 1,3,4-oxadiazoles derivatives. | |
In 2010, Banda and co-workers developed an efficient and facile route for the preparation of disubstituted 1,3,4-oxadiazoles. Fatty acid hydrazides 13a–d were treated with benzoyl chloride under inert atmospheric conditions to afford the intermediates of diacylhydrazide which were cyclized by using dehydrating agent and phosphorus oxychloride to yield the 2-(alkenyl)-5-phenyl-1,3,4-oxadiazoles 114a–d. Alternatively, the 5-(alkenyl)-2-amino-1,3,4-oxadiazoles 115a–d were prepared via treatment of fatty acid hydrazides 113a–d with cyanogen bromide in dry methanol then cyclized to form the target aminoxadiazoles 115a–d (ref. 51) (Scheme 23).
In 2017, Soliman et al.,52 described an efficient and facile route for the preparation of thiadiazole derivatives. This occurred through the reaction of palmitic thiosemicarbazide 116 (prepared via reaction of palmitic acid hydrazide with phenyl isothiocyanate in dry dioxane) and ethyl ester of hydrozonylhalides 117a and 117b in dimethylformamide and catalytic amount of triethylamine which afforded the substituted thiadiazole derivatives 118a and 118b (Scheme 24).
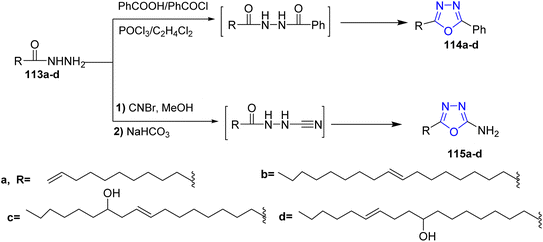 |
| Scheme 24 Synthesis of thiadiazole derivatives 118a and 118b. | |
4.2. Synthesis of triazole and benzotriazole derivatives
In 1998, a series of 1,2,3-triazole derivatives was synthesized via the reaction of acetylenic fatty acid esters such as methyl 2-undecynoate 119, 14-oxo-12-octadecynoates 121 with sodium azide in presence of dimethylformamide to afford the corresponding triazoles 120 and 122, respectively53 (Scheme 25).
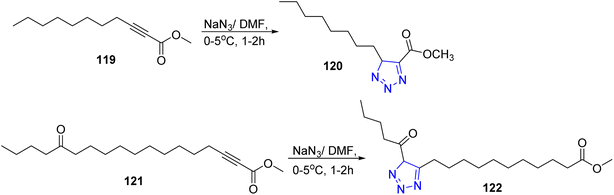 |
| Scheme 25 Synthesis of ester of 1,2,3-triazole derivatives 120 and 122. | |
Furthermore, Abdul Rauf and co-workers reported one pot synthesis of fatty –N-acyl-1H-1,2,3 benzotriazoles 126a–d by stirring benzotriazoles 123, thionylchloride 124 and fatty acids 125a–d in methylene chloride at room temperature54 (Scheme 26).
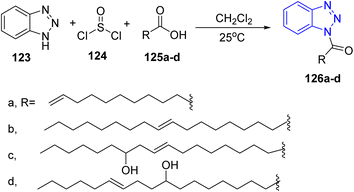 |
| Scheme 26 Preparation of benzotriazole derivatives 126a–d. | |
Similarly in 2009, Rezaei et al., described the synthesis of triazole and benzotriazoles bearing fatty acids from refluxing triazole 128 and benzotriazoles 129 with alkylbromide 127a in acetonitrile added to catalytic amount of tetra ethyl ammonium iodide (TEAI), sodium hydroxide (NaOH) and anhydrous potassium carbonate (K2CO3) to furnish the target compounds 130 and 131, respectively55 (Scheme 27).
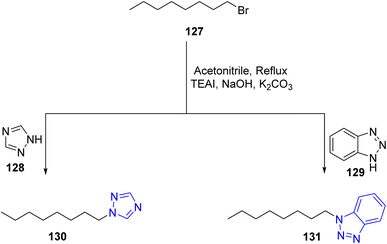 |
| Scheme 27 Synthesis of triazole 130 and benzotriazole 131. | |
In addition, the refluxing of fatty acid hydrazides 132a–d with phenyl isothiocyanate 133 in dry benzene gave thiosemicarbazides 134a–d. The later compounds were subjected to intermolecular cyclization in alkaline medium (2 M, NaOH) followed by acidification with HCl to yield 1,2,3-triazoles 135a–d (ref. 56) (Scheme 28).
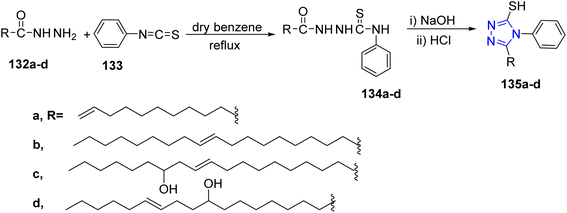 |
| Scheme 28 Synthesis of triazole derivatives 135a–d from fatty acid hydrazides. | |
Moreover, click chemistry was considered as an interesting method for triazole preparation.57–60 Therefore, Labadie and co-workers utilized click chemistry to obtain triazole compounds. Therefore, the azide compound reacted with alkynes by using sodium ascorbate as reductant in the mixture of water and t-BuOH, under catalytic activity of copper(II) sulphate to afford 1,2,3-triazole61 (Scheme 29).
 |
| Scheme 29 Synthesis of triazole 140 via click reaction. | |
In 2012, another important work for the synthesis of stearic fatty acid of triazoles by Jubie et al. was described. The key intermediate of benzoic hydrazide 143 was obtained by refluxing ethyl benzoate 141 and hydrazine hydrate 142 in ethyl alcohol. Compound 143 reacted with stearic acid in presence of phosphorous oxychloride and neutralized with NaOH to furnish 1,3,4-oxadiazole 144. The treatment of 144 with excess hydrazine yielded the 2-(heptadecyl)-5-phenyl-1,2,4-triazole 145. In addition, the reaction of acid hydrazide 143 with carbondisulfide in ethanol using potassium hydroxide led to the formation of the corresponding potassium dithiocarbazinate 143a which was refluxed with hydrazine hydrate to give 4-amino-5-phenyl-4H-1,2,4 triazole-3-thiol 146. The later compound was subjected to cyclization using stearic acid and POCl3 as a solvent to get 6(-heptadecyl)-3-phenyl-[1,2,4] triazolo[3,4-b]1,3,4-thiadiazole 147 (ref. 62) (Scheme 30).
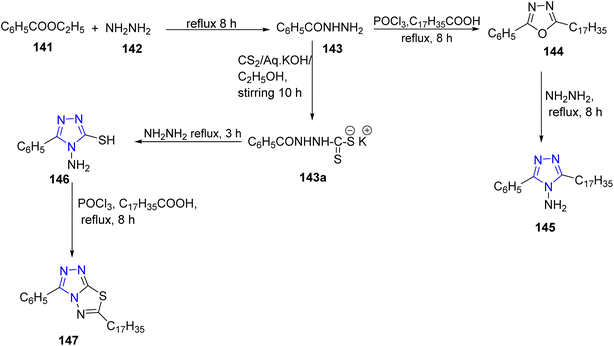 |
| Scheme 30 Synthesis of 3-heptadecyl-5-phenyl-4H-1,2,4-triazol-4-amine 145 and 6-heptadecyl-3-phenyl-[1,2,4]triazolo[3,4-b][1,3,4]thiadiazole 147. | |
5. Synthesis of five membered heterocycles with four heteroatoms
5.1. Synthesis of 5-alkyl-1H-tetrazoles
Various fatty nitriles63 were converted to the corresponding 5-alkyl-1H-tetrazoles by using 3 equivalents of sodium azide and triethylamine hydrochloride in dry toluene such as oleyl nitrile 148 which could be converted to 5-oleyl-1H-tetrazole 149 (ref. 33). It is worthy to mention that, the 5-oleyl-1H-tetrazole 149 could be converted to the corresponding 1,3,4-oxadiazole 150 in a satisfactory yield via Huisgen reaction64 (Scheme 31).
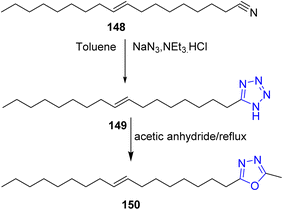 |
| Scheme 31 Synthesis of tetrazole 149 and oxadiazole 150. | |
Further, Suzuki et al.65 reported that the 1,5-disubstituted tetrazoles may be synthesized from ketones. Therefore, a mixture of methyl 9-oxoheptadecanoate 151, sodium azide and titanium(IV) chloride were refluxed in acetonitrile, to produce methyl 8-(1-octyl-1H-tetrazol-5-yl)octanoate 152 in a good yield (Scheme 32).
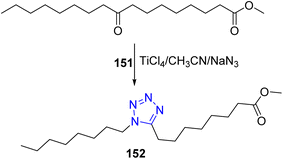 |
| Scheme 32 Synthesis of methyl 8-(1-octyl-1H-tetrazol-5-yl)octanoate 152. | |
Moreover, the alkyl branched tetrazole could be achieved by the reaction of methyl oleate 1 with iodoacetonitrile in the presence of copper powder.66 Then, the resulting cyanomethyl-iodo derivative 153 was reduced under hydrogenation in the presence of palladium on charcoal to afford the intermediate 154 which was reacted with sodium azide to give the alkyl branched tetrazole derivative 155 as a final product33 (Scheme 33).
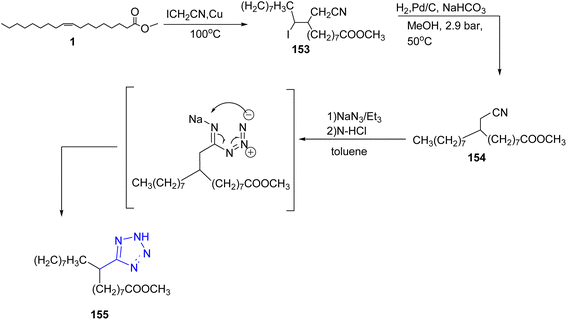 |
| Scheme 33 Synthesis of tetrazole 155 from methyl oleate 1. | |
6. Synthesis of six membered heterocycles with one heteroatom
6.1. Synthesis of quinolone and isoquinoline derivatives
In 2016, Venepally et al., performed the synthesis of dihydro-4-oxoquinoline linked to fatty acids. Starting from the reduction of 3,4-difluoro nitrobenzene 156 with iron and ammonium chloride in methanol to afford 3,4-difluorobenzenamine 157. The latter compound was treated with diethyl ethoxymethylene malonate to give diethyl-2-((3,4-difluorophenylamino) methylene) malonate 158. Then compound 158 was cyclized under reflux in presence of diphenyl ether yielding ethyl 6,7-difluoro-1,4-dihydro-4-oxoquinoline-3-carboxylate 159. Furthermore, ethyl-1-ethyl-6,7-difluoro-1,4-dihydro4-oxoquinoline-3-carboxylate 160 was obtained by the treatment of compound 159 with iodoethane in the presence of potassium carbonate. The next step aimed at the formation of azide group at C-7 position by reacting compound 160 with sodium azide in dimethyl formamide to give compound 161. This compound was reduced using zinc and ammonium chloride to yield compound 162. Finally, the fatty acyl chlorides reacted with compound 162 in the presence of triethylamine in dichloromethane to form the corresponding target compounds 163a–h (ref. 67) (Scheme 34).
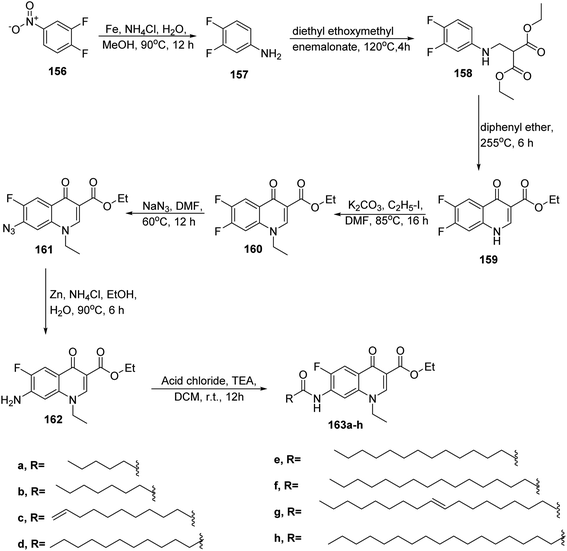 |
| Scheme 34 Preparation of dihydro-4-oxoquinoline derivatives 163a–h. | |
In addition, Malinak and co-workers published the reflux reaction of 6-hydroxyquinoline 164 with alkylbromide in acetonitrile to afford 6-hydroxyquinolinium salts bearing long chain side chain 165a–d (ref. 68) (Scheme 35).
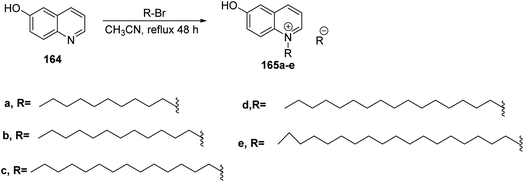 |
| Scheme 35 Synthesis of 6-hydroxyquinolinium salts bearing fatty acids derivatives 165a–e. | |
Moreover, the Pictet–Spengler reaction69,70 for the synthesis of isoquinolines was used to afford 1-substituted-1,2,3,4-tetrahydroisoquinolines 168a–d through the reaction of dopamine 166 with aldehyde derivatives 167a–d in n-propanol. The treatment of 168a–d with an excess of methyl chloroformate in the presence of pyridine gave the peracyl derivatives 169a–d. The deprotection of carbonate groups was performed via ammonolysis under very mild conditions to afford amides 170a–d which directly were di-o-methylated with methyl iodide to give the 1-substituted-6,7-dimethoxy-1,2,3,4-tetrahydroisoquinolines 171a–d in fair yields71,72 (Scheme 36).
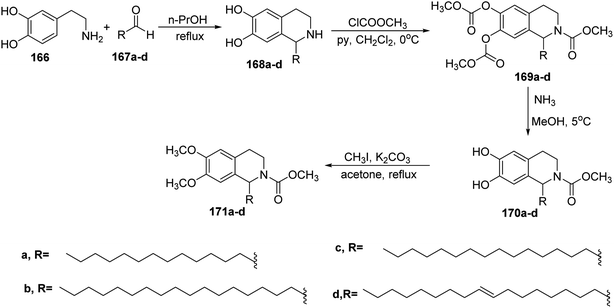 |
| Scheme 36 Synthesis of 1-substituted-6,7-dimethoxy-1,2,3,4-tetrahydroisoquinolines 171a–d by using Pictet–Spengler reaction. | |
Also, there was another method for preparation of isoquinoline skeleton named Bischler–Napieralski cyclization.69,70 In that case, it has been started by homoveratrylamine (3,4-dimethoxyphenethylamine) 172 and the appropriate fatty acid 173a–d to form amides 174a–d in mild condition reaction.73 This method afforded optimal yield of the product, in addition to the chemical and stereochemical stability of the acid used. Then, treatment of the amides 174a–d with phosphorous pentachloride in dichloromethane at 0 °C gave relatively unstable imines 175a–d in fair yields, which were pure without any contamination of chlorinated products. The later compounds 175a–d were reduced with sodium borohydride to afford the secondary amines 176a–d in high yields (around 80%), which were quite stable without observable decomposition at room temperature74 (Scheme 37).
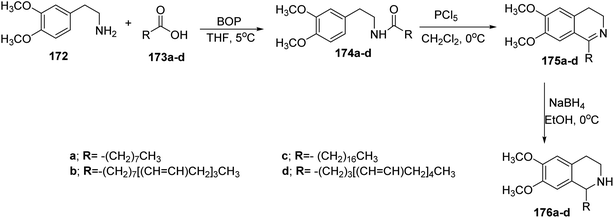 |
| Scheme 37 Synthesis of isoquinoline derivatives 176a–d. | |
In 2016, Diego da Costa Cabrera et al. utilized a multi-component reaction to prepare polyhydroquinoline with fatty acid in the presence of catalyst. In this reaction, a mixture of four-component reaction including fatty β-ketoesters 177a–c with the appropriate aromatic aldehydes 178a–e, dimedone 179 and ammonium acetate 180 were boiled in acetonitrile in presence of sulfamic acid (H2NSO3H) or indium chloride (InCl3) as a catalyst to afford the polyhydroquinoline derivatives bearing long chain fatty acid 181a–c as target compounds75 (Scheme 38).
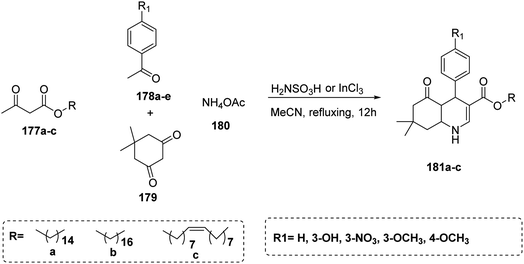 |
| Scheme 38 Synthesis of polyhydroquinoline derivatives bearing long chain fatty acid 181a–c through one-pot four-component reaction. | |
6.2. Synthesis of iso flavone and pseudopyronine fatty acid derivatives
The Iso flavone fatty acid esters were obtained by refluxing the derivatives of daidzein 184 and fatty acid chloride in pyridine as a catalyst and methylene chloride. In this reaction, 2,4-dihydroxy-4′-methoxydeoxybenzoin 183 was prepared from reaction of resorcinol 181 and 4-methoxy phenyl acetic acid 182 by Friedel–Crafts acylation using boron trifluoride etherate as a solvent. Then, compound 183 was treated with Vilsmeier reagent to afford 4′-methoxy daidzein 184 (7-hydroxy-3-(4-methoxyphenyl)-4H-chromen-4-one) under mild conditions.76 The later compound reacted with fatty acid chloride derivatives yielding daidzein derivatives 185a–e. Furthermore, 7-methoxy daidzein with fatty acid derivatives 190a–e were obtained with a similar strategy using 3-methoxyphenol 186 as starting material77,78 (Scheme 39).
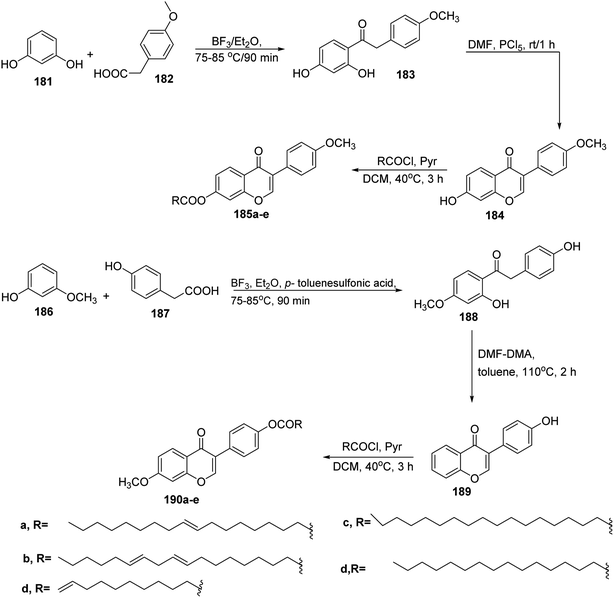 |
| Scheme 39 Formation of iso-flavone fatty acid esters derivatives 190a–e. | |
On the other hand, the preparation of pseudo pyronine 194 (ref. 79 and 80) started from the known methyl β-oxo esters 191a and 191b, which were deesterified to the corresponding carboxylic acids 192a and 192b via saponification.81 Then, cyclization of 192 using carbonyl diimidazole afforded the target acylpyrone skeleton 193a & 193b. Then the deviation started, the pseudo pyronine 194 was achieved by reducing 193a.82 Alternatively, the achievement of pseudopyrone B 197 began with the deacylation of 193b to yield 6-heptyl-4-hydroxy-2-pyrone 195 followed by acylation to furnish 196, which was reduced giving 197 (ref. 83) (Scheme 40).
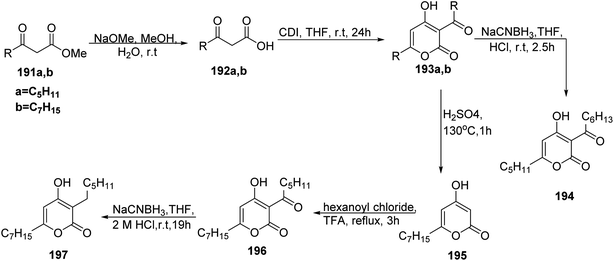 |
| Scheme 40 Synthesis of pseudo pyronine 194 and pseudopyrone B 197. | |
7. Synthesis of six membered heterocycles with two heteroatoms
7.1. Synthesis of uracil bearing fatty residues
In this study, uracil derivative 198 was subjected to silylation process using hexamethyldisilazine (HMDS) to afford bis (trimethylsilyl) derivative 199, then reacted with fatty acid of stearoyl chloride 200 to give uracil having N-fatty residue 201 (ref. 84) (Scheme 41).
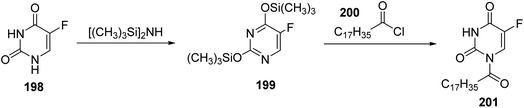 |
| Scheme 41 Synthesis of 5-fluoro-1-stearoylpyrimidine-2,4(1H,3H)-dione 201. | |
Xu and co-workers85 have reported the reaction of fluorouracil 202 with 2-chloroethanol 203 in acetonitrile and NaHCO3 affording a mixture of two compounds 204 and 205 in 1
:
6 molar ratio. Then, novel fatty acid nucleoside conjugates 207 were synthesized via the reaction of 204 with the appropriate fatty acid 206 (Scheme 42).
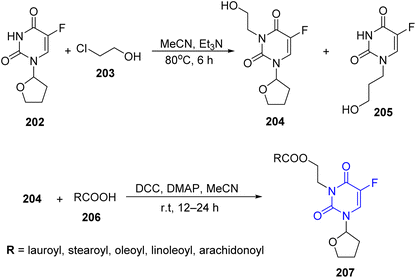 |
| Scheme 42 Synthesis of fluorouracil bearing ester of fatty acids derivatives 207. | |
Furthermore, The β-keto ester 208 was reacted with thiourea in the presence of sodium ethoxide to afford 209 having the fatty residue at position 6 (ref. 86) (Scheme 43).
 |
| Scheme 43 Synthesis of 6-heptadecyl-2-thioxo-2,3-dihydropyrimidin-4(1H)-one 209. | |
Moreover, it has been mentioned that the syntheses of 1,6-heptadienes with uracil and/or thymine was achieved starting from the 2-allyl-pent-4-enoic acid 210.87 Reduction of compound 210 to the corresponding primary alcohol, 2-allyl-pent-4-en-1-ol 211, was achieved with lithium aluminum hydride (LAH) in 98% yield.88,89 The 3N-protected uracil and thymine bases have been prepared following the reported procedures.90 The Mitsunobu reaction was then employed to couple the 3N-protected nucleic bases to 2-allyl-pent-4-en-1-ol utilizing triphenylphosphine and diisopropyl azodicarboxylate (DIAD) in dry dioxane to yield the heptadiene derivatives 212 and 213 in 69% and 77% yield, respectively.91–93 The 3N-benzoyl groups of intermediates 212 and 214 were then hydrolyzed in a methanolic solution of sodium methoxide to yield the target compounds 1-(2-allyl-pent-4-enyl)-1H-pyrimidine-2,4-dione 213 and 1-(2-allyl-pent-4-enyl)-5-methyl-1H-pyrimidine-2,4-dione 215 in 85% and 78% yield, respectively94 (Scheme 44).
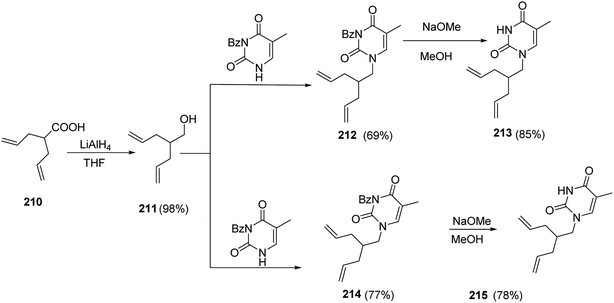 |
| Scheme 44 Synthesis of 1-(2-allyl-pent-4-enyl)-1H-pyrimidine-2,4-dione 213 and 1-(2-allyl-pent-4-enyl)-5-methyl-1H-pyrimidine-2,4-dione 215. | |
7.2. Synthesis of tetrahydropyrimidine and hydropyrimidinone derivatives
Recently, a synthetic approach to new glycolipids has been developed using Staudinger reaction. Starting from the reaction of lactose octaacetate 216 with commercially available 1,3-dichloro-2-propanol followed by a subsequent substitution of the halide 217 (ref. 95) provided diazide 218 (ref. 96) in 48% yield. Staudinger reactions with various fatty acid chlorides ranging from C8 to C12, however, failed to provide the expected diamide 219. Instead, the cyclic coupling products 220 were obtained in reproducible yields of 70%, which provided the corresponding surfactants 221a–c upon deacetylation. Although phosphine mediated cyclizations of azido-amides have been reported previously,97–99 the reaction product is rather unexpected. This does not only refer to the formation of tetrahydopyrimidines, which so far have been accessed by different strategies,100–102 but particularly relates to the different behavior of glucose and lactose, despite identical stereochemistry at the reducing sugar103 (Scheme 45).
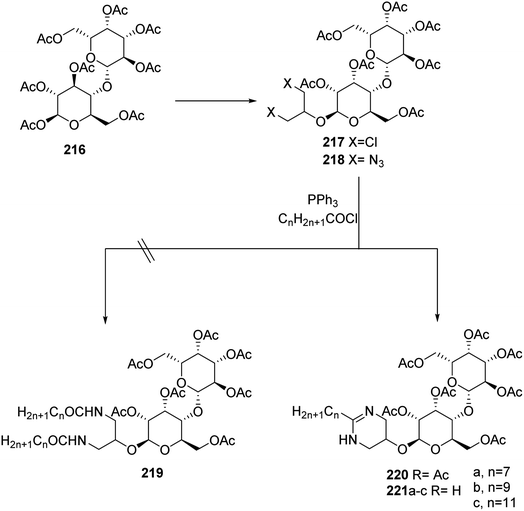 |
| Scheme 45 Synthesis of tetrahydropyrimidine derivatives 220 and 221a–c. | |
Moreover, in 2015 Treptow et al.104 synthesized new series of fatty acid 3,4-dihydropyrimidinones as a result of utilization of Biginelli multicomponent reaction. This reaction occurred by boiling a mixture of fatty acid of β-ketoester 222a–c, with aromatic aldehydes 223 and urea 224 or thiourea 225 in acetonitrile in the presence of indium chloride as catalytic amount to afford the target compounds of dihydropyrimidinone bearing fatty acids 226a–c (Scheme 46).
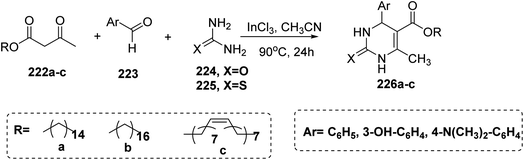 |
| Scheme 46 Preparation of dihydropyrimidinone bearing fatty acids 226a–c via one-pot three-component reaction. | |
In 2017, Kumarasamy and co-workers described an efficient strategy for the preparation of derivatives of dihydropyrimidinone 229a–m via the reaction of ethyl acetoacetate 227, with different aldehydes 228a–m and urea 224. This reaction was conducted under two different conditions: first condition was solvent free heating and the other was reflux in acetic acid and ethanol105 (Scheme 47).
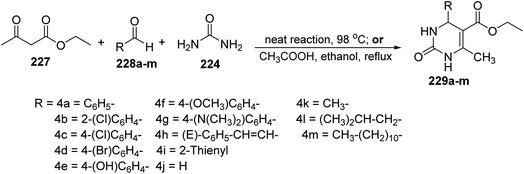 |
| Scheme 47 Synthesis of dihydropyrimidinone 229a–m. | |
7.3. Synthesis of quinazoline derivatives
It has been reported that 2-hydroxyheptadecanoic acid chloride 230 reacted with anthranilic acid 231 in pyridine to produce 2-(1-hydroxyheptadecyl)-1,3-benzoxazin-4-one 232, which was used as starting material to synthesize some condensed and non-condensed heterocyclic compounds by the reaction with nitrogen nucleophiles such as hydrazine hydrate and formamide to give 3-amino-2-(hydroxyheptadecyl)-3H-quinazolin-4-one 233 and 2-(1-hydroxy heptadecyl)-3H-quinazolin-4-one 234, respectively. Later, compound 233 was reacted with benzoyl chloride to afford 236. Also, the treatment of 234 with chloroacetyl chloride in dimethyl formamide as a solvent gave 237 which was converted to the corresponding hydrazine derivative 238 via the heating of hydrazine hydrate in butanol. The hydrazine-derivative 238 was cyclized by fusion above its melting point to 239 (ref. 106) (Scheme 48).
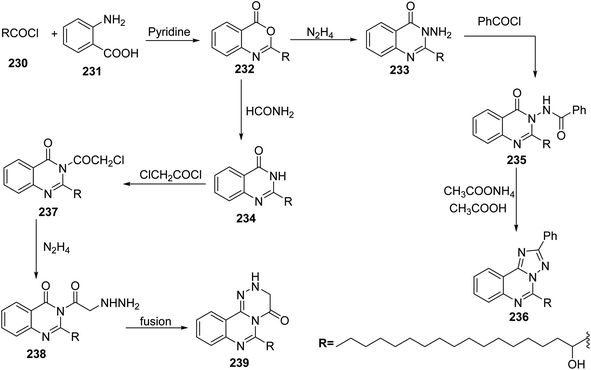 |
| Scheme 48 Synthesis of quinazoline derivatives 234–239. | |
8. Synthesis of six-membered heterocyclic compounds with three heteroatoms
8.1. Synthesis of triazines
Synthesis of 3,5,6-trisubstituted-1,2,4-triazines 242 has been achieved by condensation of 1,2-diketones 241 with various saturated and olefinic fatty acid hydrazides 240 (e.g. oleoyl hydrazide) under Microwave (MW) and solvent-free conditions in short times107 (Scheme 49).
 |
| Scheme 49 Preparation of 3,5,6-trisubstituted-1,2,4-triazines 242. | |
9. Synthesis of other heterocycles
The reaction of carboxylic acids 243a–f with 2-aminophenol 244 in the presence of N,N′-dicyclohexylcarbodiimide (DCC) and 4-dimethylaminopyridine (DMAP) in dichloromethane to afford several o-hydroxy anilide derivatives 245a–f and 1-substituted-1,3-dicyclohexylurea analogues of carboxylic acids 246a–f was conducted. During the DCC mediated reaction for the amide synthesis, the1,3-dicyclohexylurea analogues are formed as a side product108 (Scheme 50).
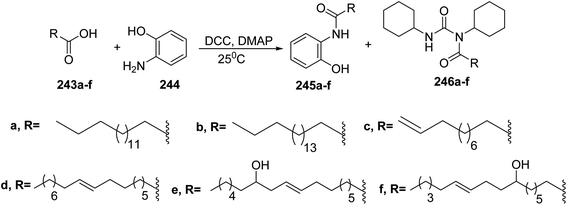 |
| Scheme 50 Synthesis of o-hydroxy anilide derivatives 245a–f and 1-substituted-1,3-dicyclohexylurea analogues of carboxylic acids 246a–f. | |
Pericyclic reaction such as the reaction of fatty esters as diene (e.g. methyl cis-9,10-epiminooctadecanoate 1) with dienophile 247 in the presence of Lewis acid (e.g.: BCl3 or SnCl4) as a catalyst, to give the cyclic adduct (Scheme 51). Moreover, cyclo addition of fatty acids with dimethyl acetylene dicarboxylate 249 gave Diels–Alder cyclo adduct 250 which was oxidized with DDQ to give the cycloarenes 251 which has a fatty acid residue109 (Scheme 52). Furthermore, it has been found that the reaction of glucose 252 with oleic acid 253 in the presence of pyridine afforded O-oleoylglucose 254 in fairly yield110 (Scheme 53).
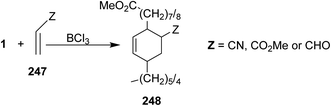 |
| Scheme 51 One-pot synthesis of 248. | |
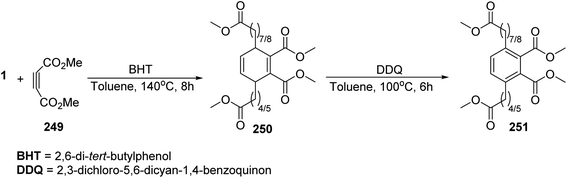 |
| Scheme 52 Synthesis of cycloarenes 251 via Diels–Alder reaction. | |
 |
| Scheme 53 Synthesis of O-oleoylglucose 254 from oleic acid. | |
The target aminoacyl 263 was prepared in 7 steps, where, the 5′ position of 255 was protected with the TBDMS group to yield compound 256, which was reacted with oleic acid to obtain 2′-oleyl derivative 257 in high yield. The 3′-azido group was reduced to 3′-amine 258 through catalytic hydrogenation. The next step involved the condensation of 258 with the protected L-proline to give the aminoacyl nucleolipid 259, which was deprotected at the 5′ position to give 260. At this stage a hydrophilic residue previously synthesized111 was attached at the 5′ position through an ester linkage giving nucleoside 261, followed by mild acidic treatment to provide the aminoacyl nucleolipid 262 as trifluoroacetate salt. Finally, the trifluoroacetate counterion was then replaced with chloride to receive the target compound 263 in biocompatible form112 (Scheme 54).
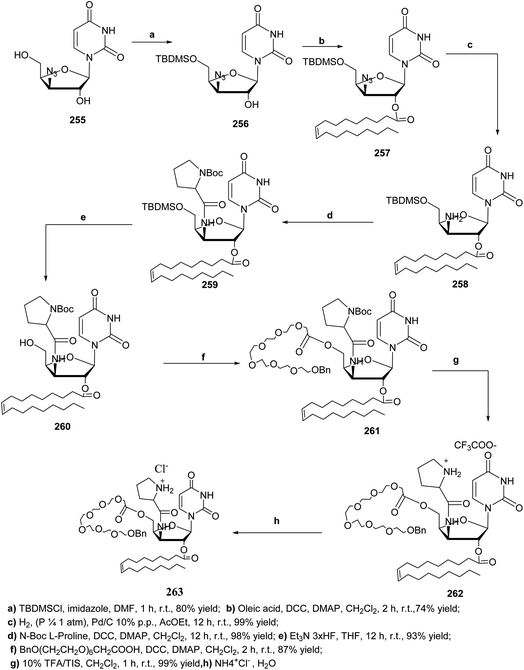 |
| Scheme 54 Synthesis of compound 263. | |
Finally, the enzymatic reactions are used to provide hybrid compounds of flavonoids and fatty acids using lipase to create esters. Ziaullah et al., used such technique to acylate isoquercitrin 264 and phloridzin 271 with fatty acids (C18–C22) using lipase novozyme under several reaction conditions113 (Scheme 55).
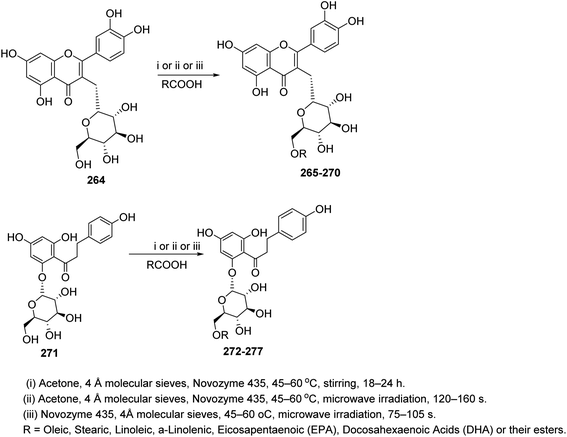 |
| Scheme 55 Acylate isoquercitrin 264 and phloridzin 271 with fatty acids. | |
10. Application and pharmacological uses of fatty substances
10.1. Application of fatty substances
10.1.1. Their usage as surface active agents. It was noticed that when the fatty acids are present in the surfactant range (C8–C16) the highly surface-active compounds are formed,7,9 so that there is a family of novel mono-alkyl glycerol ether surfactants with different hydrophobic length (C9–C16) and tryptophan were synthesized. Based on the number and polarity of groups present, the amphiphile is going from hydrophilic to lipophilic with the increase of carbon atoms in the alkyl chains (e.g. phthalazine derivative 278 as a good anionic surfactant)51 (Fig. 1).
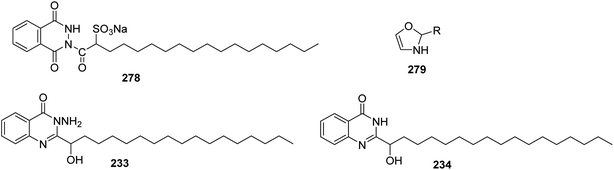 |
| Fig. 1 Compounds as surface active agents. | |
Moreover, the surface-active properties of fatty acid–dihydroxazole hybrids 279 were reported. Also, their respective salts are good cationic surfactants114 (Fig. 1).
In addition, a novel group of nonionic surface-active agents were synthesized, which consist of a hybrid from α-hydroxyl fatty acid and quinazolines 233 and 234. The active hydrogen at position 2 of quinazoline was subjected to reaction with ethylene oxide or propylene oxide115,116 to produce these hybrids having a double functions as antimicrobials and surface active agents which can be useful in the manufacture of drugs and cosmetics106 (Fig. 1).
10.1.2. As corrosion inhibitor. Heterocyclic compounds having fatty residue possess anticorrosion effect.117 Compounds 280 (UMOD) and 281 (HMOD) were prepared and their anticorrosive effect mild steel was investigated by weight-loss and potentiodynamic polarization techniques. Their inhibitory effect varied depending on concentration, temperature and immersion time. Their adsorption on the steel surface obeys Temkin's adsorption isotherm. The potentiodynamic polarization data showed that the inhibitory type of the synthesized compounds is mixed118 (Fig. 2).
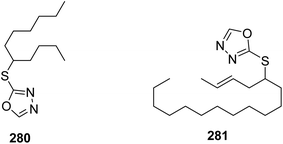 |
| Fig. 2 Heterocyclic compounds having fatty residue with anticorrosion effect. | |
10.2. Pharmaceutical uses
It was reported that the anti-proliferative profile of fatty acyl amide derivatives was higher in ovarian and colon cell lines than leukemia and breast cancer cells. Moreover, the dodecanoyl derivative was the most effective concerning in vitro test of all the examined cell lines, which indicates its potential application for slow delivery of doxorubicin. Therefore, doxorubicin bearing fatty acyl amide moiety (282) was prepared which showed higher lipophilicity than doxorubicin119 (Fig. 3).
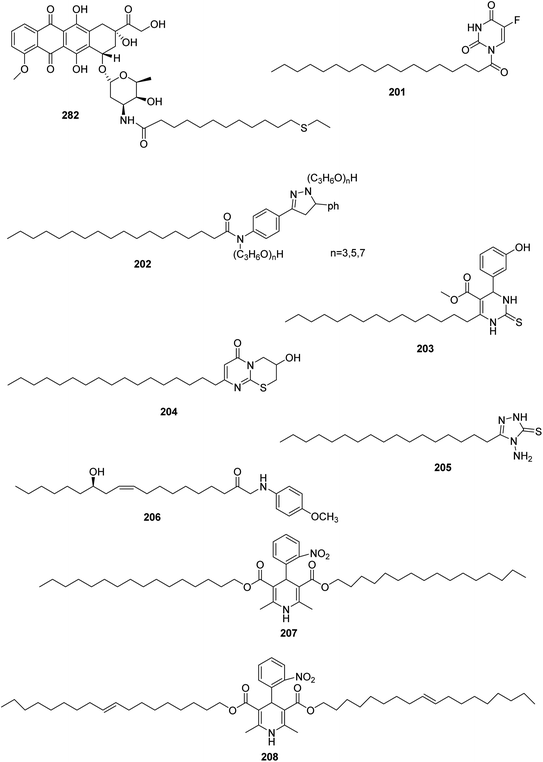 |
| Fig. 3 Compounds have pharmacological activity. | |
In addition, 201 was obtained by the acylation of 5-fluorouracil, then was incorporated into solid lipid nanoparticles (SLN) to produce the mean dimer 5-FUS-SLN. This dimer has better profile concerning liver targeting properties by enhancing drug liposoluble properties120 (Fig. 3).
Abdelmajeid et al. synthesized new series of pyrazole, isoxazole, pyrimidine and pyridine derivatives with heptadecyl fatty acids and screened them for their antioxidant and anticancer activity. The biological evaluations showed that the most effective compound concerning antioxidant and cytotoxic activities was 202 (ref. 121) (Fig. 3).
In 2018, De Oliveira et al. prepared novel derivatives of long-chain monastrol analogues as antitumor agents against rat glioblastoma cells.122 The series was synthesized via the multicomponent reaction then tested for their in vitro antitumor activity. Compound 203 showed the strongest antitumor effect (IC50 = 5.11 μM), higher than 13-fold of the reference; monastrol (IC50 = 87.83 μM) (Fig. 3).
In addition, new pyrimidine derivatives having fatty acids residue were synthesized by alkylation of 6-oleyl-2-thiouracil and evaluated for their anticancer potency. The thiazinopyrimidine derivative 204 showed the highest anticancer activity against both cancer cell lines HEPG-2 and MCF-7 with IC50 value of 20.4 and 12.5 μg mL−1, respectively,123 (Fig. 3).
The antimicrobial activity of 4-amino-1,2,4-triazole and 1,3,4-oxadiazole derivatives were synthesized by Chehrouri et al. the results revealed that compound 205 had potent effect against Gram-positive (S. aureus, and E. faecalis), Gram-negative (E. coli, and P. aeruginosa) bacteria, and one fungus (Candida albicans)124 (Fig. 3).
Costa Cabrera and coworker obtained new dihydropyridine derivatives with potential antioxidant properties. They performed the synthesis using Hantzsch multicomponent reactions including ketoester of fatty acids, aldehydes and ammonium acetate in the presence of sulfamic acid. Their antioxidant activity was evaluated using three different methods: ABTS, DPPH, and FRAP assays. Compound 207 and 208 were the most active derivatives as antioxidant activity similar to reference drug (vitamin E, and BHT)125 (Fig. 3).
In 2021, Nengroo et al. synthesized new series of fatty acids linked with 4-methoxybenzylamides via the reaction of fatty acids with 4-methoxybenzylamine (PMBA). Compound 206 with hydroxyl group on fatty acid showed the highest antifungal activity as well as antibacterial activity126 (Fig. 3).
11. Conclusions and future perspective
The purpose of this review is to include various methods of synthesizing hybrid heterocyclic molecules with fatty acids, as well as their biological applications and evaluations. Clearly, the literature review indicates that these molecules are highly promising medicinal agents with diverse applications in industry. In addition, the presence of substituents on the heterocyclic system has a great impact on the results obtained. Hence, the incorporation of heterocyclic scaffolds with fatty acids is crucial to improving the biological and application properties. It is anticipated that the present review paper will support the development of more potent, safer, and selective candidates for potential therapeutic applications in various industries.
Conflicts of interest
The authors declare no conflict of interest.
Acknowledgements
The authors extend their appreciation to the Deanship of Scientific Research at King Khalid University for funding this work through Small Groups Project under grant number (RGP.1/346/43).
References
- U. Biermann, W. Friedt, S. Lang, W. Luhs, G. Machmuller, J. O. Metzger, M. Rush gen. Klaas, H. J. Schafer and M. P. Schneider, Angew. Chem., 2000, 112, `2292–2310 CrossRef.
- M. Jóźwiak, A. Filipowska, F. Fiorino and M. Struga, Anticancer activities of fatty acids and their heterocyclic derivatives, Eur. J. Pharmacol., 2020, 172937 CrossRef PubMed.
- M. Amiri, S. Yousefnia, F. S. Forootan, M. Peymani, K. Ghaedi and M. H. Nasr Esfahani, Diverse roles of fatty acid binding proteins (FABPs) in development and pathogenesis of cancers, Gene, 2018, 676, 171–183 CrossRef CAS PubMed.
- W. E. Connor and M. Neuringer, Biological Membranes: Aberrations in Membrane Structure and Function, ed. Karnovsky M. L., Leaf A. and Bolis L. C., Alan R, Liss, Inc., New York, 1988, pp. 275–294 Search PubMed.
- L. Hooper, N. Martin, A. Abdelhamid and G. Davey Smith, Cochrane Database Syst. Rev., 2015, 6(6), CD011737 Search PubMed.
- F. M. Sacks, A. H. Lichtenstein, J. H. Wu, L. J. Appel, M. A. Creager, P. M. Kris-Etherton, M. Miller, E. B. Rimm, L. L. Rudel, J. G. Robinson, N. J. Stone and L. V. Van Horn, Circulation, 2017, 136(3), e1–e23 CrossRef PubMed.
- A. Fawzy, R. El-Sayed, A. Al Bahir, M. Morad, I. Althagafi and K. Althagafy, Assessment of new designed surfactants as eco-friendly inhibitors for the corrosion of steel in acidic environment and evaluation of their biological and surface features: thermodynamic, kinetic and mechanistic aspects, J. Adhes. Sci. Technol., 2022, 36(18), 1993–2019 CrossRef CAS.
- H. Ilikti, T. Benabdallah, K. Bentayeb, A. A. Othman and Z. Derrich, Reduction of α,β-unsaturated ketones using a Zn/NiCl2 system in aqueous media in the presence of anionic and cationic surfactants, S. Afr. J. Chem., 2008, 61, 31–36 CAS.
- R. El-Sayed and M. H. K. Almalki, Synthesis of Five and Six-Membered Heterocycles Using Activated Nitriles for Industrial Applications, J. Oleo Sci., 2017, 66(8), 925–938 CrossRef CAS PubMed.
- D. Irby, C. Du and F. Li, Lipid–Drug Conjugate for Enhancing Drug Delivery, Mol. Pharm., 2017, 14(5), 1325–1338 CrossRef CAS PubMed.
- V. Venepally and R. J. Ram Chandra, An insight into the biological activities of heterocyclic–fatty acid hybrid molecules, Eur. J. Med. Chem., 2017, 141, 113–137 CrossRef CAS PubMed.
- R. Khaddaj-Mallat, C. Morin and E. Rousseau, Novel n-3 PUFA monoacylglycerides of pharmacological and medicinal interest: anti-inflammatory and anti-proliferative effects, Eur. J. Pharmacol., 2016, 792, 70–77 CrossRef CAS PubMed.
- R. El-Sayed and S. K. Khalid, Propoxylated fatty thiazole, pyrazole, triazole, and pyrrole derivatives with antimicrobial and surface activity, J. Surfactants Deterg., 2015, 18, 661–673 CrossRef CAS.
- T. O. Akanbi, S. N. Marshall and C. J. Barrow, Polydatin-fatty acid conjugates are effective antioxidants for stabilizing omega 3-containing bulk fish oil and fish oil emulsions, Food Chem., 2019, 125297 CrossRef CAS PubMed.
- L. Siena, et al., Electrophilic derivatives of omega-3 fatty acids counteract lung cancer cell growth, Cancer Chemother. Pharmacol., 2018, 81(4), 705–716 CrossRef CAS PubMed.
- A. M. Abdel-Mawgoud and G. Stephanopoulos, Synth. Syst. Biotechnol., 2018, 3, 3 CrossRef PubMed.
- Al-W. Tarfah, A. Sabt, E. B. Elkaeed and W. M. Eldehna, Recent advancements of coumarin-based anticancer agents: An up-to-date review, Bioorg. Chem., 2020, 103, 104163 CrossRef PubMed.
- R. Janiš, A. Klasek, J. Krejčí and J. Bobalova, Influence of some chromium complexes on the conversion rate of glycidol–fatty acid reaction, Tenside, Surfactants, Deterg., 2005, 42(1), 44–48 CrossRef.
- M. Chehrouri, A. A. Othman, S. Jiménez-Cecilia, C. Moreno-Cabrerizo and J. M. Sansano, Synth. Commun., 2019, 49, 1301–1307 CrossRef CAS.
- R. El-Sayed, Synthesis of biodegradable pyrazole, pyran, pyrrole, pyrimidine and chromene derivatives having medical and surface activities, J. Surfactants Deterg., 2016, 19, 1153–1167 CrossRef CAS.
- J. O. Metzger and U. Riedner, Fat Sci. Technol., 1989, 91, 18–23 CAS.
- J. O. Metzger and R. Mahler, Angew. Chem., 1995, 107, 1012–1016 CrossRef.
- F. J. Hidalgo and R. Zamora, In vitro production of long chain pyrrole fatty esters from carbonyl-amine reactions, J. Lipid Res., 1995, 36, 725–735 CrossRef CAS.
- H. Varshney, A. Ahmad, A. Rauf, F. M. Husain and I. Ahmad, Synthesis and antimicrobial evaluation of fatty chain substituted 2, 5-dimethyl pyrrole and 1, 3- benzoxazin-4-one derivatives, J. Saudi Chem. Soc., 2017, 21, S394–S402 CrossRef CAS.
- V. Venepally, R. B. Prasad, Y. Poornachandra, C. G. Kumar and R. C. Jala, Synthesis and biological evaluation of some new N-fatty acyl derivatives of 4,5-dimethoxy tryptamine, Indian J. Chem., 2017, 56B, 531–541 CAS.
- H. A. Abd El Salam, N. O. Shaker, E. M. El Telbani and G. A. M. Nawwar, J. Chem. Res., 2009, 40, 400–404 CrossRef.
- A. Mustafa, S. M. A. D. Zayed and S. Khattab, J. Am. Chem. Soc., 1956, 78, 145 CrossRef CAS.
- J. Mulzer, in Comprehensive Organic Functional Group Transformations, ed. Katritzky, A. R., Meth-Cohn, O. and Rees, C. W., Pergamon Press, Oxford, 1995, pp. 144–276 Search PubMed.
- H.-J. Chen, Y. Liu, L.-N. Wang, Q. Shen, J. Li and F.-J. Nan, Bioorg. Med. Chem. Lett., 2010, 20, 2876–2879 CrossRef CAS PubMed.
- K. Laskar, A. Ahmad and A. Rauf, Synthesis and spectral characterization of novel fatty acid chain substituted pyrazoline derivatives, Rasayan J. Chem., 2014, 7, 276–280 CAS.
- A. Ahmad, H. Varshney, A. Rauf, F. M. Husain and I. Ahmad, Synthesis, biological screening of novel long chain derivatives of 1,3-disubstituted-1H-pyrazol-5(4H)- one and 2-substituted-3H-1,4-phthalazin-1,4-dione: structure activity relationship studies, J. Saudi Chem. Soc., 2014, 26, 290–299 Search PubMed.
- J. A. Kenar and S. Z. Erhan, Synthesis of Δ2-isoxazoline fatty acid ester heterocycles, J. Am. Oil Chem. Soc., 2001, 78, 1045–1050 CrossRef CAS.
- J. A. Kenar, Reduction of fatty ester Δ2-isoxazoline heterocycles. Preparation of fatty esters containing the b-hydroxy ketone moiety, J. Am. Oil Chem. Soc., 2002, 79, 351–356 CrossRef CAS.
- J. A. Kenar and A. R. Wetzel, Preparation of fatty 3,5-disubstituted isoxazole compounds from FA esters, J. Am. Oil Chem. Soc., 2003, 80, 711–716 CrossRef CAS.
- A. Ahmad, A. Ahmad, H. Varshney, A. Rauf, M. Rehan, N. Subbarao and A. U. Khan, Designing and synthesis of novel antimicrobial heterocyclic analogs of fattyacids, Eur. J. Med. Chem., 2013, 70, 887–900 CrossRef CAS PubMed.
- N. K. Harn, C. J. Gramer and B. A. Anderson, Tetrahedron Lett., 1995, 36, 9453–9456 CrossRef CAS.
- S. Sharma, S. Gangal and A. Rauf, Convenient one-pot synthesis of novel 2-substituted benzimidazoles, tetrahydrobenzimidazoles and imidazoles and evaluation of their in vitro antibacterial and antifungal activities, Eur. J. Med. Chem., 2009, 44, 1751–1757 CrossRef CAS PubMed.
- E. Brous and D. Lefort, C. R. Acad. Sci., 1965, 1990–1991 Search PubMed.
- J. F. Willems and A. Vandenberghe, Bull. Soc. Chim. Belg., 1961, 70, 745–757 CrossRef CAS.
- S. Furmeier and J. O. Metzger, Eur. J. Org. Chem., 2003, 885–893 CrossRef CAS.
- K. M. Hosamani, V. B. Hiremath, R. S. Keri, R. S. Harisha and S. B. HalligudiCan, Synthesis of novel 2-alkyl substituted oleobenzimidazole derivatives using ethylene glycol as solvent, J. Chem., 2008, 86, 1030–1033 CAS.
- W. Li, Y. Lu, Z. Wang, J. T. Dalton and D. D. Miller, Synthesis and antiproliferative activity of thiazolidine analogs for melanoma, Bioorg. Med. Chem. Lett., 2007, 17, 4113–4117 CrossRef CAS PubMed.
- V. Gududuru, E. Hurh, J. Sullivan, J. T. Dalton and D. D. Miller, SAR studies of 2-arylthiazolidine-4-carboxylic acid amides: a novel class of cytotoxic agents for prostate cancer, Bioorg. Med. Chem. Lett., 2005, 15, 4010–4013 CrossRef CAS PubMed.
- Y. Lu, Z. Wang, C. M. Li, J. Chen, J. T. Dalton, W. Li and D. D. Miller, Synthesis, in vitro structure-activity relationship, and in vivo studies of 2-arylthiazolidine-4-carboxylic acid amides as anticancer agents, Bioorg. Med. Chem., 2010, 18, 477–495 CrossRef CAS PubMed.
- V. Gududuru, E. Hurh, J. T. Dalton and D. D. Miller, Discovery of 2-arylthiazolidine-4-carboxylic acid amides as a new class of cytotoxic agents for prostate cancer, J. Med. Chem., 2005, 48, 2584e2588 CrossRef PubMed.
- A. Rauf, S. Gangal, S. Sharma and M. Zahin, A simple, rapid and efficient one-pot protocol for the synthesis of 2-substituted benzothiazole derivatives and their antimicrobial screening, S. Afr. J. Chem., 2008, 61, 63–67 CAS.
- H. Varshney, A. Ahmad, N. N. Farshori, A. Ahmad, A. U. Khan and A. Rauf, Synthesis and evaluation of in vitro antimicrobial activity of novel 2,3-disubstituted-4-thiazolidinones from fatty acid hydrazides, Med. Chem. Res., 2013, 22, 3204 CrossRef CAS.
- A. M. F. Eissa, Anionic surface active agents from fatty acid hydrazides containing heterocyclic moiety, Olaj, Szappan, Kozmet., 2002, 51, 155–161 CAS.
- A. Rauf, S. Sharma and S. Gangal, One-pot synthesis, antibacterial and antifungal activities of novel 2,5-disubstituted-1,3,4-oxadiazoles, Chin. Chem. Lett., 2008, 19, 5–8 CrossRef CAS.
- N. N. Farshori, M. R. Banday, A. Ahmad, A. U. Khan and A. Rauf, Synthesis, characterization, and in vitro antimicrobial activities of 5-alkenyl/hydroxyalkenyl- 2-phenylamine-1,3,4-oxadiazoles and thiadiazoles, Bioorg. Med. Chem. Lett., 2010, 20, 1933–1938 CrossRef CAS PubMed.
- M. R. Banday, R. H. Mattoo and A. Rauf, Synthesis, characterization and antibacterial activity of 5-(alkenyl)-2-amino- and 2-(alkenyl)-5-phenyl-1,3,4- oxadiazoles, J. Chem. Sci., 2010, 122, 177–182 CrossRef CAS.
- H. M. Soliman and Y. El-Shattory, Separation of Palmitic Acid from over Used Oil for Production of Heterogeneous Organic Derivatives of Potential Biological Activities, Egypt. J. Chem., 2017, 60(No. 4), 591–600 Search PubMed.
- S. F. Marcel, L. K. Jie, M. K. Pasha and M. Shahin Alam, Synthesis of novel triazole fatty acid derivatives from acetylenic fatty esters, Chem. Phys. Lipids, 1998, 91, 71–78 CrossRef.
- A. Rauf and S. Gangal, Facile one-pot synthesis of N-acyl-1H-1,2,3-benzotriazoles from internal and terminal olefinic fatty acids and their antimicrobial screening, J. Oleo Sci., 2008, 57, 453–457 CrossRef CAS PubMed.
- Z. Rezaei, S. Khabnadideh, K. Pakshir, Z. Hossaini, F. Amiri and E. Assadpour, Design, synthesis and antifungal activity of triazole and benzotriazole derivatives, Eur. J. Med. Chem., 2009, 44, 3064–3067 CrossRef CAS PubMed.
- M. R. Banday and A. Rauf, Substituted 1,2,4-triazoles and thiazolidinones from fatty acids: Spectral characterization and antimicrobial activity, Indian J. Chem., 2009, 48B, 97–102 CAS.
- D. G. Ghiano, A. de la Iglesia, N. Liu, P. J. Tonge, H. R. Morbidoni and G. R. Labadie, Antitubercular activity of 1,2,3-triazolyl fatty acid derivatives, Eur. J. Med. Chem., 2017, 125, 842–852 CrossRef CAS PubMed.
- C. Menendez, A. Chollet, F. Rodriguez, C. Inard, M. R. Pasca, C. Lherbet and M. Baltas, Chemical synthesis and biological
evaluation of triazole derivatives as inhibitors of InhA and antituberculosis agents, Eur. J. Med. Chem., 2012, 52, 275–283 CrossRef CAS PubMed.
- D. Kumar, B. Negi, G. Khare, S. Kidwai, A. K. Tyagi, R. Singh and D. S. Rawat, Synthesis of novel 1,2,3-triazole derivatives of isoniazid and their in vitro and in vivo antimycobacterial activity evaluation, Eur. J. Med. Chem., 2014, 81, 301–313 CrossRef CAS PubMed.
- J. M. Xu, E. Zhang, X. J. Shi, Y. C. Wang, B. Yu, W. W. Jiao, Y. Z. Guo and H. M. Liu, Synthesis and preliminary biological evaluation of 1,2,3-triazole-Jaspine B hybrids as potential cytotoxic agents, Eur. J. Med. Chem., 2014, 80, 593–604 CrossRef CAS PubMed.
- G. R. Labadie, A. de la Iglesia and H. R. Morbidoni, Targeting tuberculosis through a small focused library of 1,2,3-triazoles, Mol. Diversity, 2011, 15, 1017–1024 CrossRef CAS PubMed.
- S. Jubie, P. N. Ramesh, P. Dhanabal, R. Kalirajan, N. Muruganantham and A. S. Antony, Synthesis, antidepressant and antimicrobial activities of some novel stearic acid analogues, Eur. J. Med. Chem., 2012, 54, 931–935 CrossRef CAS PubMed.
- K. Koguro, T. Oga, S. Mitsui and R. Orita, Synthesis, 1998, 910–914 CrossRef CAS.
- R. Huisgen, J. Sauer, H. J. Sturm and J. H. Markgraf, Chem. Ber., 1960, 93, 2106–2124 CrossRef CAS.
- H. Suzuki, Y. S. Hwang, C. Nakaya and Y. Matano, Synthesis, 1993, 1218–1220 CrossRef CAS.
- J. O. Metzeger, R. Mahler and G. Francke, Liebigs Ann./Recl., 1997, 2303–2313 CrossRef.
- V. Venepally, R. B. Prasad, Y. Poornachandra, C. G. Kumar and R. C. Jala, Synthesis of novel ethyl 1-ethyl-6-fluoro-7-(fatty amido)-1,4-dihydro-4-oxoquinoline-3- carboxylate derivatives and their biological evaluation, Bioorg. Med. Chem. Lett., 2016, 26, 613–617 CrossRef CAS PubMed.
- D. Malinak, R. Dolezal, J. Marek, S. Salajkova, O. Soukup, M. Vejsova, J. Korabecny, J. Honegr, M. Penhaker, K. Musilek and K. Kuca, 6-Hydroxyquinolinium salts differing in the length of alkyl side-chain: synthesis and antimicrobial activity, Bioorg. Med. Chem. Lett., 2014, 24, 5238–5241 CrossRef CAS PubMed.
- M. D. Rozwadowska, Heterocycles, 1994, 39, 903–931 CrossRef CAS PubMed.
- M. Chrzanowska and M. D. Rozwadowska, Chem. Rev., 2004, 7, 3341–3370 CrossRef PubMed.
- A. J. Mancuso and D. Swern, Synthesis, 1981, 165–185 CrossRef CAS.
- Z. Czarnocki, D. B. MacLean and W. A. Szarek, Bull. Soc. Chim. Belg., 1986, 95, 749–770 CrossRef CAS.
- Z. Czarnocki, M. P. Matuszewska and I. Matuszewska, Org. Prep. Proced. Int., 1998, 30, 699–702 CrossRef CAS.
- I. Matuszewska, A. Leniewski, P. Roszkowski and Z. Czarnocki, Chem. Phys. Lipids, 2005, 135, 131–145 CrossRef CAS PubMed.
- D. da Costa Cabrera, S. B. Rosa, F. S. de Oliveira, M. A. G. Marinho, C. R. Montes D'Oca, D. Russowsky, A. P. Horn and M. G. Montes D'Oca, Synthesis and antiproliferative activity of novel hybrid 3-substituted polyhydroquinoline-fatty acids, MedChemComm, 2016, 7, 2167 RSC.
- S. Balasubramanian and M. G. Nair, Synth. Commun., 2000, 30, 469 CrossRef CAS.
- H. Xiang, W. H. Xiang, W. Fang, L. He, L. Y. Zhang and Q. J. Liao, Synthesis of daidzein derivatives and their binding affinities to estrogen receptor, J. China Pharm. Univ., 2006, 37, 23–27 CAS.
-
(a) H. Xiang, W. Zhao, H. Xiao, L. Qian, Y. Yao, X.-B. Li and Q.-J. Liao, Bioorg. Med. Chem., 2010, 18, 3036–3042 CrossRef CAS PubMed;
(b) M. P. Singh, F. Kong, G. E. Janso, D. A. Arias, P. A. Suarez, V. S. Bernan, P. J. Petersen, W. J. Weiss, G. Carter and M. J. Greenstein, Antibiotics, 2003, 56, 1033–1044 CrossRef CAS PubMed.
- M. P. Singh, F. Kong, G. E. Janso, D. A. Arias, P. A. Suarez, V. S. Bernan, P. J. Petersen, W. J. Weiss, G. Carter and M. J. Greenstein, Antibiotics, 2003, 56, 1033–1044 CrossRef CAS PubMed.
- F. Kong, M. P. Singh and G. T. Carter, J. Nat. Prod., 2005, 68, 920–923 CrossRef CAS PubMed.
- Y. Oikawa, K. Sugano and O. Yonemitsu, J. Org. Chem., 1978, 43, 2087–2088 CrossRef CAS.
- F. S. Pashkovsky, I. P. Lokot and F. A. Lakhvich, Synlett, 2001, 1391–1394 CrossRef CAS.
- A. C. Giddens, L. Nielsen, H. I. Boshoff, D. Tasdemir, R. Perozzo, M. Kaiser, F. Wang, j. C. Sacchettini and B. R. Coop, Tetrahedron, 2008, 64, 1242–1249 CrossRef CAS.
- M. J. Robins and P. W. Hatfield, Can. J. Chem., 1982, 60, 547–553 CrossRef CAS.
- X. H. Xu, H. M. Chen and R. Y. Chen, Chem. J. Chin. Univ., 2000, 21, 1410 CAS.
- K. Bouhadir, J. Zhou and P. Shevlin, Synth. Commun., 2005, 35, 1003 CrossRef CAS.
- L. Vares, A. Koulov and B. Smith, J. Org. Chem., 2003, 68(26), 10073 CrossRef CAS PubMed.
- D. Brown, A. Todd and S. Varadarajan, J. Chem. Soc., 1956, 2384 RSC.
- O. Mitsunobu, Synthesis, 1981, 1 CrossRef CAS.
- T. Jenny and S. Benner, Tetrahedron Lett., 1992, 33, 6619 CrossRef CAS.
- T. Jenny, N. Previsani and S. Benner, Tetrahedron Lett., 1991, 32, 7029 CrossRef CAS.
- J. Zhou and P. Shevlin, Tetrahedron Lett., 1998, 39, 8373 CrossRef CAS.
- R. Shatila and K. Bouhadir, Tetrahedron Lett., 2006, 47, 1767 CrossRef CAS.
- H. H. Hammud, A. M. Ghannoum, F. A. Fares, L. K. Abramian and K. H. Bouhadir, J. Mol. Struct., 2008, 881, 11–20 CrossRef CAS.
- Y. Mikata, Y. Shinohara, K. Yoneda, Y. Nakamura, K. Esaki, M. Tanahashi, I. Brudzinska, S. Hirohara, M. Yokoyama, K. Mogami, T. Tanase, T. Kitayama, K. Takashiba, K. Nabeshima, R. Takagi, M. Takatani, T. Okamoto, I. Kinshita, M. Doe, A. Hamazawa, M. Morita, F. Nishida, T. Sakakibara, C. Orvig and Y. Shigenobu, J. Org. Chem., 2001, 66, 3783–3789 CrossRef CAS PubMed.
- Y. Chen, A. Janczuk, X. Chen, J. Wang, M. Kesebati and P. G. Wang, Carbohydr. Res., 2002, 337, 1043–1046 CrossRef CAS PubMed.
- Y. G. Gololobov, N. I. Gusar and M. P. Chaus, Tetrahedron, 1985, 41, 793–799 CrossRef CAS.
- H. Takeuchi, S. Hagiwara and S. Eguchi, Tetrahedron, 1989, 45, 6375–6386 CrossRef CAS.
- L. Wu and K. Burgess, J. Am. Chem. Soc., 2008, 120, 4089–4096 CrossRef PubMed.
- S. L. Aspinall, J. Am. Chem. Soc., 1940, 62, 2160–2162 CrossRef CAS.
- G. S. Skinner and P. R. Wunz, J. Am. Chem. Soc., 1951, 73, 3814–3815 CrossRef CAS.
- R. W. Brimblecombe, R. R. Hunt, R. L. Rickard and J. V. Taylor, Br. J. Pharmacol., 1969, 37, 425–435 CrossRef CAS PubMed.
- S. M. Salman, T. Heidelberg and H. A. B. Tajuddin, Carbohydr. Res., 2013, 375, 55–62 CrossRef CAS PubMed.
- T. G. Treptow, F. Figueiro, E. H. Jandrey, A. M. Battastini, C. G. Salbego, J. B. Hoppe, P. S. Taborda, S. B. Rosa, L. A. Piovesan, R. Montes D'Oca Cda, D. Russowsky and M. G. Montes D'Oca, Novel hybrid DHPM-fatty acids: synthesis and activity against glioma cell growth in vitro, Eur. J. Med. Chem., 2015, 95, 552–562 CrossRef CAS PubMed.
- D. Kumarasamy, B. G. Roy, J. Rocha-Pereira, J. Neyts, S. Nanjappan, S. Maity, M. Mookerjee and L. Naesens, Synthesis and in vitro antiviral evaluation of 4- substituted 3,4-dihydropyrimidinones, Bioorg. Med. Chem. Lett., 2017, 27, 139–142 CrossRef CAS PubMed.
- A. M. F. Eissa and R. El-Sayed, J. Heterocycl. Chem., 2006, 43, 1161–1168 CrossRef CAS.
- A. Rauf, S. Sharma and S. Gangal, ARKIVOC, 2007, xvi, 137–147 Search PubMed.
- L. F. Fieser and M. Fieser, Reagents for Organic Synthesis, John Wiley and Sons, Inc, London, 1967, p. 233 Search PubMed.
- M. aus dem Kahmen and H. J. Schafer, Fett/Lipid, 1998, 100, 227–235 CrossRef CAS.
- J.-F. Michelet, M. Dalko, B. Bernard, D. Semeria and M. Philippe, US Pat., US2004/38912 A1, 2004 Search PubMed.
- L. Simeone, G. Mangiapia, C. Irace, A. Di Pascale, A. Colonna, O. Ortona, L. De Napoli, D. Montesarchio and L. Paduano, Mol. BioSyst., 2011, 7, 3075–3086 RSC.
- L. Simeone, C. Irace, A. Pascale, D. Ciccarelli, G. D'Errico and D. Montesarchio, Eur. J. Med. Chem., 2012, 57, 429–440 CrossRef CAS PubMed.
- Ziaullah and H. P. V. Rupasinghe, Tetrahedron Lett., 2013, 54, 1933–1937 CrossRef CAS.
- A. Frump, Chem. Rev., 1971, 71, 483–525 CrossRef.
- P. Sallary, S. Bekassy, M. H. Ahmed, I. Farkas and I. Rusznak, Tetrahedron Lett., 1997, 38, 661–664 CrossRef.
- S. Pegiadous, L. Perez and M. R. Infant, J. Surfactants Deterg., 2001, 2, 517–525 Search PubMed.
- M. Abdallah, F. H. Al-abdali, E. M. Kamar, R. El-Sayed and R. S. Abdel Hameed, Corrosion inhibition of aluminum in 1.0M HCl solution by some nonionic surfactant compounds containing five membered heterocyclic moiety, Chem. Data Collect., 2020, 28, 100407 CrossRef CAS.
- M. Aimal, D. Jamal and M. A. Quraishi, Anti-Corros. Methods Mater., 2000, 47, 77–82 CrossRef.
- B. S. Chhikara, N. S. Jean, D. Mandal, A. Kumar and K. Parang, Eur. J. Med. Chem., 2011, 46, 2037–2042 CrossRef CAS PubMed.
- Y. Bo-Tao, S. Xun and Z. Zhi-Rong, Arch. Pharmacal Res., 2003, 26(12), 1096–1101 CrossRef PubMed.
- A. Abdelmajeid, M. Saad Amine and R. Ali Hassan, Fatty Acids in Heterocyclic Synthesis: Part XIX Synthesis of Some Isoxazole, Pyrazole, Pyrimidine and Pyridine and Their Surface, Anticancer and Antioxidant Activities, Am. J. Heterocycl. Chem., 2018, 4(2), 30–41 CrossRef.
- F. S. De Oliveira, P. M. De Oliveira, L. M. Farias, R. C. Brinkerhoff, R. C. M. A. Sobrinho, T. M. Treptow, C. R. Montes D'Oca, M. A. G. Marinho, M. A. Hort, A. P. Horn, D. Russowsky and M. G. Montes D'Oca, Synthesis and antitumoral activity of novel analogues monastrol–fatty acids against glioma cells, MedChemComm, 2018 Aug 1, 9(8), 1282–1288 Search PubMed.
- E.-S. M. A. Yakout, H. A. Abd El Salam and G. A. M. Nawwar, Bioactive Small Molecules Having a Fatty Residue. Part VI: Synthesis, Cytotoxicity Evaluation, and Molecular Docking Studies of New Pyrimidine Derivatives as Antitumor Agents, Russ. J. Org. Chem., 2020, 56, 2212–2221 CrossRef CAS.
- M. Chehrouri, A. A. Othman, C. Moreno-Cabrerizo, M. Gholinejad and J. M. Sansano, Synthesis of 5-heptadecyl- and 5-heptadec-8-enyl substituted 4-amino-1,2,4-triazole-3-thiol and 1,3,4-oxadiazole-2-thione from (Z)-octadec-9-enoic acid: preparation of Palladium(II) complexes and evaluation of their antimicrobial activity, Monatsh. Chem., 2020, 151, 173–180 CrossRef CAS.
- Z. R. Nengroo, A. Ahmad, A. Tantary, A. Shafi Ganie and Z. Shah, Design and synthesis of fatty acid derived 4-methoxybenzylamides as antimicrobial agents, Heliyon, 2021, 7, e06842 CrossRef CAS PubMed.
- D. da Costa Cabrera, E. Santa-Helena, H. P. Leal, R. R. de Moura, L. E. M. Nery, C. A. N. Gonçalves, D. Russowsky and M. G. Montes D'Oca, Synthesis and antioxidant activity of new lipophilic dihydropyridines, Bioorg. Chem., 2018, 84, 1–16 CrossRef PubMed.
|
This journal is © The Royal Society of Chemistry 2023 |
Click here to see how this site uses Cookies. View our privacy policy here.