DOI:
10.1039/D3RA01336G
(Review Article)
RSC Adv., 2023,
13, 14317-14339
An overview of the synthetic strategies of C3-symmetric polymeric materials containing benzene and triazine cores and their biomedical applications
Received
27th February 2023
, Accepted 28th April 2023
First published on 10th May 2023
Abstract
C3-symmetric star-shaped materials are an emerging category of porous organic polymers with distinctive properties such as permanent porosity, good thermal and chemical stability, high surface area, and appropriate functionalization that promote outstanding potential in various applications. This review is mostly about constructing benzene or s-triazine rings as the center of C3-symmetric molecules and using side-arm reactions to add functions to these molecules. Over and above this, the performance of various polymerization processes has been additionally investigated in detail, including the trimerization of alkynes or aromatic nitriles, polycondensation of monomers with specific functional groups, and cross-coupling building blocks with benzene or triazine cores. Finally, the most recent progress in biomedical applications for C3-symmetric materials based on benzene or s-triazine have been summarized.
| Maha A. Alshubramy is a PhD candidate in the Chemistry department at King Abdulaziz University. She obtained her master's degree in organic synthesis from Qassim University in 2020. Her research focuses on synthesizing small molecules and building blocks for functionalized polymeric compounds with nanomaterials. |
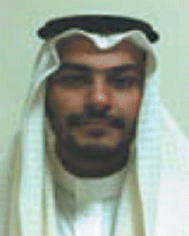 Khalid A. Alamry | K. A. Alamry is a professor of Polymer Chemistry, at the Chemistry Department, Faculty of Science, King Abdulaziz University (KAU), Jeddah, Saudi Arabia. He obtained his PhD in Polymer Chemistry from The University of Manchester in UK in 2010. He published around 300 ISI papers in the field of polymers. His research interest is focused on biopolymers from synthesis to applications for variable applications. |
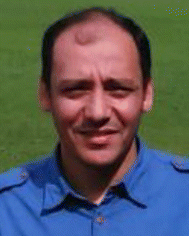 Mahmoud A. Hussein | M. A. Hussein is a professor of Polymer Chemistry, Polymer Chemistry Lab, Chemistry Department, Faculty of Science, Assiut University (AU), Egypt. He obtained his PhD in Organic Polymer Synthesis from Assiut University, Egypt in 2007. He got a position at the Chemistry Department, King Abdulaziz University (KAU), Jeddah, Saudi Arabia from 2010 – till now. He got postdoctoral positions in the University of Nice Sophia Antipolis, France and University Sains Malaysia, Malaysia. He visited the School of Industrial Technology, University Sains Malaysia and Faculty of Engineering, University of Porto (UP) as a visiting researcher. He published more than 200 ISI papers and numerous conference papers (posters and oral). His research interests are in the area of polymer synthesis, characterization and applications in different fields, polymer composite materials, polymer-doped organic and/or inorganic substances for variable industrial as well as biological interests. |
1. Introduction
Geometry in materials science relies heavily on the symmetry of molecular building components. In particular, C3-symmetric geometries are present in star-shaped molecules, dendrimers, molecular cages, metal–organic frameworks (MOFs), and covalent organic frameworks (COFs).1–5 C3-symmetric conjugated aromatic compounds have applications ranging from optoelectronic and self-assembling systems to liquid crystals, mesogens, and nonlinear material optics.6–8 Notably, C3-symmetric molecules have good physicochemical characteristics, making them useful as active building blocks in electronic devices, including organic field-effect transistors (OFETs), organic photovoltaic devices (OPVs), organic solar cells (OSCs), and organic light-emitting diodes (OLEDs).9–12 Further, C3-symmetric star-shaped molecules are used in various biomedical and pharmaceutical applications. For example, it was recently designed to use old drugs that function as tripod scaffold arms or as carriers of medicines with biologically active molecules immobilized on the star arms. In addition, C3-symmetric star-shaped molecules are applied in imaging cancer cells through fluorescence, as potent allosteric antagonists of P2X receptors, and in synthetic transport systems, besides their biological activities as antioxidants and antibacterials.13–17 In view of these interesting applications, numerous C3-symmetric aromatic molecules have piqued the interest of synthetic chemists and opened up new avenues for synthesis. Synthesis of certain molecules might begin with aldol-like events involving condensation of carbonyl compounds, cyclotrimerization reactions involving alkynes, or the functionalization of appropriately geared building blocks.
Therefore, a comprehensive view summarizing these compounds' synthetic strategies and polymerization techniques is worthwhile to provide a well-organized and methodical overview. To the best of our knowledge, several other reviews have emerged concerning the chemistry of star-shaped molecules and triazine-based organic framework. However, particular attention to synthesizing C3-symmetric compounds based on benzene or s-triazine cores in an organized manner to construct the core, the reaction of side arms, as well as polymerization techniques have yet to be reported. Given this, we have covered the literature to give an example of each method and discussed the synthetic strategies in a simplified manner to provide a better understanding for future researchers to modify these methods.
2. Formation of the core by different trimerization reactions
2.1. [2+2+2] Cyclotrimerization reactions
The transition-metal-catalyzed [2+2+2] cyclotrimerization of alkynes is considered the most classical method for efficiently synthesizing benzenes and analogous aryl derivatives.18–20 Mainly used catalysts consisted of Ti, Zr, Mo, Fe, Ru, Co, Rh, Ir, Ni, and Pd; however, the main challenges are chemo and regioselectivity issues, which generally result in a complex mixture of products.21,22 A limited amount of literature discovered methods to solve this problem, such as Grubbs-catalyzed 2-propynyl derivatives in DCM gave a mixture of products. Similarly, Kotha et al. exploited the ruthenium-catalyzed [2+2+2] cyclotrimerization reaction to generate star-shaped compounds that form various compounds, the depropargylated product and 1,2,4-trisubstituted with 1,3,5-trisubstituted, which was hard to purify by column chromatography (Table 1, entry 1-a).23
Table 1 Synthetic strategies for cores construction by different trimerization reactions
Entry |
Type of trimerization |
Reaction |
Reaction conditions |
Ref. |
1 |
[2+2+2] Cyclotrimerization reactions |
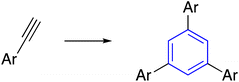 |
 |
23 |
(a) G-1, toluene, 80 °C, 24–54 h |
(b) B2(OH)4, NiCl2·DMF, CH3CN, 80 °C, 12 h |
24 |
(c) P4VP-CoCl2 II, DIPEA, 150 °C, 24 h, N2 |
25 |
2 |
Alkene |
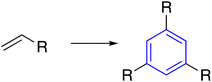 |
(a) Pd(OAc)2, HPMo8V4, CeCl3 |
26 |
(b) PdCl2, O2, DMF |
27 |
(c) Pd(OAc)2, RT |
29 |
3 |
Acetyl arenes |
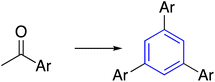 |
(a) DBSA, m-xylene, 130 °C, 24 h. SOCl2, EtOH, 80 °C, 8 h |
34 |
(b) SiCl4, EtOH, 0 °C to RT, 24 h |
35 |
(c) CF3SO3H, toluene, 110 °C, 8 h |
36 |
(d) Triethyl orthoformate |
37 |
(e) H3PW12O40 MW |
38 |
(f) Ethylenediamine TFA, 24 h, CH3NO2 |
39 |
4 |
Nitrile |
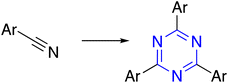 |
CF3SO3H, CHCl3, 0 °C, 24 h |
40 |
5 |
Alkynyl ketone |
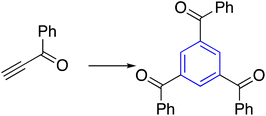 |
DMF, toluene, 48 h |
41 |
6 |
Condensation reaction |
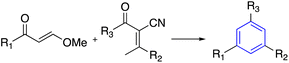 |
(a) DBU, THF, RT |
46 |
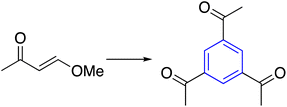 |
(b) Water, 150 °C |
47 |
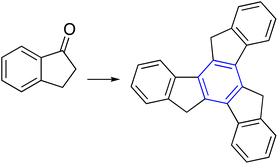 |
(c) TiCl4, Cl(CH2)4Cl, 164 °C, 30 min |
51 |
Nevertheless, K. Yang and co-workers recently reported nickel-catalyzed regioselective cyclotrimerization of terminal alkynes in good yields to synthesize C3-symmetry benzenes. This process features a cheap catalyst and mild reaction conditions; likewise, the NiB(OH)2 complex is generated in situ from NiCl2·DME and tetrahydroxydiboron could act as active catalysts. The hydrogen bonding between the hydroxyl groups on boron and the terminal alkyne plays an essential role in controlling the regioselectivity by orienting the coordination of the terminal alkynes to the nickel center (Table 1, entry 1-b).24
On top of that, polymer-assisted cobalt catalysis was used to synthesize triarylbenzenes with C3-symmetry through the [2+2+2] cyclotrimerization of terminal aryl alkynes. A complex poly(4-vinylpyridine)-cobalt(II) system was used as a stable and reusable heterogeneous catalyst. The cobalt catalyst showed the highly selective formation of 1,3,5-triarylbenzene without forming 1,2,4-triarylbenzene. Moreover, the polymer-supported catalyst was successfully recovered and used three times (Table 1, entry 1-c).25
2.2. Alkenes with metal-catalysts
Palladium-catalyzed formation of tri-substituted benzene derivatives with C3-symmetry from alkenes bearing electron-withdrawing groups has been reported frequently.26–29 Tamaso et al. developed a triannelation reaction by Pd(OAc)2, HPMo8V4, acetalization of acrylate with methanol, followed by trimerization reaction of the acetal by CeCl3 (Table 1, entry 2-a).26 Withal, a facile palladium catalyst system was developed for the highly regioselective cyclotrimerization of olefins bearing electron-withdrawing groups in PdCl2/O2/DMF (Table 1, entry 1-b).27 Furthermore, S. Midya reported a room-temperature catalytic pathway for benzene derivatives with C3-symmetry from nitroalkenes in the presence of Pd(OAc)2 (Table 1, entry 2-c).29
2.3. Acetyl arenes
The trimerization reaction was achieved through the use of acetyl arenes in acid-catalyzed. The cyclization occurs through a variety of catalysts, such as thionyl chloride SOCl2, 4-dodecylbenzene sulfonic acid DBSA, triflic acid CF3SO3H,30 phosphoric acid,31 titanium tetrachloride TiCl4 (ref. 32) and silicon tetra-chloride SiCl4.33
Shimasaki et al. established the reaction of acetyl arenes with DBSA in xylene at 130 °C for 1 day to afford the corresponding trimerized products in 66% yield. Equally, the product was obtained in 52% yield using thionyl chloride in EtOH at 80 °C for 8 h or silicon tetrachloride in EtOH at room temperature for 15 h (60% yield) (Table 1, entry 3-a).34 Also, the treatments of various acetylated thiophene derivatives with SiCl4 in EtOH afforded the same thiophene-containing star-shaped molecule (Table 1, entry 3-b).35 Okumoto and Shirota reported a CF3SO3H-catalyzed trimerization reaction to synthesize a benzene core from 4-acetylbiphenyl (Table 1, entry 3-c).36 Moreover, triethyl orthoformate was utilized to generate C3-symmetric building blocks from bromoacetophenone (Table 1, entry 3-d).37 Ramshini et al. developed a triple condensation reaction of aryl methyl ketones catalyzed by (H6P2W18O62)/NCP under solvent-free conditions (Table 1, entry 3-e).38 Additionally, ethylenediamine and trifluoroacetic acid were reported to afford 1,3,5-triaryl benzenes from aryl methyl ketones under mild conditions in good yields (Table 1, entry 3-f).39 On the other hand, Kotha and the team treated thiophene-2-carbonitrile with F3CSO3H in dry chloroform at room temperature, generating the s-triazine as the central core in 85% yield (Table 1, entry 4).40
2.4. Alkynyl ketone
Alkynyl ketones, such as benzoylacetylene derivatives, were trimerized by organocatalytic or thermally induced for synthesizing the corresponding benzene core (Table 1, entry 5). Pigge et al. approached (4-nitrobenzoyl) acetylene in DMF and toluene to deliver C3-symmetry 1,3,5-tris(4-nitrobenzoyl) benzene in 72% yield. Furthermore, Hiaki and co-workers explored the metal-free method by studying the reaction mechanism; the researchers discovered that enolate is the essential intermediate in the trimerization of alkynyl ketone to construct 1,3,5-tribenzoylbenzene under high pressure and hot water.41–45
2.5. Carbonyl compounds
Benzene as a central core was synthesized by [2+4] annulation of α,β-unsaturated carboxylic acids, or α-cyano-β-methylenes to obtain the C3-symmetry compounds. The α,β-unsaturated compound was activated via DBU as the significant step in assembling the benzene ring, which worked as Brønsted base and nucleophile (Table 1, entry 6-a).46
More than that, the ability of enaminones to furnish C3-symmetry benzenes via cyclotrimerization in excellent yield and high regioselectivity was introduced. However, the reaction usually works under acidic media or with additives like pyridine in a number of cases. Recently, more environmentally friendly cyclotrimerized enaminones used water as the solvent and a small amount of lactic acid as a catalyst (Table 1, entry 6-b).47–50
The cyclotrimerization of cyclic ketones through an aldol trimerization reaction is a powerful tool for synthesizing tris-fused benzene rings. Aaron and co-workers studied certain factors that can enhance cyclotrimerizations methodology. The factor that played a substantial role in successful aldol cyclotrimerization is dehydration of the starting aldol dimer to α,β-unsaturated ketone, while β,γ-unsaturated eliminates any possibility of producing the cyclic trimer (Table 1, entry 6-c).51,52 The following Table 1 presents a summary of the above techniques.
3. Functionalized side arms
3.1. Van Leusen oxazoles reaction
A Van Leusen oxazole reaction was utilized to synthesize star-shaped oxazole derivatives. The trisaldehyde derivative reacted with tosylmethyl isocyanide (TosMIC) in the presence of potassium carbonate and MeOH to afford the oxazole-containing star-shaped molecule. The designed trialdhyde was formed in two ways, tribromo with nBuLi and N-formylpiperidine or formylation of tristhiophene derivative by Vilsmeier–Haack reaction using POCl3 in DMF (Table 2, entry 1).53,54
Table 2 Reactions of said arms of C3-symmetric star-shaped small compound
Entry |
Type of reaction |
Reaction |
Reaction condition |
Ref. |
1 |
Van leusen oxazoles |
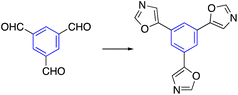 |
TosMIC, K2CO3, MeOH, reflux |
53 |
2 |
Wittig reaction |
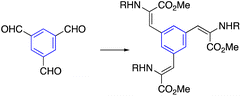 |
 |
55 |
N,N,N′,N′-Tetramethyl ethylenediamine, THF, 24 h |
3 |
Condensation reaction |
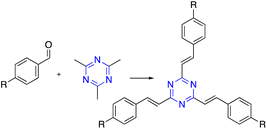 |
KOH, MeOH, 3–6 days |
56 |
4 |
Metathesis reactions |
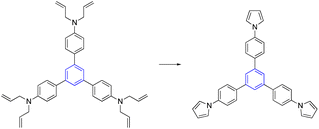 |
 |
58 |
G-1 |
CH2Cl2, 2 h |
5 |
Suzuki–Miyaura cross-coupling |
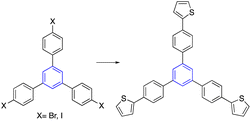 |
(a) Thiophene-2-boronic acid, Pd(PPh3)4, THF: Toluene, Na2CO3, 90 °C |
35 |
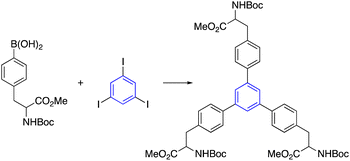 |
(b) Pd(PPh3)4, THF, toluene, Na2CO3, 90 °C |
61 |
6 |
Heck cross-coupling |
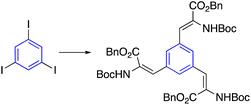 |
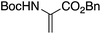 |
55 |
(a) NaHCO3, Bu4NCl, Pd(OAc)2, DMF, 80 °C 24 h |
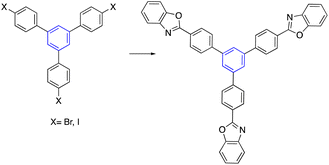 |
(b) Benzoxazoe in [Pd(OAc)2]/PPh3 |
63 |
7 |
Negishi cross-coupling |
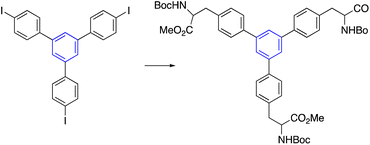 |
 |
1 |
Pd(PPh3)4, DMF, 80 °C, 12 h |
8 |
Stille cross-coupling |
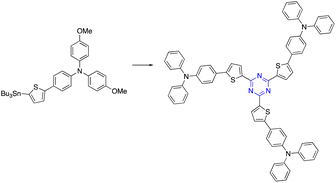 |
Cyanuric chloride, Pd(PPh3)4, toluene, 110 °C, 4 days |
64 |
9 |
Sonogashira cross-coupling |
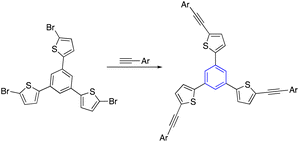 |
Pd(PPh3)4, PPh3, Et3N, CuI, 100°C, 3 days |
65 |
3.2. Wittig and related olefination
Star-shaped with alkene side arms are synthesized by an olefination in which aldehydes or ketones are reacted with phosphorus ylides to produce alkene. Frejd and co-workers examined a procedure to develop star-shaped derivatives based on amino acid (Table 2, entry 2).55 To create trifunctional alkenes, Horner–Wadsworth–Emmons olefination was used. As a result of reacting 1,3,5-triformylbenzene with the Wittig reaction, a 54% yielding olefination product was obtained. Moreover, the aldol condensation is employed to form alkene in the side arms of the star-shaped compound through a condensation reaction of aldehyde and active methyl. Meier et al. Treated 2,4,6-trimethyl-1,3,5-triazine with various benzaldehydes in potassium hydroxide and methanol to obtain a tri-condensation product (Table 2, entry 3).56
3.3. Metathesis reactions
In order to get at the pyrrole-based star-shaped molecule, ring-closing metathesis (RCM) was employed.57–59 Kotha et al. demonstrated three different routes for synthesized pyrrole-based star-shaped C3-symmetric. RCM, Buchwald–Hartwig cross-coupling reaction, and Clauson–Kaas pyrrole synthesis were utilized as key steps. Interestingly, the authors employed a G-I catalyst for a one-pot ring-closing metathesis RCM and aromatization sequence (Table 2, entry 4).58
3.4. Suzuki–Miyaura cross-coupling reactions
Kotha et al. reported a procedure for synthesizing star-shaped molecules through trimerization and Suzuki–Miyaura (SM) cross-coupling reactions. First, the acetyl thiophene refluxed with ethylenediamine in TFA and nitromethane to give the trimerized compound. Subsequent bromination by NBS provided the tribromo product, which utilized the SM cross-coupling reaction to provide star-shaped derivatives. Practically note that the tribromo compound was not formed by directly trimerized 2-acetyl-4-bromofuran. Instead, the compound was obtained by brominating the corresponding aromatic compound with NBS in DMF at room temperature (Table 2, entry 5-a).35,60
It has also been reported that star-shaped alpha-amino acid derivatives can be produced by the trimerization of an acetyl molecule, which is then accompanied by an SM cross-coupling process. In this regard, trihalo compounds synthesized from trimerization reactions gave star-shaped AAA derivatives for SM cross-coupling with AAA-containing boronic acid (Table 2, entry 5-b).61,62
3.5. Heck cross-coupling reactions
Synthesized star-shaped trihalo compounds were exploited in the Heck cross-coupling reaction to introduce a heterocycle into the star-shaped molecule. This method has been used to synthesize star-shaped molecules with benzoxazole, benzothiazole, and 1-methyl benzimidazole groups on their edges by palladium-catalyzed and K2CO3 or Cs2CO3 to produce the desired designed heterocycle (Table 2, entry 6-b).63
By using either the Horner–Wadsworth–Emmons olefination or the Heck cross-coupling reactions, Frejd and his team successfully produced star-shaped amino acid analogs with three different functions. Within the context of this investigation, a Heck cross-coupling led to the formation of a trifunctional amino acid analog. The reaction of triiodobenzene with acrylate in the presence of palladium acetate provided the coupled product. In contrast, the hydrogenation of olefin was carried out with a rhodium catalyst to give the corresponding star-shaped amino acid derivative in a good yield (Table 2, entry 6-a).55
3.6. Negishi cross-coupling reactions
Besides, palladium-catalyzed was employed through Negishi cross-coupling reactions to construct star-shaped molecules, which formed an established step to build the tris-AAA derivative. First, the 4-iodoacetophenone trimerization yielded the triiodo derivative. The resultant trimerized synthon was then used in a Negishi cross-coupling with palladium as a catalyst in DMF at 80 °C, yielding a compound with a 68% yield (Table 2, entry 7).1
3.7. Stille cross-coupling reactions
Using a Stille cross-coupling reaction between a tribromo compound and tributylstannyl analogs, we could study synthetic triazine-based star-shaped structures with C3-symmetry. Using cyanuric chloride and the proper tributyltin or trimethyltin precursor in toluene, Ko and co-workers created star-shaped molecules with s-triazine centers and substituted triphenylamine groups on their perimeter (Table 2, entry 8).63,64
3.8. Sonogashira cross-coupling reactions
Kim and co-workers have described the production of a molecule with a star-like structure and C3-symmetry by using 1,3,5-triethynylbenzene as the central core and 2,5-diphenyloxadiazole as the side arms. The compound 1,3,5-triethynylbenzene was generated when 1,3,5-tribromobenzene was treated with trimethylsilylacetylene and potassium carbonate. Second, using Sonogashira cross-coupling between the chemical and the bromo compound with [Pd(PPh3)2Cl2] as the catalyst, the required star-shaped molecule was produced with a yield of 54% (Table 2, entry 9).65
The Sonogashira cross-coupling of bromo-intermediates was accomplished by following the same approach. Since the bromine predecessors were insoluble in the MeCN. A series of tests determined that toluene is an effective solvent for carrying out this transition. As a consequence of this, bromo-intermediates were put through a Sonogashira cross-coupling reaction with 1,3,5-triethynylbenzene in the presence of [Pd(PPh3)4]/CuI as the catalyst system and (diisopropyl)amine in toluene. This resulted in the production of coupling products with a high rate of success.
Amir and his team have created an extraordinarily efficient catalyst for the Sonogashira cross-coupling of aryl halides with aromatic and aliphatic terminal alkynes. The methodology allows the use of palladium nanoparticles immobilized on a dendritic nano-silica-triazine polymer. The processes were performed in water, the most environmentally friendly solvent. Table 2 provides an overview of these different strategies.
4. Polymerization
Polymerization techniques based on the C3-symmetric star-shaped molecules have been illustrated, including the trimerization of alkynes or aromatic nitriles in the existence of acidic catalysts, a polycondensation reaction of monomers containing specific functional groups, and a cross-coupling reaction containing building blocks. A brief overview of the most important, as well as common techniques, has been displayed as follows.
4.1. Polycyclotrimerizations
Polycyclotrimerizations of diyne molecules give C3-symmetric polyphenylene with benzene ring repeated units. In this context, polycyclotrimerization was uniquely synthesized in the A2 monomer approach.66–69 Alkyne cyclotrimerization has been developed as a viable process for manufacturing C3-symmetric polymers. Tang and coworkers ran into cross-linking issues during their research, the monomers were terminal diynes connected by aromatic rings utilized. The researchers worked in molecularly engineered monomer structures and optimized polymerization conditions, and successfully synthesized C3-symmetric polypropylenes with high molecular weight and excellent solubility.70,71
Through further investigation, the same research team reported that the polycyclotrimerizations of bis(aroylacetylenes) were started by catalysts classified as nonmetallic or organocatalysts involving piperidine. The polymerization reaction proceeds smoothly to produce C3-symmetric poly(aroylarylenes) with high DBs in high yields by an ionic mechanism.72,73 Additionally, analysis of the monomer structure demonstrates that this polymerization reaction works for diynes where triple bonds are attached to electron-withdrawing groups. If the carbonyl bond in the aroylacetylene can be replaced by an ester group, the monomer synthesis becomes much more accessible. The conveniently polymerized bipropiolate monomer opened the synthetic route to the easy and economical synthesis of C3 symmetric polymers. The Tangs group investigated this possibility and showed that reflux polycyclotrimerizations of bipropiolate in DMF produce C3-symmetric polymers with perfectly branched structures and 1,3,5-regioregularity in high yields.74 The synthetic sequence for this type of polymerization is shown in Scheme 1.
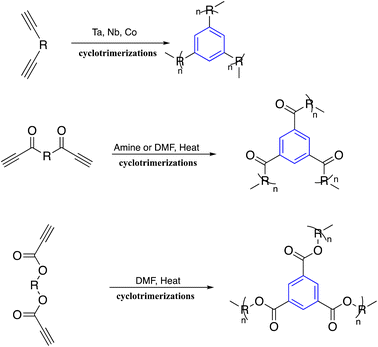 |
| Scheme 1 Trimerization of alkyne. | |
Moreover, a solvothermal method was utilized for cyclotrimerization of bifunctional acetyl arenes compounds to obtain highly porous polymers. Various acids were employed to synthesize polycyclotrimerization in solution induced, including silicon tetrachloride and molten 4-toluene sulfonic acid,75 thionyl chloride,76 and superacid-catalyzed.77
Zi-Hao Guo et al. achieved cost-effective bottom-up syntheses of organic microporous polymer networks using molten methanesulfonic acid mediated aldol triple condensation reaction. This method features inexpensive starting materials, reagents, and simple reaction procedures.78,79 Recently, ionothermal conditions demonstrated that the solvent-free cyclotrimerization of methyl ketones could be driven to high conversions using molten ZnCl2 as the reaction solvent, leading to a highly conjugated organic polymer.80 Fig. 1 shows the development of the synthesis of C3-symmetric polymeric materials containing benzene core via cyclotrimerization.
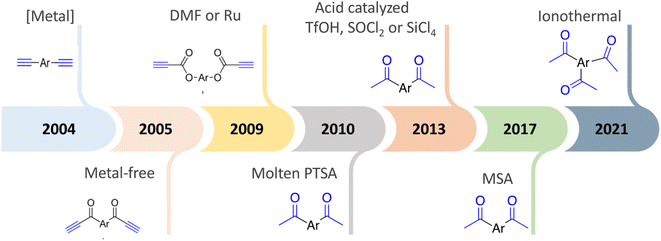 |
| Fig. 1 C3-symmetric polymeric materials containing benzene core via cyclotrimerization. | |
On the other hand, forming triazine rings required harsh conditions. Thus, Thomas et al. synthesized highly stable polymers by ionothermal trimerization of nitrile groups in molten ZnCl2, which performed as a solvent and catalyst at high temperatures ranging from 400–600 °C. Further forward, in 2010, W. Zhang and co-workers enhanced the ionothermal method by utilizing a microwave-assisted that reduced the reaction time. Moreover, Cooper et al. developed a microwave-assisted by adding Brønsted acid trifluoromethanesulfonic acid (TFMS) as a polymerization catalyst. Furthermore, W. Huang and co-workers reported a novel TFMS acid vapor-assisted solid-phase synthetic method to construct triazine from aromatic nitrile monomers. Also, CF3SO3H-catalyzed trimerization of nitriles under both room temperature and microwave conditions was reported. This process gave significant benefits by eliminating the undesirable ZnCl2 contamination and destruction of nitrile and C–H bonds. Nevertheless, CF3SO3H-catalyzed trimerization of nitriles has some limitations, such as a low synthesis temperature being required, an expensive catalyst, and brutal handling of the reaction due to the caustic and eye-irritating carcinogenic chemicals involved, along with the ice-cold saltwater considered necessary for neutralization, as illustrated in Scheme 2.81–84
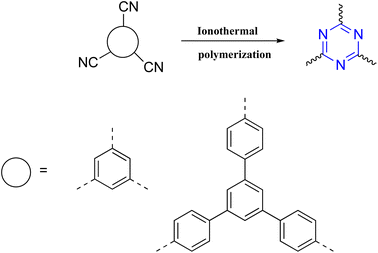 |
| Scheme 2 Trimerization of carbonitriles. | |
Nevertheless, to avoid harsh synthetic conditions and associated limited structural diversity K. Wang et al. developed a new strategy involving the condensation reaction of aldehydes and amidines to construct polymer triazine-based under mild conditions. Moreover, the phosphorus pentoxide (P2O5)-catalyzed employed as direct condensation of aromatic amide instead of aromatic nitrile to form triazine rings and likewise reported in situ oxidation of alcohols followed by amidine-aldehyde polycondensation, where the reaction rates were controlled by gradually generating aldehydes by in situ slow oxidation of alcohols to form highly crystalline polymer triazine-based.84–89 Fig. 2 shows the development of the synthesis of C3-symmetric polymeric materials containing triazine core via cyclotrimerization.
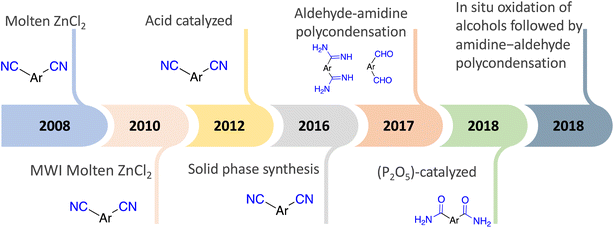 |
| Fig. 2 C3-symmetric polymeric materials containing triazine core via cyclotrimerization. | |
4.2. Addition polymerization
Free radical polymerization is one class of addition polymerization techniques developed to synthesize C3-symmetric polymer, representing the subsequent addition of free radicals to form a polymer backbone. Modak et al. reported functionalized C3-symmetric polymer by simple in situ radical polymerization of 2,4,6-triallyloxy-1,3,5-triazine in an aqueous medium in the presence of ammonium persulfate (APS) as a radical initiator and anionic surfactant sodium dodecyl sulfate (SDS) as a template under acidic conditions. TEM image and powder XRD analysis showed the pores in the ordered 2D hexagonal structure of the material. In addition, N2 sorption analysis demonstrated an excellent surface area for this C3-symmetric polymer (Scheme 3).90
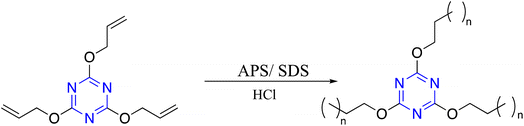 |
| Scheme 3 Free radical polymerization. | |
Sina and Majid developed a free-standing conjugated C3-symmetric polymer through a cationic polymerization reaction in a one-pot, low-temperature, and super-acid-catalyzed process. Bifunctional monomers with ethylene and nitrile groups were used, catalyzed by trifluoromethanesulfonic acid (CF3SO3H), which provided ethynyl polymerization and nitrile cyclotrimerization. Even during the polymerization process, the hard-triple bonds are transformed into stretchable double bonds, which makes it easier to build a membrane that is both flexible and has increased mechano-strength. This polymer showed extremely significant CO2 removal capacity up to 3.87 mmol g−1 (170.3 mg g−1), and the electron richness of the conjugated skeleton increased the basicity necessary for interaction with CO2 via dipole-quadrupole- and acid–base interactions (Scheme 4).91
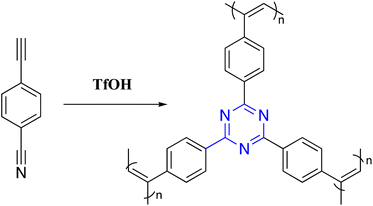 |
| Scheme 4 Cationic polymerization. | |
4.3. Oxidative coupling polymerization
The oxidative coupling polymerization of the aromatic ring in the presence of FeCl3 has been reported to construct conjugated C3-symmetric organic frameworks. Mu et al. prepared a simplistic method for carbazole-based oxidative coupling polymerization using the 2,4,6-tris-aromatic-1,3,5-triazine building block catalyzed with FeCl3 in an anhydrous solvent. The three-dimensional polymer scaffolds were highly fluorescent, with a BET surface area of 840 m2 g−1 in the microporous regime.92
Correspondingly, chemical oxidative polymerization was employed to synthesize organic conjugated polymer containing star-shaped triphenyltriazine with C3-symmetry. Chen and co-workers designed and successfully synthesized poly[1,3,5-tris(4-diphenylamino-phenyl)triazine] through functionalized triphenylamine backbone, accompanied by FeCl3-catalyzed through oxidative chemical polymerization. The polymer powder exhibits a high surface area in 930 m2 g−1 and a double Peruse distribution ranging from micropores 0.55 nm to small-diameter mesopores 26 nm (Scheme 5).93
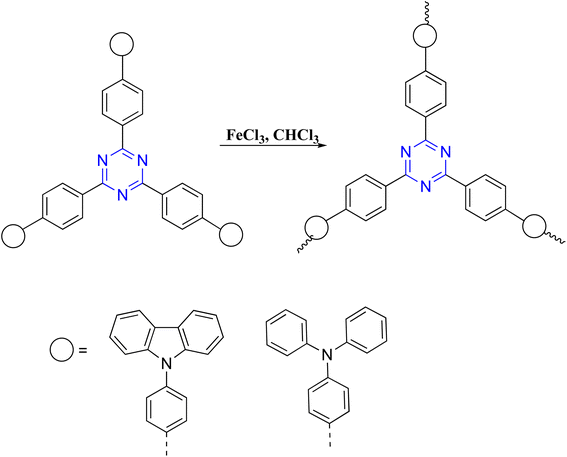 |
| Scheme 5 Oxidative polymerization. | |
Moreover, oxidative co-polymerization was reported through an electrochemical method, the research discussed the reaction between dithienylpyrrole and tris[4-(2-thienyl)phenyl]amine in CH3CN/CH2Cl2 to grant the C3-symmetry polymer (Scheme 6).94,95
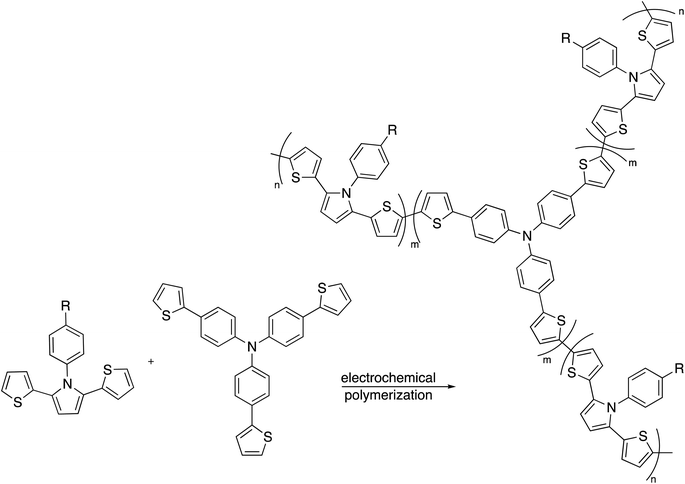 |
| Scheme 6 Oxidative co-polymerization. | |
4.4. Schiff-base polymerization
Schiff base chemistry is also applied to synthesize polymers with C3-symmetry via an A2 + B3 approach.96–102 Müllen et al. reported smart condensation reactions of triamine moieties with various dialdehydes to generate highly cross-linked porous aminal networks. The polymers were prepared by microwave irradiation, one-pot, catalyst-free synthesis, and cheap and uncomplicated monomers. The polymer networks contained up to 50 wt% nitrogen, highly stable and insoluble in aqueous and organic solvents, including DMSO, MeOH, acetone, DCM, and THF (Scheme 7).96,97
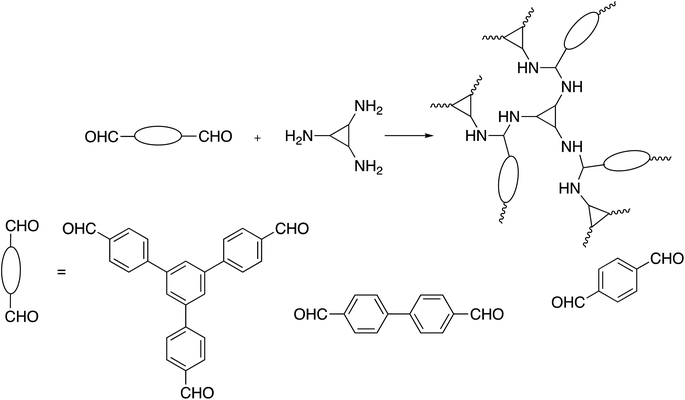 |
| Scheme 7 Synthesis of C3-symmetry with aminal networks. | |
Newly, As illustrated in Scheme 8, the trialdehyde moiety was condensed with a variety of amines, which resulted in the formation of a number of imine- or hydrazone-linked103,104 compounds. Through a Schiff base reaction involving 1,3,5-tris-(4-formyl-phenyl)triazine and p-phenylenediamine or terephthalic acid dihydrazide, Pitchumani et al. created an imine-based polymer with C3-symmetry.
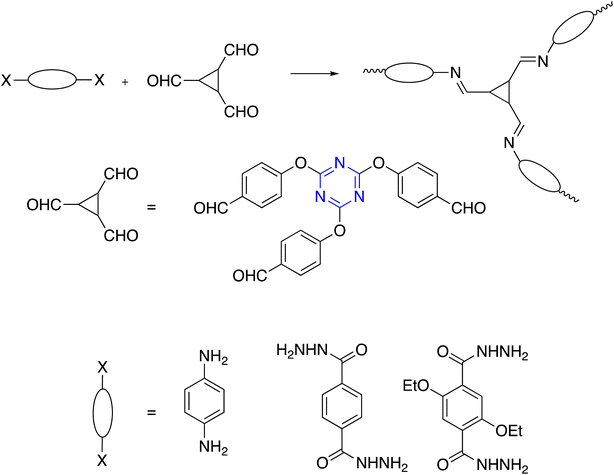 |
| Scheme 8 Synthesis of C3-symmetry with imine or hydrazone networks. | |
4.5. Nucleophilic substitution reaction
C3-symmetry polyamides were prepared in good yield from triamine and triacid chloride monomers through an A3 + B3 approach. Lately, Yavuz et al. synthesized C3-symmetry polyamides by triamine and triacid chloride monomers by solution polymerization in two variable systems of solvents (DMAc/NMP and 1,4-dioxane) at room temperature (Scheme 9).103
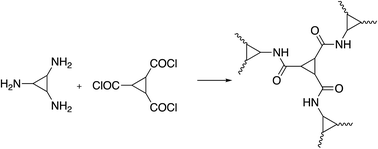 |
| Scheme 9 Synthesis of C3-symmetry with amide networks. | |
In other respects, the interaction of 2,4,6-tris(4-aminophenyl)-1,3,5-triazine and the dianhydride carried out in m-cresol as a solvent according to the A3 + B2 strategy resulted in the successful synthesis of C3-symmetry polyimides in excellent yield. The resulting polymer displayed significant chemical and thermal stability up to 450 °C. Moreover, the three-dimensional polymer showed a BET surface area of up to 809 m2 g−1 in the microporous range, as displayed in Scheme 10.104
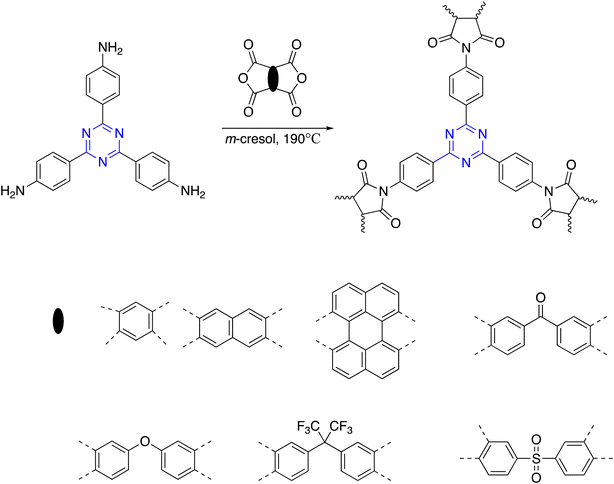 |
| Scheme 10 Synthesis of C3-symmetry with amide networks. | |
4.6. Friedel–Crafts polymerization
Friedel–Crafts reaction (FC) of cyanuric chloride and aromatic building blocks using anhydrous AlCl3 or methanesulfonic as a catalyst was published. Lim and co-workers stated that microporous polymers based on an s-triazine unit were synthesized by the FC reaction of cyanuric chloride with aromatic compounds in the presence of aluminum chloride.105,106 S. Xiong et al. developed a cost-effective approach for constructing s-triazine-based polymeric networks with a high BET surface area of up to 894 m2 g−1 that have been under the catalysis of methanesulfonic acid (Scheme 11).107
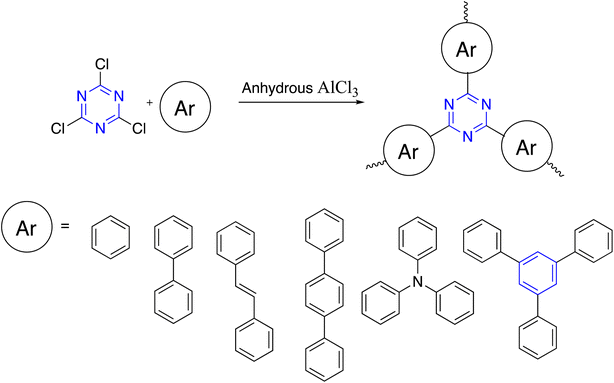 |
| Scheme 11 Synthesis of C3-symmetry triazine-based via the Friedel–Crafts reaction. | |
Dai et al. prepared C3-symmetry polymers triazine and carbazole bi-functionalized via FC reaction between the triazine-functionalized carbazole monomer and formaldehyde dimethyl acetal in the presence of FeCl3 and 1,2-dichloroethane (DCE) (Scheme 12).108
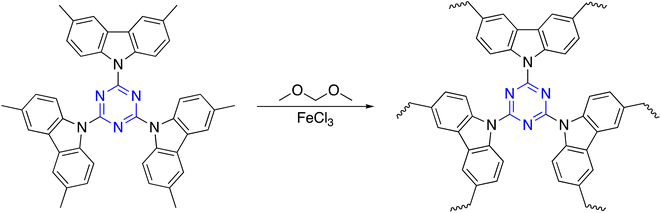 |
| Scheme 12 Synthesis of C3-symmetry triazine-based via the Friedel–Crafts reaction. | |
4.7. Coupling polymerizations
It was possible to synthesize C3-symmetry using the Sonogashira–Hagihara cross-coupling method, which was predicated on the electron-withdrawing polymers s-triazine and benzene and originated from trihalo and triayne or diayne monomers using an A3 + B3 or A3 + B2 strategy, correspondingly, as shown in Scheme 13.109
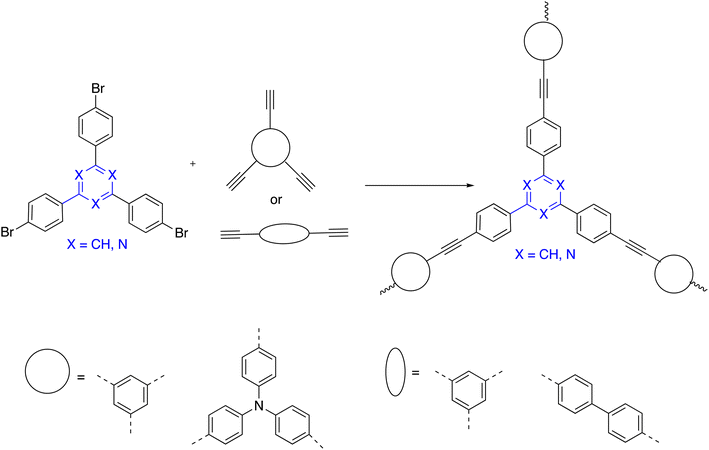 |
| Scheme 13 Synthesis of C3-symmetry via the Sonogashira coupling reaction. | |
Xiang employed the Yamamoto reaction to significantly obtain porous organic polymers that carried out a high hydrothermal-stability. Yamamoto reaction consists of a homocoupling of aryl bromides in the presence of a Ni(0) complex. Consequently, the mentioned article operated s-triazine and benzene C3-symmetry star-shaped polymer under Ni-catalyzed throughout polycondensation method (Scheme 14).110
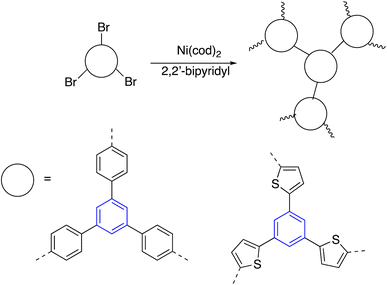 |
| Scheme 14 Synthesis of C3-symmetry via the Yamamoto coupling reaction. | |
By the same token, Glaser–Hay coupling reaction was examined to synthesize co-polyenes through homopolycouplings of aryltriynes and monoyne. The coupling reaction occurs with cuprous salts in the presence of oxygen and a base, giving soluble hyperbranched polyenes. The polymerizations were terminated by dumping the provides a good balance into acidified methanol before it reached the gel stage to avoid the network's formation (Scheme 15).111
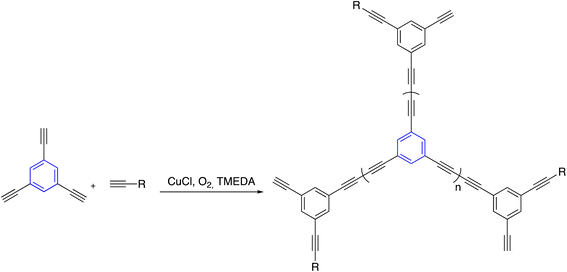 |
| Scheme 15 Synthesis of C3-symmetry via the Glaser–Hay coupling reaction. | |
On top of that, direct arylation polymerization was described as a synthetic technique for another class of triazine-core polymers. This was done in addition to the previous point. It was unnecessary to pre-activate the C–H bonds in the arene monomers to carry out the coupling reaction of 2,4,6-(tri-2-thienyl)-1,3,5-triazine with multi-brominated monomers of varied geometries. The products obtained were obtained. Because of this benefit, it is possible to incorporate the triazine unit into multidimensional polymeric structures effortlessly. As a result, this provides comonomers with a considerable amount of synthetic control over the morphologies, porosities, and optoelectronic characters of triazine-core materials (Scheme 16).112
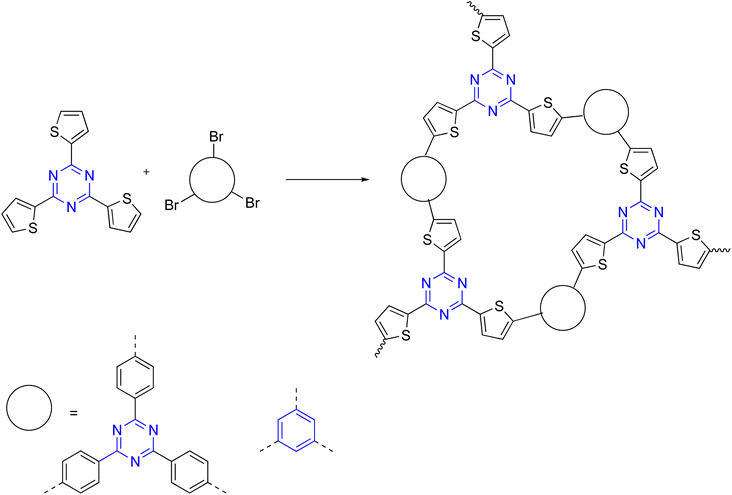 |
| Scheme 16 Synthesis of C3-symmetry via the direct arylation coupling reaction. | |
4.8. Click polymerizations
Tang and co-workers studied click polymerizations by mixing diazide and triyne monomers using the normal click tools under CuSO4/sodium ascorbate catalyst inTHF/water mixture. It's possible that the incompatibility between the species that are developing and the aqueous media is what caused the polymers to clump together and then precipitating. When initiating the click polymerization of triyne with diazides, a non-aqueous click of the catalyst Cu(PPh3)3Br was utilized. This was done so as to minimize the usage of an aqueous medium. The catalyzed polymerization of diazide and triyne monomers produced 1,4-riazin in 46% yield, and showed high solubility in DMSO, DMF, THF, and DCM. On the other hand, 1,5-regioselective click polymerization of diazide and triyne monomers catalyzed by Cp*Ru(PPh3)2Cl produced soluble 1,5-triazine in 75% yield in as short as 30 min.113–115 The two synthetic procedures were displayed as shown in Scheme 17.
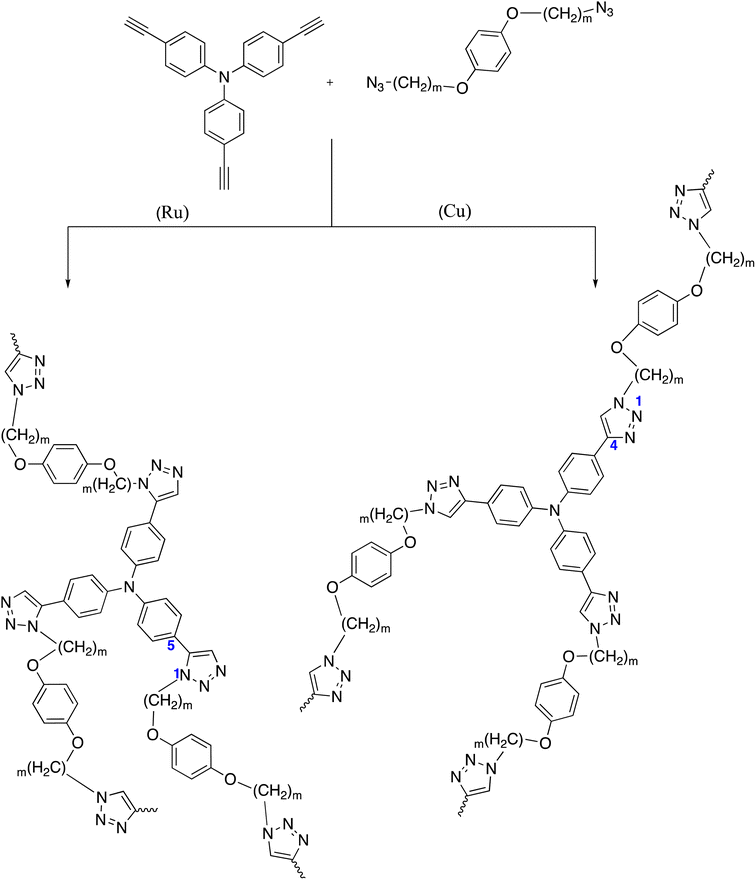 |
| Scheme 17 Synthesis of C3-symmetry via the click reaction. | |
5. Biomedical applications
C3-symmetric compounds that are based on benzene or s-triazine cores have high surface areas and thin structures, which allow for surface modification with various components. This further enables a wide range of applications, including biomedical, pharmaceutical, gas storage, photovoltaics, conductivity, catalysis, electrochemical sensing, and many more. Unfortunately, and according to our best of knowledge, there are a limited number of relevant studies in the biomedical applications field compared to the other fields. Therefore, the following table provides a concise summary of the most recent developments in biomedical applications, which include drug delivery, gene delivery, photodynamic therapy, photothermal therapy, biosensing, and bioimaging (Table 3).
Table 3 Selected recent examples of C3-symmetric based on benzene or s-triazine materials for biomedical applications
Entry |
Core |
Structure |
Application |
Features |
Ref. |
1 |
s-Triazine |
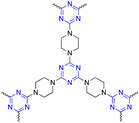 |
Drug delivery of ibuprofen (IBU) |
50% of the IBU was released in 5 h |
116 |
75% was released in 10 h |
Almost the release was completed in two days |
2 |
s-Triazine 1,3,5-trisustuted benzene |
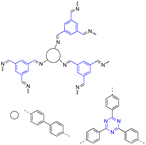 |
Drug delivery, three different drugs, captopril, IBU, and 5-fluorouracil (5-FU) |
5-FU had a 30% loading capacity and was released in 3 days |
117 |
3 |
s-Triazine |
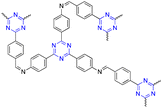 |
Drug delivery of quercetin |
Enhanced anticancer activity of quercetin |
118 |
4 |
1,3,5-Trisustuted benzene |
TAPB-DMTP (in situ one-pot method) |
Drug delivery of doxorubicin (DOX) |
DOX had a 32.1% loading capacity, and the release was pH-responsive |
119 |
5 |
1,3,5-Trisustuted benzene |
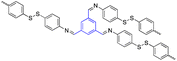 |
Drug delivery of doxorubicin (DOX) |
Drug-loading capacity (21%) |
120 |
6 |
s-Triazine |
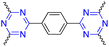 |
Gene delivery |
Strong affinity toward nucleic acids |
121 |
7 |
1,3,5-Trisustuted benzene |
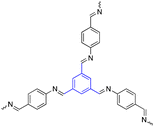 |
Photodynamic therapy PDT and photothermal therapy PTT |
Photosensitizer for PDT and PTT with enhanced antitumor efficacy |
122–124 |
8 |
s-Triazine |
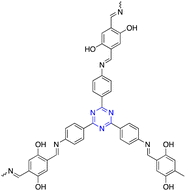 |
Photodynamic therapy |
Increase the reactive oxygen species in the tumor to improve the in vivo PDT effect of cancer |
125 |
9 |
1,3,5-Trisustuted benzene |
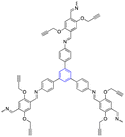 |
Electrochemical biosensor |
Accelerate signal amplification, which increase biosensor sensitivity |
126 |
10 |
1,3,5-Trisustuted benzene |
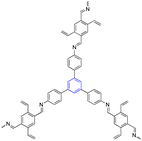 |
Biosensor |
Facilitates the migration of charge carriers and enhance the response of sensors |
127 and 128 |
Excellent size-exclusion effect, high selectivity, sensitivity, and reusability |
11 |
1,3,5-Trisustuted benzene |
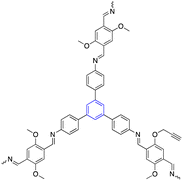 |
Bioimaging |
Exhibits excellent specificity and can only recover its fluorescence signal upon meeting the specific targets |
129 |
6. Conclusion and outlook
To sum up, the significant properties of C3-symmetric compounds based on benzene or s-triazine cores have inspired chemists to design, synthesize, and use them in numerous applications. These consist of symmetrically porous, hetero-atomic sites and multifunctional surfaces. Moreover, it has high thermal and chemical stability due to its structure via strong covalent bonds. Henceforth, synthesizing these materials requires additional effort to be simplified to achieve effortless, cheaper, and acceptable methods. Our review article will assist future researchers in developing synthetic methodologies. Likewise, the choice of an organic linker or precursor can also be explored by employing our overview.
Conflicts of interest
There are no conflicts to declare.
References
- S. Kotha and S. Todeti, Synthesis of C3-symmetric star-shaped molecules containing α-amino acids and dipeptides via Negishi coupling as a key step, Beilstein J. Org. Chem., 2019, 15, 371–377 CrossRef CAS PubMed.
- M. Imran, S. Manzoor, M. K. Siddiqui, S. Ahmad and M. H. Muhammad, On physical analysis of synthesis strategies and entropy measures of dendrimers, Arabian J. Chem., 2022, 15(2), 103574 CrossRef CAS.
- H. Zhou, Y. F. Ao, D. X. Wang and Q. Q. Wang, Inherently Chiral Cages via Hierarchical Desymmetrization, J. Am. Chem. Soc., 2022, 144(37), 16767–16772 CrossRef CAS PubMed.
- Y. Y. Zhang, et al., MOFs assembled from C3-symmetric ligands:structure, iodine capture and role as bifunctional catalysts towards the oxidation-Knoevenagel cascade reaction, Dalton Trans., 2021, 50(2), 647–659 RSC.
- S. Q. Xu, R. R. Liang, T. G. Zhan, Q. Y. Qi and X. Zhao, Construction of 2D covalent organic frameworks by taking advantage of the variable orientation of imine bonds, Chem. Commun., 2017, 53(16), 2431–2434 RSC.
- A. Sandeep, V. K. Praveen, D. S. Shankar Rao, S. Krishna Prasad and A. Ajayaghosh, Transforming a C3-Symmetrical Liquid Crystal to a π-Gelator by Alkoxy Chain Variation, ACS Omega, 2018, 3(4), 4392–4399 CrossRef CAS PubMed.
- G. Hennrich, et al., Liquid crystals from C3-symmetric mesogens for second-order nonlinear optics, Angew. Chem., Int. Ed., 2006, 45(25), 4203–4206 CrossRef CAS PubMed.
- L. Wang, Q. Fang, Q. Lu, S. J. Zhang, Y. Y. Jin and Z. Q. Liu, Octupolar (C3 and S4) Symmetric Cyclized Indole Derivatives: Syntheses, Structures, and NLO Properties, Org. Lett., 2015, 17(17), 4164–4167 CrossRef CAS PubMed.
- J. De, et al., Blue Luminescent Organic Light Emitting Diode Devices of a New Class of Star-Shaped Columnar Mesogens Exhibiting π-π Driven Supergelation, J. Phys. Chem. C, 2018, 122(41), 23659–23674 CrossRef CAS.
- A. Mishra, C. Q. Ma and P. Bäuerle, Functional oligothiophenes: Molecular design for multidimensional nanoarchitectures and their applications, Chem. Rev., 2009, 109(3), 1141–1176 CrossRef CAS PubMed.
- A. R. Murphy and J. M. J. Fréchet, Organic semiconducting oligomers for use in thin film transistors, Chem. Rev., 2007, 107(4), 1066–1096 CrossRef CAS PubMed.
- C. Wang, H. Dong, W. Hu, Y. Liu and D. Zhu, Semiconducting π-conjugated systems in field-effect transistors: A material odyssey of organic electronics, Chem. Rev., 2012, 112(4), 2208–2267 CrossRef CAS PubMed.
- M. Antonijevic, C. Rochais and P. Dallemagne, C3-Symmetric Ligands in Drug Design: When the Target Controls the Aesthetics of the Drug, Molecules, 2023, 28(2), 679 CrossRef CAS PubMed.
- D. P. Yang, M. N. L. Oo, G. R. Deen, Z. Li and X. J. Loh, Nano-Star-Shaped Polymers for Drug Delivery Applications, Macromol. Rapid Commun., 2017, 38(21), 1–25 CrossRef PubMed.
- M. Bashiri, et al., Synthesis and evaluation of biological activities of tripodal imines and β-lactams attached to the 1,3,5-triazine nucleus, Monatshefte fur Chemie, 2020, 151(5), 821–835 CrossRef CAS.
- W. Nomura, et al., A Synthetic C34 Trimer of HIV-1 gp41 Shows Significant Increase in Inhibition Potency, ChemMedChem, 2012, 7(2), 205–208 CrossRef CAS PubMed.
- A. Roy, D. Saha, A. Mukherjee and P. Talukdar, One-pot synthesis and transmembrane chloride transport properties of C3-symmetric benzoxazine urea, Org. Lett., 2016, 18(22), 5864–5867 CrossRef CAS PubMed.
- V. Gevorgyan, U. Radhakrishnan, A. Takeda, M. Rubina, M. Rubin and Y. Yamamoto, Palladium-catalyzed highly chemo- and regioselective formal [2 + 2 + 2] sequential cycloaddition of alkynes: a renaissance of the well known trimerization reaction?, J. Org. Chem., 2001, 66(8), 2835–2841 CrossRef CAS PubMed.
- V. Cadierno, S. E. García-Garrido and J. Gimeno, Efficient intermolecular [2 + 2 + 2] alkyne cyclotrimerization in aqueous medium using a ruthenium(IV) precatalyst, J. Am. Chem. Soc., 2006, 128(47), 15094–15095 CrossRef CAS PubMed.
- J. S. Cheng and H. F. Jiang, Palladium-Catalyzed Regioselective Cyclotrimerization of Acetylenes in Supercritical Carbon Dioxide, Eur. J. Org. Chem., 2004, 3, 643–646 CrossRef.
- K. Tanaka, H. Hara, G. Nishida and M. Hirano, Synthesis of perfluoroalkylated benzenes and pyridines through cationic Rh(I)/modified BINAP-catalyzed chemo- and regioselective [2 + 2 + 2] cycloaddition, Org. Lett., 2007, 9(10), 1907–1910 CrossRef CAS PubMed.
- M. Lautens, W. Klute and W. Tam, Transition metal-mediated cycloaddition reactions, Chem. Rev., 1996, 96(1), 49–92 CrossRef CAS PubMed.
- S. Kotha, D. Bansal and R. V. Kumar, Synthesis of symmetrical and unsymmetrical trisubstituted benzene derivatives through ring-closing alkyne metathesis strategy and dcpropargylation with various catalysts, Indian J. Chem., Sect. B: Org. Chem. Incl. Med. Chem., 2009, 48(2), 225–230 Search PubMed.
- K. Yang, et al., Hydrogen-Bonding Controlled Nickel-Catalyzed Regioselective Cyclotrimerization of Terminal Alkynes, Org. Lett., 2021, 23(10), 3933–3938 CrossRef CAS.
- A. Sen, T. Sato, A. Ohno, H. Baek, A. Muranaka and Y. M. A. Yamada, Polymer-Supported-Cobalt-Catalyzed Regioselective Cyclotrimerization of Aryl Alkynes, JACS Au, 2021, 1(11), 2080–2087 CrossRef CAS PubMed.
- H. C. O. System, Trisannelation of Acrylates to 1,3,5-Benzenetricarboxylates by a Pd(OAc)2 J. Org. Chem., 2007, 72(9), 3603–3605 Search PubMed.
- H. F. Jiang, Y. X. Shen and Z. Y. Wang, A simple PdCl2/O2/DMF catalytic system for highly regioselective cyclotrimerization of olefins with electron-withdrawing groups, Tetrahedron Lett., 2007, 48(42), 7542–7545 CrossRef CAS.
- H. F. Jiang, Y. X. Shen and Z. Y. Wang, Palladium-catalyzed aerobic oxidation of terminal olefins with electron-withdrawing groups in scCO2, Tetrahedron, 2008, 64(3), 508–514 CrossRef CAS.
- S. P. Midya, et al., Room-Temperature Synthesis of 1,3,5-Tri(het)aryl Benzene from Nitroalkenes Using Pd(OAc)2: Complete Mechanistic and Theoretical Studies, Org. Lett., 2022, 24(24), 4438–4443 CrossRef CAS PubMed.
- B. B. Jena, et al., Triazine-cored dendritic molecules containing multiple o-carborane clusters, Appl. Organomet. Chem., 2020, 34(9), 1–8 CrossRef.
- M. Sen Yuan, et al., Acceptor or donor (diaryl B or N)substituted octupolar truxene: synthesis, structure, and charge-transfer-enhanced fluorescence, J. Org. Chem., 2006, 71(20), 7858–7861 CrossRef PubMed.
- W. Zhang, X. Y. Cao, H. Zi and J. Pei, Single-molecule nanosized polycyclic aromatics with alternant five- and six-membered rings: Synthesis and optical properties, Org. Lett., 2005, 7(6), 959–962 CrossRef CAS PubMed.
- S. Kotha, S. Todeti and V. R. Aswar, Design and synthesis of c3-symmetric molecules bearing propellane moieties via cyclotrimerization and a ring-closing metathesis sequence, Beilstein J. Org. Chem., 2018, 14, 2537–2544 CrossRef CAS PubMed.
- T. Shimasaki, Y. Takiyama, Y. Nishihara, A. Morimoto, N. Teramoto and M. Shibata, Synthesis and properties of novel C3-symmetrical 1,3,5-tris(dibenzoheterolyl)benzenes, Tetrahedron Lett., 2015, 56(1), 260–263 CrossRef CAS.
- S. Kotha, D. Kashinath, K. Lahiri and R. B. Sunoj, Synthesis of C3-symmetric nano-sized polyaromatic compounds by trimerization and Suzuki-Miyaura cross-coupling reactions, Eur. J. Org. Chem., 2004, 19, 4003–4013 CrossRef.
- K. Okumoto and Y. Shirota, New class of hole-blocking amorphous molecular materials and their application in blue-violet-emitting fluorescent and green-emitting phosphorescent organic electroluminescent devices, Chem. Mater., 2003, 15(3), 699–707 CrossRef CAS.
- E. Koepp, New Trigonal Lattice Hosts: Stoicheiometric Crystal Inclusions, Ratio, 1988, pp. 1251–1257 Search PubMed.
- H. Ramshini, R. Tayebee, A. Bigi, F. Bemporad, C. Cecchi and F. Chiti, Identification of Novel 1,3,5-Triphenylbenzene Derivative Compounds as Inhibitors of Hen Lysozyme Amyloid Fibril Formation, Int. J. Mol. Sci., 2019, 20(22), 5558 CrossRef CAS PubMed.
- S. L. Zhang, Z. F. Xue, Y. R. Gao, S. Mao and Y. Q. Wang, Triple condensation of aryl methyl ketones catalyzed by amine and trifluoroacetic acid: straightforward access to 1,3,5-triarylbenzenes under mild conditions, Tetrahedron Lett., 2012, 53(19), 2436–2439 CrossRef CAS.
- R. Maragani and R. Misra, Donor-acceptor ferrocenyl triazines: Synthesis and properties, Tetrahedron Lett., 2013, 54(39), 5399–5402 CrossRef CAS.
- F. Christopher Pigge, Z. Zheng and N. P. Rath, 1,3,5-Triaroylbenzenes as versatile inclusion hosts via C–H···O hydrogen bonding, New J. Chem., 2000, 24(4), 183–185 RSC.
- K. K. Balasubramanian, S. Selvaraj and P. S. Venkataramani, Trimerisation of Aryl Ethynyl Ketones, Synthesis, 1980, 1, 29–30 CrossRef.
- M. Tanaka, et al., One-pot synthesis of 1,3,5-tribenzoylbenzenes by three consecutive michael addition reactions of 1-phenyl-2-propyn-1-ones in pressurized hot water in the absence of added catalysts, Chem.–Eur. J., 2011, 17(2), 606–612 CrossRef CAS.
- J. Yang and J. G. Verkade, Disilane-Catalyzed and Thermally Induced Oligomerizations of Alkynes: A Comparison, Organometallics, 2000, 19(5), 893–900 CrossRef CAS.
- T. Iwado, K. Hasegawa, T. Sato, M. Okada, K. Sue, H. Iwamura and T. Hiaki, Mechanistic and Exploratory Investigations into the Synthesis of 1,3,5-Triaroylbenzenes from 1-Aryl-2-propyn-1-ones and 1,3,5- Triacetylbenzene from 4-Methoxy-3-buten-2-one by Cyclotrimerization in Hot Water in the Absence of Added Acid or Base, J. Org. Chem., 2013, 78(5), 1949–1954 CrossRef CAS.
- C. L. Zhang, Z. F. Zhang, Z. H. Xia, Y. F. Han and S. Ye, DBU-Mediated Construction of 1,3,5-Trisubstituted Benzenes via Annulation of α,β-Unsaturated Carboxylic Acids and α-Cyano-β-methylenones, J. Org. Chem., 2018, 83(20), 12507–12513 CrossRef CAS PubMed.
- B. Al-saleh, M. M. Abdelkhalik, M. A. Eltoukhy and M. H. Elnagdi, Enaminones in Heterocyclic Synthesis: a New Regioselective, J. Heterocycl. Chem., 2002, 39, 1035–1038 CrossRef CAS.
- M. M. Abdel-Khalik and M. H. Elnagdi, Enaminones in organic synthesis: A novel synthesis of 1,3,5-trisubstituted benzene derivatives and of 2-substituted-5-aroylpyridines, Synth. Commun., 2002, 32(2), 159–164 CrossRef CAS.
- J. P. Wan, Y. Lin, K. Hu and Y. Liu, Metal-free synthesis of 1,3,5-trisubstituted benzenes by the cyclotrimerization of enaminones or alkynes in water, RSC Adv., 2014, 4(39), 20499–20505 RSC.
- A. Abdel, M. S. El and A. O. Abdelhamid, Green methodologies in organic synthesis: Microwave assisted solvent- and catalyst-free synthesis of enaminones and their conversion into 1,3,5-trisubstituted benzenes as well as 3-aroyl-6-substituted pyridines Morsy, Eur. J. Chem., 2012, 3(4), 455–460 CrossRef.
- M. M. Boorum and L. T. Scott, The Synthesis of Tris-Annulated Benzenes by Aldol Trimerization of Cyclic Ketones, Mod. Arene Chem., 2004, 20–31 Search PubMed.
- A. W. Amick and L. T. Scott, Trisannulated benzene derivatives by acid catalyzed aldol cyclotrimerizations of cyclic ketones. methodology development and mechanistic insight, J. Org. Chem., 2007, 72(9), 3412–3418 CrossRef CAS PubMed.
- S. Kotha and V. R. Shah, Synthesis of bis- and trisoxazole derivatives via Suzuki-Miyaura cross-coupling reaction and van Leusen oxazole synthesis, Synthesis, 2007, 23, 3653–3658 CrossRef.
- S. R. Shah, R. R. Thakore, T. A. Vyas and B. Sridhar, Conformationally flexible C3-symmetric 1,3-oxazoles as molecular scaffolds, Synlett, 2016, 27(2), 294–300 CrossRef CAS.
- A. Ritzén, B. Basu, A. Wållberg and T. Frejd, Phenyltrisalanine: a new, C3-symmetric, trifunctional amino acid, Tetrahedron: Asymmetry, 1998, 9(19), 3491–3496 CrossRef.
- H. Meier, H. C. Holst and A. Oehlhof, Star-Shaped Compounds Having 1,3,5-Triazine Cores, Eur. J. Org. Chem., 2003,(21), 4173–4180 CrossRef CAS.
- S. Kotha, B. U. Solanke and N. K. Gupta, Design and synthesis of C3-symmetric molecules containing oxepine and benzofuran moieties via Metathesis, J. Mol. Struct., 2021, 1244, 130907 CrossRef CAS.
- S. Kotha, S. Todeti, T. Das and A. Datta, Synthesis of star-shaped pyrrole-based C3-symmetric molecules via ring-closing metathesis, Buchwald–Hartwig cross-coupling and Clauson–Kaas pyrrole synthesis as key steps, Tetrahedron Lett., 2018, 59(11), 1023–1027 CrossRef CAS.
- S. Kotha and M. Meshram, Application of organometallics in organic synthesis, J. Organomet. Chem., 2018, 874, 13–25 CrossRef CAS.
- S. Kotha and K. Lahiri, Expanding the diversity of polycyclic aromatics through a Suzuki-Miyaura cross-coupling strategy, Eur. J. Org. Chem., 2007,(8), 1221–1236 CrossRef CAS.
- S. Kotha and V. R. Shah, Synthesis of C3-symmetric and C4-symmetric amino acid derivatives via Suzuki-Miyaura cross-coupling reaction, Amino Acids, 2008, 35(1), 83–88 CrossRef CAS PubMed.
- S. Naik, M. Kumaravel, J. T. Mague and M. S. Balakrishna, Novel Trisphosphine Ligand Containing 1,3,5-Triazine Core, [2,4,6-C3N3{C6H4PPh2-p}3]: Synthesis and Transition Metal Chemistry, Inorg. Chem., 2014, 53(3), 1370–1381 CrossRef CAS PubMed.
- S. Kotha and S. Todeti, Synthesis of C3-symmetric star-shaped molecules containing 1,3-azoles via hetero-aryl Heck coupling, Tetrahedron, 2019, 75(10), 1359–1363 CrossRef CAS.
- K. Do, H. Choi, K. Lim, H. Jo, J. W. Cho, M. K. Nazeeruddin and J. Ko, Star-shaped hole transporting materials with a triazine unit for efficient perovskite solar cells, Chem. Commun., 2014, 50(75), 10971–10974 RSC.
- B. G. Kim, S. Kim and S. Y. Park, Star-shaped discotic nematic liquid crystal containing 1,3,5-triethynylbenzene and oxadiazole-based rigid arms, Tetrahedron Lett., 2001, 42(14), 2697–2699 CrossRef CAS.
- R. Matusiak and A. Keller, New catalyst systems for polymerization of substituted acetylenes: W(NO)2(O2CR)2-MCl4M=Ti, Sn, Polym. Bull., 1999, 43(2–3), 199–206 CrossRef CAS.
- B. Z. Tang, Construction of functional polymers from acetylenic triple-bond building blocks, Macromol. Chem. Phys., 2008, 209(13), 1303–1307 CrossRef CAS.
- M. Häußler, A. Qin and B. Z. Tang, Acetylenes with multiple triple bonds: a group of versatile An-type building blocks for the construction of functional hyperbranched polymers, Polymer, 2007, 48(21), 6181–6204 CrossRef.
- M. Haussier, J. Liu, R. Zheng, J. W. Y. Lam, A. Qin and B. Z. Tang, Synthesis, thermal stability, and linear and nonlinear optical properties of hyperbranched polyarylenes containing carbazole and/or fluorene moieties, Macromolecules, 2007, 40(6), 1914–1925 CrossRef.
- M. Häußler, J. Liu, J. W. Y. Lam, A. Qin, R. Zheng and B. Z. Tang, Polycyclotrimerization of aromatic diynes: Synthesis, thermal stability, and light-emitting properties of hyperbranched polyarylenes, J. Polym. Sci., Part A: Polym. Chem., 2007, 45(18), 4249–4263 CrossRef.
- R. Zheng, H. Dong, H. Peng, J. W. Y. Lam and B. Z. Tang, Construction of hyperbranched poly(alkenephenylene)s by diyne polycyclotrimerization: single-component catalyst, glycogen-like macromolecular structure, facile thermal curing, and strong thermolysis resistance, Macromolecules, 2004, 37(14), 5196–5210 CrossRef CAS.
- H. Dong, R. Zheng, J. W. Y. Lam, M. Häussler, A. Qin and B. Z. Tang, A new route to hyperbranched macromolecules: Syntheses of photosensitive poly(aroylarylene)s via 1,3,5-regioselective polycyclotrimerization of bis(aroylacetylene)s, Macromolecules, 2005, 38(15), 6382–6391 CrossRef CAS.
- A. Qin, et al., Metal-free, regioselective diyne polycyclotrimerization: synthesis, photoluminescence, solvatochromism, and two-photon absorption of a triphenylamine-containing hyperbranched poly(aroylarylene), Macromolecules, 2007, 40(14), 4879–4886 CrossRef CAS.
- C. K. W. Jim, et al., Facile polycyclotrimerization of ‘simple’ arylene bipropiolates: a metal-free, regioselective route to functional hyperbranched polymers with high optical transparency, tunable refractive index, low chromatic aberration, and photoresponsive patternability, Macromolecules, 2009, 42(12), 4099–4109 CrossRef CAS.
- M. Rose, et al., A new route to porous monolithic organic frameworks via cyclotrimerization, J. Mater. Chem., 2011, 21(3), 711–716 RSC.
- Y. C. Zhao, et al., Thionyl chloride-catalyzed preparation of microporous organic polymers through aldol condensation, Macromolecules, 2011, 44(16), 6382–6388 CrossRef CAS.
- X. Zhu, et al., New tricks for old molecules: development and application of porous N-doped, carbonaceous membranes for CO2 separation, Adv. Mater., 2013, 25(30), 4152–4158 CrossRef CAS PubMed.
- Z. H. Guo, C. Wang, Q. Zhang, S. Che, H. C. Zhou and L. Fang, Cost-effective synthesis and solution processing of porous polymer networks through methanesulfonic acid-mediated aldol triple condensation, Mater. Chem. Front., 2018, 2(2), 396–401 RSC.
- C. Wang, et al., Aromatic porous polymer network membranes for organic solvent nanofiltration under extreme conditions, J. Mater. Chem. A, 2020, 8(31), 15891–15899 RSC.
- J. Kim, et al., Conjugated Microporous Polymers via Solvent-Free Ionothermal Cyclotrimerization of Methyl Ketones, Chem. Mater., 2021, 33(21), 8334–8342 CrossRef CAS.
- P. Kuhn, M. Antonietti and A. Thomas, Porous, covalent triazine-based frameworks prepared by ionothermal synthesis, Angew. Chem., Int. Ed., 2008, 47(18), 3450–3453 CrossRef CAS PubMed.
- W. Zhang, et al., Highly energy- and time-efficient synthesis of porous triazine-based framework: microwave-enhanced ionothermal polymerization and hydrogen uptake, J. Mater. Chem., 2010, 20(31), 6413–6415 RSC.
- S. Ren, et al., Porous, fluorescent, covalent triazine-based frameworks via room-temperature and microwave-assisted synthesis, Adv. Mater., 2012, 24(17), 2357–2361 CrossRef CAS PubMed.
- X. Zhu, et al., A superacid-catalyzed synthesis of porous membranes based on triazine frameworks for CO2 separation, J. Am. Chem. Soc., 2012, 134(25), 10478–10484 CrossRef CAS PubMed; C. Y. K. Chan, J. W. Y. Lam, C. K. W. Jim, H. H. Y. Sung, I. D. Williams and B. Z. Tang, Polycyclotrimerization of Dinitriles: A New Polymerization Route for the Construction of Soluble Nitrogen-Rich Polytriazines with Hyperbranched Structures and Functional Properties, Macromolecules, 2013, 46(24), 9494–9506 CrossRef.
- B. Yang, et al., Synthesis of surface covalent organic frameworks via dimerization and cyclotrimerization of acetyls, J. Am. Chem. Soc., 2015, 137(15), 4904–4907 CrossRef CAS PubMed.
- W. Huang, et al., Hollow nanoporous covalent triazine frameworks: Via acid vapor-assisted solid phase synthesis for enhanced visible light photoactivity, J. Mater. Chem. A, 2016, 4(20), 7555–7559 RSC.
- K. Wang, et al., Covalent Triazine Frameworks via a Low-Temperature Polycondensation Approach, Angew. Chem., Int. Ed., 2017, 56(45), 14149–14153 CrossRef CAS PubMed.
- S. Y. Yu, et al., Direct Synthesis of a Covalent Triazine-Based Framework from Aromatic Amides, Angew. Chem., Int. Ed., 2018, 57(28), 8438–8442 CrossRef CAS PubMed.
- M. Liu, et al., Crystalline Covalent Triazine Frameworks by In Situ Oxidation of Alcohols to Aldehyde Monomers, Angew. Chem., Int. Ed., 2018, 57(37), 11968–11972 CrossRef CAS PubMed.
- A. Modak, J. Mondal, M. Sasidharan and A. Bhaumik, Triazine functionalized ordered mesoporous polymer: a novel solid support for Pd-mediated C–C cross-coupling reactions in water, Green Chem., 2011, 13(5), 1317–1331 RSC.
- S. Pourebrahimi and M. Pirooz, Synthesis of a novel freestanding conjugated triazine-based microporous membrane through superacid-catalyzed polymerization for superior CO2 separation, Chem. Eng. J. Adv., 2022, 11, 100315 CrossRef CAS.
- Y. Zhang, et al., Gas uptake, molecular sensing and organocatalytic performances of a multifunctional carbazole-based conjugated microporous polymer, J. Mater. Chem. A, 2014, 2(33), 13422–13430 RSC.
- Z. Chen, C. Su, X. Zhu, R. Xu, L. Xu and C. Zhang, Micro-/Mesoporous conjugated polymer based on star-shaped triazine-functional triphenylamine framework as the performance-improved cathode of Li-organic battery, J. Polym. Sci., Part A: Polym. Chem., 2018, 56(22), 2574–2583 CrossRef CAS.
- T. Y. Wu, Y. S. Su and J. C. Chang, Dithienylpyrrole- and tris[4-(2-thienyl)phenyl]amine-containing copolymers as promising anodic layers in high-contrast electrochromic devices, Coatings, 2018, 8(5), 164 CrossRef.
- K. R. Idzik, R. Beckert, S. Golba, P. Ledwon and M. Lapkowski, Synthesis by Stille cross-coupling procedure and electrochemical properties of C3-symmetric oligoarylobenzenes, Tetrahedron Lett., 2010, 51(18), 2396–2399 CrossRef CAS.
- G. Yang, H. Han, C. Du, Z. Luo and Y. Wang, Facile synthesis of melamine-based porous polymer networks and their application for removal of aqueous mercury ions, Polymer, 2010, 51(26), 6193–6202 CrossRef CAS.
- M. G. Schwab, B. Fassbender, H. W. Spiess, A. Thomas, X. Feng and K. Müllen, Catalyst-free preparation of melamine-based microporous polymer networks through Schiff base chemistry, J. Am. Chem. Soc., 2009, 131(21), 7216–7217 CrossRef CAS PubMed.
- M. X. Tan, Y. Zhang and J. Y. Ying, Mesoporous poly(melamine-formaldehyde) solid sorbent for carbon dioxide capture, ChemSusChem, 2013, 6(7), 1186–1190 CrossRef CAS PubMed.
- M. A. Hussein, M. A. Abdel-Rahman and A. A. Geies, New heteroaromatic polyazomethines containing naphthyridine moieties: Synthesis, characterization, and biological screening, J. Appl. Polym. Sci., 2012, 126(1), 2–12 CrossRef CAS.
- M. Kohlmayr, G. Zuckerstätter and A. Kandelbauer, Modification of melamine-formaldehyde resins by substances from renewable resources, J. Appl. Polym. Sci., 2012, 124(6), 4416–4423 CAS.
- M. G. Schwab, D. Crespy, X. Feng, K. Landfester and K. Müllen, Preparation of microporous melamine-based polymer networks in an anhydrous high-temperature miniemulsion, Macromol. Rapid Commun., 2011, 32(22), 1798–1803 CrossRef CAS PubMed.
- M. X. Tan, Y. N. Sum, J. Y. Ying and Y. Zhang, A mesoporous poly-melamine-formaldehyde polymer as a solid sorbent for toxic metal removal, Energy Environ. Sci., 2013, 6(11), 3254–3259 RSC.
- S. Zulfiqar, M. I. Sarwar and C. T. Yavuz, Melamine based porous organic amide polymers for CO2 capture, RSC Adv., 2014, 4(94), 52263–52269 RSC.
- M. R. Liebl and J. Senker, Microporous functionalized triazine-based polyimides with high CO2 capture capacity, Chem. Mater., 2013, 25(6), 970–980 CrossRef CAS.
- P. Puthiaraj, S. S. Kim and W. S. Ahn, Covalent triazine polymers using a cyanuric chloride precursor via Friedel-Crafts reaction for CO2 adsorption/separation, Chem. Eng. J., 2016, 283, 184–192 CrossRef CAS.
- H. Lim, M. C. Cha and J. Y. Chang, Preparation of microporous polymers based on 1,3,5-triazine units showing high CO 2 adsorption capacity, Macromol. Chem. Phys., 2012, 213(13), 1385–1390 CrossRef CAS.
- S. Xiong, X. Fu, L. Xiang, G. Yu, J. Guan, Z. Wang, Y. Du, X. Xiong and C. Pan, Liquid acid-catalysed fabrication of nanoporous 1,3,5-triazine frameworks with efficient and selective CO2 uptake, Polym. Chem., 2014, 5(10), 3424–3431 RSC.
- X. Zhu, et al., Efficient CO2 capture by a task-specific porous organic polymer bifunctionalized with carbazole and triazine groups, Chem. Commun., 2014, 50(59), 7933–7936 RSC.
- S. Ren, et al., Functional conjugated microporous polymers: From 1,3,5-benzene to 1,3,5-triazine, Polym. Chem., 2012, 3(4), 928–934 RSC.
- Z. Xiang and D. Cao, Synthesis of luminescent covalent-organic polymers for detecting nitroaromatic explosives and small organic molecules, Macromol. Rapid Commun., 2012, 33(14), 1184–1190 CrossRef CAS PubMed.
- M. Häussler, R. Zheng, J. W. Y. Lam, H. Tong, H. Dong and B. Z. Tang, Hyperbranched polyenes: syntheses, photoluminescence, light refraction, thermal curing, metal complexation, pyrolytic ceramization, and soft magnetization, J. Phys. Chem. B, 2004, 108(30), 10645–10650 CrossRef.
- H. Bohra, P. Li, C. Yang, Y. Zhao and M. Wang, ‘greener’ and modular synthesis of triazine-based conjugated porous polymers: via direct arylation polymerization: Structure-function relationship and photocatalytic application, Polym. Chem., 2018, 9(15), 1972–1982 RSC.
- A. Qin, et al., Click polymerization: facile synthesis of functional poly(aroyltriazole)s by metal-free, regioselective 1,3-dipolar polycycloaddition, Macromolecules, 2007, 40(7), 2308–2317 CrossRef CAS.
- A. Qin, et al., Hyperbranched polytriazoles: click polymerization, regioisomeric structure, light emission, and fluorescent patterning, Macromolecules, 2008, 41(11), 3808–3822 CrossRef CAS.
- L. Zhang, et al., Ruthenium-catalyzed cycloaddition of alkynes and organic azides, J. Am. Chem. Soc., 2005, 127(46), 15998–15999 CrossRef CAS PubMed.
- H. Zhao, Z. Jin, H. Su, X. Jing, F. Sun and G. Zhu, Targeted synthesis of a 2D ordered porous organic framework for drug release, Chem. Commun., 2011, 47(22), 6389–6391 RSC.
- L. Bai, et al., Nanoscale covalent organic frameworks as smart carriers for drug delivery, Chem. Commun., 2016, 52(22), 4128–4131 RSC.
- V. S. Vyas, et al., Exploiting Noncovalent Interactions in an Imine-Based Covalent Organic Framework for Quercetin Delivery, Adv. Mater., 2016, 28(39), 8749–8754 CrossRef CAS PubMed.
- S. Liu, C. Hu, Y. Liu, X. Zhao, M. Pang and J. Lin, One-Pot Synthesis of DOX@Covalent Organic Framework with Enhanced Chemotherapeutic Efficacy, Chem.–Eur. J., 2019, 25(17), 4315–4319 CrossRef CAS PubMed.
- S. Liu, J. Yang, R. Guo, L. Deng, A. Dong and J. Zhang, Facile Fabrication of Redox-Responsive Covalent Organic Framework Nanocarriers for Efficiently Loading and Delivering Doxorubicin, Macromol. Rapid Commun., 2020, 41(4), 1–6 CrossRef CAS PubMed.
- Y. Cao, et al., Dual-Functionalized Covalent Triazine Framework Nanosheets as Hierarchical Nonviral Vectors for Intracellular Gene Delivery, ACS Appl. Nano Mater., 2021, 4(5), 4948–4955 CrossRef CAS.
- Q. Guan, et al., BODIPY-Decorated Nanoscale Covalent Organic Frameworks for Photodynamic Therapy, iScience, 2019, 14, 180–198 CrossRef CAS PubMed.
- C. Hu, Z. Zhang, S. Liu, X. Liu and M. Pang, Monodispersed CuSe Sensitized Covalent Organic Framework Photosensitizer with an Enhanced Photodynamic and Photothermal Effect for Cancer Therapy, ACS Appl. Mater. Interfaces, 2019, 11(26), 23072–23082 CrossRef CAS PubMed.
- C. Hu, L. Cai, S. Liu and M. Pang, Integration of a highly monodisperse covalent organic framework photosensitizer with cation exchange synthesized Ag2Se nanoparticles for enhanced phototherapy, Chem. Commun., 2019, 55(62), 9164–9167 RSC.
- S. B. Wang, et al., Remodeling extracellular matrix based on functional covalent organic framework to enhance tumor photodynamic therapy, Biomaterials, 2020, 234, 119772 CrossRef CAS PubMed.
- J. Lu, et al., Functionalization of Covalent Organic Frameworks with DNA via Covalent Modification and the Application to Exosomes Detection, Anal. Chem., 2022, 94(12), 5055–5061 CrossRef CAS PubMed.
- M. Wang, et al., Detection of colorectal cancer-derived exosomes based on covalent organic frameworks, Biosens. Bioelectron., 2020, 169, 112638 CrossRef CAS PubMed.
- B. Luo, J. He, Z. Li, F. Lan and Y. Wu, Glutathione-Functionalized Magnetic Covalent Organic Framework Microspheres with Size Exclusion for Endogenous Glycopeptide Recognition in Human Saliva, ACS Appl. Mater. Interfaces, 2019, 11(50), 47218–47226 CrossRef CAS PubMed.
- P. Gao, et al., Multicolor Covalent Organic Framework-DNA Nanoprobe for Fluorescence Imaging of Biomarkers with Different Locations in Living Cells, Anal. Chem., 2021, 93(40), 13734–13741 CrossRef CAS PubMed.
|
This journal is © The Royal Society of Chemistry 2023 |