DOI:
10.1039/D3RA01229H
(Paper)
RSC Adv., 2023,
13, 14855-14862
Metabolic profiling and biological activity of two Livistona species: L. chinensis and L. australis†
Received
23rd February 2023
, Accepted 26th April 2023
First published on 15th May 2023
Abstract
Livistona is a genus of family Arecaceae and widely grown in tropical areas. The phytochemical analysis of the leaves and fruits of two Livistona species, L. chinensis and L. australis were carried out using UPLC/MS and determination of the total phenolic and total flavonoid contents, in addition to the isolation and identification of five phenolic compounds and one fatty acid from L. australis fruits. The total phenolic compounds varied from 19.72 to 78.87 mg GAE g−1 dry plant, while the total flavonoid contents were in the range of 4.82–17.75 mg RE g−1 dry plant. The UPLC/MS analysis of the two species led to the characterization of forty-four metabolites belonging mainly to the different classes of flavonoids and phenolic acids, while the compounds isolated from L. australis fruits were identified as gallic acid, vanillic acid, protocatechuic acid, hyperoside, quercetin 3-O-α-D-arabinopyranoside and dodecanoic acid. The in vitro biological evaluation of L. australis leaves and fruits were estimated as anticholinesterase, telomerase reverse transcriptase (TERT) potentiation and anti-diabetic through measuring the capacity of the extracts to inhibit dipeptidyl peptidase (DPP-IV). The results revealed that the leaves showed remarkable anticholinesterase and antidiabetic activity compared to fruits with IC50 values of 65.55 ± 3.75 ng mL−1 and 90.8 ± 4.48 ng mL−1, respectively. In the TERT enzyme assay, the leaves extract triggered a 1.49-fold increase in telomerase activity. This work showed that the Livistona species are a good source for flavonoids and phenolics, which play an important role in anti-aging and the treatment of chronic diseases, such as diabetes and Alzheimer's.
1. Introduction
Alzheimer's disease (AD) is a neurodegenerative disorder and is considered to be the main cause of dementia. Worldwide, about 40 million people are expected to suffer from dementia, and this number is expected to be doubled by 2050.1 A low level of acetylcholine in the brain is the key marker of the disease. Inhibition of the acetylcholinesterase (AChE) enzyme, which is responsible for the degradation of acetylcholine, is the most promising approach for the treatment of AD. Many natural compounds, such as terpenoids, coumarins, flavonoids, alkaloids and other phenolic compounds, have shown anticholinesterase activity.2
Another sign associated with aging is telomere attrition. At the ends of linear chromosomes are DNA repeats called telomeres, which ensure chromosome stability throughout replication. Every time a cell divides, as well as when there is oxidative stress, the telomere length is decreased. When telomeres significantly shorten in length, cells can no longer divide, causing cell death. There is an enzyme called telomerase, which creates the particular DNA sequence at the telomeres.3–7 The substances that potentiate telomerase are called telomerase activators and are used as antiageing sources.
Another heath problem that has an impact on human health is T2DM. The number of patients expected to suffer from diabetes in 2040 will be 642 million worldwide, according to the International Diabetes Federation.8 Lowering the blood glucose level is the primary treatment of T2DM, which can be done through α-glucosidase inhibitors or the stimulation of insulin secretion.9 Incretins are hormones that are released from the small intestine in response to dietary intake, and have an impact similar to glucagon. The main incretins are a glucagon-like peptide (GLP-1) and a glucose-dependent insulinotropic peptide (GIP). These incretins potentiate insulin secretion from pancreatic B-cells.10 Dipeptidyl peptidase-IV is responsible for the degradation of these incretins. Thus, DPP-IV inhibitors prevent the degradation of these incretins, which led to an increase in the insulin level and lowering of the blood glucose level.11
Livistona is a genus belonging to the family Arecaceae and it is widely distributed in tropical areas, with about 36 species reported in the genus. Phytochemical studies on Livistona have reported on many compounds, such as flavonoids, phenolics, ceramides and glycerides,12–14 pyranone derivatives15 and sulfated flavonoids.16 Biologically, L. chinensis has been reported to have anticancer17 and cardioprotective18 properties, while L. decipiens has been reported to have antioxidant and cytotoxic activities.19 A recent report on the leaves and fruit extracts of the plant also showed inhibitory activity against coronavirus.20 The fruits of L. chinensis are edible in eastern Asia, they are a component of prepared soups used in the treatment of liver cancer and chronic hepatitis.21 The nutritional evaluation of the leaves and fruits of L. australis also showed that the fruits are a good source of calcium and potassium.22 This work aims to identify the secondary metabolites in the leaves and fruits of L. chinensis and L. australis using UPLC coupled with high-resolution electron spray ionization mass (HRESIMS), and evaluate their anticholinesterase, DPP-IV inhibitory potential, and telomerase activation potential.
2. Experimental
2.1. Plant material
The fruits and leaves of Livistona australis and Livistona chinensis were supplied from El-Orman Botanical Garden, Giza, Egypt on Jan. 2018. The samples were authenticated by Eng. Trease Labib (El Orman Botanical Garden) and Dr Reem Samir Hamdy (Plant Taxonomy, Botany Department, Faculty of Science, Cairo University, Giza, Egypt). Voucher specimens (BUPD-71-2018 for L. australis and BUPD-73-2018 for L. chinensis) were kept in the Pharmacognosy Department, Faculty of Pharmacy, Beni-Suef University, Egypt. The plants were cleaned, dried and finely powdered for the study.
2.2. Chemicals and reagents
Solvents such as n-hexane, dichloromethane (CH2Cl2), ethyl acetate (EtOAc), ethanol (EtOH), and methanol (MeOH), were obtained from the El-Nasr Company for Pharmaceuticals and Chemicals, Egypt. Acetonitrile, methanol (HPLC grade) and DMSO were purchased from (Sigma-Aldrich, St. Louis, MO, USA). Acetyl-cholinesterase inhibitor screening kits (cat. # IACE-100) were purchased from BioAssay Systems (Hayward, CA, USA). Donepezil (Cat # sc-218265) was purchased from Santa Cruz Biotechnology (Texas, USA). TERT ELISA kit (cat. # K4187-100) was purchased from Biovision incorporated (Milpitas, CA, USA), The curcumin standard for the TERT assay was obtained from Sigma-Aldrich, Germany.
2.3. Apparatus
The NMR analysis for the isolated compounds was carried out using Bruker Avance III 400 MHz (Bruker, USA). LC/MS analysis of the extracts was done on an Acquity Ultra Performance Liquid Chromatography (UPLC) system connected with a Synapt G2 HDMS quadrupole time-of-flight hybrid mass spectrometer (Waters, USA). Spectrophotometric determination of the total phenolic and flavonoid contents was measured using a Jenway 6305 spectrophotometer.
2.4. Metabolomics study
Three grams of each sample were exhaustively defatted using n-hexane (3 × 15 mL). The marc was then extracted with 70% ethanol, filtered with Whatman filter paper, and evaporated under vacuum to afford the dry residue. One mg of each extract was dissolved in 1 mL methanol and analyzed using LC/MS, as reported by Owis et al., 2020.23 Briefly, the samples were analyzed using a C-18 column (2.1 × 100 mm, 1.7 μm particle size; Waters, Milford, MA, USA). A binary solvent was used for separation; solvent A was 0.1% formic acid in water, while solvent B was acetonitrile, and a gradient elution from 0–100% of solvent B in 6 min with flow rate 0.3 mL min−1 was followed. Electron spray ionization mass spectrometry was used to detect the metabolites; the processed data set was subjected to molecular formula prediction, as well as peak identification. The positive and negative ionization mode data sets from the plant extract were dereplicated against the Dictionary of Natural Products (DNP) database.
2.5. Determination of total phenolics and total flavonoids contents
The fruits and leaves of L. australis and L. chinensis at 1 g each were extracted with 50 mL of 80% methanol for 2 h on an orbital shaker set at 200 rpm. Each mixture was separately centrifuged for 20 min, and the supernatants were decanted. The residues were re-extracted under the same conditions. Supernatants were separately combined. The volume was adjusted to 100 mL; these extracts were used for total phenolic and total flavonoid determination. The total phenolic content was assessed using Folin–Ciocalteu reagent as follows.24 A volume of 300 μL of each extract was mixed with 2.25 mL Folin reagent. After 5 minutes, 2.25 mL of 6% sodium carbonate was added. The mixture was rested for 90 min, and the blue color that developed was measured at 725 nm. The results were expressed as mg gallic acid equivalents per g dried sample (mg GAE g−1). Meanwhile, the flavonoid content was assessed by colorimetric method.24 Briefly, 0.5 mL of each extract was diluted with 2.25 mL distilled water, and then 150 μL of 5% NaNO2 was added. Six min later, 300 μL of 10% AlCl3 was mixed and the reaction was rested for 6 min. Finally, 1 mL of 1 M NaOH was added. The mixture was mixed well and the absorbance was measured at 510 nm. Results of the total flavonoids were expressed as mg rutin equivalents per g of dried sample (mg RE g−1).
2.6. Extraction and fractionation
Air-dried, powdered pericarp of L. australis (1.75 kg) was extracted with 70% ethanol (5 L) by cold maceration for 72 h, and then filtered through filter paper. The marc was re-extracted again until exhaustion, and the collected extracts was dried under vacuum at 45 °C to afford the crude extract (340 g). A part of the dried extract (200 g) was fractionated using n-hexane, dichloromethane and ethyl acetate to give 22, 14 and 6 g, respectively. The n-hexane fraction (6 g) was chromatographed on a silica gel (E-Merck, Germany) column using hexane with 10% ethyl acetate increments to afford one major compound 1 (500 mg). The ethyl acetate fraction (5 g) was chromatographed on a polyamide (E-Merck, Germany) column starting with 100% water as the eluent, then with decreasing polarity by 20% MeOH to afford five sub-fractions. The sub-fraction eluted with 20% water (500 mg) was re-chromatographed on a Sephadex LH-20 column using methanol to afford compounds 2 and 3 (25, 10 mg, respectively). The sub-fraction eluted with 60% water (100 mg) was re-chromatographed on a Sephadex LH-20 column (Sigma-Aldrich, USA) using methanol as an eluent to afford three compounds 4–6 (15, 12, 20 mg, respectively).
2.7. Biological studies
2.7.1. Anticholinesterase activity. The anticholinesterase potential of L. australis leaves and fruits extracts, as well as donepezil standard, were measured utilizing Ellman's method.25 The method depends on the cleavage of acetylthiocholine substrate into thiocholine by the acetylcholinesterase enzyme. Thiocholine reacts with Ellman's reagent [5,5′-dithiobis(2-nitrobenzoic acid), known as DTNB], to give 2-nitrobenzoate-5-mercaptothiocholine and 5-thio-2-nitrobenzoate, which can be detected at 412 nm. The experiment was done according to the manufacturer's instructions in triplicate, and the IC50 values for the tested extracts were calculated as follows; a mixture of 25 μL of acetylthiocholine iodide (5 mM), 125 μL of DTNB (3 mM), 50 μL of buffer B (50 mM Tris–HCl, pH 8, 0.1% BSA) and 25 μL of each test extract solution at different concentrations were added. The mixture was then incubated for 10 min at 37 °C, then 25 μL of AChE (0.05 U mL−1) was added to start the reaction. A negative control (25% DMSO in MeOH) was used.
2.7.2. Antiaging activity. In vitro assay to measure TERT in response to the tested extracts and curcumin standard was carried out using the human fibroblast cell line (HFB4), which is known as the normal human skin melanocyte cell line. The assay was carried out according to the method reported by Refaey, Abdelhamid et al. 2021.26 HFB4 was obtained from (VACSERA, Giza, Egypt) and cultured in RPMI-1640 media supplemented with 10% heat-inactivated FBS, 100 U mL−1 penicillin, and 100 U mL−1 streptomycin. In a six well plate, the cells were plated with a density of 2 × 105 cells per well and incubated at 37 °C for 24 h in a humid atmosphere of 5% CO2. The tested extracts and curcumin (positive control) were added to the cells. After 24 h, the supernatant was collected and centrifuged for 20 min, which was then used to carry out the assay. The collected supernatants were added to precoated microtiter plates with a biotin-detection antibody specific to TERT, then streptavidin conjugated to horse-radish peroxidase (SABC) was added to each microplate well and incubated. The color of the solutions in the wells containing TERT, the antibody and enzyme-conjugated streptavidin will change after the addition of 3,3′,5,5′-tetramethylbenzidine (TMB) substrate solution. The stop solution was used to stop the enzyme–substrate reaction, and the color change at 450 nm was determined spectrophotometrically. The optical density of the samples was then compared to the standard curve in order to determine the TERT concentration in the samples.
2.7.3. Antidiabetic activity. The antidiabetic potential of the leaves and fruits extract was assessed by measuring the ability of the extract to inhibit DPP-IV using the DPP-IV inhibition screening kit (Catalog Number KA3738, Abnova, Taiwan). The assay depends on the ability of DPP-IV to break down a fluorogenic substrate, Gly-Pro-Aminomethylcoumarin, and release products measured by fluorometer (Em/Ex = 360/460 nm). In the presence of a DPP-IV inhibitor, the cleavage will be inhibited. The procedure of the experiment was done according to the supplier instructions, and as reported by Kempegowda, Zameer et al. 2018.27 Different concentrations of the tested extracts were dissolved in DMSO. A volume of 10 μL of the sample solution was mixed with 30 μL assay buffer and 10 μL enzyme solution in a 96-well plate, then 50 μL of a diluted substrate solution was added to start the reaction and incubated at 37 °C for 30 min. Fluorescence with an excitation wavelength of 360 nm and an emission wavelength of 460 nm was monitored using a plate reader. The IC50 for the tested extracts and sitagliptin was calculated.
2.8. Statistical analysis
Data were presented as the mean ± standard deviation (SD). All analyses were done in triplicate. Statistical and graphical evaluations were done using Graph Pad Prism 7 and Microsoft Excel 2010.
3. Results and discussion
3.1. Metabolic analysis
Metabolic profiling of the secondary metabolites in L. chinensis and L. australis leaves and fruits were conducted using LC-HRESIMS (chromatograms in ESI data, Fig. S1–S8†) for dereplication/detection of the putative compounds responsible for the various activities assigned to Livistona. It led to the characterization of forty-four compounds (Table 1, Fig. 1) belonging to different classes of flavonoids and phenolic acids.
Table 1 LC-HR-ESIMS dereplication results of the alcoholic extract of L. chinensis and L. australis leaves and fruitsa
Metabolites name |
L. chinensis |
L. australis |
MF |
m/z |
L |
F |
L |
F |
L: leaves, F: fruits, MF: molecular formula, ND: not detected. |
Syringic acid |
+ |
+ |
+ |
+ |
C9H10O5 |
197.0447 |
4-Hydroxybenzoic acid |
ND |
+ |
ND |
+ |
C7H6O3 |
137.0238 |
Protocatechuic acid |
+ |
+ |
+ |
+ |
C7H6O4 |
153.0189 |
Rutin |
ND |
ND |
ND |
+ |
C27H30O16 |
609.1455 |
Chlorogenic acid |
+ |
+ |
+ |
+ |
C16H18O9 |
353.0881 |
Cryptochlorogenic acid |
+ |
+ |
+ |
+ |
C16H18O9 |
353.0881 |
Neochlorogenic acid |
+ |
+ |
+ |
+ |
C16H18O9 |
353.0881 |
Vanillic acid |
+ |
+ |
+ |
+ |
C8H8O4 |
167.0342 |
Isovanillic acid |
+ |
+ |
+ |
+ |
C8H8O4 |
167.0342 |
Vicenin II |
+ |
+ |
ND |
ND |
C27H30O15 |
593.1514 |
Isoorientin-7-O-sulfate |
+ |
+ |
+ |
ND |
C21H20O14S |
527.0501 |
Orientin-7-O-sulfate |
+ |
+ |
+ |
ND |
C21H20O14S |
527.0501 |
Orientin |
+ |
+ |
+ |
+ |
C21H20O11 |
447.0931 |
Isoorientin |
+ |
+ |
+ |
+ |
C21H20O11 |
447.0931 |
Cynaroside |
+ |
+ |
+ |
+ |
C21H20O11 |
447.0932 |
Caffeic acid |
+ |
+ |
+ |
+ |
C9H8O4 |
179.0346 |
5-O-Caffeoylshikimic acid |
+ |
+ |
ND |
+ |
C16H16O8 |
335.0769 |
3-O-Caffeoylshikimic acid |
+ |
+ |
ND |
+ |
C16H16O8 |
335.0769 |
Schaftoside |
+ |
+ |
+ |
ND |
C26H28O14 |
563.1405 |
Isoschaftoside |
+ |
+ |
+ |
ND |
C26H28O14 |
563.1405 |
(+)-Catechin |
ND |
+ |
+ |
+ |
C15H14O6 |
289.0713 |
(−)-Catechin |
ND |
+ |
+ |
+ |
C15H14O6 |
289.0713 |
Epicatechin |
ND |
+ |
+ |
+ |
C15H14O6 |
289.0713 |
Quercitrin |
+ |
+ |
+ |
+ |
C21H20O11 |
447.0929 |
Isovitexin 7-O-sulfate |
+ |
+ |
+ |
ND |
C21H20O13S |
511.0558 |
Vitexin 7-O-sulfate |
+ |
+ |
+ |
ND |
C21H20O13S |
511.0558 |
Isovitexin |
+ |
+ |
ND |
ND |
C21H20O10 |
431.0974 |
Vitexin |
+ |
+ |
ND |
ND |
C21H20O10 |
431.0974 |
Hyperoside |
ND |
+ |
+ |
+ |
C21H20O12 |
463.0876 |
Isoquercetin |
ND |
+ |
+ |
+ |
C21H20O12 |
463.0876 |
Quercetin |
ND |
ND |
ND |
+ |
C15H10O7 |
301.0351 |
Avicularin |
ND |
ND |
ND |
+ |
C20H18O11 |
433.0977 |
Quercetin 3-O-α-D-arabinopyranoside |
ND |
ND |
ND |
+ |
C20H18O11 |
433.0977 |
Tricin 7-O-β-glucopyranoside-2′′-sulphate sodium salt |
+ |
ND |
+ |
ND |
C23H24O15S |
571.0759 |
Isorhamnetin-3-O-β-D-glucopyranoside |
ND |
+ |
ND |
+ |
C22H22O12 |
477.1032 |
cis-3,5,3′,5′-Tetrahydroxy-4′-methoxystilbene |
ND |
+ |
ND |
+ |
C15H14O5 |
273.0766 |
trans-3,5,3′,5′-Tetrahydroxy-4′-methoxystilbene |
ND |
+ |
ND |
+ |
C15H14O5 |
273.0766 |
(−)-Epiafzelechin |
ND |
+ |
ND |
+ |
C15H14O5 |
273.0766 |
Luteolin |
+ |
+ |
+ |
+ |
C15H10O6 |
285.0395 |
Homovanillic acid |
+ |
+ |
+ |
+ |
C9H10O4 |
181.0498 |
Naringenin |
ND |
+ |
ND |
+ |
C15H12O5 |
271.0599 |
7-O-Methylluteolin |
+ |
+ |
ND |
+ |
C16H12O6 |
299.0558 |
Chrysoeriol |
+ |
+ |
ND |
+ |
C16H12O6 |
299.0558 |
Tricin |
+ |
+ |
+ |
+ |
C17H14O7 |
329.0658 |
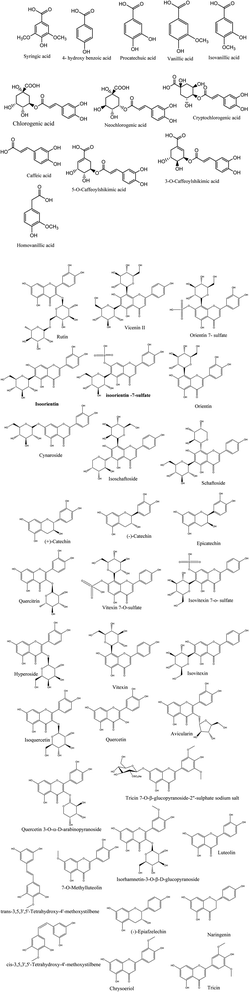 |
| Fig. 1 Structures of the dereplicated metabolites from the alcoholic extract of L. chinensis and L. australis leaves and fruits. | |
3.1.1. Phenolic acids. Twelve phenolic acids (six hydroxybenzoic acids and six hydroxycinnamic acids) were annotated in the extracts of the leaves and fruits of L. chinensis and L. australis. The hydroxybenzoic acids were identified as syringic acid, 4-hydroxy benzoic acid, procatechuic acid, vanillic acid, isovanillic acid and homovanillic acid. They were characterized from the mass ion peaks at m/z 197.0447, 137.0238, 153.0189, 167.0342, 181.0498 and molecular formulas C9H10O5, C7H6O3, C7H6O4, C8H8O4 and C9H10O4, respectively. Syringic acid, procatechuic acid, vanillic acid, isovanillic acid and homovanillic were detected in the leaves and fruit extracts of L. chinensis and L. australis, while 4-hydroxy benzoic acid was detected only in the fruit extract of both plants. These phenolic acids were detected previously in L. chinensis leaves.28 A metabolite with a mass ion peak at m/z 353.0881 and molecular formula of C16H18O9 was detected in the leaves and fruit extracts of both plants, and was annotated as chlorogenic acid or its isomers cryptochlorogenic acid or neochlorogenic acid, which were previously detected in L. chinensis leaves.28 Also caffeic acid was recognized in all analyzed extracts from the mass ion peak at m/z 179.0346 and the molecular formula C9H8O4. 5-O-caffeoylshikimic acid or 3-O-caffeoylshikimic acid was characterized from the mass ion peak at m/z 335.0769 and molecular formula C16H16O8 in the fruit and leaves of L. chinensis and in the fruit extract of L. australis. Caffeic acid and caffeoylshikimic acid were previously reported in L. chinensis fruits.29
3.1.2. Flavonoids. Thirty-two flavonoids were characterized in the leaves and fruit extracts of L. chinensis and L. australis. The metabolite with the mass ion peak at m/z 609.1455, in accordance with the molecular formula C27H30O16, was detected in the L. australis fruit extract and recognized as rutin, which was formerly isolated from the L. chinensis fruit extract.30 Another metabolite was detected in the L. chinensis leaves and fruit with molecular ion peak at m/z 593.1514, which is in agreement with the molecular formula C27H30O15 and was annotated as vienin II. This was previously reported in L. decipiens leaves.19 Moreover, the metabolites with the mass ion peaks at m/z 527.0501 and 511.0558 with the suggested molecular formulas C21H20O14S and C21H20O13S were identified as orientin-7-O-sulfate (or its isomer isoorientin-7-O-sulfate) and vitexin 7-O-sulfate (or its isomer isovitexin 7-O-sulfate), respectively. These metabolites were detected in L. chinensis leaves and fruits and L. australis leaves, and were previously reported in L. chinensis leaves.28 The mass ion peaks at m/z 447.0931 and 431.0974 with molecular formulas of C21H20O11 and C21H20O10 were identified as orientin (or its isomer isoorientin) and vitexin (or isovitexin), which are C-glycosyl flavones. Orientin was detected in all tested extracts, while vitexin was detected in L. chinensis leaves and fruits. Both compounds were previously reported in L. chinensis leaves.18 Another metabolite was detected in the leaves and fruits of L. chinensis and L. australis with a molecular ion peak at m/z 447.0932, and was in agreement with the molecular formula C21H20O11. It was identified as cynaroside (luteolin 7-glucoside) and previously reported in L. chinensis leaves.28 The metabolite with mass ion peak at m/z 563.1405 in agreement with the molecular formula C26H28O14 was identified as schaftoside (or isoschaftoside). The metabolite was detected in the leaves and fruits of L. chinensis and L. australis leaves, and was previously reported in L. chinensis leaves.28 The metabolite with mass ion peak at m/z 289.0713 in accordance with the molecular formula C15H14O6 was identified as catechin (flavan-3-ol). The compound was detected in the leaves and fruits of L. australis and L. chinensis fruits. It was previously reported in L. chinensis fruits.30 The metabolites with mass ion peaks at m/z 447.0929, 463.0876, 301.0351 and 433.0977 in accordance with the molecular formulas C21H20O11, C21H20O12, C15H10O7 and C20H18O11 were characterized as quercitrin, hyperoside (quercetin 3-galactoside), isoquercitrin, quercetin and quercetin 3-arabinoside, respectively. Quercitrin was detected in all tested extracts, and previously reported in L. chinensis fruits.30 Meanwhile, hyperoside and isoquercitrin were detected in L. chinensis fruits, L. australis leaves and fruits, they were previously reported in L. australis leaves.16 Quercetin and quercetin 3-arabinoside were detected in L. australis fruits and previously reported in L. australis leaves and Serenoa repens fruit, respectively.16,31 The metabolite with mass ion peak at 571.0759 in agreement with the molecular formula C23H24O15S was identified as tricin 7-O-β-glucopyranoside-2′′-sulphate sodium salt, which was detected in L. chinensis and L. australis leaves and previously reported in L. australis leaves.16 The mass ion peak at m/z 477.1032 and molecular formula C22H22O12 was detected in the fruits of L. chinensis and L. australis, identified as isorhamnetin-3-O-β-D-glucopyranoside, and was previously reported in L. chinensis fruits.30 The metabolite with mass ion peak at m/z 273.0766 and molecular formula C15H14O5 was characterized as 3,5,3′,5′-tetrahydroxy-4′-methoxystilbene or (−)-epiafzelechin. The metabolite was detected in the fruit extracts of both species, and previously reported in L. chinensis fruits.13 The metabolites with mass ion peaks at 285.0395, 271.0599, 299.0558 and 329.0658 in agreement with the molecular formulas C15H10O6, C15H12O5, C16H12O6 and C17H14O7 were identified as luteolin, naringenin, 7-O-methylluteolin, and tricin, respectively. Luteolin and tricin were detected in all analyzed extracts, while naringenin was detected in the fruit extracts of both species. 7-O-Methylluteolin was detected in the leaves and fruits of L. chinensis and L. australis fruits. Luteolin and naringenin were previously reported in L. australis leaves, while 7-O-methylluteolin and tricin were reported in L. chinensis.16,18,28,32
3.2. Total phenolic and total flavonoids content
Folin–Ciocalteu reagent was used to determine the total phenolic content in the fruits and leaves of L. australis and L. chinensis extracts. The Folin–Ciocalteu reagent consists of complex polymeric ions (yellow solution) formed from phosphotungstic and phosphomolybdic heteropolyacids. This reagent produces complex molybdenum-tungsten blue from the oxidation of phenolates, which can be spectrophotometrically detected at 725 nm.24 As shown in Table 2, the order of the phenolic content was L. australis leaves > L. chinensis fruit > L. australis fruits > L. chinensis leaves with the values of 78.87, 44.50, 32.82 and 19.72 mg GAE g−1, respectively.
Table 2 Total phenolic and total flavonoid content of leaves and fruits extracts of L. chinensis and L. australisa
Samples |
Total phenolic (mg GAE g−1) |
Total flavonoid (mg RE g−1) |
Values are presented in mean ± SD (n = 3), expressed as mg gallic acid equivalent per g of dry sample (mg GAE g−1) for total phenolics and as mg rutin equivalent per g of dry sample (mg RE g−1) for total flavonoids. |
L. chinensis leaves |
19.72 ± 3.49 |
4.82 ± 0.29 |
L. chinensis fruits |
44.50 ± 1.60 |
17.75 ± 1.80 |
L. australis leaves |
78.87 ± 0.29 |
8.16 ± 0.35 |
L. australis fruits |
32.82 ± 0.77 |
12.81 ± 0.612 |
Flavonoids are the widely distributed and most common group of plant phenolics, which are characterized by the presence of a benzo-γ-pyrone structure.33 The total flavonoids content was determined in the assigned extracts by reaction with sodium nitrite, followed by the formation of a colored complex of flavonoid-aluminum using aluminum chloride that was spectrophotometrically monitored at 510 nm.24 For both Livistona species, the samples containing the highest flavonoid content was L. chinensis fruit (17.75 mg RE g−1), followed by the L. australis fruit (12.81 mg RE g−1), L. australis leaves (8.16 mg RE g−1) and L. chinensis leaves (4.82 mg RE g−1), as indicated in Table 2.
The total flavonoid and phenolic contents for L. chinensis previously reported and the results were found to be 139.51 ± 5.90 μg g−1 dry plant and 112.07 ± 2.24 μg g−1 dry plant, respectively28 while the L. speciosa seed extract showed a total phenolic content of 2.35 mg of gallic acid/1 g of sample, and the total flavonoid was found 39.27 mg of rutin/1 g of sample.34 In our study, the L. australis leaves had the highest phenolic content, while the L. chinensis fruits showed a high flavonoid content.
3.3. Identification of the isolated compounds
Six known compounds were isolated and identified from the pericarp of L. australis: one fatty acid from the hexane fraction and five phenolic compounds from the ethyl acetate fraction. The chemical structures of the pure isolated compounds were elucidated using NMR spectrometry, and confirmed by comparing the obtained data with those previously reported in the literature (Fig. 2). The identified compounds were:
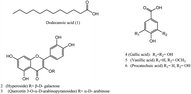 |
| Fig. 2 Structures of the isolated metabolites from the alcoholic extract of L. australis fruits. | |
(1) dodecanoic acid; (lauric acid,C12H24O2),35 (2) hyperoside (quercetin 3-O-β-D-galactopyranoside, C21H20O12),36 (3) quercetin 3-O-α-D-arabinopyranoside (C20H18O11),37 (4) gallic acid (C7H6O5),38 (5) vanillic acid (C8H8O4) and (6) procatechuic acid (C7H6O4).39 These compounds have been isolated and characterized for the first time from L. australis fruits (spectroscopic data in ESI data, Fig. S9–S20†).
3.4. Biological studies
Phenolic compounds have been reported to lower the incidence of many chronic diseases, such as cancer, cardiovascular diseases (CVD), chronic respiratory diseases, diabetes and neurodegenerative diseases.40 In this work, extracts of L. australis showed a higher content of phenolic compounds than L. chinensis, so the biological evaluation was done for L. australis regarding anticholinesterase, and antiaging and antidiabetic properties.
3.4.1. Anticholinesterase activity. A key neurotransmitter in the pathogenesis of Alzheimer's disease (AD) is acetylcholine, which is hydrolyzed by acetyl-cholinesterase (AChE) and butyryl-cholinesterase (BuChE). AD is associated with low level of acetylcholine in specific regions of the brain.41,42 AChE inhibitors, which led to high levels of acetylcholine in the brain, are considered to be the most effective strategy in AD treatment.43,44 Extracts of L. australis leaves and fruits were screened for AChE inhibitory activity using Ellman's spectrophotometric method. The results showed that the inhibitory concentration (IC50) was 65.55 ± 3.75 ng mL−1 and 122 ± 6.97 ng mL−1 for leaves and fruits, respectively, compared with donepezil as a standard with (IC50) at 49.44 ± 2.82 ng mL−1. The results (Table 3) revealed that the leaves extract was active as an antiacetylcholinesterase compared with the standard. Flavonoids are a promising class of natural compounds in the management of AD disease.45 A previous report on the anticholinesterase activity of Sabal blackburniana (Palmae) was performed, and the results showed a good activity of flavonoid aglycone against the acetylcholinesterase enzyme, such as luteolin, taxifolin, tricin, genistein, chrysoeriol and catechin.46 L. australis leaves and fruit extracts contained many flavonoid aglycones, such as catechin, luteolin and tricin. Another report revealed that apigenin, vanillic acid and acacetin-7-O-β-D-glucoside showed potent anticholinesterase activity.26 Finally, it can be concluded that the anti-cholinesterase activity may refer to flavonoids and phenolic acids detected in the analyzed extracts.
Table 3 Anticholinesterase, telomerase activation, and DPP-IV inhibitory activities of alcoholic extract from the leaves and fruits of L. australis
Extract/control |
Anticholinesterase IC50 (ng ml−1) |
Telomerase activation conc (ng ml−1) |
DPP4 inhibition IC50 (ng ml−1) |
L. australis leaves |
65.55 ± 3.75 |
12.23 ± 0.59 (1.49) |
90.8 ± 4.48 |
L. australis fruits |
122 ± 6.97 |
11.5 ± 0.65 (1.41) |
359 ± 17.7 |
Donepezil |
49.44 ± 2.82 |
— |
— |
Curcumin |
— |
12.67 ± 0.49 (1.54) |
— |
Sitagliptin |
— |
— |
63.8 ± 3.15 |
Control |
— |
8.206 ± 0.33 (1) |
— |
3.4.2. Antiaging activity. Telomere damage is one of the primary causes of aging. It is noteworthy that many chronic diseases, such as cancer, heart diseases, diabetes, and kidney diseases, are related to ageing.7 In human cells, telomerase expression and activity decline with ageing.47 Gene therapy with hTERT or telomerase activators are the strategies commonly used for treatment of telomerase-dependent disease. However, gene therapy is not a practical medical intervention, so the attractive alternative would be a chemical telomerase activator, which would allow for a more precise control over the dose and timing.48 Previous studies on the root extract of Astragalus membranaceus led to an increase in the concentration of telomerase enzyme in the tested cells.49 Our results (Table 3) revealed that L. australis leaves were a potent extract as it caused a 1.49-fold increase in the telomerase activity, followed by L. australis fruit extract with a 1.41-fold increase in the telomerase activity. Meanwhile, curcumin as the standard caused a 1.54-fold increase in telomerase activity.
3.4.3. Antidiabetic activity. DPP-IV is a serine protease that cleaves N-terminal dipeptides from polypeptides. It regulates the bioactivity of many peptides, including the glucagon-like peptide-1 (GLP-1) and glucose-dependent insulinotropic polypeptide (GIP).50 DPP-IV inhibitors are crucial for the management of type II diabetes. This study reports for the first time the DPP-IV inhibition potential of L. australis leaves and fruits extracts as an antidiabetic, and compared it with the control drug sitagliptin. The results (Table 3) showed that the leaves extract demonstrated the most significant action with an IC50 value of 90.8 ± 4.48 ng mL−1 compared to the control IC50 = 63.8 ± 3.15 ng mL−1. Previous reports on the antidiabetic potential of L. chinensis was evaluated by measuring the α-glucosidase inhibitory effect and protein tyrosine phosphatase 1B (PTP1B) inhibitory effect, and the results showed that the butyl alcohol fraction was the most potent as an α-glucosidase and PTP1B inhibitor.21 The activity of the leaf extract may be attributed to the flavonoid content of the extract. Many flavonoids reported as DPP-IV inhibitors, such as quercetin, luteolin, apigenin, kaempferol, flavone, hesperetin, naringenin and genistin, in addition to glycosides as isoquercetin, kaempferol 7-O-α-L-rhamnoside, vitexin, rutin, isorhamnetin-3-O-glucoside and isorhamnetin-3-O-rutinoside, have also been reported as DPP-IV inhibitors.8,51,52
4. Conclusion
This research focused on the phytochemical characterization of the leaves and fruits of Livistona chinensis and L. australis, and the biological activities of L. australis leaves and fruits. The extracts were rich with phenolic compounds, especially flavonoids. Biological evaluation showed that the leaves extract displayed the best inhibitory effects against acetyl cholinesterase and DPP-IV, and good potentiation for telomerase activity. However, further experimental studies, such as in vivo animal studies and in silico study, could be planned depending on the present results.
Ethical statement
Ethics approval was not required for this research.
Author contributions
Collection, drying, and extraction of the plant: S. O. A., LC/MS analysis: U. R. A., compound isolation and identification: A. E., conceptualization, and methodology: S. S. E. and A. I. O., all authors contributed to the writing, reviewing, revising and editing of the manuscript.
Conflicts of interest
The authors declare that there is no conflict of interest.
References
- K. G. Yiannopoulou and S. G. Papageorgiou, J. Cent. Nerv. Syst. Dis., 2020, 12, 1179573520907397 CrossRef PubMed.
- A. N. Tamfu, S. Kucukaydin, B. Yeskaliyeva, M. Ozturk and R. M. Dinica, Molecules, 2021, 26, 5582 CrossRef CAS PubMed.
- E. H. Blackburn, C. W. Greider and J. W. Szostak, Nat. Med., 2006, 12, 1133–1138 CrossRef CAS PubMed.
- C. B. Harley, Curr. Mol. Med., 2005, 5, 205–211 CrossRef CAS PubMed.
- M. Lulkiewicz, J. Bajsert, P. Kopczynski, W. Barczak and B. Rubis, Mol. Biol. Rep., 2020, 47, 7181–7188 CrossRef CAS PubMed.
- Y. Aydin, in Molecular Basis and Emerging Strategies for Anti-aging Interventions, ed. S. I. Rizvi and U. Çakatay, Springer Singapore, Singapore, 2018, pp. 97–109, DOI: DOI:10.1007/978-981-13-1699-9_7.
- C. López-Otín, M. A. Blasco, L. Partridge, M. Serrano and G. Kroemer, Cell, 2013, 153, 1194–1217 CrossRef PubMed.
- F. Gao, Y. Fu, J. Yi, A. Gao, Y. Jia and S. Cai, J. Agric. Food Chem., 2020, 68, 12141–12151 CrossRef CAS PubMed.
- J. Kaur, R. Singla and V. Jaitak, Lett. Drug Des. Discovery, 2018, 15, 634–642 CrossRef CAS.
- T. Wu, C. K. Rayner and M. Horowitz, Metab. Control, 2015, 137–171 CAS.
- A. K. Langley, T. J. Suffoletta and H. R. Jennings, Pharmacotherapy, 2007, 27, 1163–1180 CrossRef CAS PubMed.
- P. Chen and J. Yang, Chin. Tradit. Herb Drugs, 2007, 8, 665–667 Search PubMed.
- T. Yuan, S.-P. Yang, H.-Y. Zhang, S.-G. Liao, W. Wang, Y. Wu, X.-C. Tang and J.-M. Yue, J. Asian Nat. Prod. Res., 2009, 11, 243–249 CrossRef CAS PubMed.
- X. Zeng, Q. Qiu, C. Jiang, Y. Jing, G. Qiu and X. He, Fitoterapia, 2011, 82, 609–614 CrossRef CAS PubMed.
- S. K. El-Desouky, M. E. Kassem, Z. I. A. Fifi and A. M. G. El-Deen, Nat. Prod. Commun., 2009, 4, 499–500 CrossRef CAS PubMed.
- M. E. Kassem, S. Shoela, M. M. Marzouk and A. A. Sleem, Nat. Prod. Res., 2012, 26, 1381–1387 CrossRef CAS PubMed.
- W. Lin, J. Zhao, Z. Cao, Q. Zhuang, L. Zheng, Q. Cai, D. Chen, L. Wang, Z. Hong and J. Peng, Oncol. Rep., 2013, 29, 1859–1866 CrossRef PubMed.
- S. Li, S. Luo, H. Chen, Y. Zheng, L. Lin, H. Yao and X. Lin, Drug Des., Dev. Ther., 2019, 13, 1555 CrossRef CAS PubMed.
- H. Ibrahim, F. Elshaarawy and E. Haggag, J. Adv. Pharm. Educ. Res., 2018, 2, 256–268 Search PubMed.
- S. S. El-Hawary, T. F. Ali, S. O. A. El-Ela, A. Elwekeel, U. R. Abdelmohsen and A. I. Owis, RSC Adv., 2022, 12, 19505–19511 RSC.
- Y. Wang, J. Zhai, D. Yang, N. Han, Z. Liu, Z. Liu, S. Li and J. Yin, Oxid. Med. Cell. Longevity, 2021, 2021, 7807046 Search PubMed.
- S. S. El-Hawary, A. I. Owis, S.
O. A. El-Ela and A. Elwekeel, Egypt. J. Chem., 2022, 65(5), 291–295 Search PubMed.
- A. I. Owis, M. S. El-Hawary, D. El Amir, O. M. Aly, U. R. Abdelmohsen and M. S. Kamel, RSC Adv., 2020, 10, 19570–19575 RSC.
- M. F. A. Bakar, M. Mohamed, A. Rahmat and J. Fry, Food Chem., 2009, 113, 479–483 CrossRef.
- S. Dhanasekaran, P. Perumal and M. Palayan, J. Appl. Pharm. Sci., 2015, 5, 12–016 CrossRef.
- M. S. Refaey, R. A. Abdelhamid, H. Elimam, Y. A. Elshaier, A. Ali and M. A. Orabi, Bioorg. Chem., 2021, 108, 104643 CrossRef CAS PubMed.
- P. K. Kempegowda, F. Zameer, C. K. Narasimashetty, S. P. Kollur and S. K. Murari, Afr. J. Tradit., Complementary Altern. Med., 2018, 15, 11–25 CrossRef CAS.
- R. Ahmed, E. Elkhrisy, W. A. H. EL-kashak, M. El Raey, M. Nassar and E.-S. A. Aboutabl, J. Adv. Pharm. Educ. Res., 2019, 3, 23–29 Search PubMed.
- X. Zeng, Y. Wang, Q. Qiu, C. Jiang, Y. Jing, G. Qiu and X. He, Fitoterapia, 2012, 83, 104–109 CrossRef CAS PubMed.
- M. Wu, C. Wang, C. Mai, J. Chen, X. Lai, L. He, S. Huang and X. Zhang, J. Funct. Foods, 2019, 61, 103460 CrossRef CAS.
- D. Olennikov, I. Zilfikarov and S. Khodakova, Chem. Nat. Compd., 2013, 49, 526–529 CrossRef CAS.
- F. S Elshaarawy, S. A Mina, N. M Gabr, S. M Abdelkhalik, R. Kamel, H. A Ibrahim and E. G. Haggag, J. Adv. Pharm. Educ. Res., 2018, 2, 201–211 Search PubMed.
- T. Mabry, K. R. Markham and M. B. Thomas, The systematic identification of flavonoids, Springer Science & Business Media, 2012 Search PubMed.
- P. Takolpuckdee, Thai J. Pharm. Sci., 2016, 40 Search PubMed.
- E. S. El-ghaly, Al-Azhar J. Pharm. Sci., 2014, 49, 259–268 CrossRef.
- Z. Xiao, H. Wu, T. Wu, H. Shi and B. Hang, Chem. Nat. Compd., 2006, 42, 736–737 CrossRef CAS.
- A. De Almeida, M. Miranda, I. Simoni, M. Wigg, M. Lagrota and S. Costa, Phytother. Res., 1998, 12, 562–567 CrossRef CAS.
- Y. A. Lim, M. C. Mei, I. T. Kusumoto, H. Miyashiro, M. Hattori, M. P. Gupta and M. J. P. R. Correa, Phytother. Res., 1997, 11, 22–27 CrossRef CAS.
- Y. Yu, H. Gao, Z. Tang, X. Song and L. J. Wu, Asian J. Tradit. Med., 2006, 1, 101–104 CAS.
- C. W. Haminiuk, G. M. Maciel, M. S. Plata-Oviedo and R. M. Peralta, Int. J. Food Sci. Technol., 2012, 47, 2023–2044 CrossRef CAS.
- V. Zarotsky, J. J. Sramek and N. R. Cutler, Am. J. Health-Syst. Pharm., 2003, 60, 446–452 CrossRef CAS PubMed.
- J. Sun, B. Wang, Y. Niu, Y. Tan, C. Fan, N. Zhang, J. Xue, J. Wei and J. Xiang, Entropy, 2020, 22, 239 CrossRef CAS PubMed.
- S. E. Arnold and A. Kumar, Med. Clin. North Am., 1993, 77, 215–230 CrossRef CAS PubMed.
- J. A. Schneider, Z. Arvanitakis, W. Bang and D. A. Bennett, Neurology, 2007, 69, 2197–2204 CrossRef PubMed.
- I. Bakoyiannis, A. Daskalopoulou, V. Pergialiotis and D. Perrea, Biomed. Pharmacother., 2019, 109, 1488–1497 CrossRef CAS PubMed.
- S. S. El-Hawwary, H. M. Abd Almaksoud, F. R. Saber, H. Elimam, A. M. Sayed, M. A. El Raey and U. R. Abdelmohsen, RSC Adv., 2021, 11, 18009–18025 RSC.
- Y.-S. Cong, W. E. Wright and J. W. Shay, Microbiol. Mol. Biol. Rev., 2002, 66, 407–425 CrossRef CAS PubMed.
- B. Molgora, R. Bateman, G. Sweeney, D. Finger, T. Dimler, R. B. Effros and H. F. Valenzuela, Cells, 2013, 2, 57–66 CrossRef CAS PubMed.
- C. B. Harley, W. Liu, M. Blasco, E. Vera, W. H. Andrews, L. A. Briggs and J. M. Raffaele, Rejuvenation Res., 2011, 14, 45–56 CrossRef CAS PubMed.
- K. Fujiwara, T. Inoue, N. Yorifuji, M. Iguchi, T. Sakanaka, K. Narabayashi, K. Kakimoto, S. Nouda, T. Okada and K. Ishida, J. Clin. Biochem. Nutr., 2015, 56, 155–162 CrossRef CAS PubMed.
- S. Shaikh, E.-J. Lee, K. Ahmad, S.-S. Ahmad, J.-H. Lim and I. Choi, Pharmaceuticals, 2021, 14, 591 CrossRef CAS PubMed.
- B. T. Zhao, D. D. Le, P. H. Nguyen, M. Y. Ali, J.-S. Choi, B. S. Min, H. M. Shin, H. I. Rhee and M. H. Woo, Chem.-Biol. Interact., 2016, 253, 27–37 CrossRef CAS PubMed.
|
This journal is © The Royal Society of Chemistry 2023 |
Click here to see how this site uses Cookies. View our privacy policy here.