DOI:
10.1039/D3RA00802A
(Paper)
RSC Adv., 2023,
13, 15379-15390
Lonicera japonica Thunb. as a promising antibacterial agent for Bacillus cereus ATCC14579 based on network pharmacology, metabolomics, and in vitro experiments†
Received
6th February 2023
, Accepted 15th May 2023
First published on 22nd May 2023
Abstract
Lonicera japonica Thunb. has attracted much attention for its treatment of bacterial and viral infectious diseases, while its active ingredients and potential mechanisms of action have not been fully elucidated. Here, we combined metabolomics, and network pharmacology to explore the molecular mechanism of Bacillus cereus ATCC14579 inhibition by Lonicera japonica Thunb. In vitro inhibition experiments showed that the Lonicera japonica Thunb.'s water extracts, ethanolic extract, luteolin, quercetin, and kaempferol strongly inhibited Bacillus cereus ATCC14579. In contrast, chlorogenic acid and macranthoidin B had no inhibitory effect on Bacillus cereus ATCC14579. Meanwhile, the minimum inhibitory concentrations of luteolin, quercetin, and kaempferol against Bacillus cereus ATCC14579 were 15.625 μg mL−1, 31.25 μg mL−1, and 15.625 μg mL−1. Based on the previous experimental basis, the metabolomic analysis showed the presence of 16 active ingredients in Lonicera japonica Thunb.'s water extracts and ethanol extracts, with differences in the luteolin, quercetin, and kaempferol contents between the water extracts and ethanol extracts. Network pharmacology studies indicated that fabZ, tig, glmU, secA, deoD, nagB, pgi, rpmB, recA, and upp were potential key targets. Active ingredients of Lonicera japonica Thunb. may exert their inhibitory effects by inhibiting ribosome assembly, the peptidoglycan biosynthesis process, and the phospholipid biosynthesis process of Bacillus cereus ATCC14579. An alkaline phosphatase activity assay, peptidoglycan concentration assay, and protein concentration assay showed that luteolin, quercetin, and kaempferol disrupted the Bacillus cereus ATCC14579 cell wall and cell membrane integrity. Transmission electron microscopy results showed significant changes in the morphology and ultrastructure of the cell wall and cell membrane of Bacillus cereus ATCC14579, further confirming the disruption of the cell wall and cell membrane integrity of Bacillus cereus ATCC14579 by luteolin, quercetin, and kaempferol. In conclusion, Lonicera japonica Thunb. can be used as a potential antibacterial agent for Bacillus cereus ATCC14579, which may exert its antibacterial activity by destroying the integrity of the cell wall and membrane.
Introduction
Bacillus cereus, a widespread anaerobic or facultative anaerobic Gram-positive bacterium, can produce diarrhea-type enterotoxins and vomiting-type enterotoxins, causing diarrhea and vomiting symptoms. In particular, the pathogenic bacteria produce hemolysin, which causes extreme dehydration and even death in humans.1–3 Therefore, preventing and reducing acute and chronic hazards of Bacillus cereus in humans is essential. The emergence of antibiotics has greatly solved this problem and is considered the most effective treatment for human Bacillus cereus infections.4 However, resistant strains of Bacillus cereus have been found with the evolution of bacteria and the misuse of antibiotics.5 To mitigate the lack of novel antimicrobial agents and the increase of antibiotic resistance, herbal medicines, widely available in nature, may be a potential solution. For antibiotic-resistant bacteria, herbs, herbal extracts, and their active ingredients have been found to have multiple antimicrobial effects and effective therapeutic efficacy, such as Eucommia ulmoides Oliver, Wolfiporia cocos (F.A. Wolf) Ryvarden & Gilb, Reynoutria japonica Houtt., Mimosa pudica Linn., etc., which has led to an increasing interest in herbal medicine.6–10
Lonicera japonica Thunb. is a traditional Chinese herb that plays an important role in treating some infectious diseases such as exopathogenic wind-heat, sores, and carbuncles.11 Modern pharmacology shows that Lonicera japonica Thunb. has various pharmacological activities, such as anti-inflammatory, antibacterial, antiviral, antitumor, etc.12–15 At the same time, Lonicera japonica Thunb. contains a variety of active ingredients, mainly including phenolic acids, flavonoids, terpenoids, etc.16
In China, Lonicera japonica Thunb. has long been used to treat diseases caused by bacterial and viral infections, such as respiratory infections, pneumonia, etc.17,18 Existing studies have shown that Lonicera japonica Thunb. water extractions could inhibit Escherichia coli, Shigellosis, Bordetella pertussis, Sarcina lutea, Bacillus subtitles, Mycobacterium tuberculosis, Staphylococcus, Pseudomonas aeruginosa, Streptococcus, Diplococcus pneumoniae, etc19. Meanwhile, the bacteriostatic ability of Lonicera japonica Thunb. was highly positively correlated with the content of phenolic acid.20 However, the inhibitory effect of Lonicera japonica Thunb. and its active ingredients in Bacillus cereus are still little studied. Besides, the molecular mechanism of inhibition of Bacillus cereus in vitro by Lonicera japonica Thunb. and its active ingredients need to be further understood. In recent years, network pharmacology has become a new model and method for pharmacological research and is widely used in practice.21 The holistic analysis of network pharmacology helps to evaluate the network relationships formed among different components, targets, and pathways of herbal medicines. This, in turn, provides new insights into the complex basic research of herbal medicine. Thus, the potential and molecular mechanism of the direct action of Lonicera japonica Thunb. on Bacillus cereus can be easily assessed by employing network pharmacology. Considering the complexity of the active components of Lonicera japonica Thunb., a metabolomic method was used to analyze its active ingredients. Meanwhile, in vitro experiments, electron microscopy observation, and marker detection were conducted to verify the antibacterial effect of Lonicera japonica Thunb. and related molecular mechanisms. The workflow of the study is shown in Fig. 1.
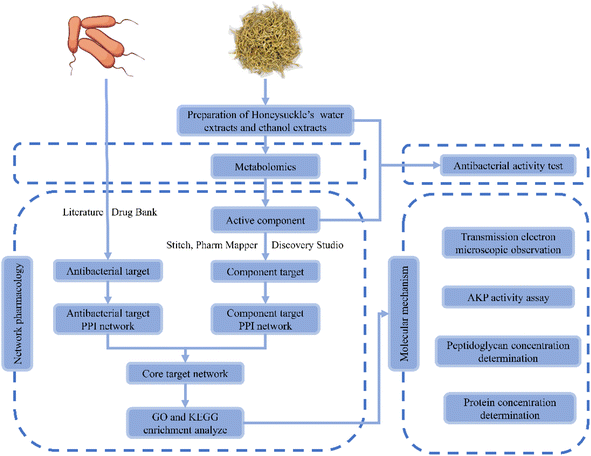 |
| Fig. 1 The flowchart of the study approach. | |
Materials and methods
Chemicals and materials
The Lonicera japonica Thunb. was obtained from the original production area of Linyi, Shandong Province, China, and the botanical identification was carried out by Associate Professor Guoping Peng (Hunan Agricultural University, Changsha, China). The samples were stored in the storage room for Hunan Engineering Laboratory for Good Agricultural Practice and Comprehensive Utilization of Famous-region Medicinal Plants until further processing.
Bacillus cereus ATCC14579 was obtained from ATCC (Rockville, MD, United States). AKP activity assay kits were purchased from Suzhou Grace Biotechnology Co, Ltd (Suzhou, China). Bradford protein assay kits were purchased from Tian Biotech (Beijing) CO, Ltd (Beijing, China). Bacteria peptidoglycan (PG) ELISA kits were purchased from Shanghai Enzyme-linked Biotechnology Co., Ltd (Shanghai, China). Ampicillin was procured from Changsha Dingguo Biotechnology CO, Ltd (Changsha, China). Chlorogenic acid was procured from Beijing Solarbio Science & Technology Co, Ltd (Beijing, China) with a purity greater than 98%. Luteolin, quercetin, and kaempferol were procured from Chengdu Aifa Biotechnology Co, Ltd (Chengdu, China), and their purities were greater than 98%. Macranthoidin B was procured from Chengdu Maidesheng Technology Co, Ltd (Chengdu, China). Agar was purchased from Beijing Labgic Technology Co, Ltd (Beijing, China). Tryptone and yeast extract were purchased from Thermo Fisher Scientific (Massachusetts, United States). Sodium chloride (AR) was procured from Sinopharm Chemical Reagent Co, Ltd (Beijing, China). Methanol and acetonitrile (HPLC-grade) were procured from Merck & Co Inc(New Jersey, United States). Formic acid was procured from Shanghai Aladdin Biochemical Technology Co, Ltd (Shanghai, China).
Preparation of water extracts, ethanol extracts, and solution of active ingredients of Lonicera japonica Thunb.
Preparation of water extracts and ethanol extracts. The 100 g of dried Lonicera japonica Thunb. powder was soaked in water or 50% ethanol (w/v = 1
:
10) for 24 h and filtered. The residue was added to an equal amount of water or 50% ethanol, steeped at 60 °C for 3 h, and filtered again. The resultant filtrate was collected and concentrated to prepare the final concentration equivalent to 1 g raw herb per mL. Finally, the concentrated liquid filtered through a 0.22 μm filter membrane was stored in a refrigerator at 4 °C.
Preparation of solution of active ingredients. Luteolin, quercetin, kaempferol, chlorogenic acid, and macranthoidin B were dissolved in DMSO to prepare a mother liquor with a final concentration of 2 mg mL−1. Solutions were stored at 4 °C in a 0.22 μm filter membrane.
Antibacterial activity test of water extract, ethanol extract, and active ingredients of Lonicera japonica Thunb.
The double-layer plate punch method was carried out for antibacterial activity on the agar plates. Pour 15 mL of LB solid medium into the lower layer of the Petri dish. After it solidified, Bacillus cereus ATCC14579 bacterial suspension at a concentration of 1 × 106 CFU mL−1 was inserted into LB solid medium at 50 °C in a 1% inoculum. Subsequently, 15 mL of LB solid medium containing the bacterial suspension was poured on the upper layer of the Petri dish, left to solidify, and punched. Experimental group: 200 μL of water extracts, ethanol extracts of Lonicera japonica Thunb., and solutions of active ingredients (working concentration, 1 mg mL−1) were added to the wells. Negative control group: 200 μL DMSO was added to the wells. The positive control group added 20 μL of the antibacterial drug (ampicillin: AMP, 100 mg mL−1) to the wells. Meanwhile, a blank control was established. Antimicrobial activity was measured as the diameter of the inhibition zone around the wells after the incubation period of 24 h at 37 °C. The results were compared with negative and positive controls.
Determination of minimum inhibitory concentration (MIC) of active ingredients
The MIC values of the solution of active ingredients (working concentration, 1 mg mL−1) against Bacillus cereus ATCC14579 were determined using the micro-broth dilution method according to Clinical Laboratory and Standards (CLSI). Briefly, the bacterial suspensions cultured to log phase were diluted to 1 × 106 CFU mL−1. 100 μL of the solution of active ingredients was serially diluted twofold with LB liquid medium to a series of concentrations in 96-well plates (1000, 500, 250, 125, 62.5, 31.25, 15.625, 7.8125 μg mL−1). Subsequently, the diluted bacterial suspension was added to each well in the same volume until the final volume was 200 μL. 96-well plates were incubated at 37 °C for 12 h. After incubation, the 96-well plates were removed, and each well's absorbance value at 600 nm was measured in a microplate reader (BioTek, United States). The concentration corresponding to the wells with no sudden change in absorbance value is the MIC value.
Chromatographic analysis and ESI-Q-TRAP-MS/MS identification of active ingredients in water extract and ethanol extract of Lonicera japonica Thunb.
The Lonicera japonica Thunb.'s water extracts and alcohol extracts were centrifuged at 4 °C and 1.2 × 104 rpm for 10 min, and the supernatant was diluted 50 times with 50% methanol. The solution was filtered through a 0.22 μm filter for the UPLC-ESI-Q-TRAP-MS/MS analysis.
UPLC conditions. Analyses were conducted on the ExionLC™ AD system (SCIEX, United States) with an Agilent SB-C18 column (2.1 mm × 100 mm, 1.8 μm), and the column temperature was maintained at 40 °C. The mobile phase consisted of 0.1% formic acid (A) and acetonitrile with 0.1% formic acid (B). Elution gradient: 0–9.0 min, 5–95% B; 9.0–10.0 min, 95–95% B; 10.0–11.1 min, 95–5% B; 11.1–14.0 min, 5–5%. The flow velocity was set as 0.35 mL per minute; The column temperature oven was set to 40 °C; the injection volume was 4 μL. The effluent was alternatively connected to an ESI-Q-TRAP-MS.
MS conditions. Analyses were conducted on an Applied Biosystems 4500 Q TRAP (SCIEX, United States). The MS parameters were as follows: source temperature 550 °C; ion spray voltage (IS) 5500 V (positive ion mode)/-4500 V (negative ion mode); ion source gas I (GSI), gas II (GSII), curtain gas (CUR) was set at 50, 60, and 25 psi, respectively; the collision-activated dissociation (CAD) was high. QQQ scans were acquired as MRM experiments with collision gas (nitrogen) set to medium. DP (declustering potential) and CE (collision energy) for individual MRM transitions were done with further DP and CE optimization. A specific set of MRM transitions were monitored for each period according to the metabolites eluted within this period.
Active ingredients in Lonicera japonica Thunb.
The main components related to Lonicera japonica Thunb. were searched in the Traditional Chinese Medicine Systematic Pharmacology Database and Analysis Platform (TCMSP, https://www.tcmspw.com/tcmsp.php), absorbability (OB) and drug-likeness (DL) were used as selection indexes to screen the chemical components with OB ≥ 30% and DL ≥ 0.18 as the main active ingredients of Lonicera japonica Thunb. In addition, metabolomic data and a literature review were conducted to supplement the main active ingredients.11 The chemical structure information of the above active ingredients was obtained from PubChem (https://pubchem.ncbi.nlm.nih.gov/) database.
Targets related to active ingredients
The active ingredient structure information obtained from PubChem was used to search for targets that interacted with the active ingredient of Lonicera japonica Thunb. on Stitch (principle: ligand structure similarity), Pharm Mapper (principle: pharmacophore matching), and Discovery Studio (principle: pharmacophore matching), databases. Upload the ingredient name in Stitch (http://stitch.embl.de/) database, select the related bacterial species, and set the minimum interaction score to 0.7 to download the targets that meet the parameter requirements. In Pharm Mapper (http://www.lilab-ecust.cn/pharmmapper/) database, upload the information of the corresponding component in SDF format, select the background library “Druggable Pharmacophore Models (v2017, 16159)” and filter proteins with score ≥ 0.7. Upload the component SDF files in Discovery Studio 2019 software and use “Ligand Profiler” to reverse screen the Discovery Studio pharmacophore database to select proteins with a score ≥ 0.5. The above proteins were transformed into the target of Bacillus cereus ATCC14579 by UniProt (https://www.uniprot.org/) database. For the convenience of description, these targets are referred to as “component targets”.
Known targets for inhibiting Bacillus cereus ATCC14579
The drug bank database was used to collect the targets of antibiotics acting on bacteria, and the relevant literature was reviewed for a supplement.22–25 The collected targets were transformed into the targets of Bacillus cereus strain ATCC14579 by the UniProt database. Likewise, these targets are referred to as “antibacterial targets” for ease of description.
Construction and analysis of the interaction network
The “component targets” and “antibacterial targets” were uploaded to the String database, and the corresponding species were selected. Set the minimum interaction score to 0.9 after submission and download the interaction network file in TSV format. Then, two protein–protein interactions (PPI) network files were imported into Cytoscape 3.7.2 software, and “intersection” was selected to intersect the two PPI networks. The intersection results were considered as core targets of antibacterial of Lonicera japonica Thunb. The common targets of “component targets” and “antibacterial targets” in the intersection results are called central targets.
GO and KEGG pathway enrichment analysis
To deeply explore the pathways and biological processes in the intersection network, GO (Gene Ontology) enrichment and KEGG (Kyoto Encyclopedia of Genes and Genomes) pathway analysis were performed with GlueGo 2.5.8 software. Among the GO enrichment analyses include cellular component (CC), biological process (BP), and molecular function (MF). The core targets were uploaded in GlueGo 2.5.8, and the related species were selected. The parameters were set as P < 0.05, “GO Trem Fusion,” “GO tree interval,” with a minimum value of 4, and other parameters have defaulted.
Transmission electron microscopy experiment
Transmission electron microscopy (TEM) was used to observe the morphological changes of Bacillus cereus ATCC14579 after treatment with luteolin, quercetin, and kaempferol. In the experimental group, the MIC doses of luteolin, quercetin, and kaempferol were added and diluted in the bacterial suspension to a final concentration of 1 × 106 CFU mL−1. The control group was the same concentration of bacterial suspension without the drug. The groups were cultured at 37 °C with shaking at 200 rpm for 8 h until the logarithmic growth period. The experimental and control groups were centrifuged at 4000 rpm min−1 for 10 min, and the bacteria were collected. The supernatant was discarded and fixed with TEM fixative for 2 h at room temperature and protected from light and then sent to the electron microscope laboratory of Wuhan Servicebio Technology CO., LTD.
Effect of active ingredients of Lonicera japonica Thunb. On bacterial cell wall
Alkaline phosphatase (AKP) activity and peptidoglycan content were determined to evaluate the change in Bacillus cereus ATCC14579 cell wall integrity. Different concentrations (0, MIC, 1/2 MIC) of luteolin, quercetin, and kaempferol were added and diluted in the bacterial suspension to a final concentration of 1 × 106 CFU mL−1. The groups were cultured at 37 °C with shaking at 200 rpm for 8 h until the logarithmic growth period. Subsequently, the groups were centrifuged at 4000 rpm for 10 min. The supernatant of each group was treated according to the method in the AKP kit and PG kit, and detected by microplate reader.
Effect of active ingredients of Lonicera japonica Thunb. On bacterial cell membrane
The integrity of the cell membrane of Bacillus cereus ATCC14579 was evaluated by determining the release of intracellular proteins. Similarly, the supernatant of each group after centrifugation was treated and measured according to the method of the Bradford protein assay kit.
Statistical analysis
All experiments were repeated three times. The results were expressed as the mean ± SD. Statistical analysis was carried out using one-way of variance (ANOVA) and Duncan's multiple range tests on SPSS Statistics 25. Statistical significance was defined as *P < 0.05, **P < 0.01, and ***P < 0.001.
Results
Antibacterial activity of water extract, ethanol extract, and active ingredients
Lonicera japonica Thunb.'s water extracts and ethanol extracts were tested for their ability to inhibit Bacillus cereus ATCC14579. Position 1 of the agar plate is a positive control, position 2 is the negative control, position 3 is the blank control, and positions 4, 5, and 6 are the drug administration groups. As shown in Fig. 2, Lonicera japonica Thunb.'s water extracts and ethanol extracts showed a significant inhibitory effect on Bacillus cereus ATCC14579 compared to the negative control. In contrast, the ethanol extracts of Lonicera japonica Thunb. were stronger than the water extracts in terms of bacterial inhibitory ability. Five active components of Lonicera japonica Thunb., luteolin, quercetin, kaempferol, chlorogenic acid, and gray felt macranthoidin B were selected to verify the network pharmacology results for the inhibition experiments in this experiment. As shown in Fig. 3, the results showed that luteolin, quercetin, and kaempferol significantly inhibited Bacillus cereus ATCC14579. In contrast, chlorogenic acid and macranthoidin B had no significant inhibitory ability on Bacillus cereus ATCC14579. Meanwhile, the minimum inhibitory concentrations (MIC) of luteolin, quercetin, and kaempferol against Bacillus cereus ATCC14579 were 31.25 μg mL−1, 7.8125 μg mL−1, and 15.625 μg mL−1, respectively. While chlorogenic acid, macranthoidin B against Bacillus cereus ATCC14579, had a minimum inhibitory concentration (MIC) of more than 1000 μg mL−1 (ESI Table 1†).
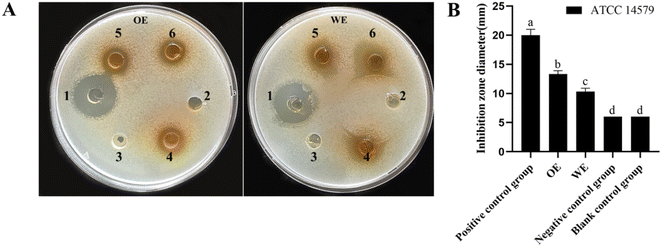 |
| Fig. 2 Antibacterial activity test. Bacterial inhibition experiments on water extracts and ethanol extracts of Lonicera japonica Thunb. (A) Visualized inhibition zone, (B) histogram of inhibition zone diameter. The average and standard deviation of the data is taken three times a repetition. Different letters indicate significant differences. P < 0.05. | |
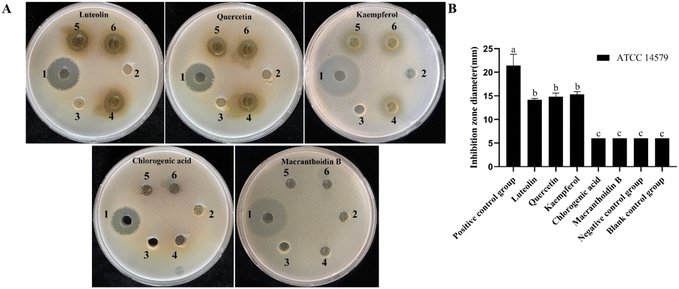 |
| Fig. 3 Antibacterial activity test. Bacterial inhibition experiments of 5 active ingredients. (A) Visualized inhibition zone, (B) histogram of inhibition zone diameter. The average and standard deviation of the data is taken three times a repetition. Different letters indicate significant differences. P < 0.05. | |
Analysis of metabolic profile and main active ingredients
By constructing a PCA diagram under the action positive and negative ions (ESI Fig. 1†), it was found that the separation of QC samples, water extracts (WE), and ethanol extracts (OE) of Lonicera japonica Thunb. were well separated, indicating that samples of the water extracts and ethanol extracts were reproducible, and the results were reliable, which could reflect the differences among the three groups of samples on the whole. Based on previous research, after UPLC-ESI-Q-TRAP-MS/MS analysis, 16 active ingredients were identified from the Lonicera japonica Thunb.'s water extracts and ethanol extracts, including substances such as luteolin, kaempferol, quercetin, chlorogenic acid, and macranthoidin A. Meanwhile, the relative contents of 16 major active ingredients in WE and OE were compared, and it was found that Lonicera japonica Thunb.’s active ingredients, luteolin, kaempferol, and quercetin, were significantly higher in the ethanol extracts than in the water extracts. While chlorogenic acid showed no significant difference between the two (Table 1).
Table 1 Active ingredients of Lonicera japonica Thunb.
No. |
Rt (min) |
M/S |
M ± H |
Chemical formula |
Common name |
Sources and trends |
P value |
Fold change |
1 |
1.13 |
192.0634 |
M − H |
C7H12O6 |
Quinic acid |
ns |
— |
— |
2 |
2.3 |
354.0951 |
M − H |
C16H18O9 |
Chlorogenic acid |
ns |
— |
— |
3 |
2.31 |
354.0951 |
M − H |
C16H18O9 |
Neochlorogenic acid |
OE↑* |
0.010001 |
1.1267 |
4 |
2.9 |
354.0951 |
M − H |
C16H18O9 |
Cryptochlorogenic acid |
WE↑* |
0.0325 |
1.7816 |
5 |
3.3 |
390.1526 |
M + H |
C17H26O10 |
Loganin |
OE↑*** |
0.0032 |
177.4061 |
6 |
3.4 |
180.0423 |
M − H |
C9H8O4 |
Caffeic acid |
WE↑*** |
0.000009 |
3.5372 |
7 |
3.52 |
388.1369 |
M + H |
C10H24O10 |
7-epi-Vogeloside |
ns |
— |
— |
8 |
3.8 |
448.1006 |
M + H |
C21H20O11 |
Luteoloside |
OE↑*** |
0.000010 |
33.9686 |
9 |
3.9 |
516.1268 |
M − H |
C25H24O12 |
Isochlorogenic acid A |
OE↑*** |
0.000072 |
1.2741 |
10 |
4.2 |
516.1268 |
M − H |
C25H24O12 |
Isochlorogenic acid B |
OE↑** |
0.0012 |
1.2378 |
11 |
4.2 |
516.1268 |
M + H |
C25H24O12 |
Isochlorogenic acid C |
OE↑** |
0.0041 |
1.3615 |
12 |
4.7 |
194.0579 |
M − H |
C10H10O4 |
Methyl caffeate |
WE↑* |
0.0330 |
1.1980 |
13 |
5 |
286.0477 |
M − H |
C15H10O6 (https://pubchem.ncbi.nlm.nih.gov/#query=C15H10O6) |
Luteolin |
OE↑*** |
0.000005 |
3.6765 |
14 |
5.1 |
302.0427 |
M + H |
C15H10O7 |
Quercetin |
OE↑** |
0.0061 |
3.5214 |
15 |
5.79 |
286.0477 |
M + H |
C15H10O6 |
Kaempferol |
OE↑*** |
0.0007 |
2.0837 |
16 |
7.75 |
414.3862 |
M + H |
C29H50O |
β-sitosterol |
ns |
— |
— |
Targets of active ingredients and inhibiting Bacillus cereus ATCC14579
After screening the TCMSP database, metabolomic data, and literature supplementation, 28 active ingredients of Lonicera japonica Thunb. were used. were obtained (ESI Table 2†). 25 active ingredients were predicted by the databases mentioned above (Stitch, Pharm Mapper, Discovery Studio). After removing duplicate targets, 92 targets were obtained from the 25 identified active ingredients (Fig. 4). After screening drug bank data and literature supplementation, 64 antimicrobial targets related to Bacillus cereus ATCC14579 were obtained.
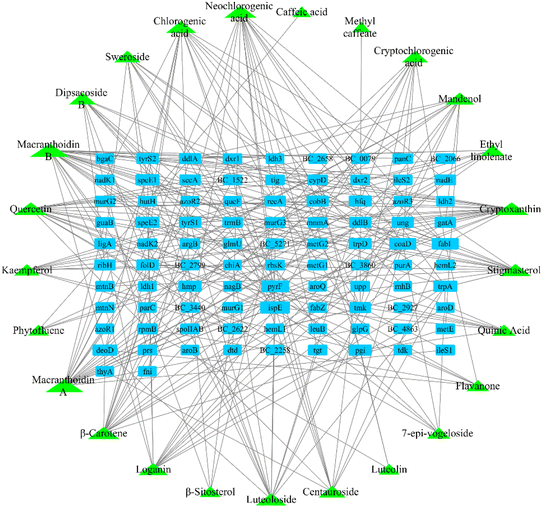 |
| Fig. 4 Component-target network. | |
PPI network analysis and core targets
Protein–protein interaction network analysis of antibacterial and component targets was performed using the String database. The direct targets of active ingredients of Lonicera japonica Thunb. were identified as 84 in the database and 200 indirect targets after PPI. A total of 284 targets and 1737 edges were obtained (ESI Fig. 2†). The direct targets of Bacillus cereus ATCC14579 associated with antibacterial were all identified, and 200 indirect targets after PPI, a total of 264 targets and 2788 edges (ESI Fig. 3†). 125 targets were obtained after taking the intersection of the two PPI networks (Fig. 5), which are the core targets. The yellow nodes are component targets, the orange nodes are bacterial targets, and the red nodes are targets common to both component and bacterial targets. The node degree values are proportional to their size.
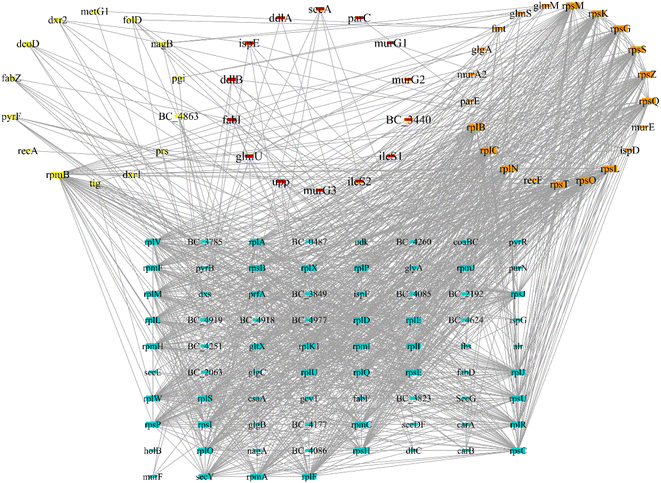 |
| Fig. 5 Core target network. The orange nodes are targets of the antimicrobial target database, the yellow nodes are targets of the component target database, the red nodes are targets common to both the antimicrobial target database and the component target database, and the blue nodes are indirect targets after PPI. Node connectivity is proportional to its size. | |
Biological function and pathway analysis
To explore the potential mechanism of Lonicera japonica Thunb. inhibition in depth, GO enrichment and KEGG pathway analysis of core targets was performed by GlueGo. 65 out of 125 targets were involved in enriching information of entries that met the parameter requirements. Among them, 29 biological processes were enriched, including the ribosome assembly process, peptidoglycan biosynthesis, phospholipid biosynthesis process, ribonucleotide biosynthesis process, etc. Eight entries on cellular components were enriched, involving ribosomal subunits, cytoplasm, etc. Meanwhile, five entries were enriched for molecular functions involving RNA binding, protein transmembrane transporter activity, etc. (Fig. 6A). Two KEGG signaling pathways were enriched, involving folate one-carbon units and ribosomes (Fig. 6B).
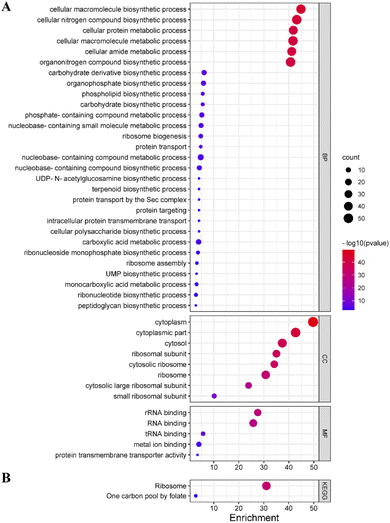 |
| Fig. 6 GO and KEGG enrichment analysis (A) GO enrichment analysis, (B) KEGG enrichment analyze. | |
TEM observation
To further investigate the mechanism of bacterial inhibition of luteolin, quercetin, and kaempferol, which have significant antibacterial effects. Transmission electron microscopy (TEM) was used to observe the structural changes of Bacillus cereus ATCC14579 after treatment with luteolin, quercetin, and kaempferol. As shown in Fig. 7, the cell wall of Bacillus cereus ATCC14579 in the control group (Fig. 7A) was intact, the cell membrane was continuous and smooth, the cytoplasmic electron density was homogeneous, and the cell morphology was structurally normal. In contrast, in the experimental group, Bacillus cereus ATCC14579 treated with luteolin, quercetin, and kaempferol (Fig. 7B–D) showed obvious cell morphological damage, cell wall and cell membrane rupture, uneven cytoplasmic electron density, and overflow of cell contents.
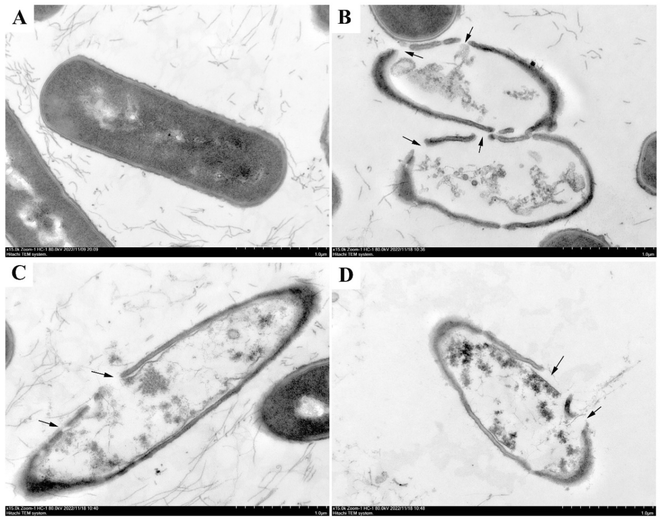 |
| Fig. 7 Transmission electron micrographs showing the effects of luteolin, quercetin, and kaempferol on Bacillus cereus ATCC14579 cell morphology. (A) Control group, (B) cells treated with MIC of luteolin, (C) cells treated with MIC of quercetin, (D) cells treated with MIC of kaempferol. | |
The integrity of the cell wall and cell membrane
The cell wall and cell membrane of Bacillus cereus ATCC14579 were examined for damage by measuring the AKP enzyme activity concentration, peptidoglycan concentration, and protein concentration of the bacterial suspension. Without drug treatment, the extracellular AKP enzyme activity concentration was 0.0021 μmol min−1 mL−1. After MIC treatment with luteolin, quercetin, and kaempferol, the extracellular AKP enzyme activity concentration increased significantly, reaching 0.0053, 0.0076, and 0.0048 μmol min−1 mL−1. Under 1/2 MIC treatment, the extracellular AKP enzyme activity concentration significantly decreased (Fig. 8A). Meanwhile, the extracellular peptidoglycan concentration without drug treatment was 0.10 μg mL−1, and the extracellular peptidoglycan concentration was significantly increased after luteolin, quercetin, and kaempferol MIC treatment, reaching 0.49, 0.53, and 0.58 μg mL−1, respectively, while the extracellular peptidoglycan concentrations were significantly decreased after 1/2 MIC treatment (Fig. 8B). In addition, the extracellular protein concentration without drug treatment was 0.021 mg mL−1. The extracellular protein concentration was significantly increased after MIC treatment with luteolin, quercetin, and kaempferol, reaching 0.042, 0.050, and 0.097 mg mL−1. There was a significant decrease in extracellular protein concentration under 1/2 MIC treatment (Fig. 8C).
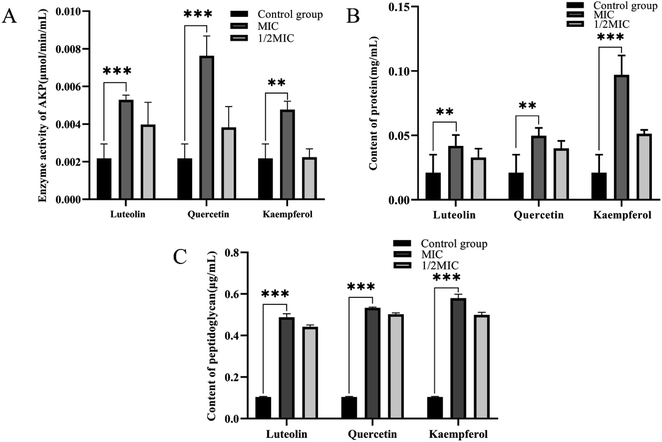 |
| Fig. 8 Bacillus cereus ATCC14579 cell integrity assay. (A) AKP activity of Bacillus cereus ATCC14579 after treatment with luteolin, quercetin, and kaempferol. (B) Peptidoglycan concentration of Bacillus cereus ATCC14579 after treatment with luteolin, quercetin, and kaempferol. (C) Protein concentration of Bacillus cereus ATCC14579 after treatment with luteolin, quercetin, and kaempferol. | |
Discussion
It has been used in clinical practice as a traditional medicine to treat some infections. Gastrointestinal disorders caused by Bacillus cereus infections occur, and their bacterial toxins cause vomiting and diarrhea, or even death in infected individuals.26 The results showed that Lonicera japonica Thunb.'s water extracts and ethanol extracts had good antibacterial effects on Bacillus cereus ATCC14579. Its main active ingredients, luteolin, quercetin, and kaempferol, also had good antibacterial effects on Bacillus cereus ATCC14579 (antibacterial activity assay, transmission electron microscopy observation, AKP enzyme activity, peptidoglycan concentration assay, and extracellular protein concentration assay), revealing the potential therapeutic function of Lonicera japonica Thunb. to disrupt the cell wall and cell membrane structure of Bacillus cereus ATCC14579. Based on network pharmacology and molecular docking, fabZ, tig, glmU, etc., were identified as possible core targets. The enrichment results showed that the core targets are involved in ribosome assembly, phospholipid biosynthesis process, peptidoglycan biosynthesis process, etc. The phospholipid biosynthesis process and peptidoglycan biosynthesis process were selected for experimental validation. The peptidoglycan biosynthesis process is an important pathway for bacterial peptidoglycan synthesis. Peptidoglycan is an important component in maintaining the structural integrity of the cell wall, which is required for the survival of bacteria. Targets related to peptidoglycan synthesis can be used as targets for bacteriostatic agents.27 Our study showed that the action of the active ingredient of Lonicera japonica Thunb. on Bacillus cereus ATCC14579 resulted in a significant increase in the extracellular AKP enzyme activity concentration, reflecting the breakage of the cell wall, suggesting that Lonicera japonica Thunb. may exert its action by inhibiting the cell wall Bacillus cereus ATCC14579 synthesis pathway and targets. Furthermore, it was found that the concentration of extracellular peptidoglycan increased significantly after Bacillus cereus ATCC14579 was treated with active ingredients luteolin, quercetin, and kaempferol. These results further confirmed the prediction of network pharmacology, suggesting that active ingredients luteolin, quercetin, and kaempferol affect the biosynthesis of peptidoglycan, hinder the cross-linking of peptidoglycan monomers, and cause the accumulation of large amounts of peptidoglycan monomers in bacteria. The phospholipid biosynthesis process is an important pathway for bacterial phospholipid synthesis, an important component of the cell membrane. The survival of bacteria depends on lipid membrane homeostasis.28 After treatment of Bacillus cereus ATCC14579 with the active ingredients of Lonicera japonica Thunb., the extracellular protein concentration was significantly increased, reflecting the infiltration of intracellular proteins and cell membrane rupture, suggesting that Lonicera japonica Thunb. may exert its better antibacterial effect by blocking the phospholipid biosynthesis of Bacillus cereus ATCC14579.
Antibacterial experiments were conducted to evaluate the antibacterial properties of Lonicera japonica Thunb. and its active ingredients. The inhibition assay revealed that Lonicera japonica Thunb.'s water extracts and ethanol extracts inhibited Bacillus cereus ATCC14579. However, the antibacterial activity of the ethanol extracts was stronger than that of the water extracts, and there was a significant difference between the two in terms of antibacterial effect. Meantime, the active ingredients luteolin (MIC = 15.625 μg mL−1), quercetin (MIC = 7.8125 μg m−1), and kaempferol (MIC = 15.625 μg mL−1) also inhibited Bacillus cereus ATCC14579 with good in inhibitory effect. Interestingly, chlorogenic acid, a major constituent of Lonicera japonica Thunb., had no inhibitory effect on Bacillus cereus ATCC14579. In a previous study, the minimum inhibitory concentration of chlorogenic acid against Escherichia coli, Staphylococcus aureus, Enterococcus faecium, and Proteus vulgaris, the minimum inhibitory concentration of chlorogenic acid against Bacillus cereus ATCC14579 in this study. Meanwhile, the present study showed that Macranthoidin B had no inhibitory effect on Bacillus cereus ATCC14579, the first report of Macranthoidin B regarding its bacterial inhibitory ability.
We detected 16 important active ingredients in water extracts and ethanol extracts of Lonicera japonica Thunb. by UPLC-ESI-Q TRAP-MS/MS: β-sitosterol, luteolin, kaempferol, quercetin, luteoloside, chlorogenic acid, neochlorogenic acid, cryptochlorogenic acid, 7-epi-vogeloside, quinic acid, eriodictyol, caffeic acid, methyl caffeate, isochlorogenic A, Isochlorogenic acid B, isochlorogenic acid C, loganin. The relative contents of 16 compounds in the water extracts and ethanol extracts of Lonicera japonica Thunb. were compared, and it was found that the relative contents of luteolin, kaempferol, and quercetin in the ethanol extracts of Lonicera japonica Thunb. were higher than those in the water extracts of Lonicera japonica Thunb. The relative content of quercetin was much higher than that of the water extracts of Lonicera japonica Thunb., suggesting the difference in the antibacterial ability of the ethanol extracts of Lonicera japonica Thunb. and the water extracts of Lonicera japonica Thunb. maybe due to the difference in the main active ingredients of both. In addition, the antibacterial effects of luteolin, quercetin, and kaempferol have been reported several times. Luteolin exerts its powerful antibacterial impact by disrupting the cell membranes of Staphylococcus aureus and Listeria monocytogenes, inducing changes in cell morphology, and inhibiting the formation of bacterial membranes and thus killing bacteria.29 Meanwhile, luteolin was found to inhibit the growth of Trueperella pyogenes by disrupting cell wall integrity.5 Quercetin is one of the main active ingredients of Lonicera japonica Thunb. It has been shown that quercetin can disrupt Escherichia coli and Staphylococcus aureus cell walls and alter the permeability of their cell membranes, thus inhibiting the growth of bacteria.30 Similarly, kaempferol exhibited anti-biofilm activity and inhibited the growth of Streptococcus mutans.31 This suggests that our Lonicera japonica Thunb. may play an important role by inhibiting bacterial cell wall cell membrane biosynthesis.
To gain more insight into the potential mechanism of Lonicera japonica Thunb. inhibition of Bacillus cereus ATCC14579, we analyzed 10 targets in the intersection network (fabZ, tig, glmU, secA, deoD, nagB, pgi, rpmB, recA, upp), and these 10 targets were considered to be the most important ones. Targets like fabZ, tig, glmU, secA, deoD, nagB, pgi, rpmB, recA, and upp targets have been shown to be inhibitory targets that bind to inhibitors, especially in terms of antibiotic binding sites. Available studies have shown that fabZ is an important enzyme in the extended cycle of saturated and unsaturated fatty acid biosynthesis in the type II fatty acid biosynthesis system (FAS II) pathway, and fabZ has been an effective target for compounds against pathogenic microorganisms.32 Studies have reported that tig can bind to ribosomes and nascent polypeptide chains. It is a prime candidate for chaperone proteins that specifically aid in folding newly synthesized proteins.33 glmU is a bifunctional enzyme with acetyltransferase and guanylyltransferase, essential for bacterial cell wall biosynthesis, and inhibition leads to bacterial inactivation. Therefore, it is a potential target for new antibacterial agents.27 secA is a membrane ATPase and channel protein, and numerous studies have shown that inhibition of secA produces antibacterial effects. secA is an effective target for developing new antimicrobials.34 deoD is responsible for the scavenging and catabolism of nucleotides and has been extensively studied as a potential drug target.35 nagB can isomerize glucosamine-6-P to fructose-6-P, and inactivation of nagB can affect the bacterial synthesis of the cell wall and thus inhibit growth.36 Recently, it was shown that pgi is an important enzyme that regulates glycolytic and glycoisomeric pathways. In levofloxacin-treated divergent Mycobacterium tuberculosis, mRNA expression of pgi is down-regulated, and methylation levels are up-regulated, playing a crucial role in levofloxacin-resistant divergent Mycobacterium tuberculosis.37 Studies have shown that rpmB encodes the L28 protein of the larger 50S ribosomal subunit, which plays an important role in ribosome assembly.38 Studies have shown that inhibition of recA facilitates antibiotic combination therapy and reduces the ability of bacteria to produce drug-resistant mutants.39 Similarly, upp is involved in the biosynthesis of peptidoglycan, lipopolysaccharide, and wall phosphate, enzymes essential for bacterial growth, and upp is expected to be an effective target against drug-resistant bacteria.40 Thus, the findings suggest that fabZ, tig, glmU, secA, deoD, nagB, pgi, rpmB, recA, and upp play important roles in peptidoglycan biosynthesis, phospholipid biosynthesis, and ribosome assembly, inhibition of which may be achieved by Lonicera japonica Thunb. through the inhibition of these targets. Therefore, luteolin, quercetin, and kaempferol could play key roles in peptidoglycan biosynthesis, phospholipid biosynthesis, and ribosome assembly. GO enrichment analysis showed that these targets were mainly involved in the peptidoglycan biosynthesis process, phospholipid biosynthesis process, and ribosome assembly. Based on these findings, we hypothesize that Lonicera japonica Thunb. may exert its inhibitory effects by inhibiting the peptidoglycan biosynthesis pathway, phospholipid biosynthesis pathway, and ribosome assembly of Bacillus cereus ATCC14579. The research has shown that the peptidoglycan biosynthesis pathway is a complex process, and peptidoglycan is an important component of fungal and bacterial cell walls. The peptidoglycan biosynthesis enzymes are considered effective targets for antibacterial agents. Similarly, the phospholipid biosynthesis pathway is essential for bacteria to maintain their life activities, phospholipids are major components of various membranes in all domains of life, and phospholipids play an important role in bacterial infections and are targets for antibiotics to exert their efficacy.41,42 Ribosome assembly is a series of alternating RNA conformational changes and protein binding processes, and affecting ribosome assembly will lead to the cessation of bacterial life activities. Cofactors involved in ribosome assembly are considered effective targets for bacteriostatic agents.43
To verify the network pharmacology results, it was observed by transmission electron microscopy that Bacillus cereus ATCC14579 treated with luteolin, quercetin, and kaempferol showed morphological cell damage, cell wall and cell membrane rupture, inhomogeneous cytoplasmic electron density, and overflow of cell contents. In particular, after treating luteolin, quercetin, and kaempferol in Bacillus cereus ATCC14579 extracellular solution, AKP enzyme activity, peptidoglycan concentration, and protein concentration were significantly increased. The results of the antibacterial assay directly demonstrated the potential mechanism of inhibition of Bacillus cereus ATCC14579 by luteolin, quercetin, and kaempferol. At the same time, it showed the good therapeutic effect of Lonicera japonica Thunb. on bacterial diseases infected by Bacillus cereus ATCC14579, which provides further experimental evidence to promote the wide application of Lonicera japonica Thunb. in bacterial infectious diseases.
Network pharmacology has become an important tool for herbal medicine research because of its comprehensiveness, systematicity, and comprehensiveness in line with the characteristics of herbal medicine with multiple compounds, multiple targets, and multiple pathways.44 Although network pharmacology has a broad application prospect, it still has certain limitations. First, the existing database for network pharmacology is incomplete, which can lead to a lack of analytical data. Second, current network pharmacology technologies make it difficult to quantify specific targets. Therefore, numerous in vivo and in vitro experiments are needed to test the scientific validity. In the future, high throughput, faster, and more accurate methods can be found to address these limitations with innovations in network pharmacology technologies.
Conclusions
Bacillus cereus is an important human pathogen. Food poisoning caused by Bacillus cereus frequently occurs, with mild vomiting and diarrhea, and severe death. At the same time, with the increasingly serious problem of bacterial resistance, it is urgent to find new antimicrobial agents. Lonicera japonica Thunb. has a wide range of medicinal activities, it is necessary to study the Lonicera japonica Thunb. for the inhibition of Bacillus cereus. Based on network pharmacology, the potential mechanism of Lonicera japonica Thunb. inhibition of Bacillus cereus ATCC14579 involves multiple biological processes and targets. Lonicera japonica Thunb. exerts its antimicrobial effect probably by inhibiting the synthesis pathway of phospholipids and peptidoglycans, thereby disrupting the cell membrane and cell wall of Bacillus cereus ATCC14579. The results of this study suggest that Lonicera japonica Thunb. maybe an effective agent for inhibiting Bacillus cereus ATCC14579 and provide further evidence for its application in diseases such as bacterial infections. At the same time, it paved the way for the subsequent development of new bacteriostatic agents.
Author contributions
NX: conceived ideas, completed experiments, and wrote the article. Y-cC: conceived ideas, and processed and organized the metabolomic data. L-hD: revised the article for language issues. J-hZ and Q-fZ: collected and organized the data. RC: assisted in completing experiments. G-pP: provided and identified plant material. Q-mW and H-zY: discussed the results. L-qR: designed and funded experiments.
Conflicts of interest
There are no conflicts to declare.
Acknowledgements
This study was supported by the Science and Technology Special Fund of the Hunan Provincial Development and Reform Commission (2016, No. 245).
Notes and references
- K. Dierick, E. Van Coillie, I. Swiecicka, G. Meyfroidt, H. Devlieger, A. Meulemans, G. Hoedemaekers, L. Fourie, M. Heyndrickx and J. Mahillon, J. Clin. Microbiol., 2005, 43, 4277–4279 CrossRef PubMed.
- E. J. Bottone, Clin. Microbiol. Rev., 2010, 23, 382–398 CrossRef PubMed.
- A. C. Lopez, J. Minnaard, P. F. Perez and A. M. Alippi, Food Microbiol., 2015, 46, 195–199 CrossRef PubMed.
- R. Pena-Miller, D. Laehnemann, G. Jansen, A. Fuentes-Hernandez, P. Rosenstiel, H. Schulenburg and R. Beardmore, PLoS Biol., 2013, 11, e1001540 CrossRef CAS PubMed.
- T. Gao, Y. Ding, Q. Wu, J. Wang, J. Zhang, S. Yu, P. Yu, C. Liu, L. Kong, Z. Feng, M. Chen, S. Wu, H. Zeng and H. Wu, Front. Microbiol., 2018, 9, 533 CrossRef PubMed.
- L. Zhang, A. S. Ravipati, S. R. Koyyalamudi, S. C. Jeong, N. Reddy, J. Bartlett, P. T. Smith, M. de la Cruz, M. C. Monteiro, A. Melguizo, E. Jimenez and F. Vicente, Asian Pac. J. Trop. Med., 2013, 6, 673–681 CrossRef PubMed.
- E. van Galen, J. Ethnopharmacol., 2014, 158, 498–502 CrossRef PubMed.
- M. Akram, M. Riaz, N. Munir, A. Rasul, M. Daniyal, S. M. Ali Shah, M. A. Shariati, G. Shaheen, N. Akhtar, F. Parveen, N. Akhter, A. Owais Ghauri, A. W. Chishti, M. Usman Sarwar and F. Said Khan, Clin. Exp. Pharmacol. Physiol., 2020, 47, 1107–1119 CrossRef CAS PubMed.
- T. Su, Y. Qiu, X. Hua, B. Ye, H. Luo, D. Liu, P. Qu and Z. Qiu, Front. Microbiol., 2020, 11, 610070 CrossRef PubMed.
- L. Luo, J. Yang, C. Wang, J. Wu, Y. Li, X. Zhang, H. Li, H. Zhang, Y. Zhou, A. Lu and S. Chen, Sci. China: Life Sci., 2022, 65, 1123–1145 CrossRef CAS PubMed.
- X. Shang, H. Pan, M. Li, X. Miao and H. Ding, J. Ethnopharmacol., 2011, 138, 1–21 CrossRef CAS PubMed.
- Y. R. Lee, S. F. Yeh, X. M. Ruan, H. Zhang, S. D. Hsu, H. D. Huang, C. C. Hsieh, Y. S. Lin, T. M. Yeh, H. S. Liu and D. D. Gan, J. Ethnopharmacol., 2017, 198, 109–121 CrossRef CAS PubMed.
- J. Yang, Y. C. Li, X. R. Zhou, X. J. Xu, Q. Y. Fu and C. Z. Liu, Nat. Prod. Res., 2018, 32, 2238–2243 CrossRef CAS PubMed.
- L. Ge, L. Xiao, H. Wan, J. Li, K. Lv, S. Peng, B. Zhou, T. Li and X. Zeng, Bioorg. Chem., 2019, 92, 103198 CrossRef CAS PubMed.
- X. Su, Z. H. Zhu, L. Zhang, Q. Wang, M. M. Xu, C. Lu, Y. Zhu, J. Zeng, J. A. Duan and M. Zhao, J. Ethnopharmacol., 2021, 267, 113502 CrossRef CAS PubMed.
- L. Ge, Q. Xie, Y. Jiang, L. Xiao, H. Wan, B. Zhou, S. Wu, J. Tian and X. Zeng, Phytomedicine, 2022, 96, 153889 CrossRef CAS PubMed.
- L. Ni, L. Chen, X. Huang, C. Han, J. Xu, H. Zhang, X. Luan, Y. Zhao, J. Xu, W. Yuan and H. Chen, Acta Pharm. Sin. B, 2020, 10, 1149–1162 CrossRef CAS PubMed.
- J. Song, J. Zhao, X. Cai, S. Qin, Z. Chen, X. Huang, R. Li, Y. Wang and X. Wang, J. Ethnopharmacol., 2022, 298, 115653 CrossRef CAS PubMed.
- Y. Li, W. Li, C. Fu, Y. Song and Q. Fu, Phytochem. Rev., 2020, 19, 1–61 CrossRef CAS PubMed.
- B. Shan, Y. Z. Cai, J. D. Brooks and H. Corke, Int. J. Food Microbiol., 2007, 117, 112–119 CrossRef CAS PubMed.
- X. Wang, Z. Y. Wang, J. H. Zheng and S. Li, Chin. J. Nat. Med., 2021, 19, 1–11 CAS.
- M. A. Kohanski, D. J. Dwyer, B. Hayete, C. A. Lawrence and J. J. Collins, Cell, 2007, 130, 797–810 CrossRef CAS PubMed.
- R. P. Lange, H. H. Locher, P. C. Wyss and R. L. Then, Curr. Pharm. Des., 2007, 13, 3140–3154 CrossRef CAS PubMed.
- L. L. Silver, in Antimicrobial Therapeutics Reviews: The Bacterial Cell Wall as an Antimicrobial Target, ed. K. Bush, 2013, vol. 1277, pp. 29–53 Search PubMed.
- D. G. Brown, Bioorg. Med. Chem., 2016, 24, 6320–6331 CrossRef CAS PubMed.
- R. Dietrich, N. Jessberger, M. Ehling-Schulz, E. Martlbauer and P. E. Granum, Toxins, 2021, 13, 98 CrossRef CAS PubMed.
- H. Barreteau, A. Kovac, A. Boniface, M. Sova, S. Gobec and D. Blanot, FEMS Microbiol. Rev., 2008, 32, 168–207 CrossRef CAS PubMed.
- Y. M. Zhang and C. O. Rock, J. Lipid Res., 2008, 49, 1867–1874 CrossRef CAS PubMed.
- W. Qian, M. Liu, Y. Fu, J. Zhang, W. Liu, J. Li, X. Li, Y. Li and T. Wang, Microb. Pathog., 2020, 142, 104056 CrossRef CAS PubMed.
- X. Wang, Y. Cao, S. Chen, J. Lin, J. Bian and D. Huang, J. Agric. Food Chem., 2021, 69, 7285–7302 CrossRef CAS PubMed.
- Y. Zeng, A. Nikitkova, H. Abdelsalam, J. Li and J. Xiao, Arch. Oral Biol., 2019, 98, 9–16 CrossRef CAS PubMed.
- L. Zhang, W. Liu, T. Hu, L. Du, C. Luo, K. Chen, X. Shen and H. Jiang, J. Biol. Chem., 2008, 283, 5370–5379 CrossRef CAS PubMed.
- E. Deuerling, A. Schulze-Specking, T. Tomoyasu, A. Mogk and B. Bukau, Nature, 1999, 400, 693–696 CrossRef CAS PubMed.
- A. S. Chaudhary, W. Chen, J. Jin, P. C. Tai and B. Wang, Future Med. Chem., 2015, 7, 989–1007 CrossRef CAS PubMed.
- J. A. Koopman, J. M. Marshall, A. Bhatiya, T. Eguale, J. J. Kwiek and J. S. Gunn, Antimicrob. Agents Chemother., 2015, 59, 76–84 CrossRef PubMed.
- H. Komatsuzawa, T. Fujiwara, H. Nishi, S. Yamada, M. Ohara, N. McCallum, B. Berger-Bachi and M. Sugai, Mol. Microbiol., 2004, 53, 1221–1231 CrossRef CAS PubMed.
- H. C. Li, H. X. Guo, T. Chen, W. Wang, Z. H. Wu, L. Chen, H. Z. Wu, G. P. Xu, X. X. Chen and L. Zhou, J. Mol. Evol., 2020, 88, 202–209 CrossRef CAS PubMed.
- B. A. Maguire and D. G. Wild, Biochim. Biophys. Acta, 1997, 1353, 137–147 CrossRef CAS PubMed.
- T. D. Thi, E. Lopez, A. Rodriguez-Rojas, J. Rodriguez-Beltran, A. Couce, J. R. Guelfo, A. Castaneda-Garcia and J. Blazquez, J. Antimicrob. Chemother., 2011, 66, 531–538 CrossRef CAS PubMed.
- N. Koyama, J. Inokoshi and H. Tomoda, Molecules, 2012, 18, 204–224 CrossRef PubMed.
- S. Kuhn, C. J. Slavetinsky and A. Peschel, Int. J. Med. Microbiol., 2015, 305, 196–202 CrossRef CAS PubMed.
- Z. Li, Y. Tang, Y. Wu, S. Zhao, J. Bao, Y. Luo and D. Li, Nat. Commun., 2017, 8, 1691 CrossRef PubMed.
- Z. Shajani, M. T. Sykes and J. R. Williamson, in Annual Review of Biochemistry, vol 80, ed. R. D. Kornberg, C. R. H. Raetz, J. E. Rothman and J. W. Thorner, 2011, vol. 80, pp. 501–526 Search PubMed.
- C. Huang, C. Zheng, Y. Li, Y. Wang, A. Lu and L. Yang, Briefings Bioinf., 2014, 15, 710–733 CrossRef PubMed.
|
This journal is © The Royal Society of Chemistry 2023 |
Click here to see how this site uses Cookies. View our privacy policy here.