DOI:
10.1039/D3RA00371J
(Paper)
RSC Adv., 2023,
13, 13834-13839
Discussion on the need for correction during isotopic analysis of nitrogen by the denitrifier method
Received
18th January 2023
, Accepted 25th April 2023
First published on 5th May 2023
Abstract
The nitrogen and oxygen isotopes of NO3− are effectively used to trace the main nitrogen sources and migration processes in the atmosphere, water and soil. NO3− can be converted into N2O by the bacterial denitrification method, which is an advanced method with high sensitivity. However, due to the existence of a small but inevitable blank during the whole experimental process, the N isotopic signal of N2O produced by denitrification superimposes on that of the N blank. Currently, the standard curve correction method is used to correct measured nitrogen isotope results to mitigate blank interference. It has been reported that high variability of the nitrogen isotope results have been produced by the denitrifier method by conducting an interlaboratory comparison of denitrifier methods and other methods on standards and environmental samples, and to reduce this problem, the nitrogen isotope calibration process with a standard curve is examined in depth in this paper, which uses PreCon-GC-IRMS to determine the nitrogen isotopes in N2O. We demonstrate for the first time that reliable results can be obtained without correction for samples with nitrogen isotope composition ranging from −9.9 to 19.5‰, which covers the natural sample range. This study establishes the double test approach for the bacterial denitrification method, ensuring the accuracy and long-term stability of different batches of nitrogen isotope results.
Introduction
The nitrogen and oxygen isotopes in nitrate are effective ways to trace the nitrogen sources and conversion processes in the atmosphere,1–5 surface water,6 groundwater,7 oceans8 and soil.9,10 Since the 1960s, the pretreatment methods of the nitrogen and oxygen isotopes in nitrate mainly include the Kjeldahl method,11 diffusion method,12 ion exchange resin method13,14 and denitrifier method. Denitrification can simultaneously determine nitrogen and oxygen isotopes and is divided into chemical denitrification15 and bacterial denitrification.16,17 Denitrification has become an internationally recognized advanced nitrogen and oxygen isotope pretreatment technology because of its high sensitivity and efficiency and its usefulness for high-salinity samples.
Since the bacterial denitrifier method was proposed by Sigman in 2001,16 this method has continuously received extensive attention from researchers. For example, the bacterial method was applied to the determination of the nitrogen and oxygen isotopic compositions of NO3− in soil KCl extracts by Rock et al.18 and Mørkved et al.19 Furthermore, the contamination and blank correction introduced by the KCl reagent during the above assay were studied in detail by Bell et al.20 The sensitivity and long-term stability of the method have been improved by researchers through modifying the online instruments used for the purification and enrichment of N2O.21,22 In recent years, laser isotope meters have also been used in the determination of the nitrogen and oxygen isotopes in the produced N2O.23
The above research improves the bacterial denitrifier method to be more sensitive, efficient and stable with a wider range of applications. However, the standard calibration method is still widely used to calculate the nitrogen isotope results. In theory, the method uses a specific denitrifying bacteria, which cannot further reduce N2O, to completely convert all of the NO3− in the sample to N2O. In this way, the nitrogen in the produced N2O should completely record the nitrogen isotope signal in the reactant NO3−. However, due to the small but inevitable whole-process blanks in the experimental process, the measured nitrogen isotope results in N2O include the contribution of N of the blank. Therefore, this issue is usually solved by the standard calibration method, in which a standard calibration curve is prepared by treating an isotope standard substance solution in the same batch as the sample to be tested.17 However, it has been reported that high variability of the nitrogen isotope results have been produced by the denitrifier method by conducting an interlaboratory comparison of denitrifier methods and other methods on standards and environmental samples.24 And one of the potential reasons which caused the big variability is that the measured results needed to be corrected by the nitrogen isotope calibration. Therefore, the standard calibration method to correct the measurement results of nitrogen isotopes should be used with caution. To reduce the potential variability caused by the calibration, this paper intends to determine whether the true value of the sample can be directly obtained without correcting the nitrogen isotope result when applying the bacterial denitrification method combined with the online preconcentration instrument (PreCon-GC-IRMS) to determine the nitrogen isotope in N2O. This study will establish a solution to verify the reliability of the currently widely used calibration method. Furthermore, the study can provide a double guarantee for obtaining reliable nitrogen isotope results.
Experimental
International standard materials that are commonly used in the bacterial denitrifier method to obtain nitrogen calibration curves are USGS-32 (KNO3, δ15N–NO3− = 180‰), USGS-34 (KNO3, δ15N–NO3− = −1.8‰) and IAEA-NO3 (KNO3, δ15N–NO3− = 4.7‰). It is important that the isotopic composition of the standards in the calibration curve can cover the natural samples to ensure the accuracy of calibration.22 Therefore, to consider samples whose nitrogen isotopic composition ranges from 4.7‰ to 180‰, and USGS-34 and USGS-32 are configured as solutions A and B, respectively, with a concentration of 20 μmol L−1 NO3−. Solutions A and B are mixed in a volume ratio of 5
:
1 and 4
:
2, respectively, to obtain nitrate solutions with different nitrogen isotopic compositions, which are described as U51 and U42, respectively. These standards were chosen to configure U42 and U51 to provide a wider isotopic range distribution of the standard curve while improving the accuracy of the test results. The mixing ratios and theoretical calculation values of the isotopic composition of the NO3− in the solution are listed in Table 1.
Table 1 Nitrogen isotopic ratio for international references and laboratory standards
Isotope standard samples |
δ15N vs. air (‰) |
U42 was mixed by isotope standard solutions. And the volume ration of USGS-34 and USGS-32 is 4 : 2. U51 was mixed by isotope standard solutions. And the volume ration of USGS-34 and USGS-32 is 5 : 1. |
International standard |
USGS-32 |
180 |
USGS-34 |
−1.8 |
IAEA-NO3 |
4.7 |
Laboratory standards |
U42a |
58.8 |
U51b |
28.5 |
Sample analysis
All reagents are analytical reagent grade. The strain used in the experiment was Pseudomonas aureofaciens. The medium was tryptic soy broth (TSB) and tryptic soy agar (TSA). The solid medium was made by dissolving 23 g TSA and 0.5 g KNO3 in 500 mL ultrapure water. This solution was sterilized for 30 min and then poured into a plate in the sterile table. The liquid medium with nitrate is made by dissolving the following materials, including 120 g TSB, 4 g KNO3, 2 g (NH4)2SO4 and 19.6 g KH2PO4 in 4000 mL ultrapure water. Another liquid medium without nitrate is made by dissolving the following materials, including 30 g TSB, 0.5 g (NH4)2SO4, and 4.9 g KH2PO4 in 1000 mL ultrapure water. These solutions are sterilized for 50 min and cooled before use.
The bacterial culture method has been described in the literature.16,17 The scheme of the denitrifier method in this study was shown in Fig. 1.
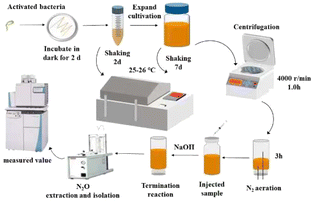 |
| Fig. 1 The scheme of the denitrifier method in this study. | |
Testing methods
The PreCon system is connected to an autosampler, with the N2O in the headspace bottle exported by a He gas flow, while the H2O and CO2 in the gas stream are removed by a chemical trap. The PreCon system enriches the N2O in liquid nitrogen and then separates N2O (m/z = 44) and CO2 (m/z = 44) using a gas chromatography (GC) column (HP-Plot-Q column, Agilent). The separated sample gas, N2O (m/z = 44), then enters a mass spectrometer. The measured isotope values correspond to international standards.
In the equation, Rsample and Rstandard are the ratios of 15N/14N of the sample and standard, respectively. The isotope measurement is conducted based on the sample relative to the reference gas isotope value. To verify whether the isotope results can be obtained directly without calibration, the N2O reference gas nitrogen and oxygen isotope are assigned by the isotope standard material IAEA-NO3.
Experiment 1: blank experiment
To identify the contribution of nitrogen in the whole-process blank, 1 mL ultrapure water, the same volume as that of the standard solution, was injected into 3 mL bacterial solution prepared using different batches of bacteria to test the entire process blank. The results show that the blank content of the whole process is within the 0.2 nmol N range. This blank content is calculated from the ratio of the m/z 44 peak area generated by the blank sample compared to that generated by the standard sample with a known N injection amount. The nitrogen isotopic composition of blank is approximately 4.8‰. The uncertainties regarding this nitrogen isotope are reflected in the data and examined in the discussion section.
Experiment 2: validity of the standard curve correction method
To verify whether the standard curve correction method can effectively deduct the contribution of the whole-process nitrogen blank as a way to obtain the true nitrogen isotope results of the samples. In this paper, a conditional experiment in which a blank with known nitrogen and isotopic compositions is artificially mixed into a natural sample is designed. First, it is assumed that the calculated nitrogen isotopic composition of natural river water sample CH-8 is the true value of the sample. The calculated nitrogen isotope is calculated by the standard curve correction method, which does not add any blank. Then, different volumes of CH-8 are separately injected into bacterial solutions. Different volumes of international standard solutions (IAEA-NO3, USGS-32 and USGS-34) are the separately injected into these solutions. The nitrogen ratios of CH-8 and the standard solutions are adjusted by changing the injection volumes of CH-8 and the standard solutions. As a result, the nitrogen in the IAEA-NO3, USGS-32 and USGS-34 standard solutions accounted for 10%, 30%, and 50% of the total nitrogen, respectively. The total amount of injected NO3− is 20 nmol. At the same time, the calibration curve is prepared under the same conditions. The calibration results of the above samples at different blank nitrogen contents and isotopic compositions are calculated by the calibration curve. Finally, the difference between the corrected result and the true value is compared, as shown in Fig. 2.
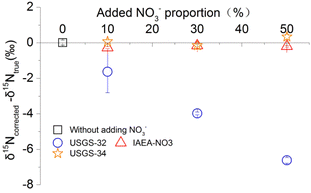 |
| Fig. 2 Influence of the added nitrate standards (USGS-32, USGS-34 and IAEA-NO3) with different NO3− addition proportions (NO3− addition proportion = nmol of NO3− added/20 nmol NO3−) and known δ15N values on the deviation of the corrected δ15N values from the true δ15N values of natural samples (assuming that the corrected δ15N values without any addition are the true values of the natural samples). δ15Ntrue is the known nitrogen isotope value given by the standard and δ15Ncorrected is the nitrogen isotope value calculated using the standard. | |
Experiment 3: the effect of the isotopic composition on the deviation of the measured isotope value from the true value
To examine whether the bacterial denitrifier method can obtain reliable nitrogen isotope results without calibration, this paper prepares the standard curve by using the international standard materials covering the largest nitrogen isotope range. USGS-32, USGS-34, IAEA-NO3, U42 and U51 were prepared as standard 20 μmol L−1 NO3− solutions. One milliliter of the above standard solution was injected into the prepared bacterial solution to determine the nitrogen isotopic composition. The measurement is carried out by using different batches of bacteria and repeating several times at different times. The deviation between the average and true values of the multiple measurements is calculated. As a result, the relationship between the degree of deviation and the isotopic composition is obtained, as shown in Fig. 3.
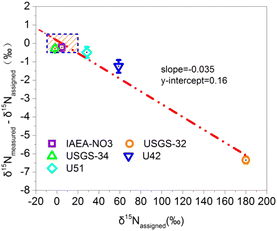 |
| Fig. 3 Deviation of the measured δ15N values from the assigned δ15N values (δ15Nmeasured − δ15Nassigned) at 20 nmol NO3− versus different δ15N values. The red dashed lines show the linear regression relationships between the deviation and isotopic values. The boxes show that the deviation is below ±0.5‰, and the values of the samples located in these boxes do not need to be corrected. | |
Experiment 4: testing of the uncorrected isotope results by the natural samples
To further verify whether nitrogen isotope results can be obtained directly without calibration, the river water samples are treated with different batches of bacteria, and the nitrogen isotopic compositions are determined at different times. The deviations of the calibration results compared with the measured values are summarized in Table 2. In addition, to test the difference between the test results using traditional correction methods and non correction methods, a one-way ANOVA was performed on the data in Table 2 using SPSS software.
Table 2 Comparison of the differences in the δ15N measurements of the natural samples between replicate batches that were corrected or not corrected by the calibration curve for δ15N
Sample no. |
Date of measurement |
δ15Nma |
δ15Ncb |
δ15Nm − δ15Ncc |
‰ |
‰ |
‰ |
δ15Nm represents the δ15N value measured by the uncorrected standard curve. δ15Nc represents the δ15N value measured by the corrected standard curve. δ15Nm − δ15Nc represents the difference between the uncorrected and corrected values. |
CH-8 |
2019.4.10 |
6.3 |
6.0 |
0.3 |
CH-12 |
2019.4.10 |
4.1 |
3.7 |
0.4 |
CH-13 |
2019.4.10 |
8.9 |
8.6 |
0.3 |
CH-15 |
2019.4.10 |
8.5 |
8.3 |
0.2 |
LH-7 |
2019.4.10 |
12.5 |
12.4 |
0.1 |
LH-8 |
2019.4.10 |
12.5 |
12.4 |
0.1 |
![[thin space (1/6-em)]](https://www.rsc.org/images/entities/char_2009.gif) |
QH4 |
2019.4.17 |
12.1 |
12.0 |
0.1 |
QH5 |
2019.4.17 |
5.7 |
5.4 |
0.3 |
QH6 |
2019.4.17 |
13.0 |
12.9 |
0.1 |
QH9 |
2019.4.17 |
16.6 |
16.7 |
−0.1 |
LH19 |
2019.4.17 |
7.1 |
6.8 |
0.3 |
LH24 |
2019.4.17 |
7.1 |
6.9 |
0.2 |
![[thin space (1/6-em)]](https://www.rsc.org/images/entities/char_2009.gif) |
HH1 |
2019.4.19 |
1.5 |
1.2 |
0.3 |
HH5 |
2019.4.19 |
1.7 |
1.3 |
0.3 |
HH12 |
2019.4.19 |
8.5 |
8.5 |
0.0 |
HH16 |
2019.4.19 |
8.8 |
8.8 |
0.0 |
KMC5 |
2019.4.19 |
11.0 |
11.0 |
0.0 |
KMC7 |
2019.4.19 |
12.4 |
12.4 |
0.0 |
Results and discussion
Evaluation of whether the standard curve correction method can effectively correct the detected nitrogen isotope values
To examine in great detail whether the standard curve correction method can effectively deduct the contribution of the blank nitrogen in the whole process, a standard substance with a known isotopic composition is artificially added as a blank. Therefore, the proportion of the blank nitrogen in the total nitrogen is increased. The additions accounted for 10%, 30% and 50% of the total nitrogen content. The specific experimental design is as described in Experiment 2.
It can be seen from Fig. 2 that even if the proportion of nitrogen in IAEA-NO3 (δ15N = 4.7‰) is as high as 50%, the corrected nitrogen isotope result deviates from the true value by less than 0.3‰. This result occurs because the isotopic composition of the mixed blank is close to that of the sample to be tested. Even if its contribution ratio is high, IAEA-NO3 has little effect on the isotope results. However, although the ratio of USGS-32 (δ15N = 180‰) is lower than 10%, the calculated nitrogen isotope result deviates from the true value by more than 1.6‰. This is because the nitrogen isotopic composition of USGS-32 deviates greatly from those of most natural samples. Even if a small amount of USGS-32 is introduced, its effect on the isotope results is large. The above results demonstrate that the standard curve correction method cannot deduct the influence of the blank when the nitrogen isotopic composition of the blank deviates greatly from those of the tested samples. Therefore, the standard curve correction method cannot completely solve the problem of blank pollution.
In bacterial denitrification blank nitrogen comes mainly from the reagent, the bacterial protocol and instrumentation. Currently, the contribution of nitrogen in the full process blank can be reduced to as low as 1% of the total nitrogen by minimizing isotopic drift and by improving the calibration of O isotopes.21,22,25 It is generally believed that as long as the blank nitrogen contribution is sufficiently low, the nitrogen isotope result deviation is 0.25‰ even if the blank nitrogen isotopic composition is 100‰.22 Sigman et al. estimated that the nitrogen isotopic composition of their laboratory blank was approximately 5‰. Additionally, the isotopic composition was not significantly different from those of most natural samples.16 That is, the nitrogen isotopic composition can be effectively calculated by the standard curve correction method under the following preconditions: (1) the nitrogen contribution ratio in the whole-process blank is strictly controlled to be small enough, (2) the deviation of the isotopic composition between the blank and natural sample is not large, and (3) the blank nitrogen has the same effect on the standard and the tested samples.
In the actual application process, the amount of nitrogen in the whole-process blank can be directly obtained from the peak area of m/z 44. However, the m/z 44 peak area of the blank is too small to directly obtain the nitrogen isotopic composition of the blank. Thus, it is difficult to judge the degree of deviation of the nitrogen isotope values between the blank and the tested sample. Therefore, to a certain extent, the accuracy and efficiency of obtaining accurate nitrogen isotope values through the calibration curve method is affected by the above factors.
Discussion on the need for correction
To investigate whether the bacterial denitrification method can obtain reliable nitrogen isotope results without calibration, the conditional experiment was carried out according to the description of Experiment 3. As a result, as shown in Fig. 3, the deviation between the nitrogen isotope measurement value and the true value is linearly related to its true value. From the calculation of the linear equation, when the nitrogen isotopic composition is 4.8‰, the difference between the measured value and the true value is close to 0‰. The isotope signal superimposed by the blank does not change the original isotope result of the sample with the same isotopic composition as the blank. Therefore, it is speculated that the nitrogen isotopic composition of the blank of the whole experiment is approximately 4.8‰. Furthermore, when the nitrogen isotopic composition deviated from the blank value by 1‰, the deviation of the measured value from the true value was 0.034‰. As shown by the shaded area in the blue dotted line in Fig. 3, when the nitrogen isotopic composition is in the range of −9.9 to 19.5‰, the deviation of the direct measurement from its true value is less than ±0.5‰, which can satisfy the accuracy requirements for natural sample isotope measurements. Therefore, it is preliminarily speculated that for a natural sample with a nitrogen isotopic composition distribution ranging from −9.9 to 19.5‰, the true nitrogen isotope value can be directly obtained without using the standard curve correction method. This range covers the nitrogen isotope distribution of most natural samples.
The deviation between the nitrogen isotope measurement and the true value is linearly related to its true value. The reason is that there is a small but inevitable blank contribution in the experimental process. That is, the measured value is the result of the overlap of two end sources. One is the N2O in the blank, and the other is the N2O converted from the NO3− in the sample. Under the premise of a stable blank and consistent pretreatment conditions of the bacterial denitrifier method, the nitrogen content and the isotopic composition of the blank contributed by different batches of bacteria and instruments can be kept within a small range. The true value of a natural sample with a nitrogen isotopic composition distribution within the range of −9.9 to 19.5‰ can be obtained directly without using the standard curve correction method.
In addition, due to differences in the experimental environments, pretreatment conditions and instrument settings of different laboratories, each laboratory can establish its uncorrected nitrogen isotope range based on the method proposed in this paper. Moreover, the smaller the nitrogen contribution of the blank is, the larger the uncorrected nitrogen isotope range is that can be covered by the uncorrected curve, and as a result, the nitrogen isotopic composition of more natural samples can be obtained without correction.
The method provides a double test method for obtaining reliable nitrogen isotope results by the bacterial denitrifier method. The standard and parallel samples can be measured in different batches. Therefore, the nitrogen isotope result directly measured can be used to control the quality of the experimental data, avoiding errors that may be superimposed in the standard curve correction process, which is beneficial for a better control of the data quality and long-term stability of different batch data comparisons. It is also possible to reduce the labor and material resources required for the standard curve correction method in measuring a large number of standard samples per batch.
Evaluation of the uncorrected isotope results by natural samples
The nitrogen isotope measurement results of river water samples treated with different batches of bacteria and measured at different times are listed in Table 2. The directly obtained results are compared to the corrected results obtained by conventional standard curve correction methods. The nitrogen isotope distribution range is from 1.5 to 16.7‰, which agrees with the uncorrected nitrogen isotope range proposed in this paper. The results demonstrate that the deviation between the measured value and the corrected result is less than 0.3‰, which confirms that nitrogen isotope results can be obtained without correction. Then, to test the differences in test results between the traditional correction method and the method without correction in this experiment, we subjected the data in Table 2 to a one-way ANOVA. Before using this statistical method, the data were checked for normality (Kolmogorov–Smirnov's test) and for homogeneity of variances (Levene's test). The results showed P > 0.05, indicating that there was no significant difference between the results of the conventional correction method and the uncorrected method when the nitrogen isotope values were in the range of −9.9–19.5‰. Therefore, it is feasible to use the method without correction directly.
Conclusions
In this paper, the commercial PreCon instrument is combined with a stable isotope mass spectrometer to determine the nitrogen isotopes in the N2O produced by the bacterial denitrifier method. Through the in-depth examination of the internal mechanism of nitrogen isotope calibration, it is first proposed that the true values of the nitrogen isotopes can be directly obtained without correction for samples with a nitrogen isotope content ranging from −9.9 to 19.5‰, which is more efficient in routine measurements. At the same time, each laboratory can determine its uncorrected nitrogen isotope range according to its blank conditions. A larger coverage of the uncorrected nitrogen isotope range can be obtained with a smaller contribution of the blank nitrogen and a smaller deviation of the blank nitrogen isotopic composition from that of the natural sample. The results of this study can provide a double test standard for the standard curve correction method. This uncorrected method can also mitigate the possible problems of preprocessing and the instrument measurement process. As a result, the problem of random errors being corrected as systematic errors when using the standard curve correction method can be avoided, and the amount of international standard substances that need to be used can be reduced, which can reduce the cost of experiments. In conclusion, this study further improved the application scope and testing efficiency of the bacterial denitrification method, and also provided a basis for other laboratories to establish a suitable bacterial denitrification method.
Conflicts of interest
There are no conflicts to declare.
Acknowledgements
The authors are very grateful to Prof. Shuji Gao at Xiamen University and his research team, Zhenzhen Zheng, Liwei Zheng, Min Xu and Ehui Tan, for their guidance and assistance in the bacterial denitrification method. This research was supported by the National Natural Science Foundation of China (Grant No. 42207300, 41991252) and Innovation Programme of Chinese Academy of Sciences, Grant/Award Number: 2024g103. We are very grateful for the financial support they have provided to us.
References
- E. M. Elliott, C. Kendall and S. D. Wankels, et al., Nitrogen isotopes as indicators of NOx source contributions to atmospheric nitrate deposition across the Midwestern and Northeastern United States, Environ. Sci. Technol., 2007, 41, 7661–7667 CrossRef CAS PubMed.
- Y. T. Fang, K. Koba and X. M. Wang, et al., Anthropogenic imprints on nitrogen and oxygen isotopic composition of precipitation nitrate in a nitrogen-polluted city in southern China, Atmos. Chem. Phys. Discuss., 2011, 10(9), 1313–1325 CrossRef.
- M. Xing and W. Liu, Variations in the concentration and isotopic composition of nitrate nitrogen in wet deposition and their relation with meteorological conditions in Xi’an city, Northwest China, Appl. Geochem., 2012, 27(4), 831–840 CrossRef CAS.
- L. Luo, X. H. Yao and H. W. Gao, et al., Nitrogen speciation in various types of aerosol in spring over the northwestern Pacific Ocean, Atmos. Chem. Phys., 2016, 16, 325–341 CrossRef CAS.
- X. Y. Liu, H. W. Xiao and H. Y. Xiao, et al., Stable isotope analyses of precipitation nitrogen sources in Guiyang, southwestern China, Environ. Pollut., 2017, 230, 486–494 CrossRef CAS PubMed.
- M. Xing and W. Liu, Using dual isotopes to identify sources and transformations of nitrogen in water catchments with different land uses, Loess Plateau of China, Environ. Sci. Pollut. Res., 2016, 23(1), 388–401 CrossRef CAS PubMed.
- S. L. Li, C. Q. Liu, H. Y. Xiao and F. X. Tao, et al., Using δ15N to assess groundwater nitrogen pollution in Guiyang, Geochimica, 2005, 34, 257–262 CAS.
- S. J. Kao, B. Y. Wang and L. W. Zheng, et al., Spatiotemporal variations of nitrogen isotopic records in the Arabian Sea, Biogeosciences, 2015, 12(1), 1–14 CrossRef.
- W. Liu and M. Xing, Isotopic indicators of carbon and nitrogen cycles in river catchments during soil erosion in the arid Loess Plateau of China, Chem. Geol., 2012, 296–297, 66–72 CrossRef CAS.
- J. Liu and W. Liu, Soil nitrogen isotopic composition of the Xifeng loess-paleosol sequence and its potential for use as a paleoenvironmental proxy, Quat. Int., 2017, 440, 35–41 CrossRef.
- D. J. Velinsky, J. R. Pennock and J. H. Sharp, et al., Determination of the isotopic composition of ammonium–nitrogen at the natural abundance level from estuarine waters, Mar. Chem., 1989, 26(4), 351–361 CrossRef CAS.
- D. M. Sigman, M. A. Altabet and R. Michener, et al., Natural abundance-level measurement of the nitrogen isotopic composition of oceanic nitrate: an adaptation of the ammonia diffusion method, Mar. Chem., 1997, 57(3–4), 227–242 CrossRef CAS.
- S. R. Silva, C. Kendall and D. H. Wilkison, et al., A new method for collection of nitrate from fresh water and the analysis of nitrogen and oxygen isotope ratios, J. Hydrol., 2000, 228(1), 22–36 CrossRef CAS.
- M. Xing and W. Liu, An improved method of ion exchange for nitrogen isotope analysis of water nitrate, Anal. Chim. Acta, 2011, 686(1), 107–114 CrossRef CAS PubMed.
- M. R. Mcilvin and M. A. Altabet, Chemical conversion of nitrate and nitrite to nitrous oxide for nitrogen and oxygen isotopic analysis in freshwater and seawater, Anal. Chem., 2005, 77(17), 5589–5595 CrossRef CAS PubMed.
- D. M. Sigman, K. L. Casciotti and M. Andreani, et al., A bacterial method for the nitrogen isotopic analysis of nitrate in seawater and freshwater, Anal. Chem., 2001, 73(17), 4145–4153 CrossRef CAS PubMed.
- K. L. Casciotti, D. M. Sigman and M. G. Hastings, et al., Measurement of the oxygen isotopic composition of nitrate in seawater and freshwater using the denitrifier method, Anal. Chem., 2002, 74(19), 4905–4912 CrossRef CAS PubMed.
- L. Rock and B. H. Ellert, Nitrogen-15 and oxygen-18 natural abundance of potassium chloride extractable soil nitrate using the denitrifier method, Soil Sci. Soc. Am. J., 2007, 71(2), 355–361 CrossRef CAS.
- P. T. Mørkved, P. Dörsch, A. K. Søvik and L. R. Bakken, Simplified preparation for the δ15N-analysis in soil by the denitrifier method, Soil Biol. Biochem., 2007, 39, 1907–1915 CrossRef.
- M. D. Bell and J. O. Sickman, Correcting for background nitrate contamination in KCl-extracted samples during isotopic analysis of oxygen and nitrogen by the denitrifier
method, Rapid Commun. Mass Spectrom., 2014, 28(5), 520–526 CrossRef CAS PubMed.
- M. R. Mcilvin and K. L. Casciotti, Technical updates to the bacterial method for nitrate isotopic analyses, Anal. Chem., 2011, 83(5), 1850–1856 CrossRef CAS PubMed.
- M. A. Weigand, J. Foriel and B. Barnett, et al., Updates to instrumentation and protocols for isotopic analysis of nitrate by the denitrifier method, Rapid Commun. Mass Spectrom., 2016, 30(12), 1365–1383 CrossRef CAS PubMed.
- D. X. Soto, G. Koehler and K. A. Hobson, Combining denitrifying bacteria and laser spectroscopy for isotopic analyses (δ15N, δ18O) of dissolved nitrat, Anal. Chem., 2015, 87(14), 7000–7005 CrossRef CAS PubMed.
- C. Biasi, S. Jokinen and J. Prommer, et al., Challenges in measuring nitrogen isotope signatures in inorganic nitrogen forms: an interlaboratory comparison of three common measurement approaches, Rapid Commun. Mass Spectrom., 2022, 36, 9370 CrossRef PubMed.
- S. H. Dai, L. H. Xie and L. Peng, et al., Determination of nitrogen and oxygen isotopes in nitrates: a minireview, Anal. Lett., 2017, 50(13), 2045–2057 CrossRef CAS.
|
This journal is © The Royal Society of Chemistry 2023 |