DOI:
10.1039/D2RA08040K
(Paper)
RSC Adv., 2023,
13, 2225-2232
An ionic Fe-based metal–organic-framework with 4′-pyridyl-2,2′:6′,2′′-terpyridine for catalytic hydroboration of alkynes†
Received
17th December 2022
, Accepted 5th January 2023
First published on 12th January 2023
Abstract
An ionic metal–organic-framework (MOF) containing nanoscale channels was readily assembled from ditopic 4′-pyridyl-2,2′:6′,2′′-terpyridine (pytpy) and a simple iron(II) salt. X-ray structural analysis revealed a two-dimensional grid-like framework assembled by classic octahedral (pytpy)2FeII cations as linkers (with pytpy as a new ditopic pyridyl ligand) and octa-coordinate FeCl2 centers as nodes. The layer-by-layer assembly of the 2-D framework resulted in the formation of 3-D porous materials consisting of nano-scale channels. The charges of the cationic framework were balanced with anionic Cl3FeOFeCl3 in its void channels. The new Fe-based MOF material was employed as a precatalyst for syn-selective hydroboration of alkynes under mild, solvent-free conditions in the presence of an activator, leading to the synthesis of a range of trans-alkenylboronates in good yields. The larger scale applicability and recyclability of the new MOF catalyst was further explored. This represents a rare example of an ionic MOF material that can be utilized in hydroboration catalysis.
Introduction
Hydroboration of unsaturated hydrocarbons provides a facile and direct approach to organoborane compounds that have been used as crucial organic synthons in numerous carbon–carbon bond forming processes. For example, the hydroboration of alkynes with pinacolborane (HBpin) or catecholborane (HBcat) leads to formation of alkenylboronate esters which can serve as key precursors in the classic Miyaura–Suzuki coupling reaction and other organic transformations.1,2 The first catalytic hydroboration, of 3-hexyne with HBcat, was developed by Männig and Nöth in 1985 using Rh.3 A number of noble metal catalysts (such as Pd, Pt, Ru, Rh, Ir, Au and Ag) have been explored since then,4 but efforts in recent years have resulted in a surge of effective earth-abundant metal catalysts with various organic ligands.5 A catalyst-free version has also been recently reported, however, elevated temperature was required to initiate reactivity (100 °C in toluene).6
Iron, as the most earth-abundant transition metal, is particularly attractive in replacing precious metals for key catalytic conversions such as hydrogenation and hydrofunctionalizations.7 Molecular Fe-based catalysts thus have long been a research focus, taking advantage of their low cost, low toxicity and environmental sustainability.8 Several well-defined iron catalysts for the hydroboration of alkynes have been developed in the past decade, as summarized in Scheme 1. Both syn- and anti-selective hydroboration of terminal alkynes by distinct iron catalysts has been established, with syn-hydroboration process being the majority (Scheme 1).9–16 In 2013, the Thomas group reported an iron complex of bis(imino)pyridine that, upon activation with EtMgBr, displayed good syn-selective catalytic activity towards a range of alkenes, where two examples of internal alkynes were included.9 A few years later, Nishibayashi and coworkers developed a pincer-PNP iron hydride catalyst for effective syn-selective hydroboration of a range of terminal alkynes without the presence of an activator, though inferior activity was found for internal alkynes.10 Recently, Findlater's group deployed a Fe(I) catalyst of bis(arylimino)-acenaphthene ligands that promoted alkyne hydroboration with good stereo- and regioselectivity in the presence of activators (NaOtBu or NaHBEt3) at 70 °C.11 In the same year, it was reported that the structurally defined Fe–H complex, FeH(CO)(NO)(Ph3P)2, enables stereoselective hydroboration of a variety of internal alkynes at 80 °C using either HBpin or bis(pinacolato)diboron (B2pin2) as a boron source.12 In addition, Fe-catalysed syn-selective hydroboration has been also carried out using simple Fe2(CO)9 (ref. 13) and Fe3O4 (ref. 14) nanoparticles as precatalysts. In contrast, anti-selective alkyne hydroboration has rarely been achieved by Fe catalysts. Examples include two well-defined Fe–H complexes of PNP and PCP pincer ligands, reported by the Kirchner and Ruiter groups, respectively.15,16
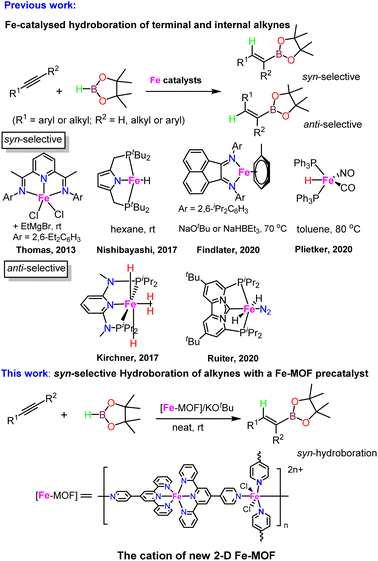 |
| Scheme 1 The state-of-the-art of iron-catalysed hydroboration of alkynes and the results presented in this work. | |
We have recently developed a handful of hydroboration catalysts of V, Mn and Al using a common tridentate chelating ligand, 2,2′:6′,2′′-terpyridine (tpy, Scheme 2).17 The redox-noninnocent nature of tpy was found to be critical in the formation of vanadium(III) and aluminum(III) complexes as well as in the catalytic cycles. We have further explored the expanded ditopic ligand L1 towards the synthesis of one-dimensional coordination polymers of cobalt and manganese, and their use as precatalysts in extremely high-efficiency hydroboration of unsaturated bonds.18 While iron has been frequently employed for tpy coordination chemistry, catalytically active Fe–tpy coordination frameworks have been sparsely reported,19 due to the formation of a stable octahedral geometry around Fe(II) with homoleptic tpy ligands.20 In search of well-defined polymeric catalysts of iron with tpy-type ligands, we report here our observation of a rare bench-stable 3-D Fe-MOF assembled from the direct reaction of ligand L1 with FeCl2, and its ability to act as a precatalyst for the syn-selective hydroboration of alkynes. This represents the first example of an ionic Fe-based MOF material as a catalyst for selective alkyne hydroboration under mild conditions.
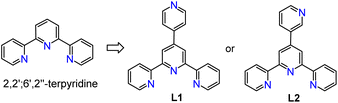 |
| Scheme 2 The structures of tpy derivatives used as ligands in this work. | |
Results and discussion
Carefully layering a CH2Cl2–MeOH solution of L1 with a MeOH solution of 2.0 equiv. of FeCl2·4H2O gave rise to the formation of dark-brown crystalline blocks of 1 in 74% yield after two weeks. 1 was characterized by IR, elemental analysis and single-crystal X-ray diffraction. X-ray diffraction analysis confirmed that 1 crystallizes in the monoclinic P21/n space group. An ORTEP representation of the repeating structural unit showing the coordination environments of the three independent FeII centers is drawn in Fig. 1. As expected, homoleptic octahedral FeII centers were formed via two tridentate tpy chelators, which serve as linear bridging bis(pyridyl) ligands. Four of these molecules are bound to another FeII center as monodentate ligands, with two chloride ligands sitting at the axial direction. Two counterions of Cl3FeOFeCl3 (ref. 21) co-crystallised in each asymmetric unit to balance the charges of the homoleptic FeII centers. The Fe–N bond lengths of the homoleptic FeII centers (Fe1 and Fe3) are between 1.869(10) and 1.998(10) Å, while those around the FeN4Cl2 center (Fe2) are significantly longer (in the range of 2.226(9)–2.320(9) Å). The latter Fe2 center with two axial chloride ligands could serve as an active reactive site through ligand exchange during catalysis. Expansion of the connectivity leads to the observation of a uniform 2-D grid-like framework, as displayed in Fig. 1. The large, nanoscale grid in the framework adopts a dimension of 2.2 × 2.2 nm, with diagonal Fe⋯Fe distances of ∼3.0–3.3 nm. The layer-by-layer packing of the 2-D framework is driven mainly by π⋯π stacking to form a 3-D MOF in which large void channels are present (see Fig. S5 in the ESI†). A number of cocrystallized solvent molecules (MeOH and H2O) are found in the void space in the X-ray structure, but they could be readily removed upon exposure to air for several hours, as evidenced by the microanalytic data of an air-dried sample. It is worth mentioning that ionic MOF materials such as these are appealing in multifunctional applications and usually challenging to synthesize,22 and Fe-based ionic MOFs are extremely rare.23 The powder X-ray diffraction analysis of a bulk sample of 1 shows a relatively poor diffraction pattern despite several attempts using different diffractors, indicating that the material may partially lose its crystallinity upon the removal of solvated molecules from the porous framework. In addition, X-ray photoelectron spectroscopy (XPS) analysis reveals the presence of both Fe2+ and Fe3+ in 1, consistent with the X-ray crystallographic results. The porosity of 1 was determined by BET surface area analysis and a relatively small BET surface area of 3.86 m2 g−1 was detected (see ESI†).
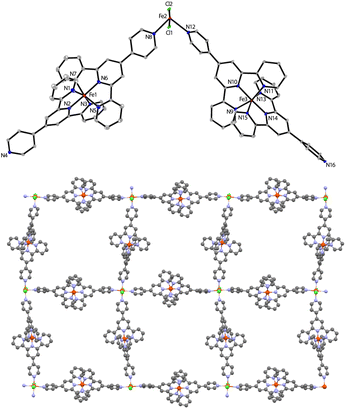 |
| Fig. 1 The ORTEP diagram of the independent molecular cation of 1 (top) with thermal ellipsoids drawn at the 30% probability level, and a ball-and-stick representation of the 2-D grid-like framework (bottom). H atoms, counterions Cl3FeOFeCl3 and co-crystallised solvent molecules are omitted for clarity. | |
In an attempt to synthesize a similar Fe-MOF structure, the closely related ligand L2 was instead employed using the same procedure. However, a discrete, mononuclear homoleptic FeII complex (2) was obtained, in which one of the uncoordinated pyridyl-N atoms is protonated (Fig. 2). Again, counterions Cl3FeOFeCl3 (1.5 per octahedral FeII center) were found to balance the protonated cationic complex in the unit cell.
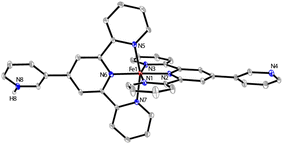 |
| Fig. 2 The ORTEP diagram of the independent molecular cation of 2 with thermal ellipsoids drawn at the 30% probability level. H atoms bound to carbons are omitted for clarity. | |
With new Fe materials in hand, catalytic reactions for the hydroboration of alkynes were then investigated. We chose phenylacetylene as a model substrate and HBpin as a boron source, and the results are summarized in Table 1. The initial test was carried out using 1 (0.2 mol%) and potassium tert-butoxide (KOtBu) as an activator in tetrahydrofuran at room temperature. It was pleasing to notice that the syn-alkenylboronate product β-(E)-3a was detected in moderate 65% yield by gas chromatography (GC) analysis, along with a small amount (<5%) of other isomeric products (entry 1, Table 1). In contrast, when 2 was used for the reaction under the same conditions, only 5% of the desired product was detected (entry 2). The inactivity of 2 as a catalyst here is consistent with the fact that in 2, FeII is in an saturated octahedral geometry with homoleptic ligands. Control experiments were conducted to examine the importance of 1 and an additive (entries 3 and 4). It was found that both 1 and the additive were required to achieve good reactivity. Next, we explored the influence of various base additives on the catalytic activity. From the results using 7 different additives, we found KOtBu was the best (entries 5–11). Finally, solvent effect was investigated to seek the best media for this reaction (entries 12–14). It was found that the reaction proceeded more smoothly in DMSO than in THF, while toluene and diethyl ether were not suitable solvents. It was further observed that the reaction actually performed better without a solvent (entry 15), where 82% yield of β-(E)-3a was detected. An attempt to improve the yield by increasing the amount of 1 to 1 mol% proved unsuccessful (entry 16). It was also noted that the reaction did not proceed at all in the air (entry 17).
Table 1 Condition screening for hydroboration of phenylacetylene with HBpina

|
Entry |
Catalyst |
Additive |
Solvent |
Yield of β-(E)-3ab (%) |
Conditions: phenylacetylene (0.5 mmol), pinacolborane (0.6 mmol), 1 (0.2 mol% based on Fe3(L)4 unit), additive (1 mol%) and solvent (0.5 mL), rt, N2, 16 h. Other isomeric products were detected with <5% yield unless otherwise stated. Determined by GC analysis with hexamethylbenzene as an internal standard. Regioisomer α-3a was detected in 8% yield. 1 mol% of 1 was used. Reaction run in the air. LiNTf2: lithium bis(trifluoromethane)sulfonimidate. |
1 |
1 |
KOtBu |
THF |
65 |
2 |
2 |
KOtBu |
THF |
5 |
3 |
1 |
None |
THF |
<5 |
4 |
None |
KOtBu |
THF |
<5 |
5 |
1 |
NaOtBu |
THF |
52 |
6 |
1 |
LiOtBu |
THF |
46 |
7 |
1 |
KOMe |
THF |
30 |
8 |
1 |
K2CO3 |
THF |
<5 |
9 |
1 |
LiNTf2 |
THF |
<5 |
10 |
1 |
NaHBEt3 |
THF |
42 |
11 |
1 |
KHBEt3 |
THF |
50 |
12 |
1 |
KOtBu |
Toluene |
<5 |
13 |
1 |
KOtBu |
Et2O |
<5 |
14c |
1 |
KOtBu |
DMSO |
78 |
15 |
1 |
KOtBu |
Neat |
82 |
16d |
1 |
KOtBu |
Neat |
83 |
17e |
1 |
KOtBu |
Neat |
Trace |
In the next step, we employed the optimal reaction conditions obtained above (entry 15, Table 1) to a variety of alkynes. The results are summarized in Scheme 3. Substituted phenylacetylenes bearing methyl, fluoro or methoxy groups are suitable substrates for the hydroboration using 1 as a precatalyst, affording the corresponding alkenylboronate products 3b–d with good yields. Aliphatic terminal alkynes are also well hydroborated under the standard conditions to give aliphatic boronate products 3e–h with high yields. The hydroboration of aliphatic internal alkynes was demonstrated by using 3-hexyne and trans-stilbene for the synthesis of 3i and 3j, respectively. Finally, two substrates containing both terminal alkene and alkyne groups were examined, and excellent chemoselectivity towards alkyne over alkene was observed, with both products 3k and 3l being isolated in appreciable yields. Unfortunately, alkynes containing heterocyclic groups such as 4-ethynylpyridine and 3-ethynylthiophene were not suitable substrates for this reaction. To demonstrate the applicability of this method, a gram-scale reaction was performed using phenylacetylene as the substrate. Thus, 3a (1.68 g) was readily isolated in 73% yield while using 10 mmol of starting materials (Scheme 3).
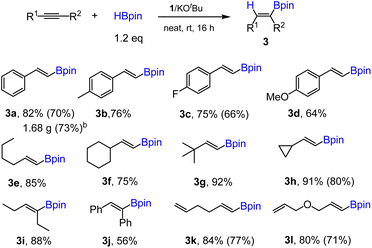 |
| Scheme 3 1-Catalysed syn-hydroboration of various alkynes.a aConditions: 1 (0.2 mol%), aldehyde (1.0 mmol) and HBpin (1.1 mmol), neat, 25 °C, 16 h, N2. GC yields are determined using hexamethylbenzene as an internal standard. Yields of isolated products are given in parenthesis. bReaction run at a 10 mmol scale. | |
To further exploit the ability of the 1/KOtBu system for the hydroboration of other common unsaturated bonds, we applied the standard catalytic conditions to acetophenone and styrene (Scheme 4). It was found that ketone could be readily hydroborated quantitively in one hour, while the hydroboration of styrene was regioselective, giving the branched and linear alkylboronate products (b/l = 10
:
1) in good total yield. Such regioselectivity has been previously reported using a structurally related 1-D Co(II) coordination polymer of L1.18b Finally, intermolecular competing experiments were carried out to further demonstrate chemoselective hydroboration. Thus, the hydroboration of phenylacetylene was conducted in the presence of equimolar acetophenone or styrene, respectively (Scheme 4). The results revealed that the ketone was selectively converted to the alkylboronate ester, while the alkyne remained intact. Phenylacetylene was the preferred substrate over styrene, in agreement with the results from the intramolecular competing hydroboration as seen in Scheme 3.
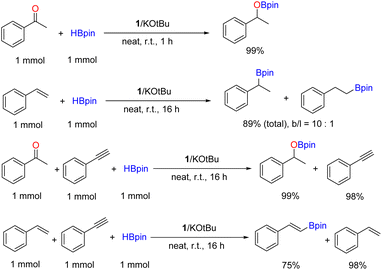 |
| Scheme 4 Hydroboration of ketone and styrene as well as chemoselective hydroboration catalysed by 1/KOtBu under standard conditions. Yields (and b/l ratio) were determined by GC analysis using hexamethylbenzene as an internal standard. | |
Since the catalytic reactions by 1 were heterogeneous, as the dark-brown solid could be fully recovered after the reaction by simply decanting the liquid and washing with THF (Fig. 3), we have performed recycling and reusing experiments to demonstrate its recyclability. Thus, 1 recovered from the standard hydroboration of phenylacetylene was reused for the next run by reloading the additive (1 mol%) and reactants, and the yield of product 3a was found to remain almost unchanged (80% vs. 82%). Meanwhile, the leaching of Fe from the MOF during the 1st run was found to be negligible as analysed by inductively coupled plasma optical emission spectrometry (ICP-OES). The same procedure was then applied to four more runs with recycled MOF catalyst, and the yield of 3a remained at the same level (ESI†). This indicates the ability of 1 as a facile and effective recyclable precatalyst for practical applications. The PXRD of a recycled sample of 1 reveals a similar, poor diffraction pattern to the one prior to catalytic reaction. The XPS analysis of fresh and recycled samples of 1 reveals identical oxidation states and binding modes of all elements. Interestingly, the BET surface area (13.04 m2 g−1) of recycled material of 1 is much higher than the unused sample, indicating a possible phase change during the catalytic conversion.
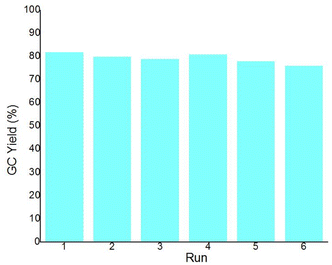 |
| Fig. 3 The bar graph results of recycling experiments for 1-catalysed hydroboration of phenylacetylene. | |
Experimental
General considerations
Unless specified otherwise, all reactions were carried out under a dry nitrogen atmosphere using standard glovebox and Schlenk techniques. Deuterated solvents were purchased from Cambridge Isotope Laboratories. Anhydrous grade solvents (stored over 4 Å molecular sieves) and alkyne substrates were purchased from Sigma-Aldrich, Fisher Scientific and TCI America. Pinacolborane was purchased from Alfa Aesar and used as received. FT-IR spectra were recorded on a Shimadzu 8400S instrument with solid samples under N2 using a Golden Gate ATR accessory. 1H NMR and 13C NMR spectra were obtained at room temperature on a Bruker AV 500 or 600 MHz NMR spectrometer, with chemical shifts (δ) referenced to the residual solvent signal. Inductively coupled plasma optical emission spectrometry (ICP-OES) analyses were conducted by Robertson Microlit Laboratories in the US. X-ray powder diffraction was conducted on a PANalytical X'Pert Pro Powder Diffractometer using a PIXcel1D detector at City College of New York. XPS analysis was conducted by MSE Supplies LLC in the US using Thermo Scientific ESCALAB 250Xi and Kratos AXIS-ULTRA DLD-600 W instruments. Measurement of BET surface area was performed by MSE Supplies LLC in the US using Quantachrome SI or Micromeritics ASAP 2460 facilities. GC-MS analysis was obtained using a Shimadzu GCMS-QP2010S gas chromatograph mass spectrometer. Elemental analyses were performed by Midwest Microlab LLC in Indianapolis in the US. L1 and L2 were prepared according to the literature.24
Synthesis of 1
A solution of ligand L1 (31.0 mg, 0.100 mmol) in MeOH/CH2Cl2 (10 mL, 1
:
3, v/v) was placed in a test tube. A blank solution of MeOH/CH2Cl2 (4 mL, 1
:
1, v/v) was layered on the top of the ligand solution, followed by a solution of FeCl2·4H2O (39.6 mg, 0.200 mmol) in MeOH (8 mL). The tube was sealed and allowed to stand at room temperature for about 2 weeks, during which time X-ray quality dark brown block-like crystals grew at the wall and bottom of the tube. The crystals were collected by decanting the solvent, washing with MeOH and then drying in vacuo. Yield: 40.0 mg (74% based on L1). FT-IR (solid, cm−1): 3389 br, 3062 w, 1600 s, 1481 w, 1410 s, 1361 m, 1286 m, 1246 w, 1158 m, 1028 s, 824 s, 786 s, 751 s, 732 m. Anal. calcd. for C80H56Cl14Fe7N16O2: C 44.47, H 2.61, N 10.37. Found C 45.05, H 2.86, N 10.45%. The co-crystallised solvents (MeOH and H2O) did not remain in the MOF for the elemental analysis.
Synthesis of 2
A solution of ligand L2 (31.0 mg, 0.100 mmol) in MeOH/PhCl (10 mL, 1
:
3, v/v) was placed in a test tube. A blank solution of MeOH/PhCl (4 mL, 1
:
1, v/v) was layered on the top of the ligand solution, followed by a solution of FeCl2·4H2O (39.6 mg, 0.200 mmol) in MeOH (8 mL). The tube was sealed and allowed to stand at room temperature for about 2 weeks, during which time X-ray quality brown plate-like crystals grew at the wall and bottom of the tube. The crystals were collected by decanting the solvent, washing with MeOH, and then drying in vacuo. Yield: 40.2 mg (65%). FT-IR (solid, cm−1) 3070 m, 1603 m, 1538 m, 1464 m, 1402 s, 1246 s, 1054 s, 1025 s, 843 s, 784 s, 749 s, 627 m. Anal. calcd. for C43H31.5Cl9.5Fe4N8O1.5: C 41.50, H 2.55, N 9.00. Found C 41.86, H 2.79, N 9.22%.
General procedure for catalytic hydroboration of alkynes
In a glovebox under N2 atmosphere, 1 (4.3 mg, 0.2 mol%) and potassium tert-butoxide (1.1 mg, 1 mol%) were placed in a 3.8 mL glass vial equipped with a stir bar, to which alkynes (1.0 mmol) and pinacolborane (1.2 mmol, 1.2 equiv.) were then added. The reaction mixture was allowed to stir at room temperature for 16 h. The reaction was exposed to the air and the crude reaction mixture was analyzed by GC-MS using hexamethylbenzene as an internal standard and then purified through column chromatography (SiO2) using ethyl acetate/hexane as an eluent. Selective alkenylboronate products were characterized by 1H and 13C NMR spectroscopies.
Gram-scale experiment
In a glovebox under N2 atmosphere, 1 (43 mg, 0.2 mol%) and potassium tert-butoxide (11 mg, 1 mol%) were placed in a 20 mL disposal vial equipped with a stir bar, to which phenylacetylene (1.02 g, 10 mmol) and pinacolborane (1.55 g, 12 mmol, 1.2 equiv.) were then added. The reaction mixture was allowed to stir at room temperature for 16 h. The crude product was subject to a flash column chromatography (silica gel) using ethyl acetate/hexane (1
:
10, v/v) as an eluent. 3a was isolated as a colorless oil which was confirmed by 1H and 13C NMR spectroscopies. Yield: 1.68 g (73%).
Procedure for recycling experiments
In a glovebox under N2 atmosphere, 1 (4.3 mg, 0.2 mol%) and potassium tert-butoxide (1.1 mg, 1 mol%) were placed in a 3.8 mL glass vial equipped with a stir bar, to which phenylacetylene (102 mg, 1.0 mmol) and pinacolborane (155 mg, 1.2 mmol, 1.2 equiv.) were then added. The reaction mixture was allowed to stir at room temperature for 16 h. Hexamethylbenzene (5 mg) was added to the mixture which was analyzed by using GC-MS to obtain the yield of 3a. Then the liquid was carefully removed and the dark brown solid was washed 3 times with THF. The organic extract (1 mL) was combined and analyzed by ICP-OES. The loss of iron from the precatalyst was determined to be 5 ppm, suggesting negligible leaching of Fe from the MOF. The solid that was recovered was then dried under reduced pressure and then placed in a small vial, to which additional KOtBu (1.1 mg) was added. Then phenylacetylene (102 mg, 1.0 mmol) and pinacolborane (155 mg, 1.2 mmol, 1.2 equiv.) were added. The resultant mixture was stirred at room temperature in air for 16 h and the product was analyzed by using GC analysis again. This procedure was repeated for 4 more cycles and the results of in total 6 runs are summarized in Fig. S1.†
X-ray crystallography
Suitable crystals of 1 and 2 were mounted on cryoloops with Paratone-N oil. X-ray diffraction data were collected on a Bruker X8 Kappa Apex II diffractometer using Mo Kα radiation. Crystal data, data collection and refinement parameters are summarized in Table S1.† The structures were solved using direct methods and standard difference map techniques, and were refined by full-matrix least-squares procedures on F2 with SHELXTL (Version 2018/3 for 1 and 2017/1 for 2).25 All hydrogen atoms bound to carbon were placed in calculated positions and refined with a riding model [Uiso(H) = 1.2–1.5Ueq(C)]. For 1, some hydrogen atoms bound to solvent oxygen atoms were located on the difference map, and others were placed in calculated positions. They were refined with a mixture of distance, angle, and riding restraints [Uiso(H) = 1.2Ueq(O)]. SQUEEZE/PLATON was used to treat additional solvent molecules as a diffuse contribution to the overall scattering.26 For 2, the hydrogen atom bound to nitrogen was located on the difference map and freely refined.
CCDC no. 2175907 and 2175908 contain the supplementary crystallographic data for this paper. Figures for crystal structures were drawn with the programs XP and Mercury v. 2.4. The crystallographic refinement data are listed in Table S1 (ESI).†
Conclusions
In summary, a novel, ionic Fe-MOF structure based on a linear ditopic tpy ligand was readily synthesized and structurally characterized. The 3-D MOF with large void channels has been assembled from a 2-D grid-like cationic network via π⋯π stacking interactions. This represents a rare example of robust Fe-based cationic MOF material. The bench-stable material was used as a precatalyst for the stereo- and regioselective hydroboration of alkynes under mild, solvent-free conditions, and the results were either comparable or superior to other molecular Fe catalysts for this reaction as we documented previously.9–16 A good substrate scope with functional group tolerance was demonstrated, along with a broader application to ketone and alkene hydroboration. The recyclability of the Fe-MOF precatalyst was demonstrated, highlighting its potential for practical catalytic applications.
Author contributions
G. Z. and S. Z. conceived and supervised the project. G. Z. performed experimental studies. M. C. N. conducted the X-ray crystallographic analysis. All authors analysed the data and wrote the article.
Conflicts of interest
There are no conflicts to declare.
Acknowledgements
We are grateful to the funding support by National Science Foundation for this work (CHE-1900500). We acknowledge the PSC-CUNY awards (63809-0051, 64254-0052 and 65203-0053) from City University of New York and a Seed Grant from the Office for Advancement of Research at John Jay College. Partial support from the American Chemical Society Petroleum Research Fund (#66150-UR1) is also acknowledged.
Notes and references
- S. Rej, A. Das and T. K. Panda, Overview of Regioselective and Stereoselective Catalytic Hydroboration of Alkynes, Adv. Synth. Catal., 2021, 363, 4818–4840 CrossRef CAS
. -
(a) K. Smith, A. Pelter and H. C. Brown, Borane Reagent, Academic Press, London, 1988 Search PubMed
;
(b) N. Miyaura and A. Suzuki, Palladium-Catalyzed Cross-Coupling Reactions of Organoboron Compounds, Chem. Rev., 1995, 95, 2457–2483 CrossRef CAS
;
(c) A. Suzuki, Cross-Coupling Reactions of Organoboranes: An Easy Way to Construct C-C Bonds, Angew. Chem., Int. Ed., 2011, 50, 6722–6737 CrossRef CAS PubMed
;
(d) J. Magano and J. R. Dunetz, Large-Scale Applications of Transition Metal-Catalyzed Couplings for the Synthesis of Pharmaceuticals, Chem. Rev., 2011, 111, 2177–2250 CrossRef CAS PubMed
. - D. Männig and H. Nöth, Catalytic Hydroboration with Rhodium Complexes, Angew. Chem., Int. Ed., 1985, 24, 878–879 CrossRef
. - For representative examples:
(a) M. Oestreich, E. Hartmann and M. Mewald, Activation of the Si–B Interelement Bond: Mechanism, Catalysis, and Synthesis, Chem. Rev., 2013, 113, 402–441 CrossRef CAS PubMed
;
(b) A. B. Cuenca, R. Shishido, H. Ito and E. Fernández, Transition-metal-free B–B and B–interelement reactions with organic molecules, Chem. Soc. Rev., 2017, 46, 415–430 RSC
;
(c) R. S. Anju, B. Mondal, K. Saha, S. Panja, B. Varghese and S. Ghosh, Chemistry of Diruthenium Analogue of Pentaborane(9) With Heterocumulenes: Toward Novel Trimetallic Cubane-Type Clusters, Chem.–Eur. J., 2015, 21, 11393–11400 CrossRef CAS PubMed
;
(d) C. Gunanathan, M. Holscher, F. F. Pan and W. Leitner, Ruthenium Catalyzed Hydroboration of Terminal Alkynes to Z-Vinylboronates, J. Am. Chem. Soc., 2012, 134, 14349–14352 CrossRef CAS PubMed
;
(e) B. Sundararaju and A. Furstner, A trans-Selective Hydroboration of Internal Alkynes, Angew. Chem., Int. Ed., 2013, 52, 14050–14054 CrossRef CAS PubMed
;
(f) J. Szyling, A. Franczyk, K. Stefanowska and J. Walkowiak, A recyclable Ru(CO)Cl(H)(PPh3)3/PEG catalytic system for regio- and stereoselective hydroboration of terminal and internal alkynes, Adv. Synth. Catal., 2018, 360, 2966–2974 CrossRef CAS
;
(g) A. Leyva, X. Zhang and A. Corma, Chemoselective hydroboration of alkynes vs. alkenes over gold catalysts, Chem. Commun., 2009, 4947–4949 RSC
;
(h) T. Ohmura, Y. Yamamoto and N. Miyaura, Rhodium- or Iridium-Catalyzed trans-Hydroboration of Terminal Alkynes, Giving (Z)-1-Alkenylboron Compounds, J. Am. Chem. Soc., 2000, 122, 4990–4991 CrossRef CAS
;
(i) Y. Lyu, N. Toriumi and N. Iwasawa, (Z)-Selective Hydroboration of Terminal Alkynes Catalyzed by a PSP–Pincer Rhodium Complex, Org. Lett., 2021, 23, 9262–9266 CrossRef CAS PubMed
;
(j) R. Mamidala, V. K. Pandey and A. Rit, AgSbF6-Catalyzed anti-Markovnikov hydroboration of terminal alkynes, Chem. Commun., 2019, 55, 989–992 RSC
;
(k) Q. Feng, H. Wu, X. Li, L. Song, L. W. Chung, Y. D. Wu and J. Sun, Ru-Catalyzed Geminal Hydroboration of Silyl Alkynes via a New gem-Addition Mechanism, J. Am. Chem. Soc., 2020, 142, 13867–13877 CrossRef CAS PubMed
. - For recent reviews, see:
(a) J. V. Obligacion and P. J. Chirik, Earth-Abundant Transition Metal Catalysts for Alkene Hydrosilylation and Hydroboration: Opportunities and Assessments, Nat. Rev. Chem., 2018, 2, 15–34 CrossRef CAS PubMed
;
(b) M. D. Greenhalgh, A. S. Jones and S. P. Thomas, Iron-Catalysed Hydrofunctionalisation of Alkenes and Alkynes, ChemCatChem, 2015, 7, 190–222 CrossRef CAS
;
(c) H. Wen, G. Liu and Z. Huang, Recent advances in tridentate iron and cobalt complexes for alkene and alkyne hydrofunctionalizations, Coord. Chem. Rev., 2019, 386, 138–153 CrossRef CAS
;
(d) S. R. Tamang and M. Findlater, Emergence and Applications
of Base Metals (Fe, Co, and Ni) in Hydroboration and Hydrosilylation, Molecules, 2021, 24, 3194 CrossRef PubMed
. - A. K. Jaladi, H. S. Choi and D. K. An, Catalyst-free and solvent-free hydroboration of alkynes, New J. Chem., 2020, 44, 13626–13632 RSC
. -
(a) J. Guo, Z. Cheng, J. Chen, X. Chen and Z. Lu, Iron- and Cobalt-Catalyzed Asymmetric Hydrofunctionalization of Alkenes and Alkynes, Acc. Chem. Res., 2021, 54, 2701–2716 CrossRef CAS PubMed
;
(b) M. Y. Hu, P. He, T. Z. Qiao, W. Sun, W. T. Li, J. Lian, J. H. Li and S. F. Zhu, Iron-Catalyzed Regiodivergent Alkyne Hydrosilylation, J. Am. Chem. Soc., 2020, 142, 16894–16902 CrossRef CAS PubMed
;
(c) D. Wei and C. Darcel, Iron-Catalyzed Hydrogen Transfer Reduction of Nitroarenes with Alcohols: Synthesis of Imines and Aza Heterocycles, J. Org. Chem., 2020, 85, 14298–14306 CrossRef CAS PubMed
. - D. Wei and C. Darcel, Iron Catalysis in Reduction and Hydrometalation Reactions, Chem. Rev., 2018, 119, 2550–2610 CrossRef PubMed
. - M. D. Greenhalgh and S. P. Thomas, Chemo-, regio-, and stereoselective iron-catalysed hydroboration of alkenes and alkynes, Chem. Commun., 2013, 49, 11230–11232 RSC
. - K. Nakajima, T. Kato and Y. Nishibayashi, Hydroboration of Alkynes Catalyzed by Pyrrolide-Based PNP Pincer-Iron Complexes, Org. Lett., 2017, 19, 4323–4326 CrossRef CAS PubMed
. - A. Singh, S. Shafiei-Haghighi, C. R. Smith, D. K. Unruh and M. Findlater, Hydroboration of Alkenes and Alkynes Employing Earth-Abundant Metal Catalysts, Asian J. Org. Chem., 2020, 9, 416–420 CrossRef CAS
. - F. Rami, F. Bächtle and B. Plietker, Hydroboration of internal alkynes catalyzed by FeH(CO)(NO)(PPh3)2: a case of boron-source controlled regioselectivity, Catal. Sci. Technol., 2020, 10, 1492–1497 RSC
. - M. Haberberger and S. Enthaler, Straightforward Iron-Catalyzed Synthesis of Vinylboronates by the Hydroboration of Alkynes, Chem.–Asian J., 2013, 8, 50–54 CrossRef CAS PubMed
. - V. S. Rawat and B. Sreedhar, Iron-Catalyzed Borylation Reactions of Alkynes: An Efficient Synthesis of E-Vinyl Boronates, Synlett, 2014, 25, 1132–1136 CrossRef
. - N. Gorgas, L. G. Alves, B. Stöger, A. M. Martins, L. F. Veiros and K. Kirchner, Stable, Yet Highly Reactive Nonclassical Iron(II) Polyhydride Pincer Complexes: Z-Selective Dimerization and Hydroboration of Terminal Alkynes, J. Am. Chem. Soc., 2017, 139, 8130–8133 CrossRef CAS PubMed
. - S. Garhwal, N. Fridman and G. de Ruiter, Z-Selective Alkyne Functionalization Catalyzed by a trans-Dihydride N-Heterocyclic Carbene (NHC) Iron Complex, Inorg. Chem., 2020, 59, 13817–13821 CrossRef CAS PubMed
. -
(a) G. Zhang, J. Wu, S. Zheng, M. C. Neary, J. Mao, M. Flores, R. J. Trovitch and P. A. Dub, Redox-noninnocent ligand-supported vanadium catalysts for the chemoselective reduction of C=X (X=O, N) functionalities, J. Am. Chem. Soc., 2019, 141, 15230–15239 CrossRef CAS PubMed
;
(b) G. Zhang, H. Zeng, S. Zheng, M. C. Neary and P. A. Dub, Vanadium-Catalyzed Stereo-and Regioselective Hydroboration of Alkynes to Vinyl Boronates, ACS Catal., 2022, 12, 5425–5429 CrossRef CAS
;
(c) G. Zhang, H. Zeng, J. Wu, Z. Yin, S. Zheng and J. C. Fettinger, Highly Selective Hydroboration of Alkenes, Ketones and Aldehydes Catalyzed by a Well-Defined Manganese Complex, Angew. Chem., Int. Ed., 2016, 55, 14369–14372 CrossRef CAS PubMed
;
(d) G. Zhang, J. Wu, H. Zeng, M. C. Neary, M. Devany, S. Zheng and P. A. Dub, Dearomatization and Functionalization of Terpyridine Ligands Leading to Unprecedented Zwitterionic Meisenheimer Aluminum Complexes and Their Use in Catalytic Hydroboration, ACS Catal., 2019, 9, 874–884 CrossRef CAS
. -
(a) J. Wu, H. S. Zeng, J. Cheng, S. P. Zheng, J. A. Golen, D. R. Manke and G. Zhang, Cobalt (II) coordination polymer as a precatalyst for selective hydroboration of aldehydes, ketones, and imines, J. Org. Chem., 2018, 83, 9442–9448 CrossRef CAS PubMed
;
(b) G. Zhang, J. Wu, S. Li, S. Cass and S. Zheng, Markovnikov-Selective Hydroboration of Vinylarenes Catalyzed by a Cobalt (II) Coordination Polymer, Org. Lett., 2018, 20, 7893–7897 CrossRef CAS PubMed
;
(c) G. Zhang, S. Li, J. Wu, H. Zeng, Z. Mo, K. Davis and S. Zheng, Highly efficient and selective hydroboration of terminal and internal alkynes catalysed by a cobalt (ii) coordination polymer, Org. Chem. Front., 2019, 6, 3228–3233 RSC
;
(d) G. Zhang, H. Zeng, S. Li, J. Johnson, Z. Mo, M. C. Neary and S. Zheng, 1-D manganese(II)-terpyridine coordination polymers as precatalysts for hydrofunctionalisation of carbonyl compounds, Dalton Trans., 2020, 49, 2610–2615 RSC
. - We reported previously a 2-D Fe-MOF using divergent 4,2′:6′,4′′-tpy for hydroboration of carbonyl compounds, see:
(a) G. Zhang, J. Cheng, K. Davis, M. G. Bonifacio and C. Zajaczkowski, Practical and selective hydroboration of aldehydes and ketones in air catalysed by an iron(II) coordination polymer, Green Chem., 2019, 21, 1114–1121 RSC
;
(b) L. Li, E. Liu, J. Cheng and G. Zhang, Iron (II) coordination polymer catalysed hydroboration of ketones, Dalton Trans., 2018, 47, 9579–9584 RSC
. -
(a) J. E. Beves, E. L. Dunphy, E. C. Constable, C. E. Housecroft, C. J. Kepert, M. Newburger, D. J. Price and S. Schaffner, Vectorial property dependence in bis{4′-(n-pyridyl)-2,2′:6′,2′′-terpyridine}iron(ii) and ruthenium(ii) complexes with n = 2, 3 and 4, Dalton Trans., 2008, 386–396 RSC
;
(b) J. E. Beves, E. C. Constable, S. Decurtins, E. L. Dunphy, C. E. Housecroft, T. D. Keene, M. Neuburger, S. Schaffner and J. A. Zampese, Structural diversity in the reactions of 4′-(pyridyl)-2,2′:6′,2′′-terpyridine ligands and bis{4′-(4-pyridyl)-2,2′:6′,2′′-terpyridine}iron(II) with copper(II) salts, CrystEngComm, 2009, 11, 2406–2416 RSC
. - The formation of Cl3FeOFeCl3 is well-known and has been previously discussed: S. Ameerunisha, J. Schneider, T. Meyer, P. S. Zacharias, E. Bill and G. Henkel, Synthesis and structural characterisation of Fe(II) and Cu(I) complexes of a new tetrafunctional N-donor ligand with dodecahedral or tetrahedral binding domains, Chem. Commun., 2000, 2155–2156 RSC
. - A. Karmakar, A. V. Desai and S. K. Ghosh, Ionic metal-organic frameworks (iMOFs): Design principles and applications, Coord. Chem. Rev., 2016, 307, 313–341 CrossRef CAS
. - Q. Hu, J. Yu, M. Liu, A. Liu, Z. Dou and Y. Yang, A Low Cytotoxic Cationic Metal–Organic Framework Carrier for Controllable Drug Release, J. Med. Chem., 2014, 57, 5679–5685 CrossRef CAS PubMed
. - J. Wang and G. S. Hanan, A Facile Route to Sterically Hindered and Non-Hindered 4′-Aryl-2,2′:6′,2′′-Terpyridines, Synlett, 2005, 8, 1251–1254 Search PubMed
. -
(a) G. M. Sheldrick, SHELXTL, An Integrated System for Solving, Refining, and Displaying Crystal Structures from Diffraction Data, University of Göttingen, Göttingen, Federal Republic of Germany, 1981 Search PubMed
;
(b) G. M. Sheldrick, SHELXT-Integrated Space-Group and Crystal-Structure Determination, Acta Crystallogr., Sect. A: Found. Adv., 2015, 71, 3–8 CrossRef PubMed
. -
(a) A. L. Spek, PLATON, A Multipurpose Crystallographic Tool, Utrecht University, Utrecht, The Netherlands, 2005 Search PubMed
;
(b) A. L. Spek, Single-crystal structure validation with the program PLATON, J. Appl. Crystallogr., 2003, 36, 7–13 CrossRef CAS
.
|
This journal is © The Royal Society of Chemistry 2023 |