DOI:
10.1039/D2RA07587C
(Paper)
RSC Adv., 2023,
13, 3743-3758
Improvements in piezoelectric and energy harvesting properties with a slight change in depolarization temperature in modified BNKT ceramics by a simple technique†
Received
29th November 2022
, Accepted 12th January 2023
First published on 26th January 2023
Abstract
For many BNT-based ceramics, an attempt to increase the piezoelectric properties usually results in a decrease in depolarization temperature (Td). This trend limits the applications of the materials. Many previous experiments have used different methods to enhance the piezoelectric properties and improve the Td characteristic. In this study, we demonstrated a simple technique (thermal annealing) to enhance the piezoelectric properties with a very slight decrease in Td by ∼2 °C for a modified BNKT ceramic (BNKT doped with ZnO). Other phase transition characteristic temperatures of the studied ceramics were also slightly changed. The optimum dielectric (εr = 651, tan
δ = 0.0503, TF–R = 167.38 °C, Tm = 305.41 °C, εmax = 5551, TB = 367.15 °C, Td = 155.98 °C, and γ = 1.43), ferroelectric (Pmax = 41.28 μC cm−2, Pr = 35.85 μC cm−2, Ec = 42.60 kV cm−1 and Rsq = 1.42), piezoelectric (d33 = 198 pC N−1, kp = 0.598, and g33 = 34.35 × 10−3 Vm N−1), and energy harvesting (FoM = 6.80 pm2 N−1) were obtained for the 8 h annealed ceramic. Furthermore, higher energy harvesting properties (which were 32% higher than that of the unannealed ceramic) were obtained after employing this technique.
1 Introduction
Piezoelectric materials have been extensively designed and applied in many industrial fields, for example in such items as sensors, micro-displacement devices, brakes, sensitive materials, and other components, due to their ability to cause a mutual conversion between mechanical and electrical energy.1 The development of piezoelectric materials with high energy conversion efficiency is of decisive importance for the requirements of advanced energy harvesting (EH) devices toward miniaturization and integration.2 Lead titanate zirconate (PZT) ceramics are the most widely used energy harvesting materials due to their excellent piezoelectric properties,3 i.e., high mechanical quality factor (Qm), high piezoelectric constant (d33), and large planar electromechanical coupling factor (kp).4 However, lead-based compounds pose a serious environmental threat, and, therefore, efforts have been made to look for cleaner alternatives.1,3
Recent reports have identified many possible lead-free candidates based on barium titanate (BaTiO3, BT), potassium sodium niobates (K0.5Na0.5NbO3, KNN), bismuth sodium titanates (Bi0.5Na0.5TiO3, BNT), bismuth potassium titanates (Bi0.5K0.5TiO3, BKT), and bismuth layered ferroelectrics.2,5 Among them, BNT has been found to be a possible energy storage material due to its high spontaneous polarization (Pm) exceeding 40 μC cm−2, which results from the hybridization of the Bi 6p and O 2p orbitals.6 Nevertheless, a pure BNT ceramic is known to be to be sintered with difficulty, and it also has other issues, such as beginning polarized with just as much complication, not to mention its having a high coercive field (Ec), high conductivity, and poor temperature stability.7 Therefore, there is an urgent need for researchers to approach to improve the performance of the BNT ceramic. Numerous approaches, such as the establishment of the morphotropic phase boundaries (MPB), acceptor/donor dopants, compositional tuning, and improvement of the fabrication process conditions, have been proposed for the property optimization of the BNT piezoelectric materials.8 Besides BNT, bismuth sodium potassium titanate or Bi0.5(Na1−xKx)0.5TiO3 (BNKT) lead-free piezoelectric ceramic is also a good candidate for the replacement of toxic lead compounds for energy harvesting applications. For the Bi0.5(Na1−xKx)0.5TiO3 solid solution, Na+ is partially substituted by K+ on the A-site of BNT,2 and it is known that better piezoelectric and/or strain properties at the MPB region between BNT rhombohedral and BKT tetragonal phases near x = 0.16–0.20 can be achieved.9–11 The dielectric constant of the BNKT ceramic was reported to be 1030.10 The good piezoelectric coefficients (d33 = 151 pC N−1, d31 = 46.9 pC N−1) were obtained at the optimal composition of x = 0.20.10,12
It is known that the energy harvesting performances of piezoelectric materials are closely related to their piezoelectric coefficient (d33 α εPr).13 Thus, a higher remanent polarization (Pr) is desirable for achieving better performances of piezoelectric energy harvesting devices.14 In order to improve the ferroelectric and piezoelectric properties of the BNKT ceramic to achieve excellent energy harvesting devices, it has been modified with other materials, such as ZnO. The ZnO particle has been widely used as a sintering aid in many dielectric and piezoelectric ceramics.15 As demonstrated in many studies, the addition of ZnO could improve densification for a wide range of dielectric and ferroelectric materials.15–18 For example, Promsawat et al.15 reported that the ZnO additive helped to facilitate the densification process and thereby improved the electrical properties of the PMNT ceramics. Lee et al.16 found that the addition of ZnO improved the piezoelectric properties of the Bi0.5Na0.5TiO3 (BNT) ceramics. The effect of ZnO addition on the electrical properties of the (K0.44Na0.52Li0.04)(Nb0.86Ta0.10Sb0.04)O3 or KNL–NTS ceramics was investigated by Marcos et al.17 They also reported that ZnO doping produced an increase in the ferroelectric performance of the KNL–NTS ceramics. Zhang et al.18 also found that the addition of ZnO could enhance both the ferroelectric and piezoelectric properties of the 0.94Bi0.5Na0.5TiO3–0.06BaTiO3 or BNT–6BT ceramics. The maximum Pr and low-field d33 values were observed for the BNT–6BT:0.04ZnO ceramics.
For piezoelectric applications, the depolarization temperature (Td) of BNT-based ceramics is an important factor.18,19 Many authors have suggested that piezoceramics with a high Td can be used in a wider range of temperatures because they are more stable. Therefore, several authors have focused on variables that relate to or influence Td.20,21 Numerous methods, including doping, the formation of solid solutions, the formation of composites, and the modulation of crystallographic orientation in texturing ceramics, have been found to affect the Td value.22–28 However, it should be noted that an effort to raise Td often leads to a drop in the value of the piezoelectric d33. This tendency restricts the use of the materials in many applications. Therefore, the work to improve the Td and piezoelectric characteristics of materials based on BNT is still challenging and worthy of continuing research.
It has been proposed that annealing gives several advantages for ferroelectric and piezoelectric characteristics, including those of lead-based piezoelectric ceramics and, more recently, lead-free piezoelectric ceramics.29,30 Xu et al.31 reported that annealing treatment improved the piezoelectric properties of the 0.95(Bi0.5Na0.5)0.97(Li0.5Nd0.5)0.03TiO3–0.05BaTiO3 or BNTLN0.03–BT5 ceramics. It also induced subtle structural changes. The microstructures of annealed samples become homogeneous, which leads to the disappearance of the Maxwell–Wagner relaxor. The optimal piezoelectric properties of d33 = 148 pC N−1 and kp = 0.33 have been obtained by annealing at 900 °C. Wang et al.32 also reported that good ferroelectric and piezoelectric properties were obtained in the Na0.5K0.5NbO3 ceramics after annealing at 900 °C for 4 h dwell time in an air atmosphere. Since annealing has several benefits for the ferroelectric characteristics, it is anticipated that this technique should have a positive effect on the Td and other properties such as piezoelectric and energy harvesting properties. In the present investigation, the annealing process was then utilized for the modified BNKT ceramics (BNKT added with ZnO). The effect of thermal annealing on phase evolution, microstructural, dielectric, Td, ferroelectric, piezoelectric, and energy harvesting properties was investigated and discussed in detail.
2 Experimental
The lead-free ceramic with the composition Bi0.5(Na0.84K0.16)0.5TiO3 or BNKT was synthesized by a solid-state reaction method. The starting materials used in this study were the reagent-grade powders of Bi2O3, Na2CO3, K2CO3, TiO2, and ZnO nanoparticles. All carbonate powders were first dried at 120 °C for 24 h to remove any moisture. The carbonate and high-purity oxide starting materials were weighed based on a stoichiometric formula of Bi0.5(Na0.84K0.16)0.5TiO3 and then mixed and ball milled in a 99.9% ethanol solution for 24 h. The slurries were dried in an oven for 24 h at a temperature of 120 °C. Dried BNKT powder was calcined in a closed alumina crucible at 900 °C for 2 h with a heating/cooling rate of 5 °C min−1. After calcination, the ZnO nanoparticles (less than 100 nm) were added into the calcined BNKT powder and then milled again in ethanol for 24 h with the formula of Bi0.5(Na0.84K0.16)0.5TiO3/0.01ZnO referred to as the BNKT/0.01ZnO. After drying and sieving, the mixed powders were granulated by adding a few drops of 4 wt% PVA as a binder and then pressed into disks with a diameter of 10 mm and a thickness of about 1.3 mm. The pellets were then preheated in the air at 500 °C for 1 h to remove the organic binder and then sintered at 1125 °C for 2 h dwell time with a heating/cooling rate of 5 °C min−1 in closed alumina crucibles. To minimize the loss of the volatile elements, the green pellets were embedded in a powder of the same composition. In order to further improve the quality of the ceramics, the sintered ceramics were annealed at 950 °C with a heating/cooling rate of 5 °C min−1 and various annealing times of 0, 4, 8, and 12 h in an air atmosphere, respectively.
The bulk density of all ceramics was determined using Archimedes' method. An X-ray diffractometer (XRD, PANalytical, X′ Pert ProMPD) was used to identify the phase of all sintered ceramics. The Raman spectra were carried out on the polished sintered pellets and recorded by Raman spectroscopy (T6400 JY, Horiba Jobin Yvon). The surface morphologies of the sintered ceramics were observed using a field-emission scanning electron microscope (FE-SEM, JEOL JSM-6335F). The average grain size of each sample was determined by a mean linear interception method from the SEM micrographs. For electrical measurements, all samples were polished to 1 mm thickness, and high-temperature silver paste was fired on both sides at 700 °C for 30 min in order to form the electrodes. Temperature dependence of dielectric properties was also carried out using a 4192A LCR-meter connected to a high-temperature furnace from room temperature (RT) to 500 °C with various frequency ranges of 1–1000 kHz. A radiant precision ferroelectric tester was used to investigate the ferroelectric properties at both room temperature (RT) and high temperatures (HT) of 25–150 °C. Various AC electric fields of 30–65 kV cm−1 at a frequency of 0.1 Hz were utilized in the hysteresis measurement. For piezoelectric characterization, the samples were poled in silicone oil under a DC electric field of 5 kV mm−1 for 15 min at RT. The piezoelectric coefficient (d33) was measured after aging the ceramics for at least 24 h using a d33 meter (KCF technologies, S5865) at a frequency of 50 Hz. The planar electromechanical coupling factor (kp) was measured by the resonance and anti-resonance technique using an impedance analyzer (HP 4284A). The piezoelectric voltage constant (g33) and the off-resonance figure of merit (FoM) for energy harvesting were also calculated in this study.
3 Results and discussion
3.1 Densification and phase evolution
In this experiment, the density as a function of annealing times (0–12 h) of the BNKT/0.01ZnO ceramics was determined, and the values are listed in Table 1. The density increased from 5.81 g cm−3 for the unannealed ceramic to the maximum value of 5.86 g cm−3 for the 8 h annealed ceramic, and then slightly decreased to 5.84 g cm−3 for the 12 h annealed ceramic. The annealing process and ZnO additives can help to improve the densification of all ceramics. However, the slightly lower density value after 12 h of annealing may be caused by the high volatilization of some components for overtime annealing,32 such as Bi and K. The high-density sample was responsible for the improved electrical characteristics,29,33 as shown by the results in the next section.
Table 1 Physical, microstructure and mechanical properties of the BNKT/0.01ZnO ceramics annealed at 950 °C with different annealing times
Annealing time (h) |
Density (g cm−3) |
Phase |
c/a |
c/a − 1 |
FWHM of (110) (°) |
Crystallite size (nm) |
Average grain size (μm) |
0 |
5.81 |
R + T |
1.0037 |
0.0037 |
0.66 |
12.54 |
1.44 ± 0.21 |
4 |
5.82 |
R + T |
1.0049 |
0.0049 |
0.60 |
13.80 |
1.59 ± 0.25 |
8 |
5.86 |
R + T |
1.0066 |
0.0066 |
0.57 |
14.52 |
1.98 ± 0.23 |
12 |
5.84 |
R + T |
1.0090 |
0.0090 |
0.55 |
15.05 |
2.28 ± 0.19 |
In order to check the phase-purity and structural parameters, all ceramics were investigated by the X-ray diffraction technique at RT,33 and the XRD pattern of the BNKT/0.01ZnO ceramics annealed at 950 °C with different annealing times, where 2θ = 20–70° is shown in Fig. 1(a). It is noted that all annealed ceramics exhibited a single perovskite structure without any detectable impurity peaks, indicating that ZnO had diffused into the BNKT lattice to form a complete solid solution. The results of a more detailed XRD analysis performed in a narrow range of 2θ = 39–41° and 2θ = 44–48° are shown in Fig. 1(b) and (c), respectively. Based on peak profile fitting, the unannealed ceramic exhibited the morphotropic phase boundary (MPB) structure, consisting of rhombohedral and tetragonal phases.
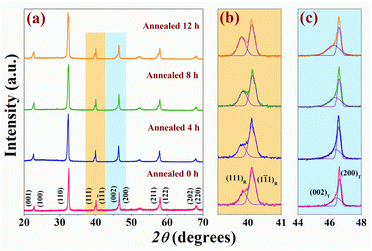 |
| Fig. 1 X-ray diffraction patterns of the BNKT/0.01ZnO ceramics annealed at 950 °C with different annealing times, where (a) 2θ = 20–70° (b) 2θ = 39–41°, and (c) 2θ = 44–48°. | |
This was evidenced by a slight splitting of (111)R/(1
1)R rhombohedral peaks at 2θ ∼ 40° and (002)T/(200)T tetragonal peaks at 2θ ∼ 46.5°. This result is also consistent with the work done by Sasaki et al.10 on the (1 − y)BNT–yBKT ceramics, who found a relatively broad MPB region in the range of y = 0.16–0.20 between BNT rhombohedral and BKT tetragonal ceramics. This result also agrees with the previous experiments.12,34,35 However, the weak splitting of the (200) peak indicates the rhombohedral rich phase. It should be noted that with a further increase in the annealing time up to 8–12 h, XRD displayed more splitting of (111)R/(1
1)R rhombohedral peaks and (002)T/(200)T tetragonal peaks. The phase transition from the mixed rhombohedral–tetragonal to a more tetragonal phase portion was observed when the annealing time was increased. To check this, the c/a − 1 value (where c/a is tetragonally) was calculated (Table 1). The c/a − 1 value increased as the annealing time increased, confirming the increase in the tetragonal phase.
The full width at half maximum (FWHM) values using a certain XRD peak for all ceramics were determined in this investigation.36,37 In the present work, the FWHM value was calculated from the (110) reflection main peak in the X-ray diffraction pattern, and the values are also included in Table 1. The FWHM of the (110) peaks of the annealed ceramics (4–12 h) was much smaller than that of the unannealed ceramics (0 h), suggesting that annealing improved the degree of crystallinity of the samples. The crystallite size (D) was also used to check the degree of crystallinity of the ceramics. In the current work, the X-ray peak broadening (110) reflection was selected for this proposed, and the calculation was performed based on the Debye–Scherrer formula:37
|
 | (1) |
where
λ is taken to be 0.15418 nm as the X-ray source is Cu-Kα,
B is the full width at half maximum (FWHM),
k is a fixed number 0.9, and
θ is the Bragg's angle.
38–43 It can be seen that crystallite size increased from 12.54 nm for the unannealed ceramic to the maximum value of 15.05 nm for the 12 h annealed ceramic. With increasing annealing time, the FWHM of the (110) peak became narrower and the crystallite size increased, indicating an improvement in the degree of crystallinity of the annealed ceramics.
40 Furthermore, the lower FWHM value (with increasing annealing time) also indicated a better homogeneous chemical distribution in the ceramics.
44,45 In addition, it was proposed that the increase in crystallite size could be accompanied by an improvement in the ceramic's electrical properties.
41,43
To further analyze the evolution of the crystal structure with varying annealing times, Raman spectra were measured in the range of 100–1200 cm−1, which are now presented in Fig. 2. Obviously, four main regions could be detected in the spectrum at room temperature (RT), which is nearly identical to those previously reported data of BNT-based ceramics:46–50 (1) the mode located below 200 cm−1 associated with the vibration of perovskite A-site, (2) the around 300 cm−1 modes should be related to Ti–O vibrations, (3) the modes approximately 450–650 cm−1 related to TiO6-octahedra vibration, and (4) the modes over 700 cm−1 related to the A1 (longitudinal optical) and E (longitudinal optical) overlapping bands.47 The Raman peaks at the mode located below 200 cm−1 slightly shifted towards the lower wavenumber for the 8–12 h ceramics. This may be linked with the volatilization of A-site ions for a longer annealing time. The broader and splitting peak of the mode around 300 cm−1 and 450–650 cm−1 was observed with increasing annealing time which corresponds to a higher degree of tetragonally. This result agrees well with previous studies.46,48 Overall, spectra recorded for the studied ceramics are in good agreement with that of the previously reported for other BNT-based ceramics which had a coexisted phase structure between rhombohedral (R3c) and tetragonal (P4bm) phases.51 This Raman result also agrees well with the XRD analysis.
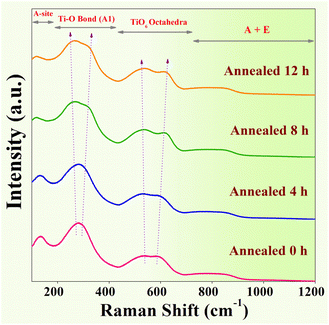 |
| Fig. 2 Raman spectra at room temperature of the BNKT/0.01ZnO ceramics annealed at 950 °C with different annealing times. | |
3.2 Microstructure analysis
Microstructural development during the annealing process was investigated by scanning electron microscopy (SEM). The FE-SEM micrographs of sintered surfaces of the BNKT/0.01ZnO ceramics annealed at 950 °C with different annealing times are shown in Fig. 3. The average grain size of each sample is given in Table 1. It is evident that all ceramics were very dense, smooth, and crack free. The grain was well-crystallized with clear grain boundaries and contained a cubic-like shape. The SEM micrographs clearly showed that the annealing time had an influence on the microstructure of the BNKT/0.01ZnO ceramics. The average grain size increased with increasing annealing time. The average grain size of the unannealed ceramic was found to be 1.44 ± 0.21 μm. The average grain size initially increased with the annealing time and reached a maximum value of 2.28 ± 0.19 μm for the 12 h annealed ceramic. Similar behavior was also reported in a recent investigation.52 As the annealing time increased, the grains grew and the average grain size increased, whereas the number and size of the pores decreased. In the current study, a line intercept method was used to find out the average grain size. It should be noted that the percentage increase in grain size for this study is 44% after 12 h of annealing. Fig. 4(a) displays plots of FWHM versus crystallite size and grain size. It was found that the crystallite size had a linearly decreasing trend with FWHM, while grain size had an exponential decay with FWHM. A relation between crystallite size and grain size is also presented in the inset of Fig. 4(a). As crystallite size increased, grain size increased exponentially. Therefore, the obtained data indicated that annealing enhanced both crystallite size and grain size. In the present experiment, the grain size increased exponentially with increasing annealing time, as seen in Fig. 4(b). Normal grain growth can be explained by the phenomenological kinetic grain growth equation, which is written as:4 |
 | (2) |
where G is the average grain size at the time t, n is the kinetic grain growth exponent, K0 is the constant, Q is the apparent activation energy, R is the gas constant, and T is the absolute temperature. It was noted from the equation that the average grain size was an exponential function of the annealing time (t) (Fig. 4(b)). To compare the experimental result with the kinetic equation better, log
G is plotted versus log
t, and there generally exists a reasonably good linear relationship between log
G and log
t (Fig. 4(c)), which is consistent with eqn (2).4 Based on Fig. 4(b), a reasonably good linear relationship between log
G and log
t was observed, where the slope is 1/n. Generally, a small n value indicates a large grain growth rate, which can be shown by differentiating eqn (2). The n value for this study was found to be 3.05. Therefore, the small n value calculation indicated that the annealing produced a moderate grain growth rate53,54 with increasing annealing time. The relationships between the grain size and the electrical properties (i.e., dielectric, ferroelectric, and piezoelectric) will be discussed in the next sections. As is known, grain size has a significant influence on the dielectric characteristics and polarization of piezoelectric materials.9,55
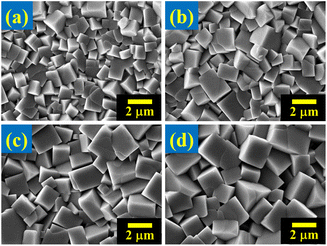 |
| Fig. 3 The FE-SEM micrographs with as sintered surfaces of the BNKT/0.01ZnO ceramics annealed at 950 °C with different annealing times, where (a) 0 h, (b) 4 h, (c) 8 h, and (d) 12 h. | |
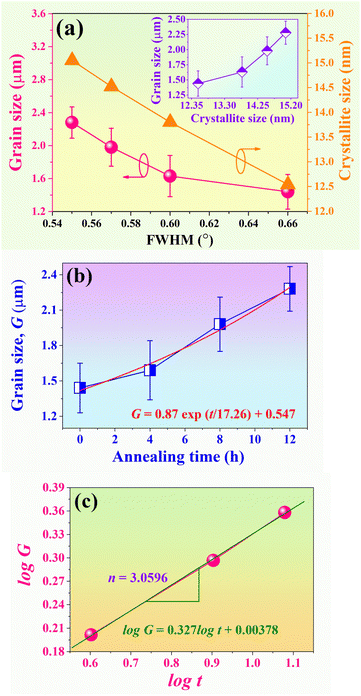 |
| Fig. 4 Plots of (a) FWHM versus grain size and crystallite size (inset shows a relation between grain size and crystallite size), (b) grain size as a function of annealing time, and (c) log G as a function of log t. | |
3.3 Dielectric properties
The room temperature dielectric constant (εr) and dielectric loss (tan
δ) at a frequency of 1 kHz are listed in Table 2. The εr of the un-annealed ceramic was found to be 501 with tan
δ of 0.0425. The εr initially increased with the annealing time and reached a maximum value of 651 for the 8 h annealed ceramic, and then slightly decreased to 529 for the 12 h annealed ceramic. The density is believed to be an important factor in the higher electrical properties of the samples,33 i.e., higher density resulted in higher dielectric properties.
Table 2 Dielectric properties obtained at HT (25–500 °C) and a frequency of 1 kHz of the BNKT/0.01ZnO ceramics annealed at 950 °C with different annealing times
Annealing time (h) |
εr |
tan δa |
TF–R (°C) |
Tm (°C) |
εmax |
tan δb |
TB (°C) |
ΔT = TB − Tm (°C) |
γ |
Td (°C) |
Dielectric loss (tan δ) measured at T = RT and a frequency of 1 kHz. Dielectric loss (tan δ) measured at T = Tm and a frequency of 1 kHz. |
0 |
501 |
0.0425 |
168.25 |
307.18 |
4836 |
0.0334 |
369.44 |
62.26 |
1.48 |
157.22 |
4 |
536 |
0.0471 |
167.60 |
306.20 |
5437 |
0.0363 |
368.33 |
62.13 |
1.46 |
156.17 |
8 |
651 |
0.0503 |
167.38 |
305.41 |
5551 |
0.0387 |
367.15 |
61.74 |
1.43 |
155.98 |
12 |
529 |
0.0443 |
166.41 |
305.24 |
5501 |
0.0384 |
366.65 |
61.41 |
1.39 |
155.89 |
Temperature dependence on the dielectric constant (εr) and dielectric loss (tan
δ) of the poled BNKT/0.01ZnO ceramics annealed at 950 °C with different annealing times, and measurements at various frequencies from 1–1000 kHz are shown in Fig. 5. The related dielectric properties are also summarized in Table 2. There were two dielectric anomalous peaks in the εr–T plot. The first dielectric anomaly peak (shoulder peak), characterized by a large dielectric peak and noticeable frequency dispersion, was noted at a temperature below 200 °C. This temperature corresponds to Ts, indicating the relaxor ferroelectrics which are usually caused by the thermal evolutions of discrete polar nanoregions.6 The secondary anomaly broadened peak, at Tm, indicates the maximum dielectric constant (εm) in εr vs. T plots. The two dielectric anomalous peaks on the dielectric permittivity curves have been commonly reported in BNT-based systems.56–58 Based on Fig. 6(a), the unannealed ceramic had the εmax of 4836. The εmax increased with the annealing time and reached a maximum value of 5551 for the 8 h annealed ceramic. At temperature ≥150 °C, there was a dispersion in tan
δ value with frequency. However, the degree of dispersion was lower than that reported in other lead-free materials such as the (Bi0.5Na0.5)TiO3–BaTiO3 system.33 From Fig. 5, the tan
δ value at Tm for all ceramics was in the range 0.03–0.04, which is acceptable for a characteristic of capacitors at a high temperature.
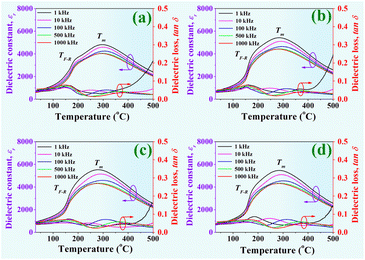 |
| Fig. 5 Temperature dependence on dielectric constant (εr) and dielectric loss (tan δ) of the poled BNKT/0.01ZnO ceramics annealed at 950 °C with different annealing times, measurement at various frequencies from 1–1000 kHz, where (a) 0 h, (b) 4 h, (c) 8 h, and (d) 12 h. | |
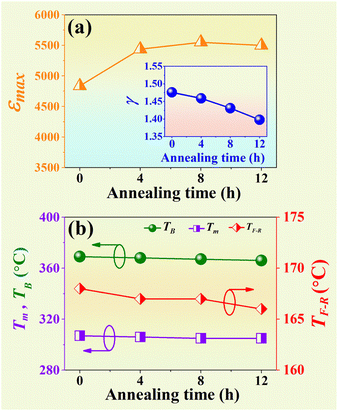 |
| Fig. 6 Plot of (a) εmax as a function of annealing time (inset: the γ value as various annealing times), and (b) Tm, TB, and TF–R values as a function of annealing time. | |
The reasons for the observed increase in the dielectric characteristics (by annealing) are complex due to the possibility of it being affected by many factors. Xia and Yao59 suggested that the observed increases in dielectric characteristics for Pb(Zn1/3Nb2/3)O3 (by annealing) are mostly attributable to many factors: (1) grain size change, (2) density change, (3) internal stress release, (4) a change in the content of the secondary phase, (5) the grain boundary layer, (6) the defect, (7) domain wall motion, and (8) a change in the order degree of B-site ions.59 However, it should be noted that the effect of grain size can be linked to a variety of factors, such as domain wall motion, internal stress release, and grain boundary layer characteristics. For the grain size effect, Mudinepalli et al.60 investigated the properties of BT based ceramics (Ba0.8Sr0.2TiO3) and proposed that the dielectric constant is affected by domain population and domain wall mobility. They also proposed that a uniform grain size (big grains) can reduce internal stress, making it easy and regular for the domain walls to move, which raises the dielectric constant.60 Zhao et al.61 reported that the critical grain size for the disappearance of ferroelectricity in BT ceramics is 10–30 nm. Furthermore, the strong broadening in the permittivity curve of their fine grain ceramics was explained by the size effect and the effect of a low-permittivity non-ferroelectric layer at the grain boundary. These effects depend on the grain size.15,61
It is known that piezoceramics with their composition near the MPB composition often present high electrical properties such as high dielectric and piezoelectric properties. This is due to the formation of a mixture phase such as that of the rhombohedral and tetragonal phases which have a larger number of reorientable polarization directions. Therefore, the improvement in the dielectric constant of the 8 h annealed ceramic in the present study may be attributed to the formation of the mixture phase between rhombohedral and tetragonal with an optimal fraction that has better optimal properties. Furthermore, the increase in grain size, degree of composition homogeneity, and density also contributed to the improvement. In addition, it should be noted that the studied was added with BT, and BT ceramics need a high sintering temperature (as compared to BNT ceramics) to achieve the best combination of density and properties. Thus, annealing can help optimize the properties of the ceramics. However, the change in the amount of the secondary phase has less effect since the studied ceramics presented no secondary phase. For the ceramics annealed at 12 h, the εmax slightly decreased to 5501. This result may be linked to the volatilization of some components for a longer annealing time (i.e., Bi and K).30,32 This can be linked with a slight decrease in density and a slight decrease in the dielectric constant of this ceramic.
3.4 Phase transition behavior
For phase transition temperatures, the Tm value was corrected from the dielectric data as presented in the previous section. The Tm value slightly shifted down by ∼2 °C with increasing annealing time up to 12 h (Fig. 6(b) and Table 2). The Burn's temperature (TB) value was also evaluated for the current investigation. Normally, at high temperatures, relaxors exist in a nonpolar paraelectric phase analogous to the ferroelectric paraelectric phase. However, as the temperature drops below the Burns temperature (TB), they undergo a transformation into an ergodic relaxor (ER) state. This temperature indicates the start of the polarization process in relaxors.62 To determine the TB, there are many techniques, such as the refractive index,62,63 electrostrictive strain,64 or deviation from the Curie–Weiss behavior.62 In the present investigation, the TB was collected from the dielectric constant that starts to deviate from the Curie–Weiss law.65 The plot of the inverse dielectric permittivity (1/εr) as a function of temperature at 1 kHz that was used to determine the TB values is shown in the supplementary information in Fig. S1.† Based on Fig. 6(b) and Table 2, the TB also slightly decreased from 369 °C for the unannealed ceramic to 366 °C for the 12 h annealed ceramic (i.e. a decrease by 3 °C). In addition, the deviation from the Curie–Weiss law can be depicted by ΔT (degree of deviation) as follows: ΔT = TB − Tm.66 The calculated values of ΔT as a function of annealing time are also listed in Table 2. The ΔT values were 62 to 61 °C, which indicated that the degree of diffuse phase transition is moderate. For 12 h annealing, the ΔT decreased by 1 °C, implying that the annealing time had less effect on the degree of deviation for the studied ceramics.67
To further confirm the diffuseness of phase transition, the modified Curie–Weiss law is used, which is given in the following equation:6,62,65,68,69
|
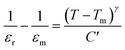 | (3) |
where
γ is the degree of diffuseness,
C′ is Curie-like constant,
Tm is the temperature of the maximum dielectric constant,
εr is the dielectric constant, and
εm is the maximum dielectric constant at
Tm. The value of
γ can be calculated from the slope of the fitting curves.
6 A higher
γ value indicates a stronger degree of diffuse phase transition. The slope of the fitting curve of ln(1/
εr − 1
/εm)
vs. ln(
T −
Tm) according to
eqn (3) is used to determine the
γ value. A linear relationship is observed for all compositions.
70 According to the
γ value in
Table 2 and the inset of
Fig. 6(a), the
γ value shows a slight change from 1.48 for the unannealed ceramic to 1.39 for the 12 h annealed ceramic. Thus, the annealing did not affect the diffuse phase transition too much.
3.5 Depolarization temperature (Td)
To determine the Td value, many techniques have been proposed.71 In the current study, the thermally stimulated depolarization current (TSDC) technique was employed. Plots of the current density–temperature (J–T) from the TSDC technique of the BNKT/0.01ZnO ceramics annealed at 950 °C with different annealing times are shown in Fig. 7. The Td value is also summarized in Table 2. It was found that the annealing time had no significant effect on the change of the Td value since the Td was slightly shifted down from 157 °C for the unannealed ceramic to 156 °C for the 12 h annealed ceramic. To confirm the trend of the value, the TF–R value was determined at a discontinuous change in dielectric loss (a small sharp peak at temperature ≤150 °C) in dielectric temperature spectra of the poled ceramics (Table 2). This temperature implies ferroelectric-relaxor transition upon heating.56 The TF–R value decreased from 168 to 166 °C after annealing for 12 h (i.e. a decrease of 2 °C). To double check the TSDC result, another different technique was performed. For this purpose, a measurement of the electromechanical coupling coefficient (kp) was carried out at a range of temperatures.72,73 The resulting shifting Td value is shown in the ESI (Fig. S2†). It was found that the Td value slightly decreased by 2 °C after annealing for 12 h. This result is consistent with the TDSC result.
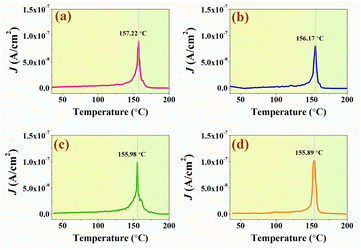 |
| Fig. 7 Plots current density–temperature (J–T) from the TSDC technique of the BNKT/0.01ZnO ceramics annealed at 950 °C with different annealing times, where (a) 0 h, (b) 4 h, (c) 8 h, and (d) 12 h. | |
To explain the shift in Td value, numerous hypotheses and models have been proposed, such as the ion doping model, the charge compensation model, and the stress field model. For the ion doping model, several authors have proposed that some dopant ions can diffuse into the host lattice during processing, hence promoting a more stable ferroelectric phase and causing the Td value to increase.19,74–77 Mahajan et al.75 also proposed that thermal depolarization behavior is considered to be strongly connected to the rhombohedral–tetragonal phase temperature. Li et al.76 found that the direct doping of Zn2+ into 0.94BNT–0.06BT may delay the thermal depolarization produced by the reformation of a long-range ferroelectric order due to the high polarizability of the Zn ion. This would cause a large dipole moment of the BO6 octahedra, enhance the coherence of neighboring dipoles, and inhibit the ferroelectric-relaxor transition. However, the diffusion of dopant ions can have a negative effect, i.e., it can cause composition and charge disorder, hence destabilizing the ferroelectric ordering in the samples. Some composites consisting of piezoelectric and semiconductor phases, such as the 0.94Bi0.5Na0.5TiO3–0.06BaTiO3/xZnO (BNT–6BT/xZnO) composites, for which ZnO is the semiconductor phase, were suggested using such a charge compensation model.18 In this case, the application of an external electric field causes a redistribution of the charges in the semiconductor ZnO at the interface between ZnO and BNT–BT. This provides a local electric field to compensate for the ferroelectric depolarization field when the external field is removed, hence stabilizing ferroelectric domains in composites.18
The stress field model was proposed for many studies on BNT-based ceramics, adding ZnO.75,78 For this model, it is believed that the thermal deviatoric stresses in a composite are caused by the difference in the coefficients of thermal expansion between ZnO and BNT–BT. This helps to stabilize the ferroelectric phase and broaden the temperature range of depolarization. This model has also been used to explain the increasing trend of Td in many composites such as Bi0.5(Na0.8K0.2)0.5TiO3:Al2O3 (BNKT:Al2O3).27 The creation of oxygen vacancies, as reported in many experiments, can raise the Td value, due to the domain walls in the grains of the ceramics being clamped. However, this is generally accompanied by a decline in piezoelectric properties.19 Doping B2O3 (as an interstitial dopant) into BNT–BT ceramics can increase the Td value. This was proposed to be due to the ability of stress generated from doping to stabilize the ferroelectric phase, thus, improving the Td value.19 Thomas79 proposed that the stability of a ferroelectric domain may be reduced if the coupling reaction between the A-site cation and the BO6 octahedron is weak. This model was utilized to explain the reduction in Td in a number of publications, which were connected with the weak coupling reaction that took place between the A-site cation and the BO6 octahedron.80,81 Furthermore, Nagata et al.82 proposed that the change in rhombohedral distortion affects the rise in Td in the 0.92BNT–0.08(Bi0.5Li0.5)TiO3 sample that had been quenched, which was caused by Bi ions being off-center. Khatua et al.83 reported an increase in Td value without a significant loss of piezoelectricity by grain size effect. They suggested the ferroelectric distortion of the field stabilized ferroelectric phase is dependent on the grain size.
In the current study, the reason for the improvements in piezoelectric properties (see next section) with the slight change in Td is complex. It could be for a variety of reasons. Normally, the annealing process would result in the formation of oxygen vacancies, which often leads to an increase in Td value, but will also be followed by a reduction in piezoelectric properties. However, for the present investigation, it is believed that the formation of oxygen vacancies is not too great because the annealing temperature is not too high. Therefore, the Td value slightly changed. Furthermore, ZnO was added to the studied ceramics. For the ZnO additive, it has been proposed that Zn ions can enter the lattices of many ceramic systems and then help stabilize the ferroelectric ordering.76 In addition, annealing improved ferroelectric properties by obtaining the optimal portion of the mixture phase and increasing grain size (see the following sections).
3.6 Ferroelectric properties
Normally, there are many factors affecting the characteristics of the ferroelectric hysteresis loop, which are associated with the material itself and the measurement conditions.84 In the current experiment, the polarization–electric field (P–E) hysteresis loop of the BNKT/0.01ZnO ceramics was measured at RT with different annealing times of 0–12 h, and under various electric fields of 30–65 kV cm−1, as shown in Fig. 8. The related ferroelectric properties are summarized in Table 3. It was found that the maximum polarization (Pmax), remanent polarization (Pr), and coercive field (Ec) values increased with an increase the applied electric field for all ceramics (Fig. 9(a) and (b)). At 30 kV cm−1 (Fig. 8), all ceramics showed an ellipse-like shape loop. This indicates a near-linear relationship of P–E, where it is known that some low electric fields are not enough to switch the domains.85 However, the polarization nonlinearity was developed in both the positive and negative field zones at 40 kV cm−1 electric fields. These results make it clearly evident that the electric field strength of 40 kV cm−1 has sufficient energy to constrain the realignment of some domains in the direction of the applied fields. At 65 kV cm−1 electric fields, polarization nonlinearity developed in both regions of the positive and negative fields with a well-saturated hysteresis loop. This indicates that the electric fields strength of 65 kV cm−1 has enough energy to constrain the realignment of all domains in the direction of the electric field.85 For an applied field greater than 65 kV cm−1, however, it caused the ceramics to undergo a dielectric breakdown.
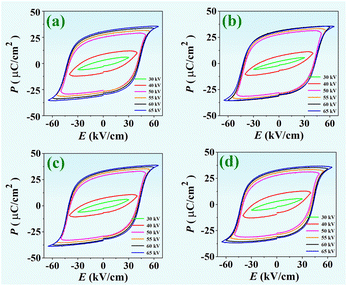 |
| Fig. 8 Polarization–electric field (P–E) hysteresis loop of the BNKT/0.01ZnO ceramics annealed at 950 °C with different annealing times, measured at RT under various electric field of 30–65 kV cm−1, where (a) 0 h, (b) 4 h, (c) 8 h, and (d) 12 h. | |
Table 3 Ferroelectric, piezoelectric, and energy harvesting properties of the BNKT/0.01ZnO ceramics annealed at 950 °C with different annealing times
Annealing time (h) |
Pmax (μC cm−2) |
Pr (μC cm−2) |
Ec (kV cm−1) |
Rsq |
d33 (pC N−1) |
kp |
g33 (10−3 Vm N−1) |
FoM (pm2 N−1) |
0 |
38.06 |
33.02 |
42.98 |
1.39 |
151 |
0.583 |
34.04 |
5.14 |
4 |
38.31 |
33.27 |
42.85 |
1.40 |
162 |
0.586 |
34.14 |
5.53 |
8 |
41.28 |
35.85 |
42.60 |
1.42 |
198 |
0.598 |
34.35 |
6.80 |
12 |
39.20 |
35.05 |
42.58 |
1.40 |
165 |
0.588 |
35.01 |
5.74 |
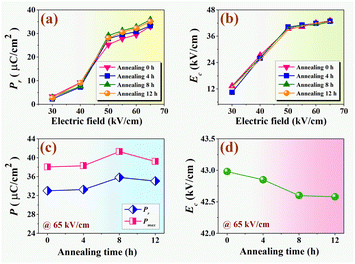 |
| Fig. 9 Plots of (a) Pr, (b) Ec values as a function of electric fields from 30–65 kV cm−1, (c) Pr and Pmax as a function of annealing time @ E = 65 kV cm−1, and (d) Ec as a function of annealing time @ E = 65 kV cm−1 of the BNKT/0.01ZnO ceramics annealed at 950 °C with different annealing times. | |
At an electric field of 65 kV cm−1, the unannealed ceramic displayed a well-saturated typical P–E hysteresis loop86 with the Pmax of 38.06 μC cm−2, Pr of 33.02 μC cm−2, and the Ec of 42.98 kV cm−1, indicating a strong ferroelectric ordering. However, the annealing had a significant influence on the Pmax, Pr and Ec values, i.e., it improved ferroelectric behavior, as seen from an enhancement trend in both the Pr and Pmax values. The Pr increased from 33.02 μC cm−2 for the un-annealed ceramic to 35.85 μC cm−2 for the 8 h annealed ceramic and then slightly decreased to 35.05 μC cm−2 when the annealing time increased up to 12 h (Fig. 9(c)). In addition, the Ec dropped with an increase in the annealing time (Fig. 9(d)). Normally, the improvement of ferroelectric properties can be related to many factors, as described in the previous section, such as MPB, grain size effect, heterogeneous chemical composition, and density. For this work, it was found that these factors were important factors, and it should be noted that ceramics with higher density and grain size values often have a higher Pr value.87 The 12 h annealed ceramic, on the other hand, has a lower density and thus a slightly lower Pr value.
For the grain size effect, Hao et al.88 studied the properties of (Ba0.85Ca0.15)(Zr0.1Ti0.9)O3 and proposed that the fraction of grains responsible for the opposite polarization reverse in ferroelectrics can be expressed using a formula:88
|
f = f0[1 − exp(−Gad3/kT)]
| (4) |
where
Ga is a constant representing the grain anisotropic energy density, and
d is the grain size. This equation illustrates that
f is relevant in relation to grain size. Therefore, as grain size increases, the number of grains that contribute to polarization reversals also increases. This increases the ferroelectricity of the ceramics.
88 For BaTiO
3, Zhao
et al.61 reported a relationship between grain size and the tetragonal distortion (
η), where
η =
c/
a − 1. They also proposed that
Ps can have a relation with
η as the following equation shows:
61 |
 | (5) |
and
k is a coefficient that relates to the electrostrictive coefficients, and
Ps is saturated polarization. When grain size increases, the
Ps2 thus also increases. In the current work, however, the increase in
η or
c/
a − 1 value indicates the increase in tetragonally of mixture phase (between rhombohedral and tetragonal). Therefore, annealing produced a good fraction of these phases and gave better or optimal ferroelectric properties.
To check the degree of improvement of the ferroelectric performance, the loop squareness (Rsq) may be an indicator for this. Haertling et al.89 used the squareness to measure not only the deviation in the polarization axis but also that in the electric field axis with the following empirical equation:90–92
|
 | (6) |
where
Pr is remanent polarization,
Ps is saturated polarization and
Ec is coercive field. The
P1.1Ec is the polarization at an electric field equal to 1.1 times of
Ec.
91 This equation can be used to quantify the changes in hysteresis behavior. For an ideal hysteresis loop,
Rsq is equal to 2.0. Based on the on the obtained data (
Table 3), the
Rsq increased with increasing annealing time, thereby confirming the improvement of ferroelectric performance. Therefore, the ferroelectric properties of the BNKT/0.01ZnO ceramics could be enhanced by optimizing annealing times.
Temperature dependence of polarization–electric field (P–E) hysteresis loops of the BNKT/0.01ZnO ceramics annealed at 950 °C with different annealing times, measured under an electric field of 65 kV cm−1 and a frequency of 0.1 kHz are shown in the ESI in Fig. S3.† All ceramics exhibited well-saturated P–E loops at RT (25 °C). With the increasing temperature up to 125 °C, the Pr and Ec values decreased, and the hysteresis loops started to deform and showed constricted loops for all samples at 150 °C. It was confirmed that the high temperature (∼150 °C) can cause lattice distortion and disrupt the ferroelectric long-range order, leading to a decrease in the polarization states.93 The decrease of Pr and Ec values with increasing temperature (Fig. S4(a) and S4(b)†) is consistent with that reported by Li et al.94 in the 0.94(Bi0.5Na0.5)TiO3–0.06BaTiO3 (BNTBT6) lead-free ceramics. They reported that the Pr and Ec decreased significantly after 96 °C, and then a pinched hysteresis was obtained with the increasing temperature. The change of ferroelectric (FE) ordering, and a phase transition from ferroelectric (FE) to ergodic relaxor (RE) were thus observed.
3.7 Piezoelectric properties
Plots of the piezoelectric coefficient (d33) value of the BNKT/0.01ZnO ceramics at different annealing times are shown in Fig. 10. The related values are also listed in Table 3. The d33 value of the unannealed ceramic in this study was 151 pC N−1. The d33 increased with an increase in the annealing time and achieved the maximum value of 198 pC N−1 for the 8 h annealed ceramic. However, as the annealing time increased to 12 h, these values decreased slightly. The planar electromechanical coupling coefficient (kp) for this experiment was determined from measured values guided by the following formula:4,95,96 |
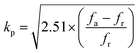 | (7) |
where fr and fa are the resonance and anti-resonance frequencies, respectively.95 In this work, the maximum kp of 0.598 was also obtained for the 8 h annealed ceramic (see Table 3). Normally, the improvements of d33 and kp for many piezoelectric ceramics can be related to many factors. The grain size effect is one of them. Kang et al.97 argued that the poling efficiency rose with increasing grain size in the case of lead-free ceramics, such as in the Pb0.9La0.1TiO3 system, which was attributable to improving the facility of 90° domain switching. They also proposed that, as the increase in grain size, it would be expected to enhance the domain switching at the same poling condition, the increases in the d33 and kp values could be explained by the increased domain mobility.
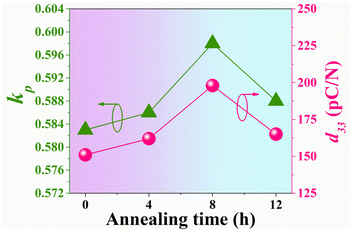 |
| Fig. 10 Plots of the kp and d33 values of the BNKT/0.01ZnO ceramics annealed at 950 °C with different annealing times. | |
The improvement in the d33 value in the many studies can be explained by the following thermodynamic theory of the ferroelectric's equation:34,88,98
where
ε33T represents the dielectric constant of the material,
Pr is the remnant polarization, and
Q11 represents the electrostrictive coefficient, which is constant for perovskite materials. This equation demonstrates that the
d33 is proportional to the
Pr value, which matches the data of the current study. Furthermore, for the 8 h annealed ceramic, the
Pr increased from 30.02 μC cm
−2 to 35.85 μC cm
−2 and
Ec slightly dropped from 42.98 kV cm
−1 to 42.58 kV cm
−1 (see
Table 3), thus resulting in a significant improvement of the
d33 value for this ceramic.
99 The observed trend in the value of the
d33 is consistent with the value of the
kp (see
Fig. 10).
7,100–103 Maqbool
et al.104 also found that the
d33 and
kp increased when the SrZrO
3 (SZ) concentration that was doped in BNT ceramics increased. The significant increase found in
d33 and
kp at SZ4 was related to a large
Pr and a lower
Ec because a lower
Ec allowed the ceramics to be more easily poled, while a high
Pr and
Pm favored piezoelectricity. Furthermore, several previous studies proposed that the dielectric constant, piezoelectric constant, and
kp followed a similar trend.
105,106 The improvement of
d33 and
kp values in the current study is also related to the ferroelectric properties (improve the
Pr,
Pm, and low
Ec), the grain size effect, the MPB, heterogeneous in composition (decrease in the chemical heterogeneity), and also accompanied by the improvement of density, where the trends of
d33 and
kp values can be matched with the trend of density value (see
Tables 1 and
3).
3.8 Energy harvesting behavior
Piezoelectric materials are now attractive for the development of energy harvesting applications. In this current experiment, the energy harvesting properties of the ceramics were investigated. The piezoelectric voltage constant (g33) is one of the important parameters for energy harvesting applications. It has been suggested that the sample with a higher piezoelectric charge constant should generate a higher voltage,107,108 thus reflecting the quantity of electrical energy produced by the pressure being applied. The g33 value can be determined by the following relation:107–111 |
 | (9) |
where εr is the dielectric constant of the piezoelectric material, ε0 is the dielectric constant in a vacuum (8.854 × 10−12 F m−1),112 and d33 is the piezoelectric charge constant. From this equation, the generated voltage of the piezoelectric energy harvester depends on the piezoelectric coefficient and dielectric constant of the piezoelectric ceramic. Therefore, the more the piezoelectric charge constant increases, the more the generated voltage also increases.107 A plot of the g33 value of the BNKT/0.01ZnO ceramics at different annealing times is shown in Fig. 11. The g33 increased with increasing annealing time, and the highest g33 of 35.01 × 10−3 Vm N−1 was observed for the 12 h annealed ceramic. The improvement of the g33 value for this ceramic is due to the increase of the d33, and the lowering of the εr value. This result is consistent with the work done by Choi et al.108
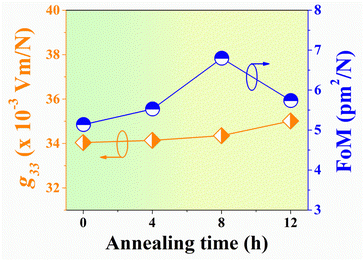 |
| Fig. 11 Plots of the g33 and FoM values of the BNKT/0.01ZnO ceramics annealed at 950 °C with different annealing times. | |
In this study the energy harvesting performance figure of merit which relates to the g33 and d33 parameters, was evaluated.111 In the present investigation, the figure of merit (FoM) parameter was evaluated based on the following equation:108,109
|
FoM (pm2 N−1) = d33 × g33
| (10) |
where
d33 is the piezoelectric charge constant, and
g33 is the piezoelectric voltage constant of the device, respectively. Normally, a piezoelectric material with a high FoM value will produce a large amount of power when utilized for energy harvesting.
113 For the present study, the highest FoM of 6.80 pm
2 N
−1 was observed for the 8 h annealed ceramic, which was ∼32% higher than that of the un-annealed ceramic (see
Fig. 11 and
Table 3). This improvement is due to the improvements in the
d33 and
g33 values for this composition. The results of a comparison of the FoM value for this study with the results of other earlier research studies for different piezoelectric lead-free ceramics are represented in
Table 4.
98,107–109,111,114–120 The
g33 and FoM values for the current experiment are considerably high when compared with other lead free piezoelectric ceramics.
Table 4 Comparison of the d33, g33, and FoM values of the BNKT/0.01ZnO ceramics annealed at 950 °C for 8 h with other lead and lead-free ceramics
System |
d33 (pC N−1) |
g33 (10−3 Vm N−1) |
FoM (pm2 N−1) |
Ref. |
BNKT/0.01ZnO (annealed at 950 °C/8 h) |
198 |
34.35 |
6.80 |
This work |
(BNT–6BT) + 0.1 mol% Al2O3 |
205 |
31.00 |
6.36 |
98 |
Ba(Zr0.04Ti0.96)O3 |
268 |
19.11 |
5.12 |
107 |
Ba(Zr0.04Ti0.96)O3 + 1 mol% CuO |
302 |
20.64 |
6.23 |
107 |
PZT-70 vol% P(VDF-CTFE) |
92 |
— |
6.44 |
108 |
0.55BZT–0.45BCT (sintered 1475 °C) |
341 |
16.00 |
5.46 |
109 |
0.99KNLN0.97−xSTx − 0.01CZ (x = 0.03) |
225 |
28.69 |
6.46 |
111 |
Ba(Zr0.07Ti0.93)O3 + 2 wt% B2O3 |
291 |
21.00 |
6.11 |
114 |
(BZT–BCT)-(epoxy-CCTO) |
16 |
— |
4.25 |
115 |
PZT bulk |
556 |
0.011 |
6.12 |
116 |
PZT micro-pillar |
91 |
0.021 |
1.91 |
116 |
80PZT-PVDF composites (0–3 connectivity) |
54 |
33.95 |
1.83 |
117 |
0.2(PbMg1/3Nb2/3O3)–0.8(PbZr0.475Ti0.525O3) (bulk-type 1 layer) |
241 |
10.89 |
2.62 |
118 |
0.97[0.995BNKT-0.005LN]-0.03BNdT ceramics |
258 |
14.71 |
3.80 |
119 |
0.92(Bi0.5Na0.5)TiO3–0.08(Ba0.5Sr0.5)TiO3 |
118 |
— |
2.85 |
120 |
Based on the obtained results, the suitable annealing conditions provided the ceramics with excellence and acceptability for all electrical properties. In this study, the optimum annealing time for the enhancement of dielectric, piezoelectric, and energy harvesting performances of the BNKT/0.01ZnO ceramics was found to be 8 h dwell time.
4 Conclusions
In summary, the effect of thermal annealing time on phase formation, microstructure, dielectric, phase transition, ferroelectric, piezoelectric, and energy harvesting properties of the BNKT/0.01ZnO ceramic prepared by a solid-state reaction technique was investigated. The ceramics which were annealed at 950 °C with different annealing times, exhibited the MPB structure where the rhombohedral and tetragonal phases coexisted, as confirmed by the XRD and Raman measurements. The annealing time made a slight change in the phase transition temperatures, also including the Td. However, it had significant positive effects on the dielectric, ferroelectric, piezoelectric, and energy harvesting properties of the BNKT/0.01ZnO ceramics, where the optimal annealing time for the current investigation was 8 h.
Author contributions
Pharatree Jaita: writing-original draft preparation. Kamonporn Saenkam: methodology-sample preparation. Gobwute Rujijanagul: conceptualization improve the manuscript and supervisions.
Conflicts of interest
There are no conflicts to declare.
Acknowledgements
This project is funded by National Research Council of Thailand (NRCT) (NRCT5-RSA63004, NRCT5-RSA63004-03) and partially supported by Chiang Mai University, Research Center in Physics, Astronomy, Materials Sciences Research Center, Global Partnership Project (B16F640001), and CMU Junior Research Fellowship Program (JRCMU2565_058). Office of Research Administration, Department of Physics and Materials Science, Faculty of Science, and Science and Technology Research Institute, Chiang Mai University are also acknowledged.
References
- J. Feng, R. Huang, Z. Liang, Z. Du, Y. Dai, J. Wu and H. T. Lin, The effect of B site doping of Nb5+ and aging process on the properties of BNKT-BT lead-free piezoelectric ceramics, Ceram. Int., 2022, 48, 2355–2361 CrossRef CAS.
- J. Camargo, S. Osinaga, M. Febbo, S. P. Machado, F. R. Marcos, L. Ramajo and M. Castro, Piezoelectric and structural properties of bismuth sodium potassium titanate lead-free ceramics for energy harvesting, J. Mater. Sci.: Mater. Electron., 2021, 32, 19117–19125 CrossRef CAS.
- A. Chauhan, S. Patel, G. Vats and R. Vaish, Enhanced thermal energy harvesting using Li, K-Doped Bi0.5Na0.5TiO3 lead-free ferroelectric ceramics, Energy Technol., 2014, 2, 205–209 CrossRef CAS.
- S. Zhao, H. Wu and Q. Sun, Study on PSN-PZN-PZT quaternary piezoelectric ceramics near the morphotropic phase boundary, Mater. Sci. Eng., B, 2005, 123, 203–210 CrossRef.
- N. P. M. J. Raj, G. Khandelwal and S. J. Kim, 0.8BNT-0.2BKT ferroelectric-based multimode energy harvester for self-powered body motion sensors, Nanomater. Energy, 2021, 83, 105848 Search PubMed.
- X. Qiao, D. Wu, F. Zhang, M. Niu, B. Chen, X. Zhao, P. Liang, L. Wei, X. Chao and Z. Yang, Enhanced energy density and thermal stability in relaxor ferroelectric Bi0.5Na0.5TiO3-Sr0.7Bi0.2TiO3 ceramics, J. Eur. Ceram. Soc., 2019, 39, 4778–4784 CrossRef CAS.
- A. Ullah, C. W. Ahn and I. W. Kim, Dielectric, piezoelectric properties and field-induced large strain of Bi(Zn0.5Ti0.5)O3-modified morphotropic phase boundary Bi0.5(Na0.82K0.18)0.5TiO3 piezoelectric ceramics, Jpn. J. Appl. Phys., 2012, 51, 09MD07 CrossRef.
- Y. Zhang, X. Liu, G. Wang, Y. Li, S. Zhang, D. Wang and H. Sun, Enhanced mechanical energy harvesting capability in sodium bismuth titanate based lead-free piezoelectric, J. Alloys Compd., 2020, 825, 154020 CrossRef CAS.
- Z. Yang, B. Liu, L. Wei and Y. Hou, Structure and electrical properties of (1-x)Bi0.5Na0.5TiO3-xBi0.5K0.5TiO3 ceramics near morphotropic phase boundary, Mater. Res. Bull., 2008, 43, 81–89 CrossRef CAS.
- A. Sasaki, T. Chiba, Y. Mamiya and E. Otsuki, Dielectric and piezoelectric properties of (Bi0.5Na0.5)TiO3-(Bi0.5K0.5)TiO3 systems, Jpn. J. Appl. Phys., 1999, 38, 5564–5567 CrossRef CAS.
- K. Yoshii, Y. Hiruma, H. Nagata and T. Takenaka, Electrical properties and depolarization temperature of (Bi1/2Na1/2)TiO3-(Bi1/2K1/2)TiO3 lead-free piezoelectric ceramics, Jpn. J. Appl. Phys., 2006, 45, 4493–4496 CrossRef CAS.
- P. Jaita, A. Watcharapasorn, N. Kumar, S. Jiansirisomboon and D. P. Cann, Lead-free (Ba0.70Sr0.30)TiO3-modified Bi0.5(Na0.80K0.20)0.5TiO3 ceramics with large electric field-induced strains, J. Am. Ceram. Soc., 2016, 99, 1615–1624 CrossRef CAS.
- X. Ren, H. Fan, Y. Zhao and Z. Liu, Flexible lead-free BiFeO3/PDMS-based nanogenerator as piezoelectric energy harvester, ACS Appl. Mater. Interfaces, 2016, 8, 26190–26197 CrossRef CAS PubMed.
- M. M. Alam, S. K. Ghosh, A. Sultana and D. Mandal, Lead-free ZnSnO3/MWCNTs-based selfpoled flexible hybrid nanogenerator for piezoelectric power generation, Nanotechnology, 2015, 26, 165403 CrossRef PubMed.
- M. Promsawat, A. Watcharapasorn, H. N. Tailor, S. Jiansirisomboon and Z. G. Ye, Enhanced dielectric, ferroelectric, and electrostrictive properties of Pb(Mg1/3Nb2/3)0.9Ti0.1O3 ceramics by ZnO modification, J. Appl. Phys., 2013, 113, 204101 CrossRef.
- Y. C. Lee, T. K. Lee and J. H. Jan, Piezoelectric properties and microstructures of ZnO-doped
Bi0.5Na0.5TiO3 ceramics, J. Eur. Ceram. Soc., 2011, 31, 3145–3152 CrossRef CAS.
- F. R. Marcos, J. J. Romero, M. G. N. Rojero and J. F. Fernandez, Effect of ZnO on the structure, microstructure and electrical properties of KNN-modified piezoceramics, J. Eur. Ceram. Soc., 2009, 29, 3045–3052 CrossRef.
- J. Zhang, Z. Pan, F. F. Guo, W. C. Liu, H. Ning, Y. B. Chen, M. H. Lu, B. Yang, J. Chen, S. T. Zhang, X. Xing, J. Rödel, W. Cao and Y. F. Chen, Semiconductor/relaxor 0-3 type composites without thermal depolarization in Bi0.5Na0.5TiO3-based lead-free piezoceramics, Nat. Commun., 2015, 6, 6615 CrossRef CAS PubMed.
- S. Manotham, P. Jaita, P. Butnoi, N. Lertcumfu and G. Rujijanagul, Improvements of depolarization temperature, piezoelectric and energy harvesting properties of BNT-based ceramics by doping an interstitial dopant, J. Alloys Compd., 2022, 897, 163021 CrossRef CAS.
- X. Zhang, G. Jiang, D. Liu, B. Yang and W. Cao, Improved depolarization behavior and electric properties in (Bi0.5Na0.5)TiO3-based piezoelectric composites, J. Alloys Compd., 2018, 769, 660–668 CrossRef CAS.
- W. P. Cao, W. L. Li, Y. Feng, D. Xu, W. Wang, Y. F. Hou, T. D. Zhang and W. D. Fei, Enhanced depolarization temperature in 0.90NBT-0.05KBT-0.05BT ceramics induced by BT nanowires, J. Phys. Chem. Solids, 2015, 78, 41–45 CrossRef CAS.
- L. Wang, T. K. Song, S. C. Lee, J. H. Cho, Y. S. Sung, M. H. Kim and K. S. Choi, Dielectric and piezoelectric properties of Li-substituted lead-free (Bi0.5Na0.5)TiO3-(Bi0.5K0.5)TiO3-BaTiO3 ceramics, Curr. Appl. Phys., 2010, 10, 1059–1061 CrossRef.
- Y. Watanabe, Y. Hiruma, H. Nagata and T. Takenaka, Phase transition temperatures and electrical properties of divalent ions (Ca2+, Sr2+ and Ba2+) substituted (Bi1/2Na1/2)TiO3 ceramics, Ceram. Int., 2008, 34, 761–764 CrossRef CAS.
- A. Maqbool, A. Hussain, J. U. Rahman, T. K. Song, W. J. Kim, J. Lee and M. H. Kim, Enhanced electric field-induced strain and ferroelectric behavior of (Bi0.5Na0.5)TiO3-BaTiO3-SrZrO3 lead-free ceramics, Ceram. Int., 2014, 40, 11905–11914 CrossRef CAS.
- Q. Xu, Y. H. Huang, M. Chen, W. Chen, B. H. Kim and B. K. Ahn, Effect of bismuth deficiency on structure and electrical properties of (Na0.5Bi0.5)0.93Ba0.07TiO3 ceramics, J. Phys. Chem. Solids, 2008, 69, 1996–2003 CrossRef CAS.
- X. S. Qiao, X. M. Chen, H. L. Lian, W. T. Chen, J. P. Zhou and P. Liu, Microstructure and electrical properties of nonstoichiometric 0.94(Na0.5Bi0.5+x)TiO3-0.06BaTiO3 lead-free ceramics, J. Am. Ceram. Soc., 2016, 99, 198–205 CrossRef CAS.
- J. Yin, Y. Wang, Y. Zhang, B. Wu and J. Wu, Thermal depolarization regulation by oxides selection in lead-free BNT/oxides piezoelectric composites, Acta Mater., 2018, 158, 269–277 CrossRef CAS.
- W. Bai, L. Wang, P. Zheng, F. Wen, J. Zhai and Z. Ji, Pairing high piezoelectric properties and enhanced thermal stability in grain-oriented BNT-based lead-free piezoceramics, Ceram. Int., 2018, 44, 11402–11409 CrossRef CAS.
- M. Ghasemifard, M. Daneshvar and M. Ghamari, The Effects of annealing process on dielectric and piezoelectric properties of BMT-base lead-free ceramics, World J. Nano Sci. Eng., 2013, 3, 100–107 CrossRef.
- G. Rujijanagul, N. Vittayakorn and S. Nabunmee, Effect of annealing time on electrical and mechanical properties of 0.7(Pb(Zr1/2Ti1/2)O3)-0.3(Pb(Zn1/2Nb2/3)O3) ceramics, Ferroelectrics, 2009, 384, 68–72 CrossRef CAS.
- J. Xu, Q. Li, L. Yang, W. Zeng, C. Zhou, C. Yuan, G. Chen and G. Rao, Effects of thermal and electrical histories on structure and dielectric behaviors of (Li0.5Nd0.5)2+-modified (Bi0.5Na0.5)TiO3-BaTiO3 ceramics, J. Materiomics, 2017, 3, 121–129 CrossRef.
- K. Wang, B. P. Zhang, J. F. Li and L. M. Zhang, Lead-free Na0.5K0.5NbO3 piezoelectric ceramics fabricated by spark plasma sintering: Annealing effect on electrical properties, J. Electroceram., 2008, 21, 251–254 CrossRef CAS.
- S. Hajra, S. Sahoo, R. Das and R. N. P. Choudhary, Structural, dielectric and impedance characteristics of (Bi0.5Na0.5)TiO3-BaTiO3 electronic system, J. Alloys Compd., 2018, 750, 507–514 CrossRef CAS.
- A. Ullah, C. W. Ahn, A. Hussain, I. W. Kim, H. I. Hwang and N. K. Cho, Structural transition and large electric field-induced strain in BiAlO3-modified Bi0.5(Na0.8K0.2)0.5TiO3 lead-free piezoelectric ceramics, Solid State Commun., 2010, 150, 1145–1149 CrossRef CAS.
- K. N. Pham, A. Hussain, C. W. Ahn, I. W. Kim, S. J. Jeong and J. S. Lee, Giant strain in Nb doped Bi0.5(Na0.82K0.18)0.5TiO3 lead-free electromechanical ceramics, Mater. Lett., 2010, 64, 2219–2222 CrossRef CAS.
- B. D. Cullity, Elements of X-ray Diffraction. Addison-Wesley Publishing, Philippines, 1978 Search PubMed.
- R. Rafique, K. N. Tonny, A. Sharmin and Z. H. Mahmood, Study on the effect of varying film thickness on the transparent conductive nature of aluminum doped zinc oxide deposited by dip coating, Mater. Focus, 2018, 7, 1–7 CrossRef.
- A. Hosseinmardi, N. Shojaee, M. K. Rad and T. Ebadzadeh, A study on the photoluminescence properties of electrospray deposited amorphous and crystalline nanostructured ZnO thin films, Ceram. Int., 2012, 38, 1975–1980 CrossRef CAS.
- M. J. Lee, T. I. Lee, J. Lim, J. Bang, W. Lee, T. Lee and J. M. Myoung, Effect of the deposition temperature and a hydrogen post-annealing treatment on the structural, electrical, and optical properties of Ga-doped ZnO films, Electron. Mater. Lett., 2009, 5, 127–133 CrossRef CAS.
- A. Zaier, A. Meftah, A. Y. Jaber, A. A. Abdelaziz and M. S. Aida, Annealing effects on the structural, electrical and optical properties of ZnO thin films prepared by thermal evaporation technique, J. King Saud Univ., Sci., 2015, 27, 356–360 CrossRef.
- A. Gahtar, A. Benali, S. Benramache and C. Zaouche, Effect of annealing time on the structural, morphological, optical and electrical properties of NiS thin films, Chalcogenide Lett., 2022, 19, 103–116 CrossRef CAS.
- K. A. Razak, C. J. Yip and S. Sreekantan, Synthesis of (Bi0.5Na0.5)TiO3 (BNT) and Pr doped BNT using the soft combustion technique and its properties, J. Alloys Compd., 2011, 509, 2936–2941 CrossRef.
- N. D. Co, L. V. Cuong, B. D. Tu, P. D. Thang, L. X. Dien, V. N. Hung and N. D. Quan, Effect of crystallization temperature on energy-storage density and efficiency of lead-free Bi0.5(Na0.8K0.2)0.5TiO3 thin films prepared by sol-gel method, J. Sci.: Adv. Mater. Devices, 2019, 4, 370–375 Search PubMed.
- S. Wada, K. Yamato, P. Pulpan, N. Kumada, B. Y. Lee, T. Iijima, C. Moriyoshi and Y. Kuroiwa, Piezoelectric properties of high Curie temperature barium titanate-bismuth perovskite-type oxide system ceramics, J. Appl. Phys., 2010, 108, 094114 CrossRef.
- S. Wada, K. Yamato, P. Pulpan, N. Kumada, B. Y. Lee, T. Iijima, C. Moriyoshi and Y. Kuroiwa, Preparation of barium titanate-bismuth magnesium titanate ceramics with high Curie temperature and their piezoelectric properties, J. Ceram. Soc. Jpn., 2010, 118, 683–687 CrossRef CAS.
- M. Difeo, S. Osinaga, M. Febbo, S. P. Machado, M. Castro and L. Ramajo, Influence of the (Bi0.5Na0.5)TiO3-BaTiO3 lead-free piezoceramic geometries on the power generation of energy harvesting devices, Ceram. Int., 2021, 47, 10696–10704 CrossRef CAS.
- D. Rout, K. S. Moon, S. J. L. Kang and I. W. Kim, Dielectric and Raman scattering studies of phase transitions in the (100-x)Na0.5Bi0.5TiO3-xSrTiO3 system, J. Appl. Phys., 2010, 108, 084102 CrossRef.
- S. Prasertpalichat, T. Siritanon, N. Nuntawong and D. P. Cann, Structural characterization of A-site nonstoichiometric (1-x)Bi0.5Na0.5TiO3-xBaTiO3 ceramics, J. Mater. Sci., 2019, 54, 1162–1170 CrossRef CAS.
- J. Wang, C. Zhou, Q. Li, W. Zeng, J. Xu, G. Chen, C. Yuan and G. Rao, Dual relaxation behaviors and large electrostrictive properties of Bi0.5Na0.5TiO3-Sr0.85Bi0.1TiO3 ceramics, J. Mater. Sci., 2018, 53, 8844–8854 CrossRef CAS.
- M. Zhong, Q. Feng, C. Yuan, X. Liu, B. Zhu, L. Meng, C. Zhou, J. Xu, J. Wang and G. Rao, Photocurrent density and electrical properties of Bi0.5Na0.5TiO3-BaNi0.5Nb0.5O3 ceramics, J. Adv. Ceram., 2021, 10, 1119–1128 CrossRef CAS.
- J. Hao, B. Shen, J. Zhai, C. Liu, X. Li and X. Gao, Large strain response in 0.99(Bi0.5Na0.4K0.1)TiO3-0.01(KxNa1−x)NbO3 lead-free ceramics induced by the change of K/Na ratio in (KxNa1-x)NbO3, J. Am. Ceram. Soc., 2013, 96, 3133–3140 CrossRef CAS.
- P. Jarupoom and G. Rujijanagul, Improvement in piezoelectric strain of annealed Ba(Zr0.07Ti0.93)O3 based ceramics, J. Appl. Phys., 2013, 114, 027018 CrossRef.
- S. Eitssayeam, P. Jarupoom and G. Rujijanagul, High dielectric and piezoelectric properties observed in annealed Pb0.88Sr0.12Zr0.54Ti0.44Sb0.02O3 ceramics, Ferroelectrics, 2013, 451, 48–53 CrossRef CAS.
- M. F. Mat, Y. H. P. Manurung, N. Muhammad, S. N. S. Ahmad, M. Graf and M. S. Sulaiman, Variable analysis for grain size prediction of austenitic stainless steel SS316l using heat treatment, J. Appl. Sci., 2020, 20, 91–96 CrossRef CAS.
- Y. Hiruma, H. Nagata and T. Takenaka, Grain-size effect on electrical properties of (Bi1/2K1/2)TiO3 ceramics, Jpn. J. Appl. Phys., 2007, 46, 1081–1084 CrossRef CAS.
- X. Zhou, G. Xue, H. Luo, C. R. Bowen and D. Zhang, Phase structure and properties of sodium bismuth titanate lead-free piezoelectric ceramics, Prog. Mater. Sci., 2021, 122, 100836 CrossRef CAS.
- Q. Li, L. Ning, C. Wang and H. Fan, Gaint electric field-induced strain and ferroelectric behavior of Bi0.5Na0.4K0.1TiO3-Na1-xLixNbO3 lead-free ceramics, Mater. Sci. Eng., B, 2021, 263, 114819 CrossRef CAS.
- M. Habib, M. Munir, S. A. Khan, T. K. Song, M. H. Kim, M. J. Iqbal, I. Qazi and A. Hussain, Evaluation of high strain response in lead-free BNBTFS-xNb ceramics by structure and ferroelectric characterizations, J. Phys. Chem. Solids, 2020, 138, 109230 CrossRef CAS.
- F. Xia and X. Yao, Postsintering annealing induced extrinsic dielectric and piezoelectric responses in lead-zinc-niobate-based ferroelectric ceramics, J. Appl. Phys., 2002, 92, 2709–2716 CrossRef CAS.
- V. R. Mudinepalli, L. Feng, W. C. Lin and B. S. Murty, Effect of grain size on dielectric and ferroelectric properties of nanostructured Ba0.8Sr0.2TiO3 ceramics, J. Adv. Ceram., 2015, 4, 46–53 CrossRef CAS.
- Z. Zhao, V. Buscaglia, M. Viviani, M. T. Buscaglia, L. Mitoseriu, A. Testino, M. Nygren, M. Johnsson and P. Nanni, Grain-size effects on the ferroelectric behavior of dense nanocrystalline BaTiO3 ceramics, Phys. Rev. B: Condens. Matter Mater. Phys., 2004, 70, 024107 CrossRef.
- S. K. Gupta, R. M. Quade, B. Gibbons, P. Mardilovich and D. P. Cann, Electric field-induced strain in Sr(Hf0.5Zr0.5)O3-modified Bi0.5(Na0.8K0.2)0.5TiO3 piezoelectric ceramics, J. Appl. Phys., 2020, 127, 074104 CrossRef.
- A. Bhalla, R. Guo, L. Cross, G. Burns, F. Dacol and R. R. Neurgaonkar, Measurements of strain and the optical indices in the ferroelectric Ba0.4Sr0.6Nb2O6: Polarization effects, Phys. Rev. B: Condens. Matter Mater. Phys., 1987, 36, 2030–2035 CrossRef CAS PubMed.
- K. Uchino, S. Nomura, L. E. Cross, R. E. Newnham and S. J. Jang, Electrostrictive effect in perovskites and its transducer applications, J. Mater. Sci., 1981, 16, 569–578 CrossRef CAS.
- Z. Yang, H. Du, L. Jin, Q. Hu, H. Wang, Y. Li, J. Wang, F. Gao and S. Qu, Realizing high comprehensive energy storage performance in lead-free bulk ceramics via designing an unmatched temperature range, J. Mater. Chem. A, 2019, 7, 27256 RSC.
- R. Kang, Z. Wang, X. Lou, W. Liu, P. Shi, X. Zhu, X. Guo, S. Li, H. Sun, L. Zhang and Q. Sun, Energy storage performance of Bi0.5Na0.5TiO3-based relaxor ferroelectric ceramics with superior temperature stability under low electric fields, Chem. Eng. J., 2021, 410, 128376 CrossRef CAS.
- S. B. Li, C. B. Wang, L. Li, Q. Shen and L. M. Zhang, Effect of annealing temperature on structural and electrical properties of BCZT ceramics prepared by plasma activated sintering, J. Alloys Compd., 2018, 730, 182–190 CrossRef CAS.
- K. Uchino and S. Nomura, Critical exponents of the dielectric constants in diffused-phase-transition crystals, Ferroelectr., Lett., 1982, 44, 55–61 CrossRef CAS.
- Q. Hu, Y. Tian, Q. Zhu, J. Bian, L. Jin, H. Du, D. O. Alikin, V. Y. Shur, Y. Feng, Z. Xu and X. Wei, Achieve ultrahigh energy storage performance in BaTiO3-Bi(Mg1/2Ti1/2)O3 relaxor ferroelectric ceramics via nano-scale polarization mismatch and reconstruction, Nano Energy, 2020, 67, 104264 CrossRef CAS.
- J. Li, D. Zhang, S. Qin, T. Li, M. Wu, D. Wang, Y. Bai and X. Lou, Large room-temperature electrocaloric effect in lead-free BaHfxTi1-xO3 ceramics under low electric field, Acta Mater., 2016, 115, 58–67 CrossRef CAS.
- E. M. Anton, W. Jo, D. Damjanovic and J. Rodel, Determination of depolarization temperature of (Bi1/2Na1/2)TiO3-based lead-free piezoceramics, J. Appl. Phys., 2011, 110, 094108 CrossRef.
- Y. J. Dai and X. W. Zhang, An approach to improve the piezoelectric property of (Bi0.5Na0.5)TiO3-(Bi0.5K0.5)TiO3-BaTiO3 lead-free ceramics, Int. J. Appl. Ceram. Technol., 2011, 8, 423–429 CrossRef CAS.
- J. J. Wang, F. F. Guo, B. Yang, S. T. Zhang, L. M. Zheng, F. M. Wu and W. W. Cao, Electrical properties of 0.94Bi0.5Na0.5TiO3-0.06Ba(Zr0.055Ti0.945)O3 lead-free ceramics with high thermal stability, J. Mater. Sci.: Mater. Electron., 2018, 29, 2357–2362 CrossRef CAS.
- J. Wang, C. Zhou, Q. Li, L. Yang, J. Xu, G. Chen, C. Yuan and G. Rao, Simultaneously enhanced piezoelectric properties and depolarization temperature in calcium doped BiFeO3-BaTiO3 ceramics, J. Alloys Compd., 2018, 748, 758–765 CrossRef CAS.
- A. Mahajan, H. Zhang, J. Wu, E. V. Ramana, M. J. Reece and H. Yan, Effect of phase transitions on thermal depoling in lead-free 0.94Bi0.5Na0.5TiO3-0.06BaTiO3 based piezoelectrics, J. Phys. Chem. C, 2017, 121, 5709–5718 CrossRef CAS.
- L. Li, M. Zhu, K. Zhou, Q. Wei, M. Zheng and Y. Hou, Delayed thermal depolarization of Bi0.5Na0.5TiO3-BaTiO3 by doping acceptor Zn2+ with large ionic polarizability, J. Appl. Phys., 2017, 122, 204104 CrossRef.
- M. Davies, E. Aksel and J. L. Jones, Enhanced high-temperature piezoelectric coefficients and thermal stability of Fe-and Mn-substituted Na0.5Bi0.5TiO3 ceramics, J. Am. Ceram. Soc., 2011, 94, 1314–1316 CrossRef CAS.
- L. M. Riemer, K. V. Lalitha, X. Jiang, N. Liu, C. Dietz, R. W. Stark, P. B. Groszewicz, G. Buntkowsky, J. Chen, S. T. Zhang, J. Rödel and J. Koruza, Stress-induced phase transition in lead-free relaxor ferroelectric composites, Acta Mater., 2017, 136, 271–280 CrossRef CAS.
- N. W. Thomas, A new framework for understanding relaxor ferroelectrics, J. Phys. Chem. Solids, 1990, 51, 1419–1431 CrossRef CAS.
- Z. Yang, Y. Hou, B. Liu and L. Wei, Structure and electrical properties of Nd2O3-doped 0.82Bi0.5Na0.5TiO3-0.18Bi0.5K0.5TiO3 ceramics, Ceram. Int., 2009, 35, 1423–1427 CrossRef CAS.
- N. Y. Quyet, L. H. Bac and D. D. Dung, Effect of Li2CO3 addition on the structural, optical, ferroelectric, and electric-field-induced strain of lead-free BNKT-based ceramics, J. Phys. Chem. Solids, 2015, 85, 148–154 CrossRef.
- H. Nagata, Y. Takagi, Y. Yoneda and T. Takenaka, Correlation between depolarization temperature and lattice distortion in quenched (Bi1/2Na1/2)TiO3-based ceramics, Appl. Phys. Express, 2020, 13, 061002 CrossRef CAS.
- D. K. Khatua, A. Mishra, N. Kumar, G. D. Adhikary, U. Shankar, B. Majumdar and R. Ranjan, A coupled microstructural-structural mechanism governing thermal depolarization delay in Na0.5Bi0.5TiO3-based piezoelectrics, Acta Mater., 2019, 179, 49–60 CrossRef CAS.
- L. Jin, F. Li and S. Zhang, Decoding the fingerprint of ferroelectric loops: comprehension of the material properties and structures, J. Am. Ceram. Soc., 2014, 97, 1–27 CrossRef CAS.
- N. Vittayakorn, G. Rujijanagul and D. P. Cann, The improvement in dielectric and ferroelectric performance of PZT-PZN ceramics by thermal treatment, Curr. Appl. Phys., 2007, 7, 582–585 CrossRef.
- G. Dong, H. Fan, J. Shi and M. Li, Composition- and temperature-dependent large strain in (1-x)(0.8Bi0.5Na0.5TiO3-0.2Bi0.5K0.5TiO3)-xNaNbO3 ceramics, J. Am. Ceram. Soc., 2015, 98, 1150–1155 CrossRef CAS.
- Y. Huan, X. Wang, J. Fang and L. Li, Grain size effect on piezoelectric and ferroelectric properties of BaTiO3 ceramics, J. Eur. Ceram. Soc., 2014, 34, 1445–1448 CrossRef.
- J. Hao, W. Bai, W. Li and J. Zhai, Correlation between the microstructure and electrical properties in high-performance (Ba0.85Ca0.15)(Zr0.1Ti0.9)O3 lead-free piezoelectric ceramics, J. Am. Ceram. Soc., 2012, 95, 1998–2006 CrossRef CAS.
- G. H. Haertling and W. J. Zimmer, An analysis of hot-pressing parameters for lead zirconate lead titanate ceramics containing two atom percent bismuth, Am. Ceram. Soc. Bull., 1966, 45, 1084–1089 CAS.
- P. Jarupoom, T. Tunkasiri, K. Pengpat, S. Eitssayeam and G. Rujijanagul, Effects of annealing time on ferroelectric and piezoelectric properties of B2O3 doped Ba(Zr0.07Ti0.93)O3 ceramics, Ferroelectrics, 2011, 415, 88–93 CrossRef CAS.
- B. M. Jin, J. Kim and S. C. Kim, Effects of grain size on the electrical properties of PbZr0.52Ti0.48O3 ceramics, Appl. Phys. A, 1997, 65, 53–56 CrossRef CAS.
- G. Ray, N. Sinha and B. Kumar, Environment friendly novel piezoelectric 0.94[Na0.8K0.2NbO3]-0.06LiNbO3 ternary ceramic for high temperature dielectric and ferroelectric applications, Mater. Chem. Phys., 2013, 142, 619–625 CrossRef CAS.
- C. C. Jin, F. F. Wang, Q. R. Yao, Y. X. Tang, T. Wang and W. Z. Shi, Ferroelectric, dielectric properties and large strain response in Zr-modified (Bi0.5Na0.5)TiO3-BaTiO3 lead-free ceramics, Ceram. Int., 2014, 40, 6143–6150 CrossRef CAS.
- Z. T. Li, H. Liu, H. C. Thong, Z. Xu, M. H. Zhang, J. Yin, J. F. Li, K. Wang and J. Chen, Enhanced temperature stability and defect mechanism of BNT-based lead-free piezoceramics investigated by a quenching process, Adv. Electron. Mater., 2019, 5, 1800756 CrossRef.
- A. B. Swain, S. D. Kumar, V. Subramanian and P. Murugavel, Influence of external electric field on the physical characteristics of lead free BZT-BCT piezoceramic, J. Alloys Compd., 2019, 787, 990–995 CrossRef CAS.
- B. H. Chen, C. L. Huang and L. Wu, Promotion of piezoelectric properties of lead zirconate titanate ceramics with (Zr,Ti) partially replaced by Nb2O5, Solid-State Electron., 2004, 48, 2293–2297 CrossRef CAS.
- B. S. Kang, D. G. Choi and S. K. Choi, Effects of grain size on pyroelectric and dielectric properties of Pb0.9La0.1TiO3 ceramics, J. Mater. Sci.: Mater. Electron., 1998, 9, 139–144 CrossRef CAS.
- P. Jaita, S. Manotham and G. Rujijanagul, Influence of Al2O3 nanoparticle doping on depolarization temperature, and electrical and energy harvesting properties of lead-free 0.94(Bi0.5Na0.5)TiO3-0.06BaTiO3 ceramics, RSC Adv., 2020, 10, 32078–32087 RSC.
- A. Ullah, C. W. Ahn, K. B. Jang, A. Hussain and I. W. Kim, Phase transition and electrical properties of BiAlO3-modified (Bi0.5Na0.5)TiO3 piezoelectric ceramics, Ferroelectrics, 2010, 404, 167–172 CrossRef CAS.
- K. Yoshii, Y. Hiruma, H. Nagata and T. Takenaka, Electrical properties and depolarization temperature of (Bi1/2Na1/2)TiO3-(Bi1/2K1/2)TiO3 lead-free piezoelectric ceramics, Jpn. J. Appl. Phys., 2006, 45, 4493–4496 CrossRef CAS.
- B. P. Zhang, J. F. Li, K. Wang and H. Zhangy, Compositional dependence of piezoelectric properties in NaxK1-xNbO3 lead-free ceramics prepared by spark plasma sintering, J. Am. Ceram. Soc., 2006, 89, 1605–1609 CrossRef CAS.
- Z. Chen, J. Hu and X. He, Piezoelectric and dielectric properties of (Na0.5K0.5)NbO3-Bi0.5(Na0.8K0.2)0.5TiO3 lead-free ceramics, J. Ceram. Soc. Jpn., 2008, 116, 661–663 CrossRef CAS.
- Z. C. Rong and C. L. Yuan, Dielectric and piezoelectric properties of Bi0.5(Na0.82K0.18)0.5TiO3-LiSbO3 lead-free piezoelectric ceramics, Bull. Mater. Sci., 2011, 34, 933–936 CrossRef.
- A. Maqbool, J. Rahman, A. Hussain, J. K. Park, T. G. Park, J. S. Song and M. H. Kim, Structure and temperature dependent electrical properties of lead-free Bi0.5Na0.5TiO3-SrZrO3 ceramics, IOP Conf. Ser.: Mater. Sci. Eng., 2014, 60, 012047 CAS.
- K. Ohbayashi, T. Matsuoka, K. Kitamura, H. Yamada, T. Hishida and M. Yamazaki, Lead-free piezoelectric (K,Na)NbO3-based ceramic with planar-mode coupling coefficient comparable to that of conventional lead zirconate titanate, Jpn. J. Appl. Phys., 2017, 56, 061501 CrossRef.
- Y. Yu, J. Wu, T. Zhao, S. Dong, H. Gu and Y. Hu, MnO2 doped PSN-PZN-PZT piezoelectric ceramics for resonant actuator application, J. Alloys Compd., 2014, 615, 676–682 CrossRef CAS.
- D. J. Shin and J. H. Koh, Comparative study on storing energy for (Ba,Zr)TiO3 and CuO-(Ba,Zr)TiO3 ceramics for piezoelectric energy harvesting applications, Ceram. Int., 2017, 43, S649–S654 CrossRef CAS.
- Y. J. Choi, M. J. Yoo, H. W. Kang, H. G. Lee, S. H. Han and S. Nahm, Dielectric and piezoelectric properties of ceramic-polymer composites with 0-3 connectivity type, J. Electroceram., 2013, 30, 30–35 CrossRef CAS.
- D. J. Shin, J. Kim and J. H. Koh, Piezoelectric properties of (1-x)BZT-xBCT system for energy harvesting applications, J. Eur. Ceram. Soc., 2018, 38, 4395–4403 CrossRef CAS.
- H. Zhao, Y. Hou, X. Yu, J. Fu, M. Zheng and M. Zhu, A wide temperature insensitive piezoceramics for high temperature energy harvesting, J. Am. Ceram. Soc., 2019, 102, 5316–5327 CrossRef CAS.
- B. Liu, P. Li, B. Shen, J. Zhai, Y. Zhang, F. Li and X. Liu, Simultaneously enhanced piezoelectric response and piezoelectric voltage coefficient in textured KNN-based ceramics, J. Am. Ceram. Soc., 2018, 101, 265–273 CrossRef CAS.
- U. De, K. R. Sahu and A. De, Ferroelectric materials for high temperature piezoelectric applications, Solid State Phenom., 2015, 232, 235–278 Search PubMed.
- H. Li, C. Tian and Z. D. Deng, Energy harvesting from low frequency applications using piezoelectric materials, Appl. Phys. Rev., 2014, 1, 041301 Search PubMed.
- P. Jarupoom, K. Pengpat and G. Rujijanagul, Enhanced piezoelectric properties and lowered sintering temperature of Ba(Zr0.07Ti0.93)O3 by B2O3 addition, Curr. Appl. Phys., 2010, 10, 557–560 CrossRef.
- P. Mishra and P. Kumar, Enhancement of dielectric properties of 0.2[BZT-BCT]-0.8[(1-x)epoxy-xCCTO] (x = 0.02, 0.04, 0.06, 0.08 & 0.1) composites for embedded capacitor and energy harvesting applications, J. Alloys Compd., 2014, 617, 899–904 CrossRef CAS.
- D. J. Shin and J. H. Koh, A micro pattern of fine scale PZT ceramic structures for the energy harvester, Int, Ferroelectrics, 2012, 140, 84–91 CrossRef CAS.
- P. Gowdhaman, V. Annamalai and O. P. Thakur, Piezo, ferro and dielectric properties of ceramic-polymer composites of 0-3 connectivity, Ferroelectrics, 2016, 493, 120–129 CrossRef CAS.
- D. J. Shin, W. S. Kang, J. H. Koh, K. H. Cho, C. E. Seo and S. K. Lee, Comparative study between the pillar and bulk-type multilayer structures for piezoelectric energy harvesters, Phys. Status Solidi A, 2014, 211, 1812–1817 CrossRef CAS.
- P. Wannasut, P. Jaita, M. Promsawat and A. Watcharapasorn, Enhanced electrical properties of new lead-free 0.995Bi0.5(Na0.80K0.20)0.5TiO3-0.005LiNbO3 ceramics by (Ba0.98Nd0.02)TiO3 doping, J. Asian Ceram. Soc., 2021, 9, 1345–1353 CrossRef.
- Y. H. Kwon, D. J. Shin and J. H. Koh, (1-x)(Bi,Na)TiO3-x(Ba,Sr)TiO3 lead-free piezoelectric ceramics for piezoelectric energy harvesting, J. Korean Phys. Soc., 2015, 66, 1067–1071 CrossRef CAS.
|
This journal is © The Royal Society of Chemistry 2023 |
Click here to see how this site uses Cookies. View our privacy policy here.