DOI:
10.1039/D2RA07412E
(Review Article)
RSC Adv., 2023,
13, 18715-18733
Applications of palladium-catalyzed C–N cross-coupling reactions in pharmaceutical compounds
Received
22nd November 2022
, Accepted 17th May 2023
First published on 20th June 2023
Abstract
C–N cross-coupling bond formation reactions have become valuable approaches to synthesizing anilines and their derivatives, known as important chemical compounds. Recent developments in this field have focused on versatile catalysts, simple operation methods, and green reaction conditions. This review article presents an overview of C–N cross-coupling reactions in pharmaceutical compound synthesis reports. Selected examples of N-arylation reactions of various nitrogen-based compounds and aryl halides are defined for preparing pharmaceutical molecules.
1. Introduction
Pharmaceutical industries have undergone a wide variety of developments regarding new forms of active ingredients and their advances in formulation and preparation technology.1,2 The development of tiny molecular drugs is a crucial driver of sales growth for large pharmacies and defines new research and development patterns for pharmaceutical firms.3–11 Pharmaceutical industries strive to produce safe and inexpensive drugs while keeping pace with the pharmaceutical market. In this regard, investigating new synthetic approaches like “transition metal catalyzed reactions” for rapid access to massive hybrid libraries with facile and cost-effective operating methods is valuable.12 During this time, cross-coupling reactions as significant synthetic transformations have revealed an abundance of uses in the generation of several biologically active compounds and natural compounds;13,14 for instance, extensive studies have been reported on the application of Pd-based catalysts in the synthesis of critical medicinal compounds.15–19 These encompass two different starting materials with active groups which reacted to each other in the presence of metal species catalysts.20
Amine is one of the most important functional groups in natural materials and pharmaceuticals; therefore, C–N bond formation reactions have attracted much attention. Ullmann and Goldberg introduced the C–N bond formation through the SNAr reaction in the presence of copper salts suffering from serious disadvantages, such as poor substrate scope restricting its effectiveness in producing complex pharmaceuticals. These reactions need an activated functional group in the reactant, polar aprotic solvents, and a long reaction time. After that, Buchwald and Hartwig developed a C–N cross-coupling reaction using Pd-catalysts,21–24 more improvements converted this reaction into a decent strategy in synthetic chemistry through the amination reaction of aryl, vinyl, and heteroaryl halides.25–35 The constant development of substrate scope, ligands, and catalytic systems led to the generality of the N-arylation reaction. Now, many scientific reports on its utility and application are available.36–39 The general mechanism of Pd-catalyzed C–N cross-coupling reaction, as described in Scheme 1, includes oxidative addition, insertion, transmetalation, and reductive elimination.40–42
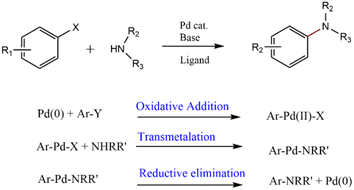 |
| Scheme 1 General reaction scheme of Buchwald–Hartwig amination reaction. | |
C–N bond formation through Pd-catalyzed cross-coupling reactions has attracted much attention in scientific efforts and industry.43–45 Numerous approved drugs and applicable organic compounds have arylated amine functional groups. These cause significant development in employed catalytic systems comprising metal sources, ligands, and reaction performance techniques.46–49 Different applications of this transformation in diverse scientific fields have inspired scientists to design new efficient ligands and study optimization reaction conditions to reach more efficient catalytic systems. Therefore, Pd-catalyzed coupling of amine with aryl halides was introduced as a pioneer of C–N bond formation reactions and promising alternating method to other traditional procedures, including nucleophilic aromatic substitution (SNAr) and Cu-catalyzed Ullmann and Goldberg couplings.50,51
Numerous novel catalytic systems with excellent efficiency on hindered amines and low-activity compounds were achieved by developing structurally diverse ligands. For instance, phosphine ligands with distinct structural properties like large, bulky, and the sterically hindered group can perform the coupling of massive kinds of amines such as heterocyclic, primary, secondary, and electron-deficient with aryl halides or heteroaryl halides.52,53 However, the investigation of the effects of the type of ligands and the detail of reaction conditions are not included in this review article to avoid the excessive length of the text.
In recent years, these transformations have attracted much attention, especially in drug design approaches, considering the point that lipophilicity of the molecules can be modulated by the inclusion of nitrogen atoms in the molecular structure, which improves their pharmacokinetic profile such as solubility, permeability, and brain penetration.54,55 These facts converted metal-catalyzed C–N formation reactions to general approaches and reliable procedures, especially in a systematic structure–activity relationship (SAR) exploration and medicinal chemistry efforts. Several review articles have been published about several cross-coupling reactions' usage in medicinal chemistry, emphasizing reaction conditions of multistep synthesis processes that have made the texts tedious and too long. Herein, this article provides a comprehensive report on applications of N-arylation reactions for the preparation of potential pharmaceutical compounds by depicting just their chemical structure and bolding newly formed bonds which made the text brief and useful.
Almost all available industrial synthesis methodologies contain C–N cross-coupling reactions employing Pd-based catalysts, and phosphine ligands are considered a limitation for them because of bearing ppm level threshold of Pd residual tolerance in active pharmaceutical ingredients and using expensive, unstable, and poisonous phosphine ligands.56 Therefore, researchers have turned their attention toward employing less expensive, less toxic, and more effective metals as an alternative to Pd. Recently, numerous approaches have been reported for the C–N cross-coupling reaction in the presence of more sustainable and greener metal, base, solvent, and milder reaction conditions, which are valuable from the green chemistry point of view.57–63
2. C–N cross-coupling reactions in pharmaceutical compounds
2.1. Primary alkylamine arylation
Primary alkylamine arylation is established as one of the most widely technologically advanced N-arylation reactions. Overcoming some drawbacks has converted this reaction into an efficient synthesis method for generating numerous pharmaceutical products.64–69 The chemical structure of some drugs synthesized by harnessing primary alkylamines arylation reaction has been illustrated in Scheme 2. For instance, several 3,7-disubstituted tricyclic imipramine 1, as an antidepressant agent, were synthesized by Jensen and co-workers through a one-pot procedure from bis-aryl bromides, microwave irradiation improved the reaction yields and reduced the production of an undesired product of monoamination.70 Microwave heating was also used by Grøtli and co-workers to generate some chromone derivatives 2, by the C–N cross-coupling reaction, products showed potential anti-cancer activity.71 Hsp90 inhibitors are known as potential antitumor agents; Gopalsamy and colleagues synthesized compound 3 via C–N coupling of linear alkyl primary amines and aryl iodide as a new Hsp90 inhibitor.72 DGAT-1 inhibitor 4 was prepared by Dow's team for obesity or type II diabetes treatment.73 In another research work, aryl bromide was coupled with aminoazetidine to reach compound 5 as a protein kinase Cθ inhibitor, and its activity as an autoimmune disease drug was also investigated.74 In continuation of the arylation reactions of primary alkylamines, a group of indazol-4-one-derived 6 was prepared through N-arylation of cyclohexyl amine by Huang and co-workers. Their anti-cancer activities were also confirmed as potential Hsp90 protein inhibitors.75 δ-Opioid agonist 7 was synthesized through two sequential C–N bond-formation reactions.76 In another scientific report, compound 8 was prepared through N-arylation of chiral amino-piperidine. More investigations exhibited that this compound acts as an anti-inflammatory agent.77 Ozenoxacin is an approved antibiotic for treating contagious skin infections, it is also effective against various kinds of bacteria.78 A successful synthetic procedure was described for generating ozenoxacin 9 through a C–N bond formation reaction catalyzed by Pd. Cyclopropanamine coupled to aryl bromide in the first step, followed by a Stille coupling reaction to reach the final product.79 The chemical structure of these compounds is illustrated in Scheme 3.
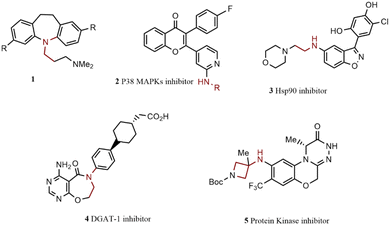 |
| Scheme 2 Bioactive compounds synthesizing from primary alkylamine arylation. | |
 |
| Scheme 3 More bioactive compounds synthesizing from primary alkylamines arylation. | |
2.2. Bioactive compounds containing piperidine
Piperidine is one of the most regular nitrogen heterocycles in pharmaceutical compounds.80 The chemical structure of some medicinal compounds synthesized using piperidine is depicted in Scheme 4. Player and co-workers prepared a series of carboxamide piperidines 10 by using a weak base that avoids side product creation. The activity of these compounds in pain management has been approved.81 Tetrahydroquinoline 11 is a potential anti-depressant medicine prepared by coupling aryl iodide with protected piperidine.82 Melatonin receptor ligand 12, as an agent for treating sleep disorders, was also synthesized via coupling of amide-substituted piperidine.83
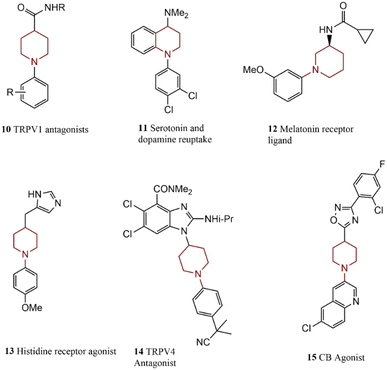 |
| Scheme 4 Bioactive compounds containing piperidine. | |
The Pd-catalyzed coupling of piperidine and 4-bromoanisole for preparing 13 acting as an H3 receptor agonist and potential agent against anxiety disorders and inflammation was presented.23 The reaction of benzimidazole-based piperidine gave a TRPV4 antagonist 14; its efficiency against lung injuries has been studied. Another research work; synthesized a CB2 agonist 15 as a pain management agent via a selective N-arylation reaction.84
2.3. Pharmaceuticals compounds containing pyrrolidine, pyrrolidine-2-one, azepine, and piperidine
The most abundant nitrogen-containing heterocycles in pharmaceuticals include pyrrolidine, pyrrolidine-2-one, azepine, and piperidine. The chemical structure of some synthesized compounds comprises them are illustrated in Scheme 5. Ernst et al. discovered an indoline-based heterocyclic compound 16 acting as an anti-urinary infection.85 Lachance and co-workers synthesized an agent 17 for treating diabetes through bispyrrolidine coupling reaction by taking advantage of the protection process to achieve selectivity in C–N bond formation transformation.86
 |
| Scheme 5 Bioactive compounds containing pyrrolidine, azepine, piperidine, and pyrrolidin-2-one. | |
A P2Y1 receptor agonist 18 used for the treatment of thrombosis was prepared by employing spiro-indoline in the presence of a special catalytic system.87 Moreover, a nicotinic acetylcholine receptor agonist 19 was also prepared by employing spiro-pyrrolidines. The therapeutic effect of this compound was studied in neurodegenerative and schizophrenia diseases.88 Compound 20 was produced through the coupling of azepine, its activity in the treatment of peripheral was also explored.89 Tomassoli and co-workers coupled a di-aza-bi-cyclic compound with 3-bromopyridine under microwave irradiation 21, in which one nitrogen protection caused to mono-arylation reaction.90 Compound GDC-0022 22 is a potential inhibitor of orphan receptor γ (RORγ) containing two chiral centers; therefore, its production route needs to be mild.
Previously reported procedures, including N-arylation reactions catalyzed by Pd, suffered from problems, such as low yield, formation of undesired side products, and a very challenging purification process. Sirois and co-workers improved available Pd-catalyzed N-arylation methods by optimizing various reaction conditions, such as the kind of solvent and base that succussed in overcoming drawbacks and achieve excellent conversion yields.91 Popovici-Muller and co-workers discovered compound AG-120 23 named ivosidenib,92 as a specific IDH1 (the isocitrate dehydrogenase-1) inhibitor, a mutation that overexpresses in numerous kinds of cancers. The presented multistep synthetic process includes an N-arylation reaction in the last step. An enantiomerically pure product was achieved easily by crystallization. Due to the success of large-scale synthesis, further clinical investigations are in progress.
2.4. Bioactive compounds containing piperazine or morpholine
Another abundant nitrogen-contained heterocycle compound is piperazine which is found as a part of numerous biologically active materials. Piperazine rings often are functionalized in the N1- and N4-positions using protected or functionalized versions. Compound 24 (Scheme 6) was synthesized through the reaction of aryl bromide and piperazine in polar media and harsh reaction conditions as an intermediate in preparing anticancer treatment agents.93 Park et al. reported functionalization of unprotected piperazine in less crowded nitrogen. Two C–N bond formation reactions were performed in the overall synthesis process. They also investigated the efficiency of product 25 in the treatment of type II diabetes.94
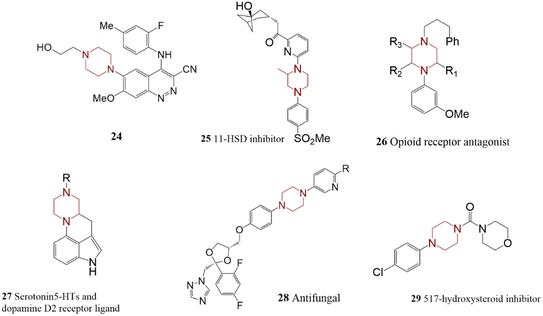 |
| Scheme 6 The chemical structure of some biologically active compounds synthesized via the coupling of piperazine. | |
To couple piperazine in more crowded nitrogen, Carroll and co-workers had to protect it; in continuous, protecting agent was removed, and an opioid receptor antagonist 26 was achieved.95 Compound 27 containing piperazine has exhibited acceptable activity against schizophrenia and Parkinson's disease. Kehler and co-workers succeeded in its synthesis through a cross-coupling reaction of 4-bromoindole and N-carbamate piperazine.96
In another research effort, the reaction of aryl bromide with a piperazine-based heterocyclic compound led to an antifungal agent 28 with excellent solubility properties.97 An approved antileukemia agent 29 was prepared by harnessing urea-containing piperazine's C–N bond formation reaction in one step of its synthesis process.98 Tang and co-workers synthesized some derivatives of piperazines such as compound 30 through the coupling of 4-bromobenzamide with functionalized piperazine by using a Pd catalyst. The product is a candidate for the treatment of type II diabetes by acting as MCD (malonyl-CoA decarboxylase) inhibitor.99 Batey et al. prepared a fluoroquinolone as an antibacterial agent, by applying cyclopropane ring-fused piperazine.100 These modifications improve the piperazine core's chemical and biological properties. In the same way, a potential B-Raf kinase inhibitor 31, as an anti-tumor treatment candidate, was generated by Wang and co-workers via an N-arylation reaction.101 The coupling of indoles with piperazinone led to the preparation of compound 32, a potential triple reuptake inhibitor and anti-depression agent.102 In another research effort, the reaction of 1,4-dibenzodiazepine and 4-iodobenzotrifluoride was employed for the synthesis of 33 by Tafesse and co-workers, and the effectiveness of the product as a painkiller was investigated.103 In studies for discovering orally bioavailable treatment agents, Ku et al. succussed in the synthesis of venetoclax 5 34. The reported procedure comprising Pd-catalyzed Buchwald–Hartwig coupling reaction between aryl bromide derivatives and piperazine derivatives led to large-scale and high-yield products. Venetoclax was introduced as suitable medicine for the treatment of chronic lymphocytic leukemia.104–106
Brexpiprazole 35 is an approved drug for the treatment of schizophrenia.107 Brexpiprazole was generated through an N-arylation reaction between 4-bromobenzo-[b]-thiophene and piperazine employing Pd catalyst. This reaction was performed at a large scale with excellent yields of product.108
Lei and co-workers discovered a new process for the synthesis of autotoxin inhibitor ziritaxestat 36, a potential drug for the treatment of chronic obstructive pulmonary. In this technique, through widespread screening, a piperazine-free base was used instead of N-Boc piperazine, omitting the re-protective step and improving the overall reaction efficiency are considered the strengths of the presented method.109 Morpholine is one of the other common heterocyclic moieties in commercially available drugs. The chemical structure of some biologically active compounds containing piperazine and morpholine has depicted in Scheme 7. A thiazine and morpholine-based N-heterocycle compound 37 was synthesized through C–N coupling reactions by harnessing a Pd-based catalyst. This new compound and its congeners were introduced as efficient potential anticancer agents.110 In the exploration of medulloblastoma therapy, C–N cross-coupling of morpholine was applied to provide some antagonists molecules such as compound 38.111
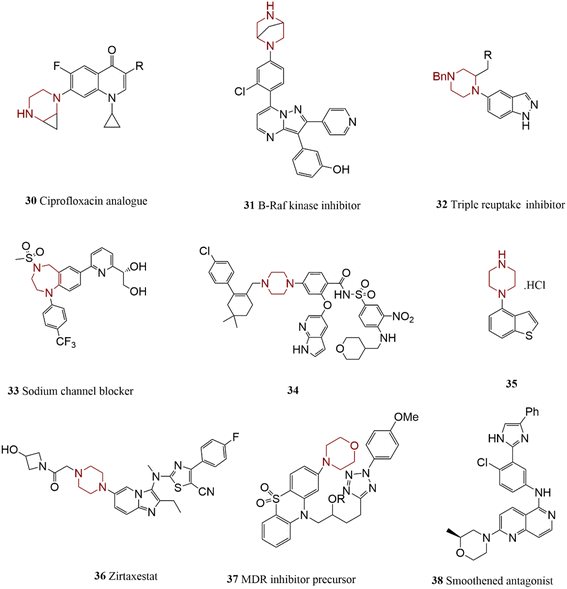 |
| Scheme 7 Bioactive compounds containing piperazine or morpholine. | |
2.5. Bioactive compounds containing piperazinen and aniline
Several B-RafV600E inhibitors with excellent selective anticancer activity have been synthesized.112 One of the most promising candidates 39 was generated via the reaction of functionalized anilines and quinazolinone by using Pd-catalyst. In another research report, cycloaddition and C–N coupling reactions were employed to synthesize potent Rac1 inhibitors, including norbornene unit 40.113 In this work, various stereoisomers of aniline were reacted with aryl iodide, and compound 41 was recognized as the most biologically active case. The relationship between chemical structure and biological activities was evaluated, and p-anisidine was coupled with different enantiomers of aryl triflate. The anticancer properties of these synthesized compounds were studied and compared. The chemical structure of more promising compounds, comprising functionalized anilines, has been depicted in Scheme 8, 42.114,115 This coupling reaction was harnessed to synthesize a series of potentially β2-agonists agents 43, acting against respiratory disorders.116 A candidate for inflammatory diseases treatment 44 was synthesized via coupling of a tricyclic core with diverse anilines, the product including a biologically stable dibenzoxepinones unit.117 A serious TRK inhibitor was prepared, acting as a tumor growth inhibition factor 45. Pd catalytic system was employed for the performance of aniline coupling.118
 |
| Scheme 8 Bioactive compounds containing aniline. | |
One alternative process of coupling heteroaryl amines with aryl halides is the reaction between hetero-aryl halides and anilines which is valuable when low conversion is achieved, or precursors are not available. A series of antitumor compounds were synthesized by Beria and co-workers by using the coupling of anilines with a hetero-aryl halide consuming a catalytic system including Pd species and specific ligands.119
In searching for new antileukemia, DNMT inhibitor agents, compound 46 was discovered and synthesized through a multistep synthesis route including SNAr reactions, low conversion, and harsh reaction conditions motivated researchers to employ a more efficient strategy. Therefore, Pd-catalyzed C–N cross-coupling reactions of 4-chloroquinoline and ethyl 4-aminobenzoate were carried out, and excellent yield of the desired product without any side reactions was achieved besides other benefits of this new synthesis technique.120
Pd-catalyzed arylation of 1,2-dichloropyrazine led to the generation of compound 47, identified as a BRAF inhibitor agent.121 Wurz and co-workers explored the reaction of pyridazine and substituted aniline; they introduced compound 48 as an anti-inflammatory agent.122 This strategy can use to generate a large number of similar compounds. This route was applied to synthesize compound 49 via an N-arylation reaction; this compound acts as a Nek2 inhibitor.123
The reaction of o-nitroaryl iodide with substituted aniline to reach RXR partial agonist 50, as an example of a new group of anti-diabetes drugs, was represented by Kakuta and co-workers.124 Similarly, a liver X receptor agonist 51 was prepared by Bernotas and co-workers through an N-arylation reaction.125 A series of pjDHFR inhibitors, potential anti-infection drugs, were synthesized by Gangjee and colleagues by employing a wide range of substituted N-methyl anilines coupling with aryl bromide. Compound 52 was identified as the most potent analog in this series.126 The chemical structure of some N-arylation products is depicted in Scheme 9.
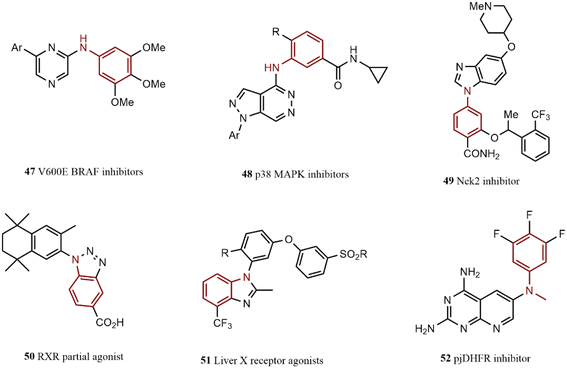 |
| Scheme 9 Bioactive compounds containing N-arylation products. | |
2.6. Biologically active compounds containing 2-aminopyridine
Several biologically active compounds containing 2-aminopyridines generated by the harnessing of C–N cross-coupling reaction have been illustrated in Scheme 10. In studies about anti-cancer promising drugs, medicinal chemists have reached a group of selective Mps1 inhibitors produced by the selective reaction of substituted 2,4-diaminopyridine and methyl 4-iodobenzoate in which compound 53 was identified as the most effective analog.127
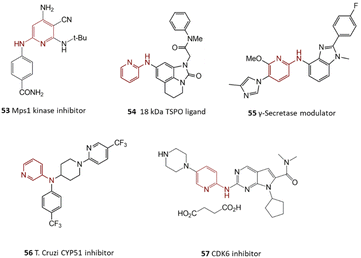 |
| Scheme 10 Bioactive compounds containing 2-aminopyridine. | |
In another research effort, the reaction of 2-aminopyridine with polycyclic aryl bromide was investigated by Fukaya and co-workers. Product 54 was found as a promising agent for the treatment of psychiatric disorders.128 Moreover, the final product of a coupling of pyridine and substituted-aryl bromide led to the formation of γ-secretase modulator 55 acting as an anti-Alzheimer drug.129 Compound 56 was distinguished as one of the promising agents for the treatment of Chagas disease. Trisubstituted amine was synthesized by using commercially accessible precursors via a C–N cross-coupling reaction of secondary 3-aminopyridine and substituted aryl bromide catalyzed by Pd-based homogenous species. It was found that the order of steps and protecting processes in total synthesis are critical factors to achieving success in this synthesis strategy.130 Ribociclib 57 was recognized as a CDK6 inhibitor agent and is used for the treatment of breast cancer in combination with aromatase inhibitors like letrozole. Various preparation techniques for its preparation are available; however, a significant development was archived via microwave-assisted C–N bond formation reaction between substituted piperazine and substituted 2-chloro-pyrimidine employing a Pd catalyst.131 Abemaciclib 58 is an approved cyclin-dependent kinase (CDK) inhibitor that could be used against breast cancer cells. The industrial synthesis approach of abemaciclib contains a Pd-catalyzed Suzuki and a C–N bond cross-coupling reaction.
Screening various reaction conditions achieved excellent optimization that led good conversion yield of the final product.132–134 In continuation, paying attention to the goals of green chemistry encouraged researchers to investigate abemaciclib synthesis by Pd-free catalytic systems and in more sustainable reaction conditions. Successful outcomes of magnetic cobalt-catalyzed C–N coupling in the abemaciclib preparation process have opened new horizons in the development of green chemistry in medicinal chemistry.135,136
2.7. Bio-compounds containing 2-aminopyrimidines
2-Aminopyrimidines coupled with aryl bromides have constructed some medicinal compounds. A series of B-Raf inhibitor agents Vasbinder and co-workers were synthesized through the microwave-assistance coupling of 2-aminopyrimidine with enantiomerically pure aryl bromide, a more promising analog marked as 59.137
Jones and co-workers coupled some imidazole to pyrimidine, the products introduced as potential anticancer drugs, the structure of more efficient compounds are given in Scheme 11, 60, 61.138,139 More examples of 2-aminopyrimidine containing heterocyclic compounds are represented. Duffey and co-workers generated some compounds with the tricyclic core as antitumor polo-like kinase inhibitors 62.140 Investigations have specified that coupling of aminopyrimidine with aryl bromide takes more advantages compared to other approaches such as condensing enamines and aryl guanidines. A Pd-catalyzed C–N bond formation reaction using Pd and supported ligands was used for the preparation of some tumor growth inhibitors such as 63.141
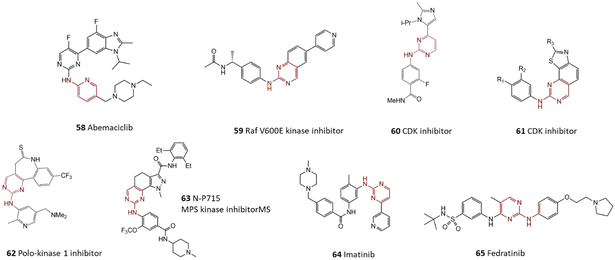 |
| Scheme 11 Bioactive compounds containing 2-aminopyrimidines. | |
In the same way, imatinib 64 was synthesized through a coupling reaction of aryl bromide and 2-aminopyrimidine in typical reaction conditions including the Pd complex, a solvent that can solve the reagents with excellent high reaction temperatures tolerate. Afterward, this synthesis route was improved as a two-step synthesis process using a lower Pd amount.142 Fedratinib 65 was known as a Janus kinase 2 (JAK2) inhibitor, and its efficiency in the treatment of myelofibrosis has been approved.143
The chemical structure of fedratinib has depicted in Scheme 11. The synthesis procedure contains a Pd-catalyzed Buchwald–Hartwig amination reaction.144 Further, the fedratinib synthesis in Pd-free conditions was investigated. Moreover, in silico evaluations exhibited the potential antitumor activities of some derivatives.135
2.8. Bioactive compounds containing 1,2,4-triazin-3-amine and 1,3,5-triazine-2,4-diamine
Nucleophilic substitution reaction is a traditional way to functionalize 1,3,5-triazine. Afterward, a more advanced technique including a C–N bond formation reaction was used to obtain some complex substituted 1,3,5-triazine. Microwave-assistance N-arylation of 1-bromo-2-nitrobenzene was carried out by Chen and co-workers for the generation of a group of FAK inhibitor candidates with angiogenic effect compounds 66 and 67 (Scheme 12).145 Compound 67 was recognized as a potential VEGFR, Src kinase inhibitor, and anti-macular degeneration agent. It was prepared by cross-coupling 3-amino-1,2,4-triazine and aryl bromide.146
 |
| Scheme 12 Bioactive compounds containing 1,2,4-triazin-3-amine and 1,3,5-triazine-2,4-diamine. | |
2.9. Bioactive compounds containing thiophene-2-amine and furan-2-amine
Sulfur-containing five-membered heterocycles amines are one of the more stable heterocyclics with low participation of nitrogen in resonance contribution, this makes them ideal for facile C–N cross-coupling reactions. Thiophene-based intermediates such as 68 were synthesized as PLK1 inhibitors under mild reaction conditions.147 The employed procedure exhibited good selectivity and excellent overall efficiency; product 69 can be used potentially as anti-schizophrenia. Compound 70 was generated via the reaction of aminothiophene with 2-fluoronitrobenzene (Scheme 13).148
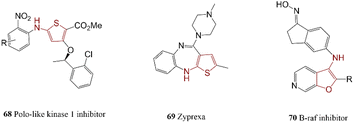 |
| Scheme 13 Bioactive compounds containing thiophene-2-amine and furan-3-amine. | |
2.10. Bioactive compounds containing imidazole, pyrazole and thiazole
Benzimidazole, imidazole, and thiazole are five-membered rings with two heteroatoms that are found frequently in biologically active molecules. C–N cross-coupling reaction of 5-aminopyrazoles and aryl bromides in the presence of a weak base and supported ligand led to the preparation of some compounds such as 71 and 72 which potentially improved the renal function.149
2-(Arylamino)thiazoles and 2-aminothiazoles containing nitrogen and sulfur atoms are other abundant five-membered heterocyclic compounds in the field of medicinal chemistry. 2-Amino(benzo)thiazoles can directly couple to aryl halide by employing a suitable catalytic system.150 In this regard, 2-arylaminobenzothiazoles 73 was synthesized as potent PARP14 inhibitors.151 In another research report, an effective synthesis approach for the preparation of an inhibitor of Epidermal Growth Factor Receptor (EGFR) T790 M 74 was presented which can use as a potential treatment for lung cancer.152 They employed palladacycle for the reaction of 1-methyl-1H-pyrazol-4-amine with a functionalized aryl halide. Li and co-workers synthesized BIIB068 75, a BTK (Bruton's Tyrosine Kinase) inhibitor that probably can act as a drug against autoimmune diseases.153 The presented efficient method employed for coupling reaction of 1-methyl-1H-pyrazol-4-amine with substituted 2-chloropyrimidine (Scheme 14).
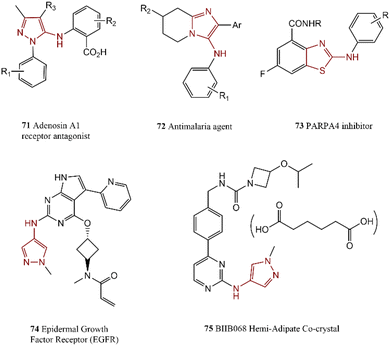 |
| Scheme 14 Bioactive compounds containing imidazole, pyrazole, and thiazole. | |
2.11. Bioactive compounds containing primary amide
A series of 3,4-diarylquinolinones was generated through primary amides coupling with aryl bromides and base-catalyzed aldol condensation.154 Among synthesized compounds, 76 gave the most effectiveness in inflammation treatment. In a research project to find new TYK2 inhibitors as anti-inflammatory agents, medicinal chemists reached compound 77.155 In the final step of its multistep reaction syntheses process, primary amide was coupled to aryl bromide. In the presented procedure, the reaction was carried out under microwave heating by applying a Pd complex, supported ligand, and weak base. A series of potent anticoagulant drugs such as 78 was prepared,156 similar to the previous report of cyclic amides and tricyclic oxazolidinone combination. The multistep synthesis contains Suzuki coupling, Miyaura borylation, and double ring closure including two intramolecular amidation reactions developed by Hergenrother and co-workers, and the synthesis of product 79 with potential anticancer activity was also reported.157 Nonoyama and co-workers synthesized a new renin inhibitor candidate 80.158 Plasma renin activity (PRA) has a significant role in hypertension pathogenesis, and PRA-inhibitor decreases the danger of myocardial infarction (Scheme 15).
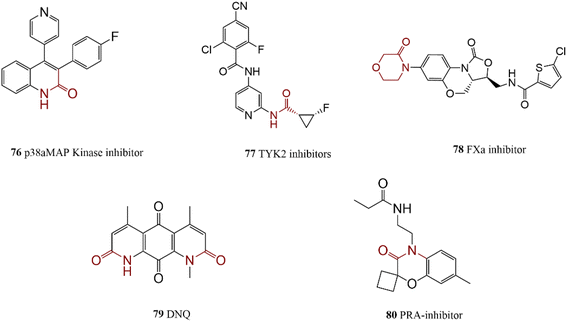 |
| Scheme 15 Bio-active compounds containing primary amide. | |
2.12. Bio-active compounds containing primary sulfamide
In the reported procedure, the benzoxazine core was generated through an intermolecular C–N cross-coupling reaction, after screening different reaction conditions, the reaction was performed in optimized conditions using Pd and Pt-based catalyst, xylene as a solvent, and K2CO3 as a base. The product dofetilide 81 is one of the FDA-approved antiarrhythmic medicine that was synthesized by Ruble et al. through a new procedure by employing Pd-catalyzed N-arylation reactions in two steps with an excellent yield of product at suitable industrial reaction conditions.159 A group of 8-sulfamide imidazopyrazines was synthesized by coupling p-toluenesulfonamide with various heteroaryl chlorides in the presence of Pd salt and heterogeneous ligands. Product 82 was introduced as a potent antibacterial agent.160 Secondary sulfonamides were coupled to dichloroisonicotinate in the initial steps of the total synthesis of nicotinamide 83, a candidate for Alzheimer's treatment (Scheme 16).161
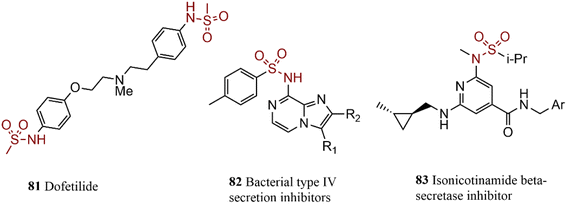 |
| Scheme 16 Bio-active compounds containing primary sulfamide. | |
2.13. Bio-active compounds containing urea
A group of modulators of PRMTs (protein arginine methyltransferases) was prepared by C–N cross-coupling of symmetrical ureas inhibitor 84. This excellent process was used for the synthesis of a structural unit of complex protease inhibitors. This method contains the coupling of chloropyrazinone with monosubstituted ureas using a Pd catalyst and specific bidentate ligands.162 Compound 85 was generated by applying this reaction to couple urea, as an inhibitor of hepatitis C virus (HCV) NS3 protease.163 Omecamtiv mecarbil 86 is unsymmetrical diaryl urea that was generated by Buchwald and co-workers in a two-step selective C–N coupling reaction using Pd salt and dialkylbiaryl phosphine ligand as a catalyst (Scheme 17).164
 |
| Scheme 17 Bio-active compounds containing urea. | |
2.14. Bioactive compounds synthesized via the coupling of protected amine
The protected aniline is used to prevent undesirable reactions during the synthesis process and can be removed in the final step. A group of 9-aminopentacyclines including 87 was prepared by Xiao and co-workers using Pd-catalyzed to connect polycycle amines where the protected group was removed in the last stage.165 Pd-catalyzed C–N cross-coupling reaction between benzophenone imine and aryl triflates was introduced as a successful strategy to gain amine derivatives. Herein compound 88 is a precursor of the total synthesis of an anti-heroin addiction.166 Similarly, 89 was generated to reach an opioid receptor antagonist.167 N-Arylation of LHMDS, lithium bis(trimethylsilyl)amide, applying a Pd-based catalyst, and dialkylbiarylphosphines ligand is known as one of the practical ways to synthesize primary anilines (Scheme 18).
 |
| Scheme 18 Bioactive compounds synthesized via the coupling of protected amine. | |
2.15. Bioactive compounds synthesized via N-arylation of LHMDS
Heteroarylamine was provided by the reaction of 2-bromopyridine and LHMDS in the initial step of total synthesis of compound 90 which was recognized as a c-Met inhibitor with potential activity against cancer cells.168 Heteroarylamines 91 and 92 were also generated by harnessing the reaction of 2-chloropyridines and LHMDS. Products are important precursors for the synthesis of valuable anticancer agents.169,170 The prodrug of antitumor seco-CBI-indole 93, containing a stable cyclic carbamate, was synthesized through primary aniline preparation by Pd-catalyzed reaction of Ph3SiNH2 and LHMDS.171 Lasmiditan 94 is an approved anti-migraine drug synthesized employing benzophenone in a C–N cross-coupling reaction in the presence of a Pd-catalyst. The following hydrolysis attained primary aniline.172 Technique solved the difficulties of primary aniline preparation besides the merits of mild reaction conditions.173 Product 95 exhibited selective 5-HT1F agonist activity with minimum side effects (Scheme 19).
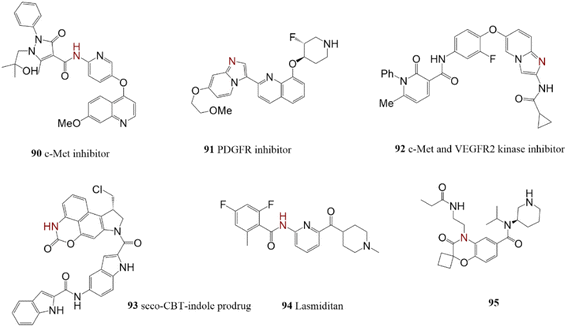 |
| Scheme 19 Bioactive compounds synthesized via N-arylation of LHMDS. | |
2.16. Bio-active compounds containing indazol, indole, and imidazole
In this regard, protected (s)-pyrrolidine-2-carboxamide was coupled to bis-aryl chloride derivative applying Pd catalyst during the synthesis of pibrentasvir 96. This compound was recognized as an effective anti-viral drug and employ in the treatment of hepatitis C patients.174
Pibrentasvir was commercialized in 2017 under the trade name of Mavyret. Qian and co-workers presented a method for the reaction of substituted aryl bromide with N,N-disubstituted hydrazines to produce 2-N-substituted imidazolines 97 as a candidate for the treatment of type II diabetes.175 Tomoo and co-workers described the successful C–N coupling of indole and aryl bromides by Pd catalyst. The product belongs to cytosolic phospholipase A2α inhibitors acting as an anti-inflammatory agent.176 Nilotinib 98, an approved drug for chronic myelogenous leukemia, has been prepared by arylation of unsymmetrical imidazoles catalyzed by a Pd-based catalytic system.177
Traditional SNAr reactions in the presence of Cu catalyst suffered from some disadvantages such as poor selectivity and low product yield that were resolved by this new strategy using imidazole in excess and aryl halide in low amounts. Regioselective C–N coupling of functionalized imidazole gave 99 in an excellent conversion method, an anti-leukemia drug.178 In another report, a series of benzimidazole derivatives were formed by a selective N-arylation reaction between 4-chloropyrimidine and benzimidazole, the final product 100 is known as a candidate for the treatment of neurodegenerative diseases (Scheme 20).179
 |
| Scheme 20 Bio-active compounds containing indazol, indole, and imidazole. | |
2.17. Bioactive compounds synthesized via N-arylation of sulfur-containing five-membered rings
The five-membered rings containing sulfur and nitrogen are found in many heterocyclic drugs. These compounds are also synthesized via C–N coupling reactions. Potent anti-inflammatory agent 101 was synthesized using the coupling of aryl bromide and benzophenone imine.180 In the same way, Canales and co-workers described the C–N coupling of benzophenone hydrazone alkyl hydrazines with halothiophenes.181 According to these reactions, a potential HCV inhibitor 102 and anticancer drug 103 were produced.182 C–N coupling of benzothiophene at the C3-position was also reported for the preparation of compound 104.183 Moreover, 2-bromothiazole was applied in a C–N coupling reaction where coupled with amides to generate a biologically active compound 105 (Scheme 21).
 |
| Scheme 21 Bioactive compounds synthesized via N-arylation of sulfur-containing five-membered rings. | |
2.18. Bio-active compounds containing other N-heterocyclic
MK-0429 is a potent pan-integrin inhibitor that successfully passed human clinical trials and has been introduced as anti-prostate cancer and anti-osteoporosis agent. A short and efficient synthesis method comprising the carbon–nitrogen bond formation process has been reported to reach 106.184 GDC-4379 is a well-known JAK1 inhibitor for the therapy of asthma. To synthesize, Stumpf et al. discovered a new method comprising condensation, nitro reduction by NaBH4 in the presence of copper, amidation, and C–N bond cross-coupling of pyrazole. Their reported method delivered GDC-4379 crystals 107 with a high yield of product and an easy purification process.185 A series of 4-tetrazolyl-substituted 3,4-dihydroquinazoline derivatives were prepared through cascade cross-coupling of azide-isocyanide and cyclization reaction in the presence of a Pd catalyst. Compound 108 was recognized as an efficient anti-breast cancer cell agent via broad biological investigations (Scheme 22).186
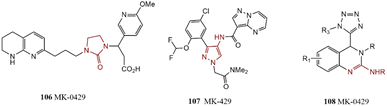 |
| Scheme 22 Bio-active compounds containing N-heterocyclic. | |
2.19. Biologically active compounds synthesized using LiHMDS
C–N coupling reactions of halo-imidazoles, -pyrazoles, -pyrroles, and their derivatives have attracted much attention due to the usefulness of the final products in medicinal chemistry; however, such these reactions suffer from some difficulties because of their free NH group. A p38 map kinase inhibitor 109 was synthesized by Bullington and co-workers as an anti-inflammatory agent. Pd catalysts promote the C–N coupling reaction of 2-bromopyrrole and acetylpiperazine in excellent yields.187 In another research report, epidermal growth factor receptor (EGFR) inhibitors such as 110 were synthesized through two following C–N coupling steps.188
N-Arylation reactions can be performed in haloimidazole in every position;189 for example, Roberge and co-workers presented a new procedure for the preparation of ceratamine A 111, a potential oncology medicine, via N-arylation of fused imidazole in the presence of Pd catalytic system by using LiHMDS/Ph3SiNH2 as ammonia source for conversion of 2-chloroimidazole to its primary aniline.190 Pd-catalyzed N-arylation of imidazopyridines led to the formation of tumor inhibitor drugs including 112 and 113.191 Moreover, a series of promising antimalarial agents 114 was synthesized via C–N cross-coupling reaction. Compound 115 is a small-molecule CDK4/6 inhibitor approved for the therapy of advanced-stage breast cancer. The Buchwald–Hartwig amination is an essential step in its synthesis process; LiHMDS was applied to the generation of protected amine without any impurities and by-products. Chloropicoline derivative formed a C–N bond via coupling with amide by employing a Pd catalyst and xantphos ligand (Scheme 23).192
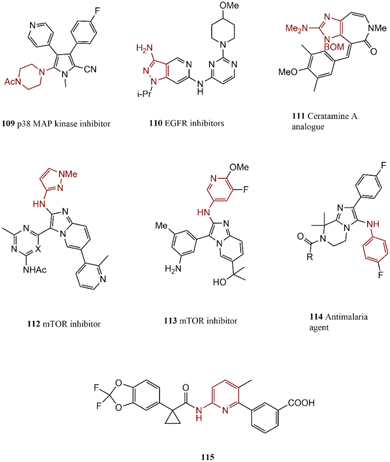 |
| Scheme 23 Biologically active compounds synthesized using LiHMDS. | |
2.20. More biologically active compounds constructed from amination reaction
Larghi and co-workers reported a development in Liebeskind–Srogl cross-coupling reaction in the presence of Pd catalysts as a facile process to access compounds containing purine. Compound 116 was identified as a biological active against PC-3 and MDA-MB231 cancer cell lines.193 A series of 2,3-diiodinated N-arylbenzimidamide derivatives was synthesized through a highly regioselective Buchwald–Hartwig amination reaction. Compound 117 was introduced as the most effective derivative as a potential compound for MDM2 and MDM4 activity-related disease therapy.194 Its large-scale synthesis comprises two main steps C–N bond formation and ring-closing metathesis reaction.
Furaprevir 118, 119 was found as a safe and effective drug in clinical trials phases I and II.195 Compound 120, MK-4688, is an HDM2–p53 protein–protein interaction inhibitor whose generation procedure involves a metal-catalyzed C–N bond formation reaction.196
One of the well-known inflammatory autoimmunity treatment options is an immunomodulatory enzyme, indoleamine 2,3-dioxygenase 1 (IDO1). IDO2 is its other homolog with a lower expression. However, researchers have revealed that IDO1/IDO2 dual inhibitors have very good in vitro inhibitory activity (IC50 = 112 nM) and it was introduced as a potential drug for rheumatoid arthritis. 121 was prepared on a large scale through a double C–N bond formation reaction in high yields.197
CDK7 has a role in transcription and the cell cycle of cancer cells; therefore, research on CDK7 inhibitors and their action mechanism has attracted much attention. A synthesis technique to gain SY-5609 122 was described based on C–N bond formation reactions. SY-5609 is an FDA-approved CDK7 inhibitor agent with high selectivity and metabolic stability.198
Zang and co-workers discovered a macrocyclic ATP citrate lyase inhibitor, which is a metabolic enzyme acting in fatty acid and cholesterol synthesis. Therefore, ATP citrate lyase inhibitor agents were identified as a candidate for the treatment of several metabolic diseases and malignancies. The biological evaluation confirmed the excellent binding affinity of this compound, leading to good inhibitory activity. Its synthesis contains a ring-closing C–N bond formation reaction.199
Fibroblast growth factor receptors (FGFRs) have a bold pattern in numerous cancers. Currently, the effectiveness of selective FGFRs-inhibitors is being evaluated in clinical trials as potential ant-cancer agents. Compound 122 was generated easily through a C–N bond formation reaction as an effective anti-FGFR agent (Scheme 24).200
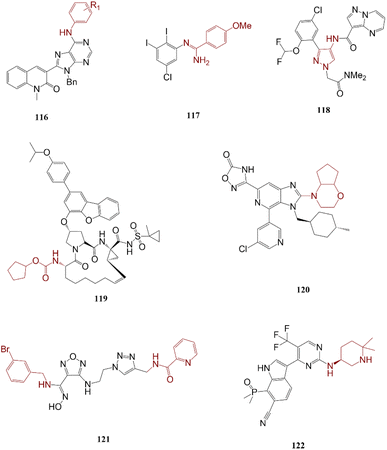 |
| Scheme 24 The chemical structure of biologically active compounds is constructed from an amination reaction. | |
2.21. Examples of N-contain pharmaceutical drug molecules synthesis routes
Herein, the most important pragmatical compounds synthesized through C–N cross-coupling reaction have been presented; however, considering the importance of reaction sequence and conditions some examples of synthesizing various pharmaceutical drug molecules of N-contain heterocyclic compounds have been reviewed. Developing synthesized methods to get effective drug compounds have converted them into a hot topic and their importance has excited researchers to investigate C–N bond formation as one of the most employed reactions.201–205 Tanatani and co-workers reported the arylation of N-protected 3-amino pyrroles to synthesize a five-membered heterocycle. The synthesized compound 123 was identified as an anti-tumor agent (Scheme 25). The employed catalytic system consists of Pd salt, a phosphorus ligand, and Cs2CO3 as the base leading to a good yield of product.206 In another research report, synthesis methods were described for the production of nitrogen-based heterocycles 124 via coupling primary alkylamines in the presence of a Pd-based catalyst. In the presented strategy, 2-bromothiophenol, a primary amine, and a functionalized 1-bromo-2-iodobenzene were coupled together by using a single catalyst (Scheme 26), further this procedure was applied to synthesize a series of disubstituted phenothiazines.207
 |
| Scheme 25 Synthesis route for preparation of functionalized N-contain heterocyclic. | |
 |
| Scheme 26 Synthesis rout for preparation of a series of phenothiazines. | |
Tetrahydroquinoline 125 was synthesized by employing N-Boc-protected piperidine, the synthesis route has been depicted in Scheme 27. This compound was recognized as an antidepressant agent.208
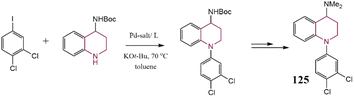 |
| Scheme 27 Synthesis route for the preparation of a functionalized tetrahydroquinoline. | |
By using a catalytic system consisting of Pd2(dba)3, ligand, and base, a series of alkyl and aryl amidines were coupled with aryl chlorides, bromides, and triflates. Notably, monoarylated products were gained as the main product by applying N-unsubstituted amidines. After optimization examination, the free base conditions were found as the best conditions. This procedure was used for the one-pot synthesis of 4-arylquinazolines, this transformation has been illustrated in Scheme 28. In the second step, electrocyclization was performed by DDQ oxidation, and 4-quinazolines 126 were generated in moderate yield. Several 4-arylquinazolines derivatives with diverse electronic natures were successfully prepared.209
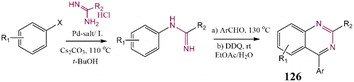 |
| Scheme 28 Synthesis route for the preparation of a 4-arylquinazolines. | |
In another research report, varied indazole quinazolinones were prepared through a one-step protocol. This process started with a reaction of the isatoic anhydride and hydrazine, generating an intermediate formed by trapping CO2, and further cyclization with o-iodobenzaldehyde led to the creation of quinazolinone. In the next step, Pd-catalyzed intramolecular coupling occurred. The final fused heterocycle 127 was obtained by air-oxidation reaction (Scheme 29).210
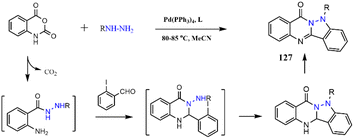 |
| Scheme 29 Schematic of one-step protocol synthesis of indazolo quinazolinones derivatives. | |
3. Conclusions
Over the past few decades, compounds containing nitrogen have been in high demand, which has encouraged the progress of C–N bond formation reactions catalyzed by metals in line with the production reactions of amines. This review article focused on published works that have demonstrated the academic and industrial valuable use of C–N coupling reactions in aniline derivatives synthesis. Numerous research areas on this topic have been attractive; however, herein application of this transformation in medicinal chemistry has been highlighted. It is anticipated that this article will help medicinal chemists working on C–N coupling reactions synthesize more potent structures with higher yields and lower side effects in treating either simple or more complicated diseases. We hope this review will inspire chemists to improve N-arylation conditions of more challenging synthetic reactions to reach novel high-tech pharmaceutical products. This inspires researchers to investigate the possibility of performing the C–N cross-coupling reaction on a large scale. The new wave of reaction performance in mild reaction conditions using lower-cost materials will lead to more eco-friendly and economic advantages in industrial processes. Palladium and traditional phosphine ligands are poisonous and precious; therefore, Pd-precursors with optimized reaction conditions or palladium-free catalysts have been widely considered. Moreover, studying and investigating the chemical structures of compounds with desirable biological activity can support medicinal chemists in new drug design processes.
Conflicts of interest
There are no conflicts to declare.
Notes and references
- M. C. Newman and W. H. Clements, Ecotoxicology: a comprehensive treatment, CRC Press, 2007 Search PubMed.
- K. G. Gadamasetti, Process Chemistry in the Pharmaceutical Industry, Marcel Dekker, New York, 1999, pp. 3–17 Search PubMed.
- J. G. Lombardino and J. A. Lowe, Nat. Rev. Drug Discovery, 2004, 3, 853–862 CrossRef CAS PubMed.
- P. Garner, P. B. Cox, U. Rathnayake, N. Holloran and P. Erdman, ACS Med. Chem. Lett., 2019, 10(11), 1512–1517 CrossRef.
- A. A. Noser, A. H. Abdelmonsef and M. M. Salem, Bioorg. Chem., 2019, 10, 106299 Search PubMed.
- N. A. Frolov and A. N. Vereshchagin, Int. J. Mol. Sci., 2023, 24, 2937 CrossRef CAS PubMed.
- G. F. Zha, K. P. Rakesh, H. M. Manukumar, C. S. Shantharam and S. Lon, Eur. J. Med. Chem., 2019, 162, 465–494 CrossRef CAS.
- M. Erdoğan and A. Daştan, Synth. Commun., 2020, 50, 3845–3853 CrossRef.
- M. M. Heravi and V. Zadsirjana, RSC Adv., 2020, 10, 44247–44311 RSC.
- T. N. Tran and M. Henary, Molecules, 2022, 27, 2700 CrossRef CAS PubMed.
- L. Mamani, R. Cruz, S. Mallqui and A. Catenazz, Diversity, 2022, 14, 215 CrossRef.
- J. Magano and J. R. Dunetz, Transition Metal-Catalyzed Couplings in Process Chemistry: Case Studies from the Pharmaceutical Industry, John Wiley & Sons, 2013 Search PubMed.
- F. Kakiuchi and T. Kochi, Synthesis, 2008, 2008, 3013–3039 CrossRef.
- S. Sultan and B. A. Shah, Chem. Rec., 2019, 19, 644–660 CrossRef CAS PubMed.
- P. Devendar, R.-Y. Qu, W.-M. Kang, B. He and G.-F. Yang, J. Agric. Food Chem., 2018, 66, 8914–8934 CrossRef CAS PubMed.
- Z.-Y. Huang, J.-F. Yang, K. Song, Q. Chen, S.-L. Zhou, G.-F. Hao and G.-F. Yang, J. Org. Chem., 2016, 81, 9647–9657 CrossRef CAS PubMed.
- Z.-Y. Huang, J.-F. Yang, Q. Chen, R.-J. Cao, W. Huang, G.-F. Hao and G.-F. Yang, RSC Adv., 2015, 5, 75182–75186 RSC.
- R.-Y. Qu, Y.-C. Liu, Q.-Y. Wu, Q. Chen and G.-F. Yang, Tetrahedron, 2015, 71, 8123–8130 CrossRef CAS.
- Y.-C. Liu, Z.-Y. Huang, Q. Chen and G.-F. Yang, Tetrahedron, 2013, 69, 9025–9032 CrossRef CAS.
- Y.-C. Liu, C.-J. Ye, Q. Chen and G.-F. Yang, Tetrahedron Lett., 2013, 54, 949–955 CrossRef CAS.
- J. Bariwal and E. Van der Eycken, Chem. Soc. Rev., 2013, 42, 9283–9303 RSC.
- P. Ruiz-Castillo and S. L. Buchwald, Chem. Rev., 2016, 116, 12564–12649 CrossRef CAS.
- A. Hosseinian, L. Zare Fekri, A. Monfared, E. Vessally and M. Nikpassand, J. Sulfur Chem., 2018, 39, 674–698 CrossRef CAS.
- F. Perez and A. Minatti, Org. Lett., 2011, 13, 1984–1987 CrossRef CAS.
- P. A. Forero-Cortés and A. M. Haydl, Org. Process Res. Dev., 2019, 23, 1478–1483 CrossRef.
- M. Kosugi, M. Kameyama and T. Migita, Chem. Lett., 1983, 12, 927–928 CrossRef.
- M. S. Driver and J. F. Hartwig, J. Am. Chem. Soc., 1997, 119, 8232–8245 CrossRef CAS.
- J. P. Wolfe, H. Tomori, J. P. Sadighi, J. Yin and S. L. Buchwald, J. Org. Chem., 2000, 65, 1158–1174 CrossRef CAS.
- X. Huang, K. W. Anderson, D. Zim, L. Jiang, A. Klapars and S. L. Buchwald, J. Am. Chem. Soc., 2003, 125, 6653–6655 CrossRef CAS PubMed.
- K. W. Anderson, R. E. Tundel, T. Ikawa, R. A. Altman and S. L. Buchwald, Angew. Chem., Int. Ed., 2006, 45, 6523–6527 CrossRef CAS PubMed.
- T. Ikawa, T. E. Barder, M. R. Biscoe and S. L. Buchwald, J. Am. Chem. Soc., 2007, 129, 13001–13007 CrossRef CAS PubMed.
- J. P. Wolfe, J. Åhman, J. P. Sadighi, R. A. Singer and S. L. Buchwald, Tetrahedron Lett., 1997, 38, 6367–6370 CrossRef CAS.
- X. Huang and S. L. Buchwald, Org. Lett., 2001, 3, 3417–3419 CrossRef CAS PubMed.
- S. K. Kashani, J. E. Jessiman and S. G. Newman, Org. Process Res. Dev., 2020, 24, 1948–1954 CrossRef CAS.
- D. A. Culkin and J. F. Hartwig, Acc. Chem. Res., 2003, 36, 234–245 CrossRef CAS.
- D. S. Surry and S. L. Buchwald, Chem. Sci., 2011, 2, 27–50 RSC.
- R. J. Lundgren and M. Stradiotto, Chem.–Eur. J., 2012, 18, 9758–9769 CrossRef CAS.
- A. Biffis, P. Centomo, A. Del Zotto and M. Zecca, Chem. Rev., 2018, 118, 2249–2295 CrossRef CAS PubMed.
- X. Chen, K. M. Engle, D. H. Wang and J. Q. Yu, Angew. Chem., Int. Ed., 2009, 48, 5094–5115 CrossRef CAS PubMed.
- G. P. McGlacken and I. J. Fairlamb, Eur. J. Org. Chem., 2009, 24, 4011–4029 CrossRef.
- K. L. Hull and M. S. Sanford, J. Am. Chem. Soc., 2009, 131, 9651–9653 CrossRef CAS PubMed.
- F. Diederich and P. J. Stang, Metal-catalyzed cross-coupling reactions, John Wiley & Sons, 2008 Search PubMed.
- C. Torborg and M. Beller, Adv. Synth. Catal., 2009, 351, 3027–3043 CrossRef CAS.
- J. P. Corbet and G. Mignani, Chem. Rev., 2006, 106, 2651–2710 CrossRef CAS PubMed.
- B. Schlummer and U. Scholz, Adv. Synth. Catal., 2004, 346, 1599–1626 CrossRef CAS.
- D. S. Surry and S. L. Buchwald, Chem. Sci., 2011, 2, 27–50 RSC.
- J. F. Hartwig, Nature, 2008, 455, 314–322 CrossRef CAS PubMed.
- J. Bariwal and E. Van der Eycken, Chem. Soc. Rev., 2013, 42, 9283–9303 RSC.
- R. J. Lundgren and M. Stradiotto, Chem.–Eur. J., 2012, 18, 9758–9769 CrossRef CAS PubMed.
- I. P. Beletskaya and A. V. Cheprakov, Organometallics, 2012, 31, 7753–7808 CrossRef CAS.
- C. Fischer and B. Koenig, Beilstein J. Org. Chem., 2011, 7, 59–74 CrossRef CAS PubMed.
- J. F. Hartwig, M. Kawatsura, S. I. Hauck, K. H. Shaughnessy and L. M. Alcazar-Roman, J. Org. Chem., 1999, 64, 5575–5580 CrossRef CAS PubMed.
- J. F. Hartwig, Angew. Chem., Int. Ed., 1998, 37, 2046–2067 CrossRef CAS PubMed.
- M. J. Buskes and M.-J. Blanco, Molecules, 2020, 25, 3493–3515 CrossRef CAS PubMed.
- R. Dorel, C. P. Grugel and A. M. Haydl, Angew. Chem., Int. Ed., 2019, 58, 17118–17129 CrossRef CAS PubMed.
- G. Burton, P. Cao, G. Li and R. Rivero, Org. Lett., 2003, 5, 4373–4376 CrossRef CAS PubMed.
- M. Nasrollahzadeh, A. Rostami-Vartooni, A. Ehsani and M. Moghadam, J. Mol. Catal. A: Chem., 2014, 387, 123–129 CrossRef CAS.
- M. Sajjadi, M. Nasrollahzadeh and H. Ghafuri, J. Organomet. Chem., 2021, 950, 121959 CrossRef CAS.
- M. Nasrollahzadeh, N. Motahharifar, M. Sajjadi, A. Naserimanesh and M. Shokouhimehr, Inorg. Chem. Commun., 2022, 136, 109135 CrossRef CAS.
- M. Nasrollahzadeh, S. M. Sajadi and M. Khalaj, RSC Adv., 2014, 4, 47313–47318 RSC.
- M. Nasrollahzadeh, B. Jaleh, P. Fakhri, A. Zahraei and E. Ghadery, RSC Adv., 2015, 5, 2785–2793 RSC.
- S. E. Hooshmand, B. Heidari, R. Sedghi and R. S. Varma, Green Chem., 2019, 21, 381–405 RSC.
- V. Polshettiwar and R. S. Varma, Green Chem., 2010, 12, 743–754 RSC.
- V. Polshettiwar and R. S. Varma, Chem. Soc. Rev., 2008, 37, 1546–1557 RSC.
- A. R. Hajipour, Z. Khorsandi and S. F. M. Metkazini, J. Organomet. Chem., 2019, 899, 120793 CrossRef CAS.
- A. R. Hajipour, M. Check and Z. Khorsandi, Appl. Organomet. Chem., 2017, 31, e3769 CrossRef.
- A. R. Hajipour, Z. Khorsandi, Z. Abeshtiani and S. Zakeri, J. Inorg. Organomet. Polym. Mater., 2020, 30, 2163–2171 CrossRef CAS.
- A. R. Hajipour and Z. Khorsandi, New J. Chem., 2016, 40, 10474–10481 RSC.
- A. R. Hajipour, Z. Khorsandi, M. Mortazavi and H. Farrokhpour, RSC Adv., 2015, 5, 107822–107828 RSC.
- H. Christensen, C. Schjøth-Eskesen, M. Jensen, S. Sinning and H. H. Jensen, Chem.–Eur. J., 2011, 17, 10618–10627 CrossRef CAS PubMed.
- C. Dyrager, L. N. Möllers, L. K. Kjäll, J. P. Alao, P. Dinér, F. K. Wallner, P. Sunnerhagen and M. Grøtli, J. Med. Chem., 2011, 54, 7427–7431 CrossRef CAS PubMed.
- A. Gopalsamy, M. Shi, J. Golas, E. Vogan, J. Jacob, M. Johnson, F. Lee, R. Nilakantan, R. Petersen and K. Svenson, J. Med. Chem., 2008, 51, 373–375 CrossRef CAS PubMed.
- R. L. Dow, J.-C. Li, M. P. Pence, E. M. Gibbs, J. L. LaPerle, J. Litchfield, D. W. Piotrowski, M. J. Munchhof, T. B. Manion and W. J. Zavadoski, ACS Med. Chem. Lett., 2011, 2, 407–412 CrossRef CAS PubMed.
- D. M. George, E. C. Breinlinger, M. Friedman, Y. Zhang, J. Wang, M. Argiriadi, P. Bansal-Pakala, M. Barth, D. B. Duignan and P. Honore, J. Med. Chem., 2015, 58, 222–236 CrossRef CAS PubMed.
- K. H. Huang, J. M. Veal, R. P. Fadden, J. W. Rice, J. Eaves, J.-P. Strachan, A. F. Barabasz, B. E. Foley, T. E. Barta and W. Ma, J. Med. Chem., 2009, 52, 4288–4305 CrossRef CAS PubMed.
- A. M. Griffin, W. Brown, C. Walpole, M. Coupal, L. Adam, M. Gosselin, D. Salois, P.-E. Morin and M. Roumi, Bioorg. Med. Chem. Lett., 2009, 19, 5999–6003 CrossRef CAS PubMed.
- R. Gosmini, V. L. Nguyen, J. Toum, C. Simon, J.-M. G. Brusq, G. Krysa, O. Mirguet, A. M. Riou-Eymard, E. V. Boursier and L. Trottet, J. Med. Chem., 2014, 57, 8111–8131 CrossRef CAS PubMed.
- C. Wren, E. Bell and L. S. Eiland, Ann. Pharmacother., 2018, 52, 1233–1237 CrossRef CAS PubMed.
- A. C. Flick, H. X. Ding, C. A. Leverett, R. E. Kyne Jr, K. K.-C. Liu, S. J. Fink and C. J. O'Donnell, J. Med. Chem., 2017, 60, 6480–6515 CrossRef CAS PubMed.
- E. Vitaku, D. T. Smith and J. T. Njardarson, J. Med. Chem., 2014, 57, 10257–10274 CrossRef CAS PubMed.
- W. S. Cheung, R. R. Calvo, B. A. Tounge, S.-P. Zhang, D. R. Stone, M. R. Brandt, T. Hutchinson, C. M. Flores and M. R. Player, Bioorg. Med. Chem. Lett., 2008, 18, 4569–4572 CrossRef CAS PubMed.
- L. Shao, M. C. Hewitt, S. C. Malcolm, F. Wang, J. Ma, U. C. Campbell, N. A. Spicer, S. R. Engel, L. W. Hardy and Z.-D. Jiang, J. Med. Chem., 2011, 54, 5283–5295 CrossRef CAS PubMed.
- G. Li, H. Zhou, Y. Jiang, H. Keim, S. W. Topiol, S. B. Poda, Y. Ren, G. Chandrasena and D. Doller, Bioorg. Med. Chem. Lett., 2011, 21, 1236–1242 CrossRef CAS PubMed.
- E. F. DiMauro, J. L. Buchanan, A. Cheng, R. Emkey, S. A. Hitchcock, L. Huang, M. Y. Huang, B. Janosky, J. H. Lee and X. Li, Bioorg. Med. Chem. Lett., 2008, 18, 4267–4274 CrossRef CAS PubMed.
- X. Jiang, D. Abgottspon, S. Kleeb, S. Rabbani, M. Scharenberg, M. Wittwer, M. Haug, O. Schwardt and B. Ernst, J. Med. Chem., 2012, 55, 4700–4713 CrossRef CAS PubMed.
- N. Lachance, Y. Gareau, S. Guiral, Z. Huang, E. Isabel, J.-P. Leclerc, S. Léger, E. Martins, C. Nadeau and R. M. Oballa, Bioorg. Med. Chem. Lett., 2012, 22, 980–984 CrossRef CAS PubMed.
- C. H. Hu, J. X. Qiao, Y. Han, T. C. Wang, J. Hua, L. A. Price, Q. Wu, H. Shen, C. S. Huang and R. Rehfuss, Bioorg. Med. Chem. Lett., 2014, 24, 2481–2485 CrossRef CAS PubMed.
- J.-P. Strachan, J. J. Farias, J. Zhang, W. S. Caldwell and B. S. Bhatti, Bioorg. Med. Chem. Lett., 2012, 22, 5089–5092 CrossRef CAS PubMed.
- D. F. Burdi, R. Hunt, L. Fan, T. Hu, J. Wang, Z. Guo, Z. Huang, C. Wu, L. Hardy and M. Detheux, J. Med. Chem., 2010, 53, 7107–7118 CrossRef CAS PubMed.
- I. Tomassoli and D. Gündisch, Bioorg. Med. Chem., 2015, 23, 4375–4389 CrossRef CAS PubMed.
- L. E. Sirois, D. Lao, J. Xu, R. Angelaud, J. Tso, B. Scott, P. Chakravarty, S. Malhotra and F. Gosselin, Org. Process Res. Dev., 2020, 24, 567–578 CrossRef CAS.
- J. Popovici-Muller, R. M. Lemieux, E. Artin, J. O. Saunders, F. G. Salituro, J. Travins, G. Cianchetta, Z. Cai, D. Zhou and D. Cui, ACS Med. Chem. Lett., 2018, 9, 300–305 CrossRef CAS PubMed.
- D. A. Scott, L. A. Dakin, K. Daly, D. J. Del Valle, R. B. Diebold, L. Drew, J. Ezhuthachan, T. W. Gero, C. A. Ogoe and C. A. Omer, Bioorg. Med. Chem. Lett., 2013, 23, 4591–4596 CrossRef CAS PubMed.
- J. H. Ryu, S. Kim, J. A. Lee, H. Y. Han, H. J. Son, H. J. Lee, Y. H. Kim, J.-S. Kim and H.-g. Park, Bioorg. Med. Chem. Lett., 2015, 25, 1679–1683 CrossRef CAS PubMed.
- F. I. Carroll, J. P. Cueva, J. B. Thomas, S. W. Mascarella, S. P. Runyon and H. A. Navarro, ACS Med. Chem. Lett., 2010, 1, 365–369 CrossRef CAS PubMed.
- N. Krogsgaard-Larsen, A. A. Jensen, T. J. Schrøder, C. T. Christoffersen and J. Kehler, J. Med. Chem., 2014, 57, 5823–5828 CrossRef CAS PubMed.
- Y. Liu, Z. Liu, X. Cao, X. Liu, H. He and Y. Yang, Bioorg. Med. Chem. Lett., 2011, 21, 4779–4783 CrossRef CAS PubMed.
- J. U. Flanagan, G. J. Atwell, D. M. Heinrich, D. G. Brooke, S. Silva, L. J. Rigoreau, E. Trivier, A. P. Turnbull, T. Raynham and S. M. Jamieson, Bioorg. Med. Chem., 2014, 22, 967–977 CrossRef CAS PubMed.
- H. Tang, Y. Yan, Z. Feng, R. K. de Jesus, L. Yang, D. A. Levorse, K. A. Owens, T. E. Akiyama, R. Bergeron and G. A. Castriota, Bioorg. Med. Chem. Lett., 2010, 20, 6088–6092 CrossRef CAS PubMed.
- R. R. Taylor, H. C. Twin, W. W. Wen, R. J. Mallot, A. J. Lough, S. D. Gray-Owen and R. A. Batey, Tetrahedron, 2010, 66, 3370–3377 CrossRef CAS.
- X. Wang, D. M. Berger, E. J. Salaski, N. Torres, Y. Hu, J. I. Levin, D. Powell, D. Wojciechowicz, K. Collins and E. Frommer, Bioorg. Med. Chem. Lett., 2009, 19, 6571–6574 CrossRef CAS PubMed.
- D. S. Carter, H.-Y. Cai, E. K. Lee, P. S. Iyer, M. C. Lucas, R. Roetz, R. C. Schoenfeld and R. J. Weikert, Bioorg. Med. Chem. Lett., 2010, 20, 3941–3945 CrossRef CAS PubMed.
- S. M. Lynch, L. Tafesse, K. Carlin, P. Ghatak, B. Shao, H. Abdelhamid and D. J. Kyle, Bioorg. Med. Chem. Lett., 2015, 25, 48–52 CrossRef CAS PubMed.
- E. D. Deeks, Drugs, 2016, 76, 979–987 CrossRef CAS PubMed.
- Y.-Y. Ku, V. S. Chan, A. Christesen, T. Grieme, M. Mulhern, Y.-M. Pu and M. D. Wendt, J. Org. Chem., 2019, 84, 4814–4829 CrossRef CAS PubMed.
- S. L. Greig, Drugs, 2015, 75, 1687–1697 CrossRef CAS PubMed.
- J. L. Beyer and R. H. Weisler, Expert Opin. Pharmacother., 2016, 17, 2331–2339 CrossRef CAS PubMed.
- A. Rathore, V. Asati, S. K. Kashaw, S. Agarwal, D. Parwani, S. Bhattacharya and C. Mallick, Mini-Rev. Med. Chem., 2021, 21, 362–379 CrossRef CAS PubMed.
- H. Lei, Y. Yang, C. Li, F. Jia, N. Jiang, P. Gong and X. Zhai, Org. Process Res. Dev., 2020, 24, 997–1005 CrossRef CAS.
- D. Takács, O. Egyed, L. Drahos, P. Szabó, K. Jemnitz, M. Szabó, Z. Veres, J. Visy, J. Molnár and Z. Riedl, Bioorg. Med. Chem., 2013, 21, 3760–3779 CrossRef PubMed.
- D. Cheng, D. Han, W. Gao, Q. Jing, J. Jiang, Y. Wan, N. P. Englund, T. Tuntland, X. Wu and S. Pan, Bioorg. Med. Chem. Lett., 2012, 22, 6573–6576 CrossRef CAS PubMed.
- S. Wenglowsky, L. Ren, J. Grina, J. D. Hansen, E. R. Laird, D. Moreno, V. Dinkel, S. L. Gloor, G. Hastings and S. Rana, Bioorg. Med. Chem. Lett., 2014, 24, 1923–1927 CrossRef CAS PubMed.
- A. Ruffoni, N. Ferri, S. K. Bernini, C. Ricci, A. Corsini, I. Maffucci, F. Clerici and A. Contini, J. Med. Chem., 2014, 57, 2953–2962 CrossRef CAS PubMed.
- A. W. Sromek, B. A. Provencher, S. Russell, E. Chartoff, B. I. Knapp, J. M. Bidlack and J. L. Neumeyer, ACS Chem. Neurosci., 2014, 5, 93–99 CrossRef CAS PubMed.
- M. Decker, Y.-G. Si, B. I. Knapp, J. M. Bidlack and J. L. Neumeyer, J. Med. Chem., 2010, 53, 402–418 CrossRef CAS PubMed.
- R. M. McKinnell, U. Klein, M. S. Linsell, E. J. Moran, M. B. Nodwell, J. W. Pfeiffer, G. R. Thomas, C. Yu and J. R. Jacobsen, Bioorg. Med. Chem. Lett., 2014, 24, 2871–2876 CrossRef CAS PubMed.
- B. Baur, K. Storch, K. E. Martz, M. I. Goettert, A. Richters, D. Rauh and S. A. Laufer, J. Med. Chem., 2013, 56, 8561–8578 CrossRef CAS PubMed.
- P. Albaugh, Y. Fan, Y. Mi, F. Sun, F. Adrian, N. Li, Y. Jia, Y. Sarkisova, A. Kreusch and T. Hood, ACS Med. Chem. Lett., 2012, 3, 140–145 CrossRef CAS PubMed.
- I. Beria, B. Valsasina, M. G. Brasca, W. Ceccarelli, M. Colombo, S. Cribioli, G. Fachin, R. D. Ferguson, F. Fiorentini and L. M. Gianellini, Bioorg. Med. Chem. Lett., 2010, 20, 6489–6494 CrossRef CAS PubMed.
- P. García-Domínguez, C. Dell'Aversana, R. Alvarez, L. Altucci and A. R. de Lera, Bioorg. Med. Chem. Lett., 2013, 23, 1631–1635 CrossRef PubMed.
- I. Niculescu-Duvaz, E. Roman, S. R. Whittaker, F. Friedlos, R. Kirk, I. J. Scanlon, L. C. Davies, D. Niculescu-Duvaz, R. Marais and C. J. Springer, J. Med. Chem., 2008, 51, 3261–3274 CrossRef CAS PubMed.
- R. P. Wurz, L. H. Pettus, B. Henkle, L. Sherman, M. Plant, K. Miner, H. J. McBride, L. M. Wong, C. J. Saris and M. R. Lee, Bioorg. Med. Chem. Lett., 2010, 20, 1680–1684 CrossRef CAS PubMed.
- S. Solanki, P. Innocenti, C. Mas-Droux, K. Boxall, C. Barillari, R. L. van Montfort, G. W. Aherne, R. Bayliss and S. Hoelder, J. Med. Chem., 2011, 54, 1626–1639 CrossRef CAS PubMed.
- H. Kakuta, N. Yakushiji, R. Shinozaki, F. Ohsawa, S. Yamada, Y. Ohta, K. Kawata, M. Nakayama, M. Hagaya and C. Fujiwara, ACS Med. Chem. Lett., 2012, 3, 427–432 CrossRef CAS PubMed.
- J. M. Travins, R. C. Bernotas, D. H. Kaufman, E. Quinet, P. Nambi, I. Feingold, C. Huselton, A. Wilhelmsson, A. Goos-Nilsson and J. Wrobel, Bioorg. Med. Chem. Lett., 2010, 20, 526–530 CrossRef CAS PubMed.
- A. Gangjee, O. A. Namjoshi, S. Raghavan, S. F. Queener, R. L. Kisliuk and V. Cody, J. Med. Chem., 2013, 56, 4422–4441 CrossRef CAS PubMed.
- K.-I. Kusakabe, N. Ide, Y. Daigo, T. Itoh, K. Higashino, Y. Okano, G. Tadano, Y. Tachibana, Y. Sato and M. Inoue, ACS Med. Chem. Lett., 2012, 3, 560–564 CrossRef CAS PubMed.
- T. Fukaya, T. Kodo, T. Ishiyama, H. Nishikawa, S. Baba and S. Masumoto, Bioorg. Med. Chem., 2013, 21, 1257–1267 CrossRef CAS PubMed.
- F. Bischoff, D. Berthelot, M. De Cleyn, G. Macdonald, G. Minne, D. Oehlrich, S. Pieters, M. Surkyn, A. S. A. Trabanco and G. Tresadern, J. Med. Chem., 2012, 55, 9089–9106 CrossRef CAS PubMed.
- M. Keenan, J. H. Chaplin, P. W. Alexander, M. J. Abbott, W. M. Best, A. Khong, A. Botero, C. Perez, S. Cornwall and R. A. Thompson, J. Med. Chem., 2013, 56, 10158–10170 CrossRef CAS PubMed.
- Y. Y. Syed, Drugs, 2017, 77, 799–807 CrossRef CAS PubMed.
- F. Musumeci, A. Cianciusi, I. D’Agostino, G. Grossi, A. Carbone and S. Schenone, Molecules, 2021, 26(23), 7069 CrossRef CAS PubMed.
- C. C. Ayala-Aguilera, T. Valero, Á. Lorente-Macías, D. J. Baillache, S. Croke and A. Unciti-Broceta, J. Med. Chem., 2021, 65, 1047–1131 CrossRef PubMed.
- M. Poratti and G. Marzaro, Eur. J. Med. Chem., 2019, 172, 143–153 CrossRef CAS PubMed.
- Z. Khorsandi, A. R. Hajipour, M. R. Sarfjoo and R. S. Varma, Green Chem., 2021, 23, 5222–5229 RSC.
- Z. Khorsandi, F. Keshavarzipour, R. S. Varma, A. R. Hajipour and H. Sadeghi-Aliabadi, Mol. Catal., 2022, 517, 112011 CrossRef CAS.
- M. M. Vasbinder, B. Aquila, M. Augustin, H. Chen, T. Cheung, D. Cook, L. Drew, B. P. Fauber, S. Glossop and M. Grondine, J. Med. Chem., 2013, 56, 1996–2015 CrossRef CAS PubMed.
- C. D. Jones, D. M. Andrews, A. J. Barker, K. Blades, K. F. Byth, M. R. V. Finlay, C. Geh, C. P. Green, M. Johannsen and M. Walker, Bioorg. Med. Chem. Lett., 2008, 18, 6486–6489 CrossRef CAS PubMed.
- N. A. McIntyre, C. McInnes, G. Griffiths, A. L. Barnett, G. Kontopidis, A. M. Slawin, W. Jackson, M. Thomas, D. I. Zheleva and S. Wang, J. Med. Chem., 2010, 53, 2136–2145 CrossRef CAS PubMed.
- M. O. Duffey, T. J. Vos, R. Adams, J. Alley, J. Anthony, C. Barrett, I. Bharathan, D. Bowman, N. J. Bump and R. Chau, J. Med. Chem., 2012, 55, 197–208 CrossRef CAS PubMed.
- M. Caldarelli, M. Angiolini, T. Disingrini, D. Donati, M. Guanci, S. Nuvoloni, H. Posteri, F. Quartieri, M. Silvagni and R. Colombo, Bioorg. Med. Chem. Lett., 2011, 21, 4507–4511 CrossRef CAS PubMed.
- M. D. Hopkin, I. R. Baxendale and S. V. Ley, Chem. Commun., 2010, 46, 2450–2452 RSC.
- H. A. Blair, Drugs, 2019, 79, 1719–1725 CrossRef CAS PubMed.
- T. Zhou, S. Georgeon, R. Moser, D. Moore, A. Caflisch and O. Hantschel, Leukemia, 2014, 28, 404–407 CrossRef CAS PubMed.
- P. Dao, C. Garbay and H. Chen, Tetrahedron, 2012, 68, 3856–3860 CrossRef CAS.
- M. S. Palanki, H. Akiyama, P. Campochiaro, J. Cao, C. P. Chow, L. Dellamary, J. Doukas, R. Fine, C. Gritzen and J. D. Hood, J. Med. Chem., 2008, 51, 1546–1559 CrossRef CAS PubMed.
- K. R. Hornberger, J. G. Badiang, J. M. Salovich, K. W. Kuntz, K. A. Emmitte and M. Cheung, Tetrahedron Lett., 2008, 49, 6348–6351 CrossRef CAS.
- J. Hartwig, S. Ceylan, L. Kupracz, L. Coutable and A. Kirschning, Angew. Chem., Int. Ed., 2013, 52, 9813–9817 CrossRef CAS PubMed.
- N. Griebenow, L. Bärfacker, H. Meier, D. Schneider, N. Teusch, K. Lustig, R. Kast and P. Kolkhof, Bioorg. Med. Chem. Lett., 2010, 20, 5891–5894 CrossRef CAS PubMed.
- T. Wu, A. Nagle, K. Kuhen, K. Gagaring, R. Borboa, C. Francek, Z. Chen, D. Plouffe, A. Goh and S. B. Lakshminarayana, J. Med. Chem., 2011, 54, 5116–5130 CrossRef CAS PubMed.
- M. A. McGowan, J. L. Henderson and S. L. Buchwald, Org. Lett., 2012, 14, 1432–1435 CrossRef CAS PubMed.
- H. Cheng, S. K. Nair, B. W. Murray, C. Almaden, S. Bailey, S. Baxi, D. Behenna, S. Cho-Schultz, D. Dalvie and D. M. Dinh, J. Med. Chem., 2016, 59, 2005–2024 CrossRef CAS PubMed.
- C. Li, L. Franklin, R. Chen, T. Mack, M. Humora, B. Ma, B. T. Hopkins, J. Guzowski, F. Zheng and M. MacPhee, Org. Process Res. Dev., 2020, 24, 1199–1206 CrossRef CAS.
- C. Peifer, R. Urich, V. Schattel, M. Abadleh, M. Röttig, O. Kohlbacher and S. Laufer, Bioorg. Med. Chem. Lett., 2008, 18, 1431–1435 CrossRef CAS PubMed.
- J. Liang, A. van Abbema, M. Balazs, K. Barrett, L. Berezhkovsky, W. Blair, C. Chang, D. Delarosa, J. DeVoss and J. Driscoll, J. Med. Chem., 2013, 56, 4521–4536 CrossRef CAS PubMed.
- T. Xue, S. Ding, B. Guo, Y. Zhou, P. Sun, H. Wang, W. Chu, G. Gong, Y. Wang and X. Chen, J. Med. Chem., 2014, 57, 7770–7791 CrossRef CAS PubMed.
- J. S. Bair, R. Palchaudhuri and P. J. Hergenrother, J. Am. Chem. Soc., 2010, 132, 5469–5478 CrossRef CAS PubMed.
- A. Nonoyama, Y. Nakai, S. Lee, S. Suzuki, T. Ando, N. Fukuda, H. Tanaka and K. Takahashi, Org. Process Res. Dev., 2019, 23, 499–511 CrossRef CAS.
- B. R. Rosen, J. C. Ruble, T. J. Beauchamp and A. Navarro, Org. Lett., 2011, 13, 2564–2567 CrossRef CAS PubMed.
- J. R. Sayer, K. Walldén, T. Pesnot, F. Campbell, P. J. Gane, M. Simone, H. Koss, F. Buelens, T. P. Boyle and D. L. Selwood, Bioorg. Med. Chem., 2014, 22, 6459–6470 CrossRef CAS PubMed.
- M. G. Stanton, S. R. Stauffer, A. R. Gregro, M. Steinbeiser, P. Nantermet, S. Sankaranarayanan, E. A. Price, G. Wu, M.-C. Crouthamel and J. Ellis, J. Med. Chem., 2007, 50, 3431–3433 CrossRef CAS PubMed.
- N. Fontán, P. García-Domínguez, R. Álvarez and Á. R. de Lera, Bioorg. Med. Chem., 2013, 21, 2056–2067 CrossRef PubMed.
- A. K. Belfrage, J. Gising, F. Svensson, E. Åkerblom, C. Sköld and A. Sandström, Eur. J. Org. Chem., 2015, 2015, 978–986 CrossRef CAS.
- S. Breitler, N. J. Oldenhuis, B. P. Fors and S. L. Buchwald, Org. Lett., 2011, 13, 3262–3265 CrossRef CAS PubMed.
- C. Sun, D. K. Hunt, R. B. Clark, D. Lofland, W. J. O'Brien, L. Plamondon and X.-Y. Xiao, J. Med. Chem., 2011, 54, 3704–3731 CrossRef CAS PubMed.
- P. T. Bremer and K. D. Janda, J. Med. Chem., 2012, 55, 10776–10780 CrossRef CAS PubMed.
- M. P. Wentland, Q. Lu, R. Ganorkar, S.-Z. Zhang, S. Jo, D. J. Cohen and J. M. Bidlack, Bioorg. Med. Chem. Lett., 2009, 19, 365–368 CrossRef CAS PubMed.
- L. Liu, A. Siegmund, N. Xi, P. Kaplan-Lefko, K. Rex, A. Chen, J. Lin, J. Moriguchi, L. Berry and L. Huang, J. Med. Chem., 2008, 51, 3688–3691 CrossRef CAS PubMed.
- E. J. Hicken, F. P. Marmsater, M. C. Munson, S. T. Schlachter, J. E. Robinson, S. Allen, L. E. Burgess, R. K. DeLisle, J. P. Rizzi and G. T. Topalov, ACS Med. Chem. Lett., 2014, 5, 78–83 CrossRef CAS PubMed.
- S. Matsumoto, N. Miyamoto, T. Hirayama, H. Oki, K. Okada, M. Tawada, H. Iwata, K. Nakamura, S. Yamasaki and H. Miki, Bioorg. Med. Chem., 2013, 21, 7686–7698 CrossRef CAS PubMed.
- A. L. Wolfe, K. K. Duncan, N. K. Parelkar, S. J. Weir, G. A. Vielhauer and D. L. Boger, J. Med. Chem., 2012, 55, 5878–5886 CrossRef CAS PubMed.
- M. Capi, F. de Andrés, L. Lionetto, G. Gentile, F. Cipolla, A. Negro, M. Borro, P. Martelletti and M. Curto, Expert Opin. Invest. Drugs, 2017, 26, 227–234 CrossRef CAS PubMed.
- D. Zhang, M.-J. Blanco, B.-P. Ying, D. Kohlman, S. X. Liang, F. Victor, Q. Chen, J. Krushinski, S. A. Filla and K. J. Hudziak, Bioorg. Med. Chem. Lett., 2015, 25, 4337–4341 CrossRef CAS PubMed.
- Y. N. Lamb, Drugs, 2017, 77, 1797–1804 CrossRef CAS PubMed.
- Y. Qian, D. Bolin, K. Conde-Knape, P. Gillespie, S. Hayden, K.-S. Huang, A. R. Olivier, T. Sato, Q. Xiang and W. Yun, Bioorg. Med. Chem. Lett., 2013, 23, 2936–2940 CrossRef CAS PubMed.
- T. Tomoo, T. Nakatsuka, T. Katayama, Y. Hayashi, Y. Fujieda, M. Terakawa and K. Nagahira, J. Med. Chem., 2014, 57, 7244–7262 CrossRef CAS PubMed.
- S. Ueda, M. Su and S. L. Buchwald, J. Am. Chem. Soc., 2012, 134, 700–706 CrossRef CAS PubMed.
- A. H. Sandtorv, C. Leitch, S. L. Bedringaas, B. T. Gjertsen and H. R. Bjørsvik, ChemMedChem, 2015, 10, 1522–1527 CrossRef CAS PubMed.
- M.-h. Kim, J. Lee, K. Jung, M. Kim, Y.-J. Park, H. Ahn, Y. H. Kwon and J.-M. Hah, Bioorg. Med. Chem., 2013, 21, 2271–2285 CrossRef CAS PubMed.
- J. Chao, A. G. Taveras and C. J. Aki, Tetrahedron Lett., 2009, 50, 5005–5008 CrossRef CAS.
- E. Canales, J. S. Carlson, T. Appleby, M. Fenaux, J. Lee, Y. Tian, N. Tirunagari, M. Wong and W. J. Watkins, Bioorg. Med. Chem. Lett., 2012, 22, 4288–4292 CrossRef CAS PubMed.
- S. Bindi, D. Fancelli, C. Alli, D. Berta, J. A. Bertrand, A. D. Cameron, P. Cappella, P. Carpinelli, G. Cervi and V. Croci, Bioorg. Med. Chem., 2010, 18, 7113–7120 CrossRef CAS PubMed.
- R. Romagnoli, P. G. Baraldi, M. Kimatrai Salvador, D. Preti, M. Aghazadeh Tabrizi, M. Bassetto, A. Brancale, E. Hamel, I. Castagliuolo and R. Bortolozzi, J. Med. Chem., 2013, 56, 2606–2618 CrossRef CAS PubMed.
- A. Gupta and M. L. Condakes, J. Org. Chem., 2021, 86, 17523–17527 CrossRef CAS PubMed.
- A. Stumpf, J. Burkhard, D. Xu, A. Marx, D. Lao, M. Ochsenbein, R. Ranjan, R. Angelaud and F. Gosselin, Org. Process Res. Dev., 2021, 25, 2537–2550 CrossRef CAS.
- J. Xiong, H.-T. He, H.-Y. Yang, Z.-G. Zeng, C.-R. Zhong, H. Shi, M.-L. Ouyang, Y.-Y. Tao, Y.-L. Pang and Y.-H. Zhang, J. Org. Chem., 2022, 87, 9488–9496 CrossRef CAS PubMed.
- J. Bullington, D. Argentieri, K. Averill, D. Carter, D. Cavender, B. Fahmy, X. Fan, D. Hall, G. Heintzelman and P. Jackson, Bioorg. Med. Chem. Lett., 2006, 16, 6102–6106 CrossRef CAS PubMed.
- E. J. Hanan, C. Eigenbrot, M. C. Bryan, D. J. Burdick, B. K. Chan, Y. Chen, J. Dotson, R. A. Heald, P. S. Jackson and H. La, J. Med. Chem., 2014, 57, 10176–10191 CrossRef CAS PubMed.
- E. A. Peterson, A. A. Boezio, P. S. Andrews, C. M. Boezio, T. L. Bush, A. C. Cheng, D. Choquette, J. R. Coats, A. E. Colletti and K. W. Copeland, Bioorg. Med. Chem. Lett., 2012, 22, 4967–4974 CrossRef CAS PubMed.
- M. Nodwell, C. Zimmerman, M. Roberge and R. J. Andersen, J. Med. Chem., 2010, 53, 7843–7851 CrossRef CAS PubMed.
- M. M. Stec, K. L. Andrews, Y. Bo, S. Caenepeel, H. Liao, J. McCarter, E. L. Mullady, T. San Miguel, R. Subramanian and N. Tamayo, Bioorg. Med. Chem. Lett., 2015, 25, 4136–4142 CrossRef CAS PubMed.
- A. Nagle, T. Wu, K. Kuhen, K. Gagaring, R. Borboa, C. Francek, Z. Chen, D. Plouffe, X. Lin and C. Caldwell, J. Med. Chem., 2012, 55, 4244–4273 CrossRef CAS PubMed.
- E. L. Larghi, A. Bruneau, F. Sauvage, M. Alami, J. Vergnaud-Gauduchon and S. Messaoudi, Molecules, 2022, 27, 412 CrossRef CAS PubMed.
- R. M. Al-Zoubi, W. K. Al-Jammal, M. S. Al-Zoubi, M. J. Ferguson, A. Zarour, A. Yassin and A. Al-Ansari, ChemistrySelect, 2021, 6, 3417–3423 CrossRef CAS.
- W. Zhang, S. Li, H. Liu, Y. Zhang, H. Xie, D. Peng, H. Peng, Z. Ou, Z. Peng and W. Dong, Org. Process Res. Dev., 2022, 26, 1078–1093 CrossRef CAS.
- M. H. Reutershan, M. R. Machacek, M. D. Altman, S. Bogen, M. Cai, C. Cammarano, D. Chen, M. Christopher, J. Cryan and P. Daublain, J. Med. Chem., 2021, 64, 16213–16241 CrossRef CAS PubMed.
- G. He, S. Wan, Y. Wu, Z. Chu, H. Shen, S. Zhang, L. Chen, Z. Bao, S. Gu and J. Huang, J. Med. Chem., 2022, 65, 14348–14365 CrossRef CAS PubMed.
- J. J. Marineau, K. B. Hamman, S. Hu, S. Alnemy, J. Mihalich, A. Kabro, K. M. Whitmore, D. K. Winter, S. Roy and S. Ciblat, J. Med. Chem., 2021, 65, 1458–1480 CrossRef PubMed.
- Y. Zang, L. Tai, Y. Hu, Y. Wang, H. Sun, X. Wen, H. Yuan and L. Dai, J. Chem. Inf. Model., 2022, 62, 3123–3132 CrossRef CAS PubMed.
- L. D. Turner, C. H. Trinh, R. A. Hubball, K. M. Orritt, C.-C. Lin, J. E. Burns, M. A. Knowles and C. W. Fishwick, J. Med. Chem., 2021, 65, 1481–1504 CrossRef PubMed.
- D. Zuo, Q. Wang, L. Liu, T. Huang, M. Szostak and T. Chen, Angew. Chem., Int. Ed., 2022, 13, 61 Search PubMed.
- Z. Li, L. Liu, K. Xu, T. Huang, X. Li, B. Song and T. Chen, Org. Lett., 2020, 22, 5517–5552 CrossRef CAS PubMed.
- J. Magano and J. R. Dunetz, Chem. Rev., 2011, 111, 2177–2250 CrossRef CAS PubMed.
- S. L. Buchwald, C. Mauger, G. Mignani and U. Scholz, Adv. Synth. Catal., 2006, 348, 23–39 CrossRef CAS.
- J. S. Carey, D. Laffan, C. Thomson and M. T. Williams, Org. Biomol. Chem., 2006, 4, 2337–2347 RSC.
- K.-I. Wakabayashi, K. Imai, H. Miyachi, Y. Hashimoto and A. Tanatani, Bioorg. Med. Chem., 2008, 16, 6799–6812 CrossRef CAS PubMed.
- T. Dahl, C. W. Tornøe, B. Bang-Andersen, P. Nielsen and M. Jørgensen, Angew. Chem., Int. Ed., 2008, 47, 1726–1728 CrossRef CAS PubMed.
- L. Shao, J. Ma, F. Wang, S. C. Malcolm, M. C. Hewitt, U. C. Campbell, N. A. Spicer, L. W. Hardy, R. Schreiber, K. L. Spear and M. A. Varney, Bioorg. Med. Chem. Lett., 2011, 21, 520–523 CrossRef CAS PubMed.
- M. A. McGowan, C. Z. McAvoy and S. L. Buchwald, Org. Lett., 2012, 14(14), 3800–3803 CrossRef CAS PubMed.
- K. Siva Kumar, P. Mahesh Kumar, V. Sreenivasa Rao, A. A. Jafar, C. L. T. Meda, R. Kapavarapu, K. V. L. Parsa and M. Pal, Org. Biomol. Chem., 2012, 10(15), 3098–3103 RSC.
|
This journal is © The Royal Society of Chemistry 2023 |
Click here to see how this site uses Cookies. View our privacy policy here.