DOI:
10.1039/D2RA07398F
(Paper)
RSC Adv., 2023,
13, 4958-4962
Metal-free regioselective mono- and poly-halogenation of 2-substituted indazoles†
Received
21st November 2022
, Accepted 4th January 2023
First published on 7th February 2023
Abstract
An unprecedented metal-free regioselective halogenation of 2H-indazoles has been revealed, which not only realized the highly selective synthesis of mono-halogenated products, but also completed poly-halogenations by fine tuning the reaction conditions. Various mono-/poly-/hetero-halogenated indazoles were obtained in moderate to excellent yields. Notably, this approach features environmentally friendly solvents, mild reaction conditions, simple execution and short reaction time.
Halogens can significantly alter the biological properties of molecules, rendering the use of these compounds as drugs, agrochemicals, biocides, etc.1 In addition, organic halides are one of the most widely used precursors or intermediates for numerous organic transformations.2 For example, hetero-aromatic bromides and iodides play an important role in Grignard reactions3 and cross-coupling.4 Therefore, the construction of halogenated hetero-aromatic compounds through direct C–H halogenation is highly desirable.
Indazole, a nitrogen-containing heterocycle, has attracted much attention for its biological properties and a broad spectrum of medicinal values,5 such as anti-ovarian cancer drug Niraparib,6 selective estrogen receptor degrading agents,7 liver X receptor agonist,8 selective CRAF inhibitor,9 anticancer drugs Pazopanib,10 MK-482714,9 and gamedazoleq11 (Fig. 1). Notably, these drugs could be synthesized from halogenated indazole intermediates.
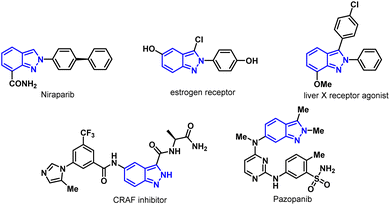 |
| Fig. 1 Bioactive compounds containing 2H-indazole. | |
Recognizing the importance of these molecules, chemists have developed various methods to synthesize indazole halides. However, C–H direct bromination of indazoles without metal catalysts has been rarely reported. Clarisse declared the bromination of 2-phenyl-2H-indazole employing Br2 as brominating reagent.12 Although 3-bromo-2H-indazole was formed in high yield, a mixture of 3,5-dibromo- and 3,7-dibromo-2H-indazole was obtained with poor selectivity and low yield. At the same time, the use of Br2 was environmentally unfriendly and troublesome. Herein, an efficient C–H direct halogenation of 2H-indazoles employing NXS (X = Br, Cl) was reported, which achieved the selective synthesis of mono-, poly- and hetero-halogenated products in high yields by adjusting reaction conditions.
In our initial study, 2-phenyl-2H-indazole (1a) and NBS (1.0 equiv.) were selected as model substrates to react at 25 °C. It was delighted that 88% mono-brominated product 2a was obtained after 2.0 h (Table 1, entry 1). Preliminary investigation of the reaction temperature demonstrated that the yield of target product 2a increased to 98% with the increase of reaction temperature (Table 1, entry 2). Similarly, the screening of solvents was also within our consideration for the purpose of corresponding green chemistry. Switching the MeCN to H2O or CH3OH, led to the decreased yield of 2a (Table 1, entries 3 and 4). But gratifyingly, in green solvent EtOH, 1a could be cleanly converted into mono-substituted product 2a with an excellent yield of 97% (Table 1, entry 5). The reaction temperature and equiv. of NBS were further investigated when H2O was used as solvent. The result indicated that in the presence of 1.3 equiv. NBS, it was suitable for mono-bromination and 2a was isolated by simple filtration with high yield of 96% under 95 °C (Table 1, entry 6). To our surprise, gradually increasing the equiv. of NBS produced disubstituted products 3,7-dibromo-2H-indazole 3a (Table 1, entry 7). The yield of 3a was greatly improved, when H2O was replaced by EtOH or MeCN, but higher temperature seemed to have a detrimental effect (Table 1, entries 8–10). It was worth to mention that no byproduct 3,5-dibromo-2H-indazole was detected. This reaction was then carried out at 80 °C by increasing the equiv. of NBS, suggesting that trisubstitution was best performed at 4.0 equiv. of NBS in MeCN and the yield of tribrominated product 4a could be increased to 71% (Table 1, entry 13).
Table 1 Screening of reaction parametersa
With the mentioned optimized reaction protocol in hand, first of all, the scopes of the mono-bromination were examined (Table 2). The effects of different substituents on the N-phenyl ring of 2H-indazoles were investigated, and the desired products could be obtained in the yield of 80–98% for both electron-donating and electron-withdrawing groups (2a–2l). Steric hindrance had effect on the yield, m-substituents on the phenyl ring resulting in lower yields compared to p-substituents (2b and 2g), and 3,4-disubstituents on the phenyl ring furnishing the desired products in moderate yields (2m and 2n). However, the situation changed when the substituents was on the indazole skeleton. It was found that electron-withing groups such as F or Cl were compatible with the optimized reaction conditions and afforded the corresponding desired products in good to excellent yields (2p vs. 2q). While the substituent was methoxy, the raw material could not be completely converted giving product in 31% yield (2o). Furthermore, this method could be extended to the mono-bromination of N-pyridyl indazole with 81% yield (2r). In addition, applicability of aliphatic substituted substrates was also explored. The yield decreased sharply to 36% when the substituent was tert-butyl (2s), while none product was detected with n-butyl substituted indazole (2r).
Table 2 Substrate scope for mono-bromination of 2H-indazolesa,b
Reaction conditions: (A) 1 (0.3 mmol), NBS (0.3 mmol), EtOH (3.0 mL), 50 °C, air, 2.0 h. (B) 1 (0.3 mmol), NBS (0.39 mmol), H2O (3.0 mL), 95 °C, air, 5.0 h. Isolated yield. |
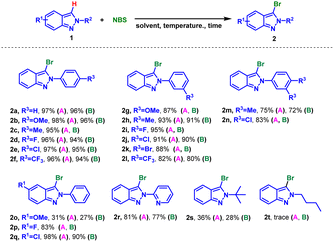 |
Inspired by the successful mono-bromination of 2H-indazoles under environmentally friendly conditions, the mono-chlorination was subsequently tested using NCS as chlorinating reagent (Table 3). The substrates with substituents on N-phenyl ring or indazole skeleton exhibited good reactivity both in H2O and EtOH (5a–5r). Interestingly, m-substituents on the phenyl ring gave higher yields than p-substituents, as opposed to mono-bromination (5b vs. 5h). 2-Pyridyl-2H-indazole and 2-(tert-butyl)-2H-indazole also worked giving the desired products in 81% and 36% yield respectively (5s and 5t). It was a pity that iodination of 2H-indazoles in EtOH with N-iodo-succinimide (NIS) was not succeeded.
Table 3 Substrate scope for mono-chloramination of 2H-indazolesa,b
Reaction conditions: (A) 1 (0.3 mmol), NCS (0.3 mmol), EtOH (3.0 mL), 50 °C, air, 2.0 h. (B) 1 (0.3 mmol), NCS (0.39 mmol), H2O (3.0 mL), 95 °C, air, 5.0 h. Isolated yield. |
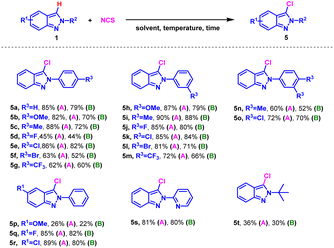 |
We then turned our attention to poly-halogenation of 2H-indazoles (Table 4), affording the corresponding di-halogenated products in 64–70% yields (3a–3d). Based on this, the conversion of 2H-indazoles to hetero-halogenated indazoles was realized by ‘one-pot, two step’ method. 3-Bromo-7-chloro-2H-indazoles were prepared by bromination followed by chlorination with moderate yield (3e and 3f). And 3-chloro-7-bromo-2H-indazoles were produced in 65–74% yield through chlorination-bromination process (3g–3j). It was found that the yield of 3-bromo-7-chloro-2H-indazoles were higher than 3-chloro-7-bromo-2H-indazoles (3e vs. 3h, 3f vs. 3i), which might be due to the low reactivity of indazole C7 position and stronger activity of NBS than NCS. By increasing the amount of NBS and prolonging the reaction time, the tribrominated product 4b was obtained in 72% yield from 2-(m-tolyl)-2H-indazole.
Table 4 Substrate scope for poly-halogenation of 2H-indazolesa,b
Reaction conditions: (3a–3d) 1 (0.3 mmol), NXS in batches (0.6 mmol), EtOH (3.0 mL), 50 °C, air, 6.0 h. (3e–3f) step 1: 1 (0.3 mmol), NBS (0.3 mmol), EtOH (3.0 mL), 50 °C, air, 2.0 h; step 2: NCS (0.3 mmol), 50 °C, air, 4.0 h. (3g–3j) step 1: 1 (0.3 mmol), NCS (0.3 mmol), EtOH (3.0 mL), 50 °C, air, 2.0 h; step 2: NBS (0.3 mmol), 50 °C, air, 4.0 h. Isolated yield. (4b) MeCN (5.0 mL), 50 °C, air, 8.0 h. |
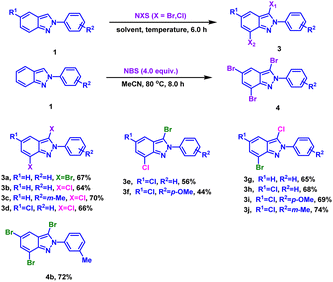 |
To identify the structures, we took product 3c and 3j as examples to measure DEPT135, 1H–1H COSY, 1H–13C HSQC and 1H–13C HMBC spectra (Fig. 2), the details are listed in the ESI.†
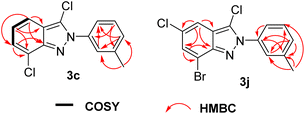 |
| Fig. 2 Key COSY and HMBC correlations of compounds 3c and 3j. | |
For purpose of demonstrating the suitability of this halogenation method on a large scale, a gram-scale reaction was investigated. The results showed that 6.0 mmol of 1a (1.164 g) could be cleanly converted to 2a with either EtOH or H2O as solvent (Scheme 1).
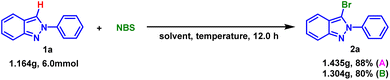 |
| Scheme 1 Gram-scale reaction. | |
In order to gain more insights into the mechanism, a series of control experiments were conducted. Firstly, using isolated mono-brominated product 2a as substrate and 1.0 equiv. NBS as brominating reagent, TLC monitoring showed that the dibrominated product 3a was generated, indicating that dihalogenation occurred after mono-halogenation (Scheme 2a). Second, when 3.0 equiv. 2,2,6,6-tetramethyl-piperidine-1-oxyl (TEMPO) or 2,6-di-tert-butyl-4-methylphenol (BHT) was respectively added under the standard reaction conditions, no desired products were formed (Scheme 2b). In addition, bromine radical was captured and 6 was detected by HRMS when ethene-1,1-diyldibenzene was used (Scheme 2c).
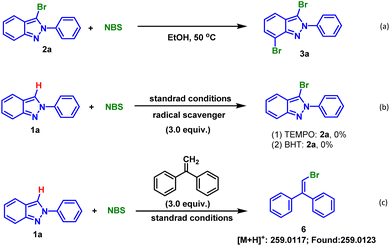 |
| Scheme 2 Control experiments. | |
We considered that a radical pathway mechanism could be involved on the grounds of experimental results and previous reports.13 At first, NBS was pyrolyzed under heating conditions to generate bromine radical and radical A. Then substrate 1a reacted with bromine radical to generate intermediate I, which would further oxidize by radical A to produce cationic intermediate II and succinimide anionic B. The proton transfer occurred between the above two ions, and finally succinimide C and mono-brominated product 2a were generated. Similarly, dibrominated product 3a could be obtained from 2a via the above pathway (Scheme 3).
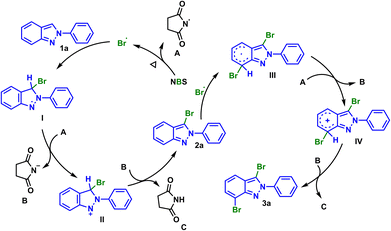 |
| Scheme 3 Proposed reaction mechanisms. | |
Conclusions
In summary, we have successfully developed a simple and universal metal-free method for the synthesis of mono- and poly-halogenated 2H-indazoles. The mono-halogenation could be carried out in water giving products with good yields. Furthermore, hetero-halogenated 2H-indazole compounds were also achieved via a one-pot reaction. In addition, the gram-scale reaction also produced excellent yields. This new transformation exhibits high selectivity, good functional group tolerance, easy handing and eco-friendliness, rendering the “green” methodology as potential applications in agrochemical and pharmaceutical industries.
Conflicts of interest
There are no conflicts to declare.
Acknowledgements
This project was supported by National Key R&D Program of China (2021YFC2100800); National Natural Science Foundation of China, NSFC (Grant No. 21978273); Research and Application Service Platform Project of API Manufacturing Environmental Protection and Safety Technology in China (2020-0107-3-1); Catalysis Base Construction for Chemical and Pharmaceutical Intermediates (2019-00900-2-1); Zhejiang Key R&D Program (No. 2021C03085); China Postdoctoral Science Foundation, CPSF (No. 2021M702897).
Notes and references
-
(a) K. H. van Pée, Biosynthesis of halogenated metabolites by bacteria, Annu. Rev. Microbiol., 1996, 50, 375–399 CrossRef PubMed;
(b) A. Castellanos and S. P. Fletcher, Current methods for asymmetric halogenation of olefins, Chem. - Eur. J., 2011, 17, 5766–5776 CrossRef CAS PubMed;
(c) P. Pimviriyakul, T. Wongnate, R. Tinikul and P. Chaiyen, Microbial degradation of halogenated aromatics: molecular mechanisms and enzymatic reactions, Microbiol. Biotechnol., 2020, 13, 67–86 CrossRef PubMed;
(d) Q. Li, C. Li, J. Kim, M. Ishida, X. Li, T. Gu, X. Liang, W. Zhu, H. Ågren, H. Furuta and Y. Xie, Regioselectively halogenated expanded porphyrinoids as building blocks for constructing porphyrin–porphyrinoid heterodyads with tunable energy transfer, J. Am. Chem. Soc., 2019, 141, 5294–5302 CrossRef CAS PubMed;
(e) J. S. Neto, R. A. Balaguez, M. S. Franco, V. C. de Sa Machado, S. Saba, J. Rafique, F. Z. Galetto and A. L. Braga, Trihaloisocyanuric acids in ethanol: an eco-friendly system for the regioselective halogenation of imidazo-heteroarenes, Green Chem., 2020, 22, 3410–3415 RSC;
(f) C. Wang, H. Lu, J. Lan, K. A. Zaman and S. Cao, A review: Halogenated compounds from marine fungi, Molecules, 2021, 26, 458 CrossRef CAS PubMed.
-
(a) J. S. Neto, R. A. Balaguez, M. S. Franco, V. C. de Sa Machado, S. Saba, J. Rafique, F. Z. Galetto and A. L. Braga, Trihaloisocyanuric acids in ethanol: an eco-friendly system for the regioselective halogenation of imidazo-heteroarenes, Green Chem., 2020, 22, 3410–3415 RSC;
(b) E. T. Martin, C. M. McGuire, M. S. Mubarak and D. G. Peters, Electroreduction remediation of halogenated environmental pollutants, Chem. Rev., 2016, 116, 15198–15234 CrossRef CAS PubMed;
(c) W. Chung and C. D. Vanderwal, Stereoselective halogenation in natural product synthesis, Angew. Chem., Int. Ed., 2016, 55, 4396–4434 CrossRef CAS PubMed;
(d) R. Lin, A. P. Amrute and J. Pérez-Ramírez, Halogen-mediated conversion of hydrocarbons to commodities, Chem. Rev., 2017, 117, 4182–4247 CrossRef CAS PubMed;
(e) M. R. Scheide, C. R. Nicoleti, G. M. Martins and A. L. Braga, Electrohalogenation of organic compounds, Org. Biomol. Chem., 2021, 19, 2578–2602 RSC.
-
(a) G. S. Silverman, Common methods of Grignard reagent preparation, Chem. Indu, New York-Marcel Dekker, 1996, pp. 9–22 Search PubMed;
(b) R. G. Kultyshev, S. Liu, H. T. Leung, J. Liu and S. G. Shore, Synthesis of mono- and dihalogenated derivatives of (Me2S)2B12H10 and palladium-catalyzed boron−carbon cross-coupling reactions of the iodides with Grignard reagents, Inorg. Chem., 2003, 42, 3199–3207 CrossRef CAS PubMed;
(c) K. Yagi, H. Shinokubo and K. Oshima, Synthesis of silyl aziridines and α-amino acylsilanes with silyldibromomethyllithium, Org. Lett., 2004, 6, 4339–4341 CrossRef CAS PubMed;
(d) L. Nicolas, P. Angibaud, I. Stansfield, P. Bonnet, L. Meerpoel, S. Reymond and J. Cossy, Diastereoselective metal-catalyzed synthesis of C-aryl and C-vinyl glycosides, Angew. Chem., Int. Ed., 2012, 124, 11263–11266 CrossRef;
(e) W. Muramatsu and K. Nakano, Organocatalytic approach for C(sp3)–H bond arylation, alkylation, and amidation of isochromans under facile conditions, Org. Lett., 2014, 16, 2042–2045 CrossRef CAS PubMed;
(f) D. D. Chronopoulos, A. Bakandritsos, P. Lazar, M. Pykal, K. Čépe, R. Zbořil and M. Otyepka, High-yield alkylation and arylation of graphene via Grignard reaction with fluorographene, Chem. Mater., 2017, 29, 926–930 CrossRef CAS PubMed;
(g) S. Chen, T. Wu and C. J. C. Zhao, Chem. Europe, 2020, 13, 5516–5522 CAS.
-
(a) A. De Meijere, S. Bräse and M. Oestreich, Metal catalyzed cross-coupling reactions and more, 3 Volume Set, John Wiley & Sons, 2013 Search PubMed;
(b) I. J. S. Fairlamb, Regioselective (site-selective) functionalisation of unsaturated halogenated nitrogen, oxygen and sulfur heterocycles by Pd-catalysed cross-couplings and direct arylation processes, Chem. Soc. Rev., 2007, 36, 1036–1045 RSC;
(c) F. M. Moghaddam, G. Tavakoli, B. Saeednia, P. Langer and B. Jafari, Palladium-catalyzed carbamate-directed regioselective halogenation: a route to halogenated anilines, J. Org. Chem., 2016, 81, 3868–3876 CrossRef CAS PubMed;
(d) S. Zhang, Z. Xia, T. Ni, H. Zhang, C. Wu and Y. Qu, Tuning chemical compositions of bimetallic AuPd catalysts for selective catalytic hydrogenation of halogenated quinolines, J. Mater. Chem. A, 2017, 5, 3260–3266 RSC;
(e) Q. Li, L. Zhou, X. Shen, K. Yang, X. Zhang, Q. Dai, H. Leng, Q. Li and J. Li, Stereoselective construction of halogenated quaternary carbon centers by brønsted base catalyzed [4+2] cycloaddition of α-haloaldehydes, Angew. Chem., Int. Ed., 2018, 57, 1913–1917 CrossRef CAS PubMed.
-
(a) H. Cerecetto, A. Gerpe, M. Gonzalez, V. J. Aran and C. O. de Ocariz, Pharmacological properties of indazole derivatives: Recent developments, Mini-Rev. Med. Chem., 2005, 5, 869–878 CrossRef CAS PubMed;
(b) A. Schmidt, A. Beutler and B. Snovydovych, Recent advances in the chemistry of indazoles, Eur. J. Org. Chem., 2008, 2008, 4073–4095 CrossRef;
(c) D. D. Gaikwad, A. D. Chapolikar, C. G. Devkate, K. D. Warad, A. P. Tayade, R. P. Pawar and A. J. Domb, Synthesis of indazole motifs and their medicinal importance: An overview, Eur. J. Med. Chem., 2015, 90, 707–731 CrossRef CAS PubMed.
- L. J. Scott, Niraparib: first global approval, Drugs, 2017, 77, 1029–1034 CrossRef PubMed.
-
(a) S. M. Moore, A. J. Khalaj, S. Kumar, Z. Winchester, J. Yoon, T. Yoo, L. Martinez-Torres, N. Yasui, J. A. Katzenellenbogen and S. K. Tiwari-Woodruff, Multiple functional therapeutic effects of the estrogen receptor β agonist indazole-Cl in a mouse model of multiple sclerosis, Proc. Natl. Acad. Sci. U. S. A., 2014, 111, 18061–18066 CrossRef CAS PubMed;
(b) S. P. Govek, J. Y. Nagasawa, K. L. Douglas, A. Lai, M. Kahraman, C. Bonnefous, A. M. Aparicio, B. D. Darimont, K. L. Grillot, J. D. Joseph, J. A. Kaufman, K. Lee, N. Lu, M. J. Moon, R. Y. Prudente, J. Sensintaffar, P. J. Rix, J. H. Hager and N. D. Smith, Optimization of an indazole series of selective estrogen receptor degraders: Tumor regression in a tamoxifen-resistant breast cancer xenograft, Bioorg. Med. Chem. Lett., 2015, 25, 5163–5167 CrossRef CAS PubMed.
- A. Katz, C. Udata, E. Ott, L. Hickey, M. E. Burczynski, P. Burghart, O. Vesterqvist and X. Meng, Safety, pharmacokinetics, and pharmacodynamics of single doses of LXR-623, a novel liver X-receptor agonist, in healthy participants, J. Clin. Pharmacol., 2009, 49, 643–649 CrossRef CAS PubMed.
- W. Aman, J. Lee, M. Kim, S. Yang, H. Jung and J. M. Hah, Discovery of highly selective CRAF inhibitors, 3-carboxamido-2H-indazole-6-arylamide: In silico FBLD design, synthesis and evaluation, Bioorg. Med. Chem. Lett., 2016, 26, 1188–1192 CrossRef CAS PubMed.
- W. T. A. Van Der Graaf, J. Y. Blay, S. P. Chawla, D. W. Kim, B. Bui- Nguyen, P. G. Casali, P. Schöffski, M. Aglietta, A. P. Staddon and Y. Beppu, Pazopanib for metastatic soft-tissue sarcoma (PALETTE): a randomised, double-blind, placebo-controlled phase 3 trial, Lancet, 2012, 379, 1879–1886 CrossRef CAS PubMed.
- A. Veerareddy, G. Surendrareddy and P. K. Dubey, Total syntheses of AF-2785 and gamendazole-experimental male oral contraceptives, Synth. Commun., 2013, 43, 2236–2241 CrossRef CAS.
-
(a) P. S. Waalwijk, P. Cohen-Fernandes and C. L. Habraken, Indazole studies. 3. The bromination of 2-phenyl-2H-indazole. Formation and structure determination of mono-, di-, and tribromo-2-phenyl-2H-indazoles, J. Org. Chem., 1984, 49, 3401–3403 CrossRef CAS;
(b) K. Wang, T. Wei, Y. Zhang, J. Hou, R. Bai and Y. Xie, Metal-free regioselective C–H amination for the synthesis of pyrazole-containing 2H-indazoles, Org. Biomol. Chem., 2021, 19, 1787–1794 RSC;
(c) T. Wei, K. Wang, Z. Yu, J. Hou and Y. Xie, Electrochemically mediated trifluoromethylation of 2H-indazole derivatives using CF3SO2Na, Tetrahedron Lett., 2021, 86, 153313 CrossRef CAS;
(d) J. Hou, K. Wang, C. Zhang, T. Wei, R. Bai and Y. Xie, Metal-Free Electrochemical Oxidative Dihalogenation of Quinolines on the C5 and C7 Positions Using N-Halosuccinimides, Eur. J. Org. Chem., 2020, 2020, 6382–6386 CrossRef CAS.
-
(a) M. Singsardar, S. Laru, S. Mondal and A. Hajra, Visible-light-induced regioselective cross-dehydrogenative coupling of 2H-Indazoles with ethers, J. Org. Chem., 2019, 84, 4543–4550 CrossRef CAS PubMed;
(b) J. Chen, T. Wang, Y. Liu, T. Wang, A. Lin, H. Yao and J. Xu, Metal-free C5-selective halogenation of quinolines under aqueous conditions, Org. Chem. Front., 2017, 4, 622–626 RSC.
|
This journal is © The Royal Society of Chemistry 2023 |
Click here to see how this site uses Cookies. View our privacy policy here.