DOI:
10.1039/D2RA06206B
(Paper)
RSC Adv., 2023,
13, 388-398
Bioremediation of a salty petrochemical wastewater containing bisphenol A by a novel indigenous Pseudomonas pseudoalcaligenes
Received
3rd October 2022
, Accepted 6th December 2022
First published on 21st December 2022
Abstract
One novel indigenous halotolerant, Pseudomonas sp, with high potential for bisphenol A (BPA) biodegradation was isolated from an outlet of petrochemical wastewater in Iran. The optimal temperature and pH for degradation of BPA by this strain were 30 °C and 7, respectively. This strain was able to decrease COD (chemical oxygen demand) of basal salt medium containing 300 mg L−1 BPA as sole carbon source and 40 g L−1 NaCl from 655.2 to 109.2 mg L−1 (about 83% decrease) after 36 h. The bacterium degraded 56.3 (19%), 202.43 (67%), 288.86 (96%) and 300 mg L−1 (100%) BPA in basal salt medium containing 300 mg L−1 BPA and 40 g L−1 NaCl within 12, 18, 24 and 36 h, respectively. In addition, this strain could degrade phenol (100 mg L−1) and BPA (300 mg L−1) in salty petrochemical wastewater within 24 h, completely. In batch fermentation of petrochemical wastewater using this strain higher growth and phenol (100 mg L−1), BPA (372 mg L−1) removal within 6 h were achieved. High performance liquid chromatography (HPLC) and gas chromatography mass spectrometry (GC/MS) analysis revealed several intermediates during the BPA degradation process. These intermediates were identified as 4-hydroxybenzaldehyde, 4-hydroxyacetophenone, 4-hydroxyphenylacetate, M-hydroxymandelic acid, 2-phenylpropane-1,2-diol, 2-phenyl-2-propanol and lactic acid. The possible BPA-biodegradation pathway based on the identified metabolites and in agreement with recorded pathway in KEGG database was proposed. Preliminary 16S rDNA sequence analysis and subsequent genetically characterization through comprehensive genomic analysis identified the strain as Pseudomonas pseudoalcaligenes strain YKJ.
1 Introduction
Polycyclic aromatic hydrocarbons (PAHs) are the most common environmental pollutants. These compounds have been extensively distributed in the various environments including water resources and many peoples around the world are exposed to them.1,2 Bisphenol A (BPA; 2,2-bis(4-hydroxyphenyl) propane; CAS registry no. 80-05-7) as a man-made PAH consisting of two phenol rings, is widely used in production of synthetic polymers, in particular, polycarbonate plastics and epoxy resins.3–9 Despite important industrial applications, this compound has been recognized as one of the endocrine-disrupting chemicals (EDCs) which can affect the reproduction of humans and other organisms due to its androgenic or estrogenic activity. Furthermore, results of several studies have shown mutagenicity, carcinogenicity, immunotoxicity and embryotoxicity effects of BPA that can represent a serious threat to ecological and human health.3–11 Nowadays, widespread global demand for BPA has led to its production increase. In this way, considerable amounts of BPA may be released into the environment, especially industrial and municipal wastewaters during production, transportation, or consumption processes.3,5,9,12–15 Therefore, providing an efficient eco-friendly solution for its removal from exposed natural environments is so necessary. According to the results of several studies3,5,6,8,9,11,16–24 as well as our previous research,25 bioremediation using BPA-degrading bacteria has been proved to be an effective way to remove this compound. Up to now, many BPA-degrading bacteria have been isolated from soil, sediment, water and petrochemical wastewater and BPA-biodegradation pathways have been recognized in most of them.3–9,11,16–29 Lobos et al.19 and Spivack et al.30 were the first to describe two major and minor pathways for BPA-biodegradation by Sphingomonas sp. strain MV1. The major proposed pathway produces 4-hydroxyacetophenone and 4-hydroxybenzoic acid as metabolic intermediary compounds, whereas the minor one produces 2,2-bis(4-hydroxyphenyl)-1-propanol and 2,3-bis(4-hydroxyphenyl)1,2-propanediol as primary metabolites.19,30 Later, in addition to confirmation of these pathways in other bacterial strains, different metabolic pathways were also identified in specific bacterial strains such as Bacillus pumilus strains BP-2CK, BP-21DK, and BP-22DK isolated from kimchi by Yamanaka et al. in 2007 and Pseudomonas aeruginosa PAb1 isolated from effluent of thermal paper industry by Vijayalakshmi et al. in 2018.8,9,16,18,31
Several BPA-degrading bacteria have been reported but most of them are only able to degrade low amounts of high BPA concentrations within several days in lab conditions and thereby cannot be used for practical removing of this compound. Hence, in the present study, isolation and characterization of a novel indigenous BPA-degrading bacterium for elimination of BPA from salty wastewater was considered. Potential of isolated bacterium for BPA removal from petrochemical wastewater was investigated in lab scale. The possible metabolic pathway for BPA biodegradation by isolated bacterium were also proposed by identification of the metabolic intermediary compounds using high performance liquid chromatography (HPLC) and gas chromatography mass spectrometry (GC/MS) analysis.
2 Experimental methods
2.1 Sampling
Different outlets of Khuzestan petrochemical wastewater and Fajr wastewater treatment plant at the Bandar Imam Khomeini petrochemical complex in Iran were considered as habitat of phenol, BPA degrading bacteria. Five samples were collected in screw capped sterilized bottles from those outlets under standard conditions.
2.2 Strains and chemicals
Escherichia coli (DH5a) was used as host strain for gene cloning (Novagen, Germany). BPA, 4-hydroxybenzaldehyde (4-HBAL), 4-hydroxybenzoic acid (4-HBA) and 4-hydroxyacetophenone (4-HAP) with purity of 99% were purchased from Alfa Aesar (Spain). All chemicals, enzymes, plasmids and kits were purchased from specific manufacturers. Solvents for HPLC were of HPLC grade.
2.3 Media and growth conditions
Basal salt medium (BSM, containing 1.0 g K2HPO4, 1.0 g (NH4)2SO4, 0.2 g MgSO4·7H2O, 0.01 g FeCl3, 0.05 g NaCl and 0.05 g CaCl2 per liter, pH 7) and Luria-Bertani (LB) medium (10 g Peptone, 10 g Na Cl, 5 g Bacto Yeast extract per liter, pH 7) were used for isolation and cultivation of BPA and phenol utilizing bacteria. BPA was added to BSM and LB medium through 2 methods. In some experiments, BPA as a sole carbon source was added to the above-mentioned media before autoclaving at a final concentration of 300 mg L−1 (BSMB (300) and LB-BPA (300)), unless other concentrations stated. In the other experiments, BPA solution (1 g L−1) was prepared by dissolving 100 mg of BPA in 5 mL of pure ethanol (99%) and adding distilled water up to 100 mL and resulting BPA solution was added to BSM (BSMBE); at final concentrations of 300 mg L−1 and 1% (v/v), for BPA and ethanol respectively.25 Moreover, phenol–BPA degradation activity of the selected isolates was measured in BSMB 300 containing 50 mg L−1 phenol.
Growth and phenol, BPA-degradation activity of the selected bacterial isolates in the original petrochemical wastewater were also confirmed in 250 mL Erlenmeyer flasks containing Khuzestan petrochemical wastewater (PWW) and supplemented with concentrated solutions of BSM (PWW-BSM) and 200 mg L−1 BPA (PWW-BSMB (200)). After incubation of cultures with rotary shaking in dark (200 rpm at 30 °C), growth was monitored based on absorbance at 600 nm (OD600) spectrophotometrically (Beckman, USA) during different cultivation times. Solid BSMBE and BSMB containing 300 mg L−1 BPA and 1.5% (w/v) pure agar were used for colony purifying and growth of the individual isolates of the bacterial consortium (from 2 to 4 days of incubation at 30 °C).
2.4 Isolation of BPA utilizing bacteria
In primary screening experiments, 1 mL of each petrochemical wastewater samples were inoculated directly into 50 mL of BSMBE (100) containing 20 g L−1 NaCl. The resultant cultures were incubated at 200 rpm and 30 °C for 7 days. When the turbidity was appeared, 1 mL of the grown culture media was transferred in a stepwise manner into 50 mL fresh BSMB (200) and BSMB (300) containing 40 g L−1 NaCl for secondary screening. Finally, the grown bacterial cells in BSMB (300) containing 40 g L−1 NaCl were cultivated on BSMB (300) agar plates. The morphologically distinct bacterial isolates were purified on the BSMB (300) plates by repeated streak plate method and stored in 30% (v/v) glycerol and 1% (v/v) tryptone solution at −70 °C.
2.5 Effects of temperature and pH on the growth of selected isolate
Five mL of LB medium was inoculated with a colony of selected isolate from BSMB (300) agar plate. The culture was incubated at 37 °C and 200 rpm until OD600 of 0.6. Thereafter, the resultant pre-culture was inoculated into 50 mL of BSMB (300) containing 40 g L−1 NaCl at final OD600 of 0.2. Cultures were incubated under shaking (200 rpm) at 25, 30, 35 and 40 °C for 48 h. At 12 h intervals, the OD600 of each culture was determined spectrophotometrically (Beckman, USA). The effect of pH on the growth rates of the selected isolate was determined by cultivation of pre-culture in BSMB (300) containing 40 g L−1 NaCl media with pH 5, 6, 6.5, 7, 7.5, 8 and 9 under the same above-mentioned conditions. During the growth of the cultures, OD600 were determined periodically as described previously.
2.6 Chemical oxygen demand (COD) reduction
The cells from log-phase culture (18 h at 37 °C) of selected isolate in LB medium were harvested by centrifugation (5000 rpm, 20 min). The pellet was re-suspended in BSM medium and washed twice. Then, appropriate amount of the resulting suspension was inoculated in 50 mL BSMB (300) containing 40 g L−1 NaCl to obtain an initial absorbance of 0.2 at 600 nm. The culture was grown in an incubator shaker at 30 °C and 200 rpm for 48 h. Samples were collected from the culture at time points of 6, 12, 18, 24, 30, 36, 42 and 48 h and centrifuged at 13
000 rpm for 5 min. The supernatant was used for estimation of COD (chemical oxygen demand) accordance to the instruction (no. 5220-B), in the “open reflux method”.32 Each COD value indicated the mean value of the two independent determinations repeated each time in duplicate.
2.7 Characterization and molecular identification of the selected isolate
Morphological and biochemical tests of selected isolate such as Gram-staining and specification of colony in terms of color, surface, margin, size, and transparency as well as oxidase and catalase tests were performed. For molecular identification, the selected isolate was cultivated in liquid LB medium (at 37 °C, 180 rpm for 18 h). DNA was extracted and purified from the cells collected by centrifugation (13
000 rpm, 5 min) using DNA purification kit (Najm Biotech; Iran). The 16S rDNA fragment was amplified by PCR using Taq DNA polymerase and universal primer pair of fD1 (5′AGAGTTTGATCCTGGCTTAG3′) (10 pmol) and rD1 (5′TAAGGAGGTGATCCAGCC3′) (10 pmol).33 The PCR conditions were as follows: 10 min at 95 °C; followed by 30 cycles of 95 °C for 1 min, 50 °C for 1 min, 72 °C for 1 min; and a final extension step of 10 min at 72 °C. The amplified 16S rDNA fragment was inserted into the T/A cloning vector. Then, recombinant vectors were introduced into E. coli (DH5α) cells and the recombinant colonies were selected by plating on the LB medium supplemented with 100 μg mL−1 ampicillin, 0.2 mM IPTG and 40 μg mL−1 X-gal.34 Finally, the vectors carrying amplicons were extracted using High Pure Plasmid Isolation Kit (Roche, Germany) and sequencing of 16S rDNA was performed in both directions using M13 universal primers by the dideoxy termination method with an ABI automated sequencer (Bioneer, korea). GenBank and EzBioCloud databases were used for alignment of the 16S rDNA sequence of the selected isolate with available 16S rDNA sequences.
2.8 Determination of phenol and BPA degradation
Selected isolate was pre-cultured in LB medium and grown aerobically under shaking (200 rpm) for 18 h at 37 °C. The cells were centrifugally separated (5000 rpm, 20 min) and washed twice with 5 mL of fresh culture medium. The cells inoculated into BSMB (300), BSMB (300) containing 50 mg L−1 phenol, PWW (petrochemical wastewater), PWW-BSM and PWW-BSMB (200) media at an OD600 of 0.2 and incubated under shaking (200 rpm) at 30 °C for 48 h. After incubation, samples (1 mL) were collected from the cultures at certain time points and centrifuged at 13
000 rpm for 5 min. The resultant supernatants were filtrated through a 0.2μ membrane filter (Millipore. USA). The amount of phenol and BPA in the filtrates were determined by a high performance liquid chromatography (HPLC) and reverse phase C18 column (4.6 × 250 mm, 5 mm Zorbax RX-C18). The samples were eluted with a linear gradient (10–90% acetonitrile–water) at 1 mL min−1 for 40 min. The injection volume was 25 μL and the absorbance was monitored at 280 nm.
The decrease of phenol and BPA in the samples was calculated from the remained phenol and BPA in the samples, compared with the phenol and BPA content of the controls (non-inoculated media). All phenol and BPA-degradation experiments were carried out in duplicates and the data presented are the average of duplicate experiments.
2.9 Identification of BPA metabolites
Three compounds of 4-HAP, 4-HBAL, and 4-HBA acid were previously reported as BPA degradation metabolites of Sphingomonas sp. strain MV1, Pseudomonas alkylphenolica and other bacterial strains in the KEGG database. For identification of metabolic intermediary compounds of BPA-biodegradation pathway in the selected isolate, the standard solutions of three above-mentioned compounds (200 mg L−1) and BPA (300 mg L−1) were prepared in the BSM medium and analyzed by HPLC. The metabolites derived from the biodegradation of BPA by selected isolate were identified based on comparison and matching of the peak retention time belonged to known (standard) and unknown compounds.
2.10 Identification of metabolites from BPA decomposition with gas chromatography-mass spectrometry (GC/MS)
The results of HPLC were also evaluated by GC/MS (Agilent 7000 Series Triple Quad. Column 30 m × 250 μm × 0.25 μm). The samples were prepared according to Thermo Fisher scientific, Instruction BSTFA, 0255.1, 2008. Then the prepared samples were analyzed by the GC/MS (Shahid Beheshti Research Comprehensive Laboratory-Iran).
2.11 Phenol and BPA removal from petrochemical wastewater in batch fermenter
Pre-culture for fermentation was prepared by inoculating 200 mL LB medium with a single colony of selected isolate. Flasks were incubated 18 h at 37 °C aerobically under shaking at 200 rpm. Batch fermentation was carried out in a 2 L fermenter with 1.2 L working volume (Bioflo 3000; New Brunswick Scientific Co., New Jersey, USA). The fermenter was equipped with a built-in controller for pH, temperature, agitation, dissolved oxygen (DO), and peristaltic pumps for base and acid additions. Pre-culture was centrifuged (5000 rpm, 20 min) and collected cells washed twice with fresh BSM medium. The washed cells inoculated into PWW, PWW-BSM and PWW-BSMB (300) at 5% (v/v) in separate batch tests. After inoculation, the temperature and pH of fermenters were automatically maintained at 35 °C and 7, respectively. The DO was maintained automatically at 10% by controlling the agitation speed up to 500 rpm. Sampling was carried out at certain time points. Phenol and BPA concentration of samples was determined using HPLC analysis at the same condition mentioned in Section 2.8.
3 Results
3.1 Isolation and characterization of BPA utilizing bacteria
Each collected sample was inoculated into liquid BSMBE (100) containing 20 g L−1 NaCl and cultivated at 30 °C for 7 days. Considerable turbidity (OD600 = 0.6–1.1) was observed in 4 samples during 1 to 3 days of incubation. For obtaining bacteria with higher BPA-degrading activity in salty conditions, four grown samples were cultured in BSMB containing 200 and 300 mg L−1 BPA as sole carbon source and 40 g L−1 NaCl using stepwise enrichment manner. Only one BSMB (300) culture showed turbidity after 24 h; suggesting the existence of BPA-degrading and NaCl-tolerant bacteria in that sample. Colony purification was performed by spreading of the grown liquid culture on BSMB (300) agar plate. All colonies on the solid medium were derived from one bacterial strain on basis of the colony morphology. Consequently, one isolate was selected for further experiments and designated as YKJ isolate.
The YKJ isolate was a Gram-negative, catalase and oxidase-positive bacilliform bacterium. Colonies of this isolate on LB agar plates were milk-white (1–2 mm size), non-transparent, circular with convex appearance, and smooth margin. Antibiotics resistance evaluation on LB agar plates containing different antibiotics and antibiogram test (Fig. 1) showed sensitivity of YKJ isolate to rifampicin (100 μg L−1), kanamycin (50 μg L−1), and tetracycline (25 μg L−1) and its resistance to ampicillin (50 μg L−1) and chloramphenicol (34 μg L−1) as previously realized genetically.35
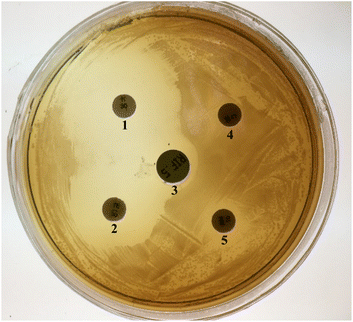 |
| Fig. 1 A representative antibiogram figure of the YKJ isolate. Kanamycin (1), tetracycline (2), rifampicin (3), chloramphenicol (4), ampicillin (5). | |
3.2 Determination of growth conditions
The growth parameters including suitable temperature and pH were determined for YKJ isolate in the presence of BPA as sole carbon and energy sources in salty condition. The results showed that the isolate was able to grow in liquid BSMB (300) containing 40 g L−1 NaCl at 25, 30, 35 and 40 °C. In addition, measuring OD600 of collected samples within 12, 24, 36 and 48 h of cultivation demonstrated the higher growth rate of YKJ isolate at 30 °C compared to other temperatures (Fig. 2). Therefore, temperature of 30 °C was applied as one of the growth parameters in further experiments.
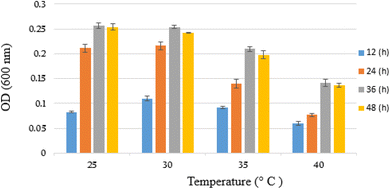 |
| Fig. 2 Growth (OD600) of YKJ isolate in BSMB (300) containing 40 g L−1 NaCl within 12, 24, 36 and 48 h at temperatures of 25, 30, 35 and 40 °C. | |
Subsequently, the growth rate (OD600) of YKJ isolate was evaluated in liquid BSMB (300) containing 40 g L−1 NaCl at pH of 5–9 and temperature of 30 °C. OD600 of cultures after 12, 24, 36 and 48 h of incubation showed that YKJ isolate was able to grow at all tested pHs except 5. However, the best growth of this isolate was at pH of 6.5, 7 and 7.5. The growth pattern in different pHs indicated that growth of the YKJ isolate was not limited to a specific pH (Fig. 3). Moreover, the potential of YKJ isolate for growth in high concentrations of NaCl (up to 40 g L−1) as well as different pHs (6–9) and temperatures (25–40 °C) can presumably illustrate the ability of the isolate to grow in the conditions existing in the petrochemical wastewater.
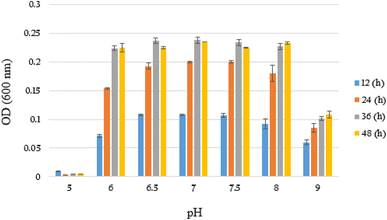 |
| Fig. 3 Growth (OD600) of YKJ isolate in BSMB (300) containing 40 g L−1 NaCl within 12, 24, 36 and 48 h after cultivation at pH of 5, 6, 6.5, 7, 7.5, 8, 9 and temperature of 30 °C. | |
3.3 Chemical oxygen demand (COD) reduction by YKJ isolate
BPA-degradation activity of YKJ isolate was determined by measuring the COD of the isolate culture in BSMB (300) containing 40 g L−1 NaCl within 6, 12, 18, 24, 30, 36, 42 and 48 h of cultivation. According to the results, the COD of above-mentioned culture reduced from the initial value of 655.2 mg L−1 to 109.2 mg L−1 (about 83% decrease) after 36 hours and remained almost constant up to 48 hours (Fig. 4). Also, growth monitoring of the culture at the same time points showed close correlation between growth rate of YKJ isolate and COD reduction in BSMB (300) containing 40 g L−1 NaCl (Fig. 4). Reducing COD of BSMB (300) culture and increasing the growth of YKJ isolate could indicate the ability of this isolate for utilization of BPA as sole carbon and energy sources.
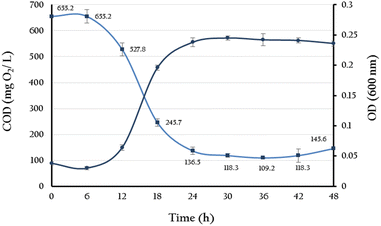 |
| Fig. 4 Correlation between growth (OD600) of YKJ isolate in BSMB (300) containing 40 g L−1 NaCL ( ) and reducing COD of the resulting culture ( ). | |
3.4 Identification of the YKJ isolate
The 16S rDNA sequence of the YKJ isolate was aligned with available 16S rDNA sequences in the NCBI and EzBioCloud databases using BLAST algorithm. The analysis of 16S rDNA sequence of the YKJ isolate showed more than 99% identity to Pseudomonas alcaliphila JCM 10630 and chengduensis MBR strains in NCBI and EzBioCloud databases respectively. Due to the highest similarity to many Pseudomonas species, the isolate was named as Pseudomonas sp. strain YKJ. The sequence was deposited in the Gen Bank under Accession number of KR229982. The isolate was subsequently genetically characterized through comprehensive genomic analysis as Pseudomonas pseudoalcaligenes strain YKJ.35
3.5 BPA-biodegradation activity assay
BPA-degradation activity of P. pseudoalcaligenes strain YKJ was also confirmed in BSMB (300) containing 40 g L−1 NaCl by HPLC analysis. According to the chromatogram of the HPLC, the retention time of BPA was 23.772 min (Fig. 5a). The calibration curve equation for detection of the BPA concentration was as follows: peak area = 21.12CBPA − 9.742 (R2 = 0.999), where CBPA was the BPA concentration (within the range of 1–300.0 mg L−1). The results (Fig. 5b–e) demonstrated that BPA at 300 mg L−1 was reduced to 243.7, 97.57, 11.14 and 0 mg L−1 by P. pseudoalcaligenes strain YKJ within 12, 18, 24, and 36 h, respectively. This strain was able to degrade high levels (288.86 mg L−1) of BPA within 24 h (Fig. 5d) and utilize 100% (300 mg L−1) of BPA without detectable new peaks in HPLC analysis within 48 h (Fig. 5f). Therefore, P. pseudoalcaligenes strain YKJ can utilize BPA as sole carbon source to produce CO2, H2O and all cell components.
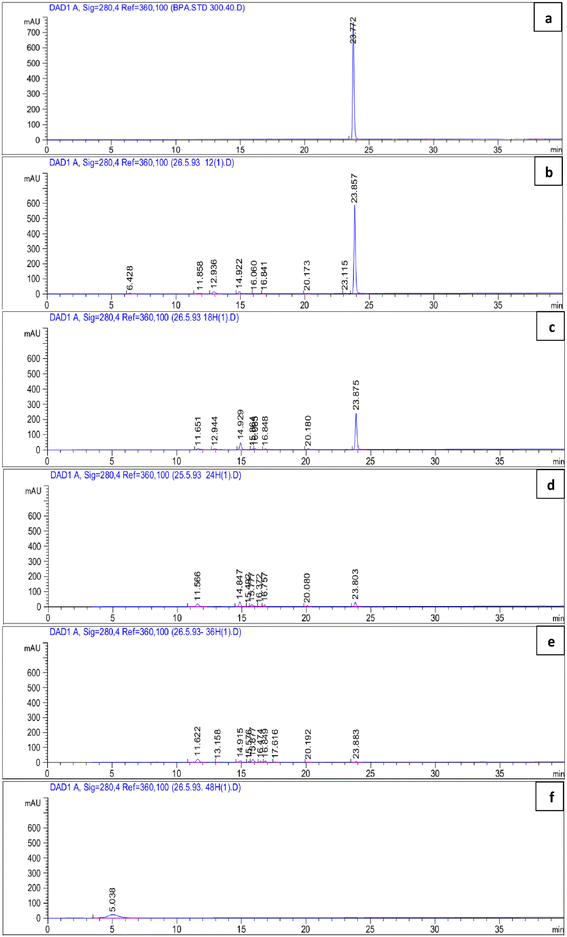 |
| Fig. 5 The chromatograms of the HPLC system for measurement of 300 mg L−1 BPA in BSM medium (a), and metabolites of BPA degradation by P. pseudoalcaligenes strain YKJ in BSMB (300) containing 40 g L−1 NaCl after 12 h (b), 18 h (c), 24 h (d), 36 h (e) and 48 h (f) (linear gradient 10–90% acetonitrile–water; flow rate: 1 mL min−1). | |
3.6 Metabolic intermediary compounds and metabolic pathway of BPA biodegradation
In metabolic pathways identified for BPA biodegradation in some bacterial strains such as Pseudomonas alkylphenolica (KEGG database, PATHWAY: map00363), 4-HAP, 4-HBAL, and 4-HBA are detected as intermediate compounds. For identification of BPA metabolic pathways in P. pseudoalcaligenes strain YKJ, a mixture of the above-mentioned intermediary compounds as well as BPA were analysed by HPLC. In resulting chromatogram, the retention times of BPA, 4-HAP, 4-HBAL, and 4-HBA peaks were 23.820, 12.863, 11.890 and 9.401 min, respectively (Fig. 6a). This chromatogram was applied as standard for identification of metabolites produced during biodegradation of BPA by P. pseudoalcaligenes strain YKJ. In this way, HPLC analysis of collected samples within 18 h after cultivation of strain YKJ in BSMB (300) containing 40 g L−1 NaCl (Fig. 6b) was compared to standard one. In chromatogram of samples, two peaks were in retention times similar to the peaks of 4-HAP and 4-HBAL in the standard chromatogram. Therefore, these two metabolic intermediary compounds were considered as some intermediates resulted in BPA degradation by P. pseudoalcaligenes strain YKJ. This result suggested that metabolic pathway for BPA biodegradation by strain YKJ might be the same as those identified in strain MV1 (ref. 19 and 30) or recorded in KEGG database for P. alkylphenolica.
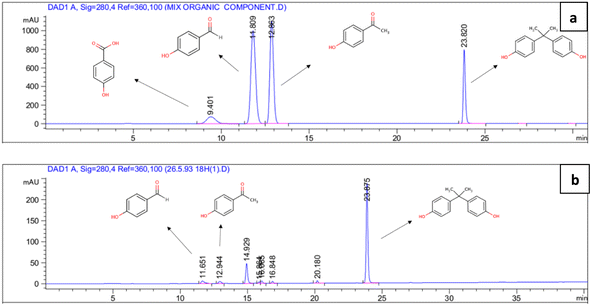 |
| Fig. 6 Chromatogram of the HPLC system for detection of retention times of 4-HBA, 4-HBAL, 4-HAP and BPA peaks (a). HPLC analysis of BPA biodegradation by P. pseudoalcaligenes strain YKJ in BSMB (300) containing 40 g L−1 NaCl after 18 h (b). | |
Furthermore, the peak of BPA was disappeared in HPLC analysis of collected samples after 36 h (Fig. 5e). However, the peaks belonged to the by-products of BPA degradation by strain YKJ were yet being seen in the above-mentioned HPLC analysis. Therefore, these metabolic intermediary compounds of BPA degradation were also evaluated using GC/MS. The organic compounds such as 4-hydroxyphenylacetate, M-hydroxymandelic acid, 2-phenylpropane-1,2-diol, 2-phenyl-2-propanol and lactic acid were detected in GC/MS analysis (Fig. 7).
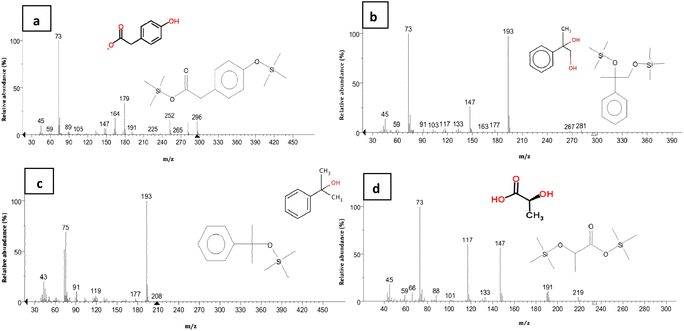 |
| Fig. 7 Mass spectra from GC/MS during BPA-degradation by P. pseudoalcaligenes strain YKJ in BSMB (300) containing 40 g L−1 NaCl after 36 h and detected intermediate compounds, 4-hydroxyphenylacetate (a), M-hydroxymandelic acid (b), 2-phenylpropane-1,2-diol (c), 2-phenyl-2-propanol (d). | |
4-Hydroxyphenylacetate has been previously identified as decomposition product of 4-HAP in major pathway of BPA biodegradation by strain MV1.19,30 Disappearance of 4-HAP peak (retention time = 12.863 min) in HPLC analysis after 36 h (Fig. 5e) and then identification of 4-hydroxyphenylacetate by GC/MS analysis (Fig. 7a) confirmed that strain YKJ could degrade BPA in pathway similar to the major pathway for BPA biodegradation by strain MV1.19,30 4-HBAL peak was also appeared in HPLC analysis 12, 18, 24 and 36 h after cultivation and was disappeared after 48 h (Fig. 5). Although, peak of 4-HBA acid was not detected in none of HPLC analysis but presumed as decomposition product of 4-HBAL in the pathway. In this way, the major BPA metabolic pathway in strain MV1 and P. alkylphenolica (KEGG database, PATHWAY: map00363) was proposed for BPA biodegradation by strain YKJ (Fig. 8). Therefore, strain YKJ probably hydroxylates BPA to 1,2-bis(4-hydroxyphenyl)-2-propanol which is then dehydrated to 4,4-dihydroxy-alpha-methylstilbene. This compound is further oxidized to 4-HBAL and 4-HAP. 4-HAP is metabolized to 4-hydroxyphenylacetate (4-HPA) and then to hydroquinone (HQ). Both of hydroxybenzoic acid (HBA) and hydroquinone are assumed to mineralize to carbon dioxide (CO2) and bacterial biomass through benzoate degradation pathway (Fig. 8). Furthermore, other compounds identified by GC/MS including M-hydroxymandelic acid, 2-phenylpropane-1,2-diol, 2-phenyl-2-propanol and lactic acid can also indicate that new BPA metabolic pathways might exist in the P. pseudoalcaligenes strain YKJ.
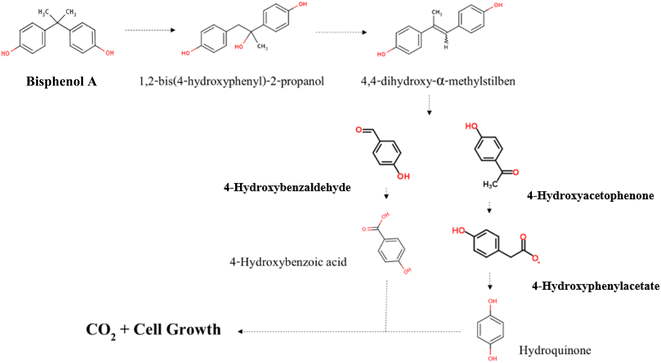 |
| Fig. 8 The proposed metabolic pathway for BPA biodegradation by P. pseudoalcaligenes strain YKJ. This pathway is started by hydroxylation of BPA to form 1,2-bis(4-hydroxyphenyl)-2-propanol and then its dehydration to 4,4-dihydroxy-alpha-methylstilbene. Resulted compound is oxidized to 4-HBAL and 4-HAP. 4-HAP is metabolized to 4-HPA and then to HQ. Both of HBA and HQ are mineralized to CO2 and bacterial biomass. | |
3.7 Phenol and BPA-degradation activity assay
P. pseudoalcaligenes strain YKJ was cultivated in BSMB containing 300 mg L−1 BPA and 50 mg L−1 phenol as carbon sources (30 °C, 200 rpm). After 24 and 48 h, the growth was monitored by measuring the OD600 and the residual concentration of BPA in the cultures was determined by HPLC analysis. The results showed that P. pseudoalcaligenes strain YKJ could grow up to OD600 of 0.575 ± 0.001 and degrade 44.56 mg L−1 phenol (89.12%) and all of the BPA (100%) within 48 h. Therefore, this strain is also able to degrade and utilize phenol along with BPA in the basal salt medium and may metabolize it to CO2, H2O and cell components similar to what was concluded for BPA biodegradation.
Subsequently, the growth and phenol, BPA-degradation activity of P. pseudoalcaligenes strain YKJ were evaluated in PWW and PWW-BSM which contained 100 mg L−1 phenol and 300 mg L−1 BPA on the basis of HPLC analysis. Growth increased to OD600 of 0.597 ± 0.009 in PWW and OD600 of 0.570 ± 0.005 in PWW-BSM until 24 h and thereafter until 48 h, it did not increase. Phenol and BPA was decreased to an undetectable level by HPLC in both cultures within 24 h (data not shown). Thus, similarity of the results in both cultures showed that the BSM mineral salts could not stimulate the growth and phenol, BPA-degradation activity of P. pseudoalcaligenes strain YKJ in PWW. In addition, this strain was able to grow up to OD600 of 0.5 and degrade 100 mg L−1 phenol (100%) and 300 mg L−1 BPA (100%) in PWW and PWW-BSM within 24 h which were higher than that in the BSMB containing 300 mg L−1 BPA and 50 mg L−1 phenol within 48 h. Therefore, it was probable that other organic compounds existing in the petrochemical wastewater could stimulate the growth and degradation activity of P. pseudoalcaligenes strain YKJ. In addition, other living microorganisms existing in petrochemical wastewater might synergistically enhance the growth and BPA degradation activity of this strain.
In the other experiment, P. pseudoalcaligenes strain YKJ was cultured in PWW (100 mg L−1 phenol and 300 mg L−1 BPA) supplemented with concentrated solutions of BSM and 200 mg L−1 BPA (PWW-BSMB (200)). Growth in this culture increased up to OD600 of 0.70 ± 0.011 within 24 h and the amounts of phenol and BPA removal were 100 mg L−1 (100%) and 453.05 mg L−1 (90.61%) within 48 h, respectively. Therefore, it was concluded that increasing BPA concentration in PWW-BSM not only does not inhibit the growth and phenol, BPA-degradation activity of the strain but also enhance them. These results suggest that this strain is promising for the bioremediation of wastewater containing phenol and BPA.
3.8 Phenol and BPA-removal in petrochemical wastewater under batch fermentation conditions
In order to evaluate phenol and BPA-removal activity of strain YKJ in conditions closer to those of petrochemical wastewater treatment by aeration and activated sludge method, batch fermentation process was performed. Three fermenters containing PWW, PWW-BSM and PWW-BSMB (300) were inoculated with 5% (v/v) of YKJ strain pre-culture, separately. In the first batch test, growth increased to OD600 of 0.113 ± 0.002 in PWW within 12 h. Phenol concentration was slightly reduced up to 8% of initial concentration while 57% of BPA was removed during this time. In the second batch test, strain YKJ grew up to OD600 of 0.666 ± 0.013 and degraded 100 mg L−1 phenol (100%) and 111 mg L−1 BPA (37%) in PWW-BSM within only 6 h. It is noteworthy that such growth rate and phenol degradation activity was almost similar to those obtained in flask conditions within 24 h. Furthermore, BPA-removal activity of strain YKJ in this test within 6 h was one third of that in flask containing PWW-BSM within 24 h. Phenol and BPA were also removed in batch fermentation of this strain in PWW-BSM at almost similar rates. Strain YKJ was able to grow up to OD600 of 0.818 ± 0.021 and degrade 100 mg L−1 phenol (100%) and 372 mg L−1 BPA (62%) in the third batch fermenter containing PWW-BSMB (300) within 6 h. Similar to flask conditions, increasing BPA concentration in PWW-BSM led to enhance growth and BPA-degradation activity of strain YKJ. In all three batch fermentation tests, the strain YKJ was able to further grow and degrade higher phenol and BPA amounts relative to the flask conditions during a time equivalent to a quarter of the flask conditions. From a practical and operational point of view, reducing the growth time of the bacterium while maintaining its function is extremely important.
4 Discussion
The success of microbial bioremediation depends mainly on the ability of the microorganism to grow and metabolize organic pollutants in the contaminated environments.36 Thus, use of bacterial strains isolated from environments exposed to BPA for the treatment of this hazardous pollutant is promising. In the present study, P. pseudoalcaligenes strain YKJ was isolated from an outlet of petrochemical wastewater exposed to BPA for a long time. This strain was able to grow in basal salt medium containing 300 mg L−1 BPA and 40 g L−1 NaCl and degrade 96.28% of BPA within 24 h (Fig. 5d). It is worthy to notice that YKJ strain could utilize 100% (300 mg L−1) of BPA without producing detectable metabolites based on HPLC analysis within 48 h (Fig. 5f). While previous studies have shown obvious estrogenic activities of some BPA metabolites that may create new environmental pollution concerns.29,37 Up to now, many bacteria with BPA-biodegradation activity have been isolated from different environments, but only a few of them have a strong capacity for its biodegradation.3–5,8,9,11,16–22,24,26–29,31 However, strains that can quickly and completely degrade and utilize BPA as sole carbon source without accumulation of intermediate compounds in the medium are more desirable. Pseudomonas sp. strain KU1, KU2 and Bacillus sp. strain KU3 isolated by Kamaraj et al.17 (2014) were relatively potent strains for the BPA biodegradation and could degrade 780, 810 and 740 mg L−1 of BPA in a basal medium within 12 days, respectively. Eltoukhy et al.3 (2020) also isolated Pseudomonas putida strain YC-AE1 that could degrade 200 mg L−1 within 20
h under optimum environmental factors. In comparison to above-mentioned strains, our strain could degrade higher amounts of BPA (288.86 mg L−1) within shorter time (24 h or 1 day). Furthermore, strain YKJ utilized this amount of BPA in the presence of 40 g L−1 NaCl. This is great advantage, because high concentrations of inorganic salts are often present in industrial wastewaters containing BPA that affect biodegradation efficiency. For example, aromatic polycarbonates are mainly produced through the interfacial polycondensation of BPA with phosgene. In this process a large amounts of inorganic salts such as NaCl are produced as byproducts, thereby dilution of resulted wastewater with a large amount of water is necessary for biotreatment of BPA.31 To our knowledge, only Bacillus pumilus strains BP-2CK, BP-21DK, and BP-22DK isolated from Kimchi exhibited BPA-degradation activity in the rich medium containing 10% NaCl. But, these strains were not able to grow in the mineral salt medium containing BPA as a sole carbon source. Moreover, BP-2CK, BP-21DK, and BP-22DK strains had low potential for BPA biodegradation and showed neither degradation activity nor cell growth in the rich medium containing 100 mg L−1 of BPA.31 No other BPA-degrading bacteria have been reported to show activity in the presence of such high concentrations of NaCl. Even, in 2020, Jia et al.5 reported that BPA-biodegradation efficiency of Sphingobium sp. YC-JY1 was significantly inhibited in 6 to 10 g L−1 NaCl. Their results indicated that NaCl has a negative effect in BPA degradation by Sphingobium sp. YC-JY1. In addition, ability of strain YKJ for BPA removal in high concentrations of NaCl is significant because different outlets of Khuzestan petrochemical wastewater and Fajr wastewater treatment plant contain 40 g L−1 NaCl. Therefore, experiments in a small-scale system for BPA removal from these outlets, as main purpose of current study, is useful for design and construction of wastewater treatment system for the above-mentioned petrochemical wastewater (Table 1).
Table 1 Comparison of BPA-biodegradation activity in YKJ strain and the other high potential bacterial strains
Microorganism |
BPA concentration mg L−1 |
NaCl concentration g L−1 |
BPA removal (%) |
Time (h) |
Ref. |
P. pseudoalcaligenes strain YKJ |
300 |
40 |
96.28 |
24 |
Present study |
Pseudomonas putida strain YC-AE1 |
200 |
0 |
100 |
20 |
Eltoukhy et al. (2020)3 |
Sphingobium sp. YC-JY1 |
100 |
0 |
100 |
12 |
Jia et al. (2020)5 |
Sphingobium sp. YC-JY1 |
100 |
6–10 |
0 (inhibited) |
10 |
Jia et al. (2020)5 |
Pseudomonas sp. strain KU1 |
1000 |
0 |
78 |
288 |
Kamaraj et al. (2014)17 |
Pseudomonas sp. strain KU2 |
1000 |
0 |
81 |
288 |
Kamaraj et al. (2014)17 |
Bacillus sp. strain KU3 |
1000 |
0 |
74 |
288 |
Kamaraj et al. (2014)17 |
Enterobacter gergoviae strain BYK-7 |
200 |
0 |
11.55 |
8 |
Badiefar et al. (2015)25 |
Bacillus pumilus strains BP-2CK |
25 |
10 |
100 |
48 |
Yamanaka et al. (2007)31 |
Bacillus pumilus strains BP-21DK |
25 |
10 |
100 |
48 |
Yamanaka et al. (2007)31 |
Bacillus pumilus strains BP-22DK |
50 |
10 |
100 |
120 |
Yamanaka et al. (2007)31 |
In our previous project, we investigated on BPA-degradation activity of one new isolated Enterobacter gergoviae strain BYK-7 in the petrochemical wastewater. Our results showed that this strain was able to degrade 31.35 ± 4.05 mg L−1 BPA in PWW and 78.33 ± 5.26 mg L−1 BPA in PWW-BSM within 72 h, respectively.25 In current study, we succeeded to isolate strain YKJ with so higher potential for BPA removal in petrochemical wastewater. This strain is able to degrade 300 mg L−1 BPA and 100 mg L−1 phenol in both PWW and PWW-BSM within 24 h as well as 453.05 mg L−1 BPA and 100 mg L−1 phenol in PWW-BSMB (200) within 48 h without detectable by products in HPLC analysis. Furthermore, batch fermentation of petrochemical wastewater using strain YKJ enhanced its ability for complete BPA biodegradation so that it could degrade 372 mg L−1 BPA and 100 mg L−1 phenol in PWW-BSMB (300) within 6 h. The more remarkable point is that BPA biodegradation rate of strain YKJ enhanced from 18.5 mg L−1 h−1 to 62 mg L−1 h−1 by increasing BPA concentration at controlled conditions of batch fermentation. According to all of the above, the strain YKJ has high BPA-degradation potential in basal salt medium and petrochemical wastewater containing high concentrations of BPA and NaCl, which is so promising for application in practical BPA removal systems.
Sasaki et al.38 (2008) indicated that bisdAB are key genes for BPA degradation in S. bisphenolicum AO1 and expression of these genes in Escherichia coli BL21 (DE3) increased its BPA-biodegradation activity. Results of our previous study also confirmed increasing BPA-biodegradation activity of E. gergoviae BYK-7 expressing bisdAB genes in BSMB (200) from 23 mg L−1 of BPA to 45 mg L−1.25 Therefore, it is probable that modification of the BPA-degradation pathways of strain YKJ using genetic engineering techniques as well as optimization of its environmental conditions increase the potential of this strain for BPA-degradation and its application in the treatment of salty petrochemical wastewater containing high amounts of BPA.
It is also worth noting that biodegradation of PAHs as BPA depends on environmental factors such as temperature, pH, and salinity.39–42 These parameters have important effects on the growth of bacteria and catabolic activity of the enzymes involved in the BPA-biodegradation process. The P. pseudoalcaligenes YKJ can grow in a temperature range of 25–40 °C with optimum growth temperature of 30 °C. Increasing the temperature improves solubility of the BPA, and thus, significantly increases the bioavailability of BPA molecules. In return, higher temperature reduces the metabolic activity of mesophilic aerobic microorganisms which is also seen as reduced growth of this bacterium in BSMB (300) containing 40 g L−1 NaCl. Optimum growth of YKJ strain at 30 °C can be due to the optimum temperature for activity of the enzymes involved in the BPA-biodegradation pathway that is lower than 40 °C. pH of the medium also affects microbial activity including enzymatic activity, solubility, and accessibility of nutrients.43 P. pseudoalcaligenes YKJ is able to grow at pH 6–9 with the best growth in the pH of 6.5–7.5. The growth pattern indicates that the BPA-biodegradation activity of this strain is not limited to a specific temperature and pH (Fig. 2 and 3). These results again suggest that strain YKJ may be suitable for industrial application.
Most antibiotic resistance genes are located in bacterial plasmids which can be horizontally transferred between environmental bacteria. Therefore, the strains with the least resistance to antibiotics should be considered for potential applications to minimize environmental risks. P. pseudoalcaligenes YKJ is resistant to Chloramphenicol and slightly to Ampicillin (which can be removed through genetic engineering approach) but not to other antibiotics. These characteristics may be suited for the bioremediation purpose.35
We hypothesized that, P. pseudoalcaligenes YKJ utilizes a pathway similar to major pathway for the BPA metabolism by strain MV1, in which the compound is oxidized to 1,2-bis(4-hydroxyphenol)-2-propanol, 4-HBAL, and 4-HAP.19,30 During BPA biodegradation by strain YKJ, 4-HAP was detected as a degradation intermediate, while 4-HBA was not detected. Really, degradation of 4-HAP by strain YKJ was slower than that of 4-HBA, exactly like what Sakai et al. (2014) reported about BPA-biodegradation by Sphingomonas sp. strain BP-7.7 They suggested that the degradation pathway of 4-HAP of the strain is a rate-limiting step in the degradation of BPA, due to low activities of initial enzymes in the pathway or an inhibition effect of 4-HAP on the growth of the strain. The same conclusion can also be true about strain YKJ.
5 Conclusion
We succeeded to isolate and identify a novel BPA-degrading P. pseudoalcaligenes strain YKJ. It was able to degrade BPA as the only source of carbon and energy in the basal salt medium containing high concentration of NaCl more rapidly than the other reported bacteria. BPA biodegradation pathways by this strain were proposed based on the analysis of the metabolites. Our results showed that strain YKJ was applicable for treatment of salty petrochemical wastewater containing high concentrations of phenol and BPA.
Author contributions
Each author contributed to this project as following: Bagher Yakhchali, contributed to perusing conception, project design, also identified the bacterial species (Pseudomonas pseudoalcaligenes strain YKJ) and critically revising the manuscript for momentous intellectual content and ultimate approval of the version to be published. Sasan Jahanshahi isolated the bacterial strain (Pseudomonas pseudoalcaligenes strain YKJ) and carried out the first phase of laboratory work. Leila Badiefar contributed to scrutinizing conception and design, analysis and interpretation of data, writing the manuscript and response to reviewers for ultimate approval of the version to be published. Mahvash Khodabandeh contributed to designing laboratory procedures to isolate and characterize bacterial species and revising the manuscript. Mohammad Ali Heidarnia contributed to characterizing the effluent samples and performed the second phase of the laboratory work.
Conflicts of interest
The authors declare that they have no conflicts of interest.
Acknowledgements
We gratefully acknowledge the partial financial grant received from the National Petrochemical Company (no. 1-109/65899SP) for laboratory work. The authors would like to thank Dr Ghodratollah Nasiry and Mr Mohammadreza Sarafrazy for their great help. We also thank Mrs Elaheh Asiaei from NIGEB for some laboratory help including antibiogram test.
References
- S. Katiyar, R. Agarwal and H. Mukhtar, Boca, 1993, 1–17 Search PubMed.
- K.-H. Kim, S. A. Jahan, E. Kabir and R. J. C. Brown, Environ. Int., 2013, 60, 71–80 CrossRef CAS PubMed.
- A. Eltoukhy, Y. Jia, R. Nahurira, M. Abo-Kadoum, I. Khokhar, J. Wang and Y. Yan, BMC Microbiol., 2020, 20, 1–14 CrossRef PubMed.
- M. Ike, C.-S. Jin and M. Fujita, Jpn. J. Water Treat. Biol., 1995, 31, 203–212 CrossRef.
- Y. Jia, A. Eltoukhy, J. Wang, X. Li, T. S. Hlaing, M. M. Aung, M. T. Nwe, I. Lamraoui and Y. Yan, Int. J. Mol. Sci., 2020, 21, 3588 CrossRef CAS PubMed.
- I. Louati, M. Dammak, R. Nasri, L. Belbahri, M. Nasri, S. Abdelkafi and T. Mechichi, 3 Biotech, 2019, 9, 1–11 CrossRef PubMed.
- K. Sakai, H. Yamanaka, K. Moriyoshi, T. Ohmoto and T. Ohe, Biosci., Biotechnol., Biochem., 2007, 0612070212 Search PubMed.
- V. Vijayalakshmi, P. Senthilkumar, K. Mophin-Kani, S. Sivamani, N. Sivarajasekar and S. Vasantharaj, J. Radiat. Res. Appl. Sci., 2018, 11, 56–65 CrossRef CAS.
- W. Zhang, K. Yin and L. Chen, Appl. Microbiol. Biotechnol., 2013, 97, 5681–5689 CrossRef CAS PubMed.
- K. Nowak, E. Jabłońska and W. Ratajczak-Wrona, Environ. Int., 2019, 125, 350–364 CrossRef CAS PubMed.
- B. Suyamud, D. Inthorn, B. Panyapinyopol and P. Thiravetyan, Water, Air, Soil Pollut., 2018, 229, 1–12 CrossRef CAS.
- E. N. de Freitas, G. A. Bubna, T. Brugnari, C. G. Kato, M. Nolli, T. G. Rauen, R. d. F. P. M. Moreira, R. A. Peralta, A. Bracht and C. G. de Souza, Chem. Eng. J., 2017, 330, 1361–1369 CrossRef.
- E. J. Eio, M. Kawai, K. Tsuchiya, S. Yamamoto and T. Toda, Int. Biodeterior. Biodegrad., 2014, 96, 166–173 CrossRef.
- Y. Huang, C. Wong, J. Zheng, H. Bouwman, R. Barra, B. Wahlström, L. Neretin and M. H. Wong, Environ. Int., 2012, 42, 91–99 CrossRef CAS PubMed.
- D. Mohapatra, S. Brar, R. Tyagi and R. Surampalli, Chemosphere, 2010, 78, 923–941 CrossRef CAS PubMed.
- J. Fischer, U. Kappelmeyer, M. Kastner, F. Schauer and H. J. Heipieper, Int. Biodeterior. Biodegrad., 2010, 64, 324–330 CrossRef CAS.
- M. Kamaraj, R. Sivaraj and R. Venckatesh, Int. Biodeterior. Biodegrad., 2014, 93, 216–222 CrossRef CAS.
- G. Li, L. Zu, P.-K. Wong, X. Hui, Y. Lu, J. Xiong and T. An, Bioresour. Technol., 2012, 114, 224–230 CrossRef CAS PubMed.
- J. H. Lobos, T. Leib and T.-M. Su, Appl. Environ. Microbiol., 1992, 58, 1823–1831 CrossRef CAS PubMed.
- M. Noszczyńska, M. Chodór, Ł. Jałowiecki and Z. Piotrowska-Seget, Biodegradation, 2020, 1–15 Search PubMed.
- Z. Ronen and A. Abeliovich, Appl. Environ. Microbiol., 2000, 66, 2372–2377 CrossRef CAS PubMed.
- T. Toyama, Y. Sato, D. Inoue, K. Sei, Y.-C. Chang, S. Kikuchi and M. Ike, J. Biosci. Bioeng., 2009, 108, 147–150 CrossRef CAS PubMed.
- H. Yamanaka, K. Moriyoshi, T. Ohmoto, T. Ohe and K. Sakai, J. Biosci. Bioeng., 2008, 105, 157–160 CrossRef CAS PubMed.
- C. Zhang, G. Zeng, L. Yuan, J. Yu, J. Li, G. Huang, B. Xi and H. Liu, Chemosphere, 2007, 68, 181–190 CrossRef CAS PubMed.
- L. Badiefar, B. Yakhchali, S. Rodriguez-Couto, A. Veloso, J. M. García-Arenzana, Y. Matsumura and M. Khodabandeh, RSC Adv., 2015, 5, 29563–29572 RSC.
- J. H. Kang, N. Ri and F. Kondo, Lett. Appl. Microbiol., 2004, 39, 178–180 CrossRef CAS PubMed.
- M. Masuda, Y. Yamasaki, S. Ueno and A. Inoue, Extremophiles, 2007, 11, 355–362 CrossRef CAS PubMed.
- Y. Matsumura, C. Hosokawa, M. Sasaki-Mori, A. Akahira, K. Fukunaga, T. Ikeuchi, K.-I. Oshiman and T. Tsuchido, Biocontrol Sci., 2009, 14, 161–169 CrossRef CAS PubMed.
- K.-i. Oshiman, Y. Tsutsumi, T. Nishida and Y. Matsumura, Biodegradation, 2007, 18, 247–255 CrossRef CAS PubMed.
- J. Spivack, T. Leib and J. Lobos, J. Biol. Chem., 1994, 269, 7323–7329 CrossRef CAS PubMed.
- H. Yamanaka, K. Moriyoshi, T. Ohmoto, T. Ohe and K. Sakai, Appl. Biochem. Biotechnol., 2007, 136, 39–51 CrossRef CAS PubMed.
- APHA-AWWA-WPCF, Standard Methods for the Examination of Water and Wastewater, APHA American Public Health Association, 1981 Search PubMed.
- W. G. Weisburg, S. M. Barns, D. A. Pelletier and D. J. Lane, J. Bacteriol., 1991, 173, 697–703 CrossRef CAS PubMed.
- J. Sambrook and D. W. Russell, Molecular cloning: A laboratory manual (3-volume set), Cold Spring Harbor Laboratory Press, New York, 2001 Search PubMed.
- M. Safari, B. Yakhchali and V. Shariati.J, Sci. Rep., 2019, 9, 1–14 CrossRef CAS PubMed.
- S. Saiyood, A. Vangnai, P. Thiravetyan and D. Inthorn, J. Hazard. Mater., 2010, 178, 777–785 CrossRef CAS PubMed.
- M. Ike, M. Y. Chen, C. S. Jin and M. Fujita, Environmental Toxicology: An International Journal, 2002, 17, 457–461 CrossRef CAS PubMed.
- M. Sasaki, T. Tsuchido and Y. Matsumura, J. Appl. Microbiol., 2008, 105, 1158–1169 CrossRef CAS PubMed.
- M. Alexander, Biodegradation and Bioremediation, Gulf Professional Publishing, 1999 Search PubMed.
- S. Y. Yuan, S. H. Wei and B. V. Chang, Chemosphere, 2000, 41, 1463–1468 CrossRef CAS PubMed.
- R. Margesin and F. Schinner, Appl. Microbiol. Biotechnol., 2001, 56, 650–663 CrossRef CAS PubMed.
- N. Christensen, D. J. Batstone, Z. He, I. Angelidaki and J. E. Schmidt, Water Sci. Technol., 2004, 50, 237–244 CrossRef CAS PubMed.
- J. Wong, K. Lai, C. Wan, K. Ma and M. J. W. Fang, Water Air Soil Pollut., 2002, 139, 1–13 CrossRef CAS.
|
This journal is © The Royal Society of Chemistry 2023 |