DOI:
10.1039/D2RA06189A
(Paper)
RSC Adv., 2023,
13, 4019-4031
N-Formamide as a carbonyl precursor in the catalytic synthesis of Passerini adducts under aqua and mechanochemical conditions†
Received
1st October 2022
, Accepted 23rd January 2023
First published on 27th January 2023
Abstract
A new simple, efficient, and environmentally friendly protocol is presented for the catalytic synthesis of α-acyloxycarboxamides using N-formamides as a carbonyl precursor under aqua and mechanochemical conditions. Immobilized sulfuric acid on silica gel was employed for the synthesis of desired products, via the reaction of benzoic acid, 1-napthylisocyanide and various heterocyclic N-formamides. After a careful optimization of the reaction conditions, the desired Passerini products were obtained in high to excellent yields in short reaction times (10–30 min) at room temperature. The highly efficient and environmentally friendly method provides a facile access to a library of α-acyloxycarboxamides derivatives for future research on bioactivity screening.
Introduction
Multicomponent condensations have been shown to be efficient in producing large collections of molecules.1 The ability to produce three or more points of variability in a single step opens the possibility of a large range of diversity in the products generated. As a starting point for the development of new drugs, the pharmaceutical industry has employed certain methods to obtain enormous sets of compounds.2 The capacity to anticipate which structural elements would be included in such collections has grown in usefulness. Therefore, diversity-oriented synthesis is valuable for probing large areas of chemical structure space in the search for new bioactive small molecules that may be undetected by traditional natural product screening tests.3 These experiences led to the development of pre-synthetic designs for numerous modern libraries, which results in the formation of products within each library that satisfy the criteria that are most frequently shared by well-known medicines.4,5 The Passerini reaction is useful in this regard because it has been explicitly applied to the synthesis of potentially bioactive molecules, most notably protease inhibitors, in terms of allowing for the fast assembly of many compounds.6,7 In synthetic chemistry, the effective and selective synthesis of complex heterocycles remains a challenge.8 Synthetic techniques like multicomponent reactions (MCRs), which efficiently and swiftly synthesize complicated multifunctional binding sites, have grown in popularity since they need fewer steps and low costs.7,9 Isocyanides (or isonitriles) exhibit unusual reactivity and can react with both nucleophiles and electrophiles at the same time to generate reactive α-adducts. As a result, the isocyanide-based multicomponent reactions (I-MCRs)10–12 have been demonstrated to be efficient techniques for the one-pot, high-throughput synthesis of diverse libraries of potentially bioactive and densely functionalized molecules. The Passerini three-component reaction (P-3CR)13 was developed after Passerini's discovery of the reaction between isocyanides, aldehydes, and carboxylic acids to produce α-acyloxy carboxamides in 1921.14,15 It has since grown to be a potent tool in combinatorial chemistry and hetero cyclic chemistry for drug discovery as well as natural product synthesis.16–18
Passerini reaction has been known for over 80 years, little is known about the scope of carbonyl-type electrophiles that undergo the reaction (Scheme 1). The best candidates for this reaction are aldehydes and (to a lesser extent) ketones.19,20 On the other hand, the impact of including neighbouring reactive groups within the carbonyl component has not been investigated.
 |
| Scheme 1 Passerini 3-component reaction utilizing carboxylic acid, aldehyde, and isocyanide. | |
Additionally, the application of alternative carbonyls such esters and formamides has not been studied. We set out to replace the carbonyl component, keeping in mind the potential effects of even minor functional modifications, by simply substituting N-formamides for the carbonyl in the Passerini reaction (Scheme 2).
 |
| Scheme 2 Aqua/mechanochemical mediated Passerini reaction utilizing various heterocyclic N-formamides as a replacement for the carbonyl component. | |
Recent studies have shown that Passerini chemistry and other multicomponent reactions can be accelerated in either water alone or in combination with an organic solvent.21 One theory put out to explain this phenomenon is that the water employed in this system has a high cohesive energy density, which acts as a driving force analogous to pressure. Another is that the presence of a hydroxyl group provides a second reaction pathway through which the products can be formed, providing several pathways for the reaction, and enabling it to progress more quickly than in the pathway provided when a hydroxyl group is absent.22–24
On the other hand, mechanochemical methods have been used in synthesis for a long time, but their perception in the synthetic world has altered recently, and they are on their way to becoming mainstream.25–27 However, the combination of mechanochemical synthesis with techniques meant to improve synthetic efficiency by enabling the generation of multiple bonds in a single operation is a relatively new approach to sustainable chemistry that has already shown to be very promising.28–30 The two basic methods for promoting mechanochemistry are mechanical milling and hand grinding. Most of the mechanical milling is done in a mixer/shaker mill or a planetary mill at a frequency of 5–60 Hz, while hand milling, also known as manual milling, is often done in a mortar and pestle. The energy output of mechanical milling is substantially larger, and it is also more dependable and sophisticated than manual grinding, which can yield a range of experimental outcomes depending on the speed and force of the grinding.30 Various investigations have shown that mechanochemical activation and methods have several advantages, such as shorter reaction milling times, greater yield, reduced waste formation, improved selectivity, and stoichiometry control, to name a few.31,32
Aqua and mechanochemistry are widely used for quick organic synthesis because they take less time, produce better results, and are simpler to utilize than traditional techniques. A crucial tool in the construction of diversity-oriented compound libraries is the Passerini three-component reaction, which uses aldehyde, substituted carboxylic acid, and different functionalized isocyanide.33 Various libraries of compounds can be constructed by changing the aldehyde component of these reactions to N-formamides. In this regard, a mechanochemical and aqua versions of this transformation have been developed, and they are based on the use of water (aqueous reaction) and high-speed vibration milling with a double agate ball (6 mm diameter) in an agate jar and water as a liquid-assisted grinding agent to produce the carboxamide derivatives.
An heterogenous catalytic approach can be anticipated to be advantageous as an alternative to the commonly used metallocatalysis or organocatalysis due to the high efficiency, short reaction time, high yield, and mild reaction conditions.34 Brønsted acid catalysts have been used in several organic transformations to overcome limitations, such as toxicity, volatility, and hazardous nature of the conventional methods.35 Catalysts are adsorbed on silica gel to provide the benefits and advantages of ready availability, simple work-up procedure, long catalytic life, environment safe, excellent yields, and recyclability.36,37 The aim of the present work was to combine the activity of catalysts with the diversity offered by a Passerini reaction to obtain functionally distinct α-acyloxycarboxamide scaffolds with the feature of broad substrate scope and sufficient molecular diversity needed for fine tuning biological activity (using various substituted heterocyclic N-formamides as carbonyl surrogate).
Results and discussion
The Passerini reaction of benzoic acid (1 mmol), 1-naphthylisocyanide (1 mmol) and 4-formamido pyridine (1 mmol) as the carbonyl surrogate was chosen as model reaction using dichloromethane as solvent (Scheme 3). Interestingly, a new product 4a was observed and isolated in 69% yield (Table 1 entry 4). This revealed the successful replacement of carbonyl by 4-formamido pyridine 3a (N-formamides) in the Passerini reaction. We investigated the impact of various solvents on the reaction course first in the absence of catalyst, to determine the most suitable reaction conditions and to compare the aqua conditions' results with those obtained under standard conditions (organic solvents) (Table 1).
 |
| Scheme 3 Optimization of the model Passerini reaction in water in the presence of catalyst. The reaction was performed with carboxylic acids (1a, 1 mmol), 1-naphthylisocyanide (2a, 1 mmol), and various heterocyclic N-formamides (3a–p, 3s 1 mmol) under aqueous conditions (a) using DCM as solvent (b) in the presence of water as solvent (c) in the presence of H2SO4–SiO2 in aqueous media at room temperature. | |
Table 1 Effect of solvents on the scope of Passerini reaction in the presence/absence of catalyst
Entry |
Solvent |
Catalyst (g) |
Time |
Yield (%) |
1 |
Tetrahydrofuran |
No |
48 h |
14 |
2 |
Toluene |
No |
24 h |
23 |
3 |
Diethyl ether |
No |
48 h |
30 |
4 |
Dichloromethane |
No |
48 h |
69 |
5 |
Methanol |
No |
48 h |
47 |
6 |
Water |
No |
15 min |
82 |
7 |
Tetrahydrofuran |
SiO2–H2SO4 (0.02) |
24 h |
33 |
8 |
Toluene |
SiO2–H2SO4 (0.02) |
6 h |
51 |
9 |
Diethyl ether |
SiO2–H2SO4 (0.02) |
24 h |
44 |
10 |
Dichloromethane |
SiO2–H2SO4 (0.02) |
6 h |
78 |
11 |
Methanol |
SiO2–H2SO4 (0.02) |
6 h |
63 |
12 |
Water |
SiO2–H2SO4 (0.02) |
10 min |
95 |
The P-3CR in the absence of H2SO4–SiO2 proceeded smoothly in dichloromethane (CH2Cl2) and methanol (CH3OH) with higher yields of 69% and 47% respectively (Table 1 entries 4–5). Solvents such as tetrahydrofuran, toluene, and diethyl ether were ineffective even after 48 hours producing trace amount of the products (Table 1 entry 1–3). When the reaction was carried out in water in the absence of catalyst, the product 4a was obtained in high yield (82%) within 15 min (Table 1 entry 6).
The reaction products distribution showed strong dependency on the hydrophobicity of the reactants, according to Pirrung, Das Sarma,24 while the more hydrophobic ones producing the typical Passerini product in high yield and at faster rate. The model reaction was performed in the presence of 0.02 g of H2SO4–SiO2 in DCM at room temperature and 78% yield product was observed after 6 h (Table 1, entry 10). Also, when the reaction was performed in the presence of 0.02 g of H2SO4–SiO2 in methanol at room temperature, the yield increases slightly to 55% after 6 h (Table 1, entry 11). The highest yield (95%) was obtained when the reaction was carried out in water in the presence of 0.02 g of the H2SO4–SiO2 at room temperature (Table 1, entry 12). Therefore, as indicated from the results, the heterogenous catalyst H2SO4–SiO2 has higher efficiency and activity, consequently, reactions are carried out on a wider surface producing high yields of products in a very short time. The next survey of catalysts revealed that further increase in the catalyst amount has little or no effect on the yield of the reaction product (4a) as 0.02 g was found sufficient to carry out the reaction with highest possible yields. Thus, the best reaction efficiency was achieved in the presence of 0.02 g H2SO4–SiO2 in water (2 mL) at room temperature (Table 1, entry 12).
To study the effectiveness and generality of the catalyst, new functionalized α-acyloxyamide derivatives were synthesized using benzoic acid 1a, 1-naphthylisocyanide 2a and various heterocyclic N-formamides 3a–p, as shown in Table 3. In comparison to conventional Passerini reaction which leverage on volatile organic solvent, the synthesized compounds exhibited good yields and considerably faster reaction times, which confirms the catalyst's effectiveness. The results are presented in Table 3. With the optimal condition in hand (Table 1, entry 12), sixteen (16) N-formamides derivatives 3a–p, three (3) isocyanides 2a, 2q, 2r and a benzoic acid (1a) were used to construct a series of analogues 4a–s. All desired products were isolated by column chromatography, and the yields are ranging from 83–96% (Table 3).
In the course of our investigations, we found that a variety of functionalized N-formamides containing heteroatoms could be used as beneficial inputs in Passerini MCRs to provide adducts in high to excellent yields. For example, the heterocyclic N-formamides of anthraquinone 3o, antipyrine 3e, isoxazole 3i, ephedrine 3j, thiazole 3s, benzothiazole 3h, benzimidazole 3g, furfural 3p, and 1,2,3-triazole 3c-d moieties were each found to participate in Passerini MCRs that proceeded readily in one-pot operations in the presence of immobilized sulfuric acid on silica gel. Generally, the reactions which were carried out with 1-Napthylisocyanide (2a) resulted in products (4a–4p, 4s) with yields ranging from 83–96%. In the case of ethyl 2-isocyanobenzoate (2q) the yields (4q) obtained were about 93%. Similarly, the reactions involving 1-isocyano-3-bromobenzene (2r) performed under the same conditions, furnished products (4r) in good yields 91%. To our knowledge, there are no reports in the literature employing this set of heterocyclic N-formamides in Passerini MCRs; thus, we present some novel functionalized Passerini adducts.
Mechanochemical synthesis
The physical state of the reactants is an important factor to consider in this experiment. For the first experiment (Exp A), both reagents are liquids, a grinding auxiliary was necessary to facilitate mixing and energy transfer. The reagents for the second experiment (Exp B), however, are solids, and the addition of a grinding agent could have a diluting impact. Initially the mechanochemical synthesis of various functionalized α-acylcarboxamide was investigated, we decided to employ fewer variables in this study by using the same jar and ball materials as in our earlier studies while also maintaining the same ball size, ball number, and jar size. Thus, the experiments (Exp-A/B) relied on the use of a stainless-steel reactor (diameter: 2.0 cm; height: 2.0 cm; volume of reactor: 13.2 mL) that contained two balls of the same material (diameter: 6 mm; mass: 0.90 g).
Experiment A. The model reaction first selected was the reaction between benzoic acid (1 mmol), 1-naphthylisocyanide (1 mmol), and 4-formamido pyridine (1 mmol). The three substrates were subjected to milling in the first series of experiments without using an auxiliary material, the yield of Passerini adduct 4a obtained was dismal after 30 minutes at 25 Hz (Table 4, entry 1). Applying liquid substrates 2a, 3a, under these circumstances was disappointing because the yields of the resulting 4a were much lower than those seen in the previously reported aqua mediated synthesis method (Scheme 3, versus Scheme 4). A highly watery slurry was visible when the ball mill jar for these reactions were opened after the milling, leading one to conclude that insufficient mass transfer had occurred. This limitation was removed by employing neutral alumina as a grinding auxiliary in the substrate combination. As a result, a dry, powdery crude product was produced, which supported our prediction because all products were now produced with noticeably better yields. Interestingly, introducing silica gel to the reaction mixture gave the desired Passerini product in 86% yield after milling for 30 minutes (Table 4, entry 2). There may be numerous functions for the inclusion of a grinding auxiliary. Specifically, in mechanochemical processes where the reaction mixture might be defined as a gum, paste, or liquid, we believe that the main advantages pertain to enhanced mixing and enabling energy transfer. Notably, the same reaction carried out in aqueous media produced almost the same yield in the absence of catalyst (Table 1, entry 6).
 |
| Scheme 4 Optimization of the model reaction conditions for (exp A). The reaction was performed with carboxylic acids (1a, 1 mmol), 1-naphthylisocyanide (2a, 1 mmol), and various heterocyclic N-formamides (3a–p, 3s 1 mmol) under mechanochemical conditions (a) without any auxiliary (b) in the presence SiO2 (c) in the presence of H2SO4–SiO2 as solid auxiliary at room temperature. | |
To further increase the yield of 4a, we resorted to the employment of the immobilized sulfuric acid on silica gel (H2SO4–SiO2) as a griding auxiliary instead of silica gel, since it has been established in our previous studies the positive impact of the catalyst in Passerini reaction.38 We started by subjecting the model substrates in to milling in the presence of 0.01 g of catalyst for 20 minutes. The yield of 4a decline rapidly to 55%, increase in the reaction time to 30 min had a negligible effect (Table 4, entries 4–6). Then, the catalyst amount was varied. At this point the yield rose as the amount of catalyst utilized increased to 0.03 g producing the Passerini product in 97% yield in milling time of 20 min (Table 4, entries 7–12). However, it should be noted that the result was superior to those obtained under aqueous media in the presence of catalyst (Table 4, entry 11, versus Table 2, entry 2). With the optimized conditions, we evaluated the scope of the liquid substrates via Passerini reaction in the ball mill. Starting with pyridine moieties (3a, 3b) as carbonyl surrogate, 1-naphthylisocyanides and benzoic acid. The reaction proceeded smoothly producing the corresponding Passerini adducts in excellent yields (90–97%) after milling for 20 min under catalytic conditions. Similarly, liquid heterocyclic N-formamides containing ephedrine (3j), and furan (3p) moieties also proved to be suitable for the multicomponent reaction in the ball mill, affording the products (4j, 4p) preferentially (Fig. 1). Finally, a set of experiments using 1-isocyano-3-bromo benzene (2r) and ethyl 2-isocyanobenzoate (2q) as isocyanide component with benzoic acid (1a) and 4-formamido pyridine (3a)) were also successful, highlighting the wide range of applicability for the mechanochemical method.
Table 2 Screening for best catalytic reaction conditions
Entry |
Reaction condition |
Solvent |
Catalyst (g) |
Time |
Yield (%) |
1 |
(1a, 2a, 3a) |
Distilled water |
SiO2–H2SO4 (0.01) |
10 min |
93 |
2 |
(1a, 2a, 3a) |
Distilled water |
SiO2–H2SO4 (0.02) |
10 min |
95 |
3 |
(1a, 2a, 3a) |
Distilled water |
SiO2–H2SO4 (0.03) |
10 min |
95 |
4 |
(1a, 2a, 3a) |
Dichloromethane |
SiO2–H2SO4 (0.01) |
6 h |
72 |
5 |
(1a, 2a, 3a) |
Dichloromethane |
SiO2–H2SO4 (0.02) |
6 h |
78 |
6 |
(1a, 2a, 3a) |
Dichloromethane |
SiO2–H2SO4 (0.03) |
6 h |
78 |
Table 3 New synthesized Passerini adducts under catalytic conditions
Entry |
N-Formamides (3a–s) |
Isocyanides |
Acid |
Product |
1 |
 |
 |
R–COOH |
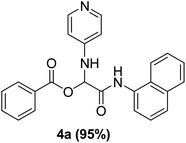 |
2 |
 |
 |
R–COOH |
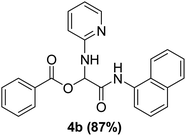 |
3 |
 |
 |
R–COOH |
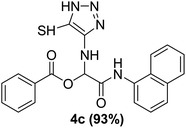 |
4 |
 |
 |
R–COOH |
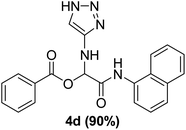 |
5 |
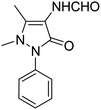 |
 |
R–COOH |
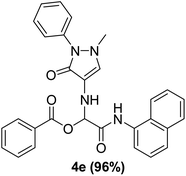 |
6 |
 |
 |
R–COOH |
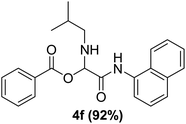 |
7 |
 |
 |
R–COOH |
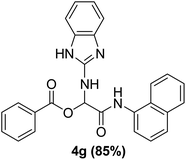 |
8 |
 |
 |
R–COOH |
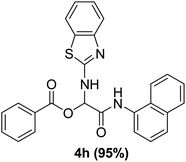 |
9 |
 |
 |
R–COOH |
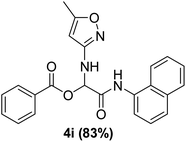 |
10 |
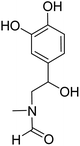 |
 |
R–COOH |
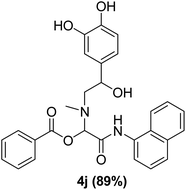 |
11 |
 |
 |
R–COOH |
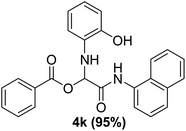 |
12 |
 |
 |
R–COOH |
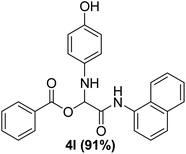 |
13 |
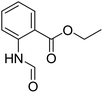 |
 |
R–COOH |
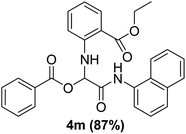 |
14 |
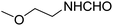 |
 |
R–COOH |
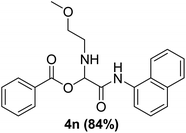 |
15 |
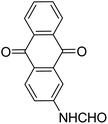 |
 |
R–COOH |
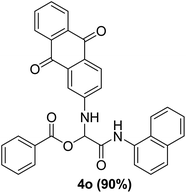 |
16 |
 |
 |
R–COOH |
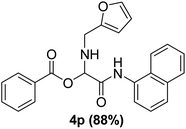 |
17 |
 |
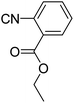 |
R–COOH |
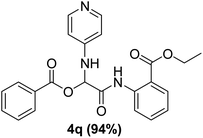 |
18 |
 |
 |
R–COOH |
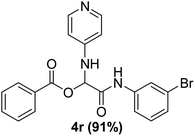 |
19 |
 |
 |
R–COOH |
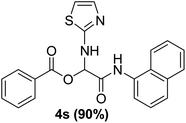 |
Table 4 Optimization of the model reaction conditions for (Exp A)
Entry |
React. |
Catalyst (g) |
Time |
Yield (%) |
1 |
(1a, 2a, 3a) |
No catalyst |
30 |
15 |
2 |
(1a, 2a, 3a) |
SiO2 (0.03) |
30 |
86 |
3 |
(1a, 2a, 3a) |
SiO2 (0.03) |
40 |
86 |
4 |
(1a, 2a, 3a) |
SiO2–H2SO4 (0.01) |
10 |
41 |
5 |
(1a, 2a, 3a) |
SiO2–H2SO4 (0.01) |
20 |
55 |
6 |
(1a, 2a, 3a) |
SiO2–H2SO4 (0.01) |
30 |
55 |
7 |
(1a, 2a, 3a) |
SiO2–H2SO4 (0.02) |
10 |
74 |
8 |
(1a, 2a, 3a) |
SiO2–H2SO4 (0.02) |
20 |
77 |
9 |
(1a, 2a, 3a) |
SiO2–H2SO4 (0.02) |
30 |
77 |
10 |
(1a, 2a, 3a) |
SiO2–H2SO4 (0.03) |
10 |
95 |
11 |
(1a, 2a, 3a) |
SiO2–H2SO4 (0.03) |
20 |
97 |
12 |
(1a, 2a, 3a) |
SiO2–H2SO4 (0.03) |
30 |
97 |
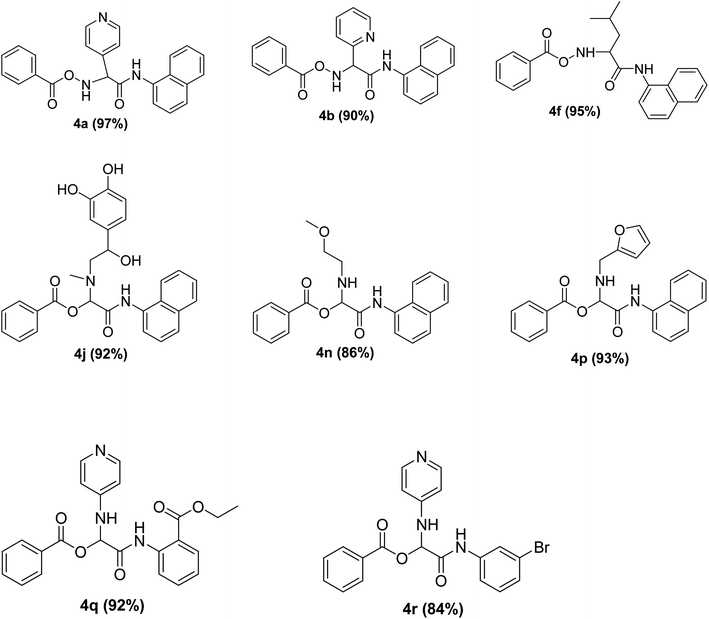 |
| Fig. 1 Variation of heterocyclic N-formamide moieties under mechanochemical activation. | |
Experiment B. For the initial test reactions, benzoic acid 1a, 1-naphthylisocyanide 2a and 2-formamidoanthracene-9,10-dione (3o) were chosen as representative substrates. In the second experiment, both compounds were milled at 25 Hz for 20 min, and to our delight, product formation occurred providing the Passerini adducts 4o in a yield of 71% (Table 5, entry 1). Increasing the milling time from 20 to 30 min improved the yield of 4o to 87% and no further increase was observed at 40 min (Table 5, entries 2-4). However, 30 minutes of milling at 25 Hz produced 4o with an 87% optimum yield, but a shorter reaction time (10 min) had a detrimental impact on the yield of 4o (79%) (Table 5, entries 1-4) respectively. At this point, the newly developed mechanochemical method for 4o produced outcomes similar to those of the previously reported aqua-mediated procedure (Table 1, entry 12), nonetheless, no solvent was employed this time yet. The idea of performing these reactions under liquid-assisted milling conditions was then entertained. We were thrilled to discover that when 75 μl water was employed as liquid auxiliary at room temperature after 30 minutes of milling, the yield of 4o increases further to 92% of the Passerini adducts (Table 5, entry 6). When 100 μl and 150 μl of water was used as an additive for the LAG, the yield decreased significantly to 72 and 66% respectively (Table 5, entries 7–8). The latter observation may be caused by the more liquid changing the reaction mixture's texture and so lessening effective mixing. In our previously reported Passerini reactions in aqueous media, the presence of immobilized sulfuric acid on silica gel had proven advantageous.37 We anticipate that using liquid acid in replacement of water (LAG) may have a beneficial effect on the reaction yield, so such effects on the formation of 4o were therefore also investigated here under mechanochemical conditions. In each instance, 10 mol% of the additive were used. The yield of 4o decreased to 69% in the presence of aqueous HCl (Table 5, entry 9). Additionally, H2SO4 and HNO3 had detrimental impacts, lowering the yield of 4o to 74 and 81%, respectively (Table 5, entries 10-11). It was discovered that adding 75 μl of Acetic acid slightly boosted the yield to 88%. Compound 4o was now successfully produced with a yield of 88% with acetic acid (weak) compared to earlier trial with acids (strong) (Table 5, entry 12). An alternative justification is that at higher acid loading, the ‘on–off’ activation of the formamide to nucleophilic attack is slow, meaning that the nucleophilicity is greatly retarded compared to lower acid loadings. Although good yield was obtained with weak acid (acetic acid), however the yield (88%) (Table 5, entry 12) is not comparable to that obtained using water (92%) (Table 5, entry 6) under the same milling conditions. Consequently, water was chosen as an additive of choice in this case and is explored further for subsequent research. Accordingly, the optimal mechanochemical conditions for the formation of 4o involved a milling of 1a, 2a, and 3o in the presence of water (75 μl) at 25 Hz for 30 min at room temperature. At the end of the reaction, the crude mixture was subjected to column chromatography to furnished 4o in 92% yield (Scheme 5).
Table 5 Optimization of the model reaction conditions for (Exp B)
Entry |
Reaction condition |
Additive |
Time min |
Yield (%) |
1 |
(1a, 2a, 3o) |
No additive |
10 |
79 |
2 |
(1a, 2a, 3o) |
No additive |
20 |
84 |
3 |
(1a, 2a, 3o) |
No additive |
30 |
87 |
4 |
(1a, 2a, 3o) |
No additive |
40 |
87 |
5 |
(1a, 2a, 3o) |
Water (50 μl) |
30 |
89 |
6 |
(1a, 2a, 3o) |
Water (75 μl) |
30 |
92 |
7 |
(1a, 2a, 3o) |
Water (100 μl) |
30 |
72 |
8 |
(1a, 2a, 3o) |
Water (150 μl) |
30 |
66 |
9 |
(1a, 2a, 3o) |
Aqueous HCl (75 μl) |
30 |
69 |
10 |
(1a, 2a, 3o) |
Aqueous H2SO4 (75 μl) |
10 |
74 |
11 |
(1a, 2a, 3o) |
Aqueous HNO3 (75 μl) |
20 |
81 |
12 |
(1a, 2a, 3o) |
Acetic acid (75 μl) |
30 |
88 |
 |
| Scheme 5 Model Passerini reaction with solid substrate and water as grinding auxiliary. The reaction was performed with carboxylic acids (1a, 1 mmol), 1-naphthylisocyanide (2a, 1 mmol), and various heterocyclic N-formamides (3c–e, 3g-h, 3i, 3k–o, 3s 1 mmol) under mechanochemical conditions (a) without any auxiliary (b) in the presence water at room temperature. | |
With the optimized conditions at hand, we explored the scope of the method by using different substituted solid N-formamides such as those that contain the electron-donating groups 2-formamido phenol (3k), and 4-formamido phenol (3l). The corresponding Passerini adducts 4k–l were produced in good to excellent yields (78–86%) (Fig. 2). Heterocyclic N-formamides with five membered ring such as 1,2,3-triazole-5-thiol (3c), 1,2,3-triazole (3d), isoxazole (3i), and thiazole (3s) moieties were also studied and found to furnish products 4c, 4d, 4i, and 4s in good to excellent yields (82–95%). Additionally, heterocyclic aromatic N-formamides such as Antipyrine (3e), benzimidazole (3g) and benzothiazole (3h), generated the anticipated products 4e, 4g, and 4h in good to excellent yields (80–88%) after purification by column chromatography.
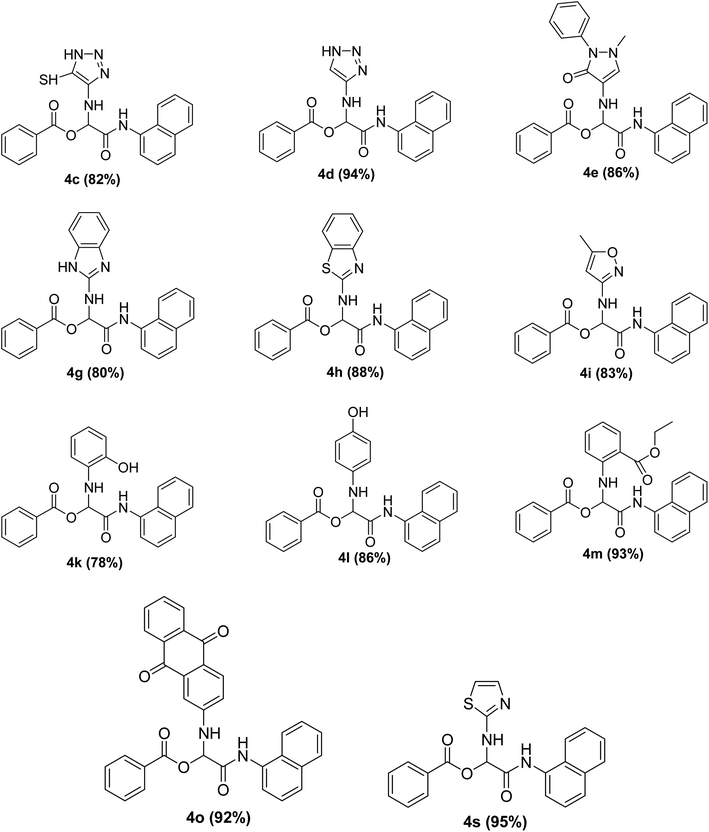 |
| Fig. 2 Variation of heterocyclic N-formamide moieties under mechanochemical activation. | |
For the synthesis of novel α-acyloxycarboxamide derivatives in the presence of SiO2–H2SO4, the proposed reaction mechanism is depicted in Scheme 6. The N-formamide's group is first activated by the catalyst (SiO2–H2SO4) (4). The activated N-formamide (5) group is then nucleophilically attacked by the isocyanide, resulting in the formation of an intermediate called nitrilium (6). After the intermediate (6) is attacked by the carboxylate of (7), an acyl transfer and Mumm rearrangement occur to produce derivatives of acyloxycarboxamides (9).
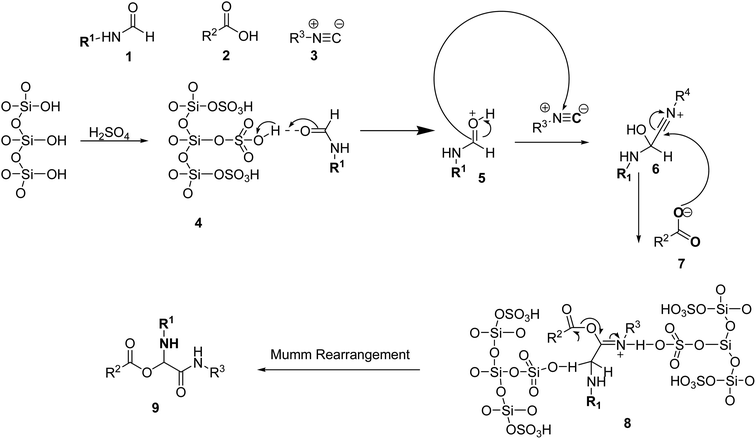 |
| Scheme 6 Proposed mechanism for the formation of α-acyloxycarboxamides in the presence of the immobilized sulfuric acid on silica gel (SiO2–H2SO4). | |
Conclusions
A practical synthesis of Passerini 3-CR adducts (4a–s, 79–98% yield) under aqua and mechanochemical (ball-milling) conditions were developed to demonstrate the usefulness of aqueous organic reaction and mechanochemical activation for isocyanide-based multicomponent reactions. These protocols allow for the preparation of valuable molecules in good to excellent yields under catalytic conditions. Furthermore, these MCRs have high atom economy, experimental simplicity, short reaction times, and low energy costs. Additionally, our studies have opened the possible construction of diversity-oriented compound libraries and biologically relevant or natural-product-like molecular frameworks via Passerini reaction from various heterocyclic N-formamides, benzoic acid and functionalized isocyanide. Finally, the Passerini processes could be scaled up to 100 mmol. We believe that these findings will undoubtedly offer value to the growing area of mechanochemistry and aqueous organic reaction.
Materials and methods
Mechanochemical experiments were carried out in an in-house modified Makita (Jigsaw) ball mill that was equipped with (reactor diameter: 2.0 cm; height: 2.0 cm; volume of reactor: 13.2 mL). All reactors are stainless steel and equipped with stainless steel balls (diameter: 6 mm; mass: 0.90 g). A FT-IR-Eco ATR Bruker Alpha II Spectrometer was used for the FT-IR analysis. The IR spectra were obtained by the attenuated total reflection (ATR) method. For each experiment, 16 scans were performed in the frequency range from 650 to 4000 cm−1. Melting points of all the compounds were determined using a Koffler hot-stage apparatus and are uncorrected. NMR spectra were recorded on a Bruker Advance III 400 spectrometer using CDCl3 or DMSO-d6 as a solvent with tetramethyl silane used as internal standard. LC-MS/MS data was recorded on a Bruker Compact quadrupole time of flight (QToF) mass spectrometer. Raw mass spectrometry data were processed using MZmine software (version 2.38). Solvents and chemicals used were of analytical grade, which were purchased from Sigma Aldrich and used without further purification. The purity determination of the starting materials and reaction monitoring was performed by thin-layer chromatography (TLC) on Merck silica gel G F254 plates.
Preparation of sulfuric acid adsorbed on silica gel (SiO2–H2SO4)
This has been reported previously.38 In short, to a suspension of silica gel (29.5 g, 230–400 mesh size) in EtOAc (60 mL), H2SO4 (1.5 g, 15.5 mmol, 0.8 mL of a 98% aq. solution of H2SO4) was added and the mixture was stirred magnetically for 30 min at room temperature. EtOAc was removed under reduced pressure (rotary evaporator) and the residue was heated at 100 °C for 72 h under vacuum to afford SiO2–H2SO4 as a free-flowing powder.
General experimental procedures for the aqua synthesis of Passerini adducts (4a–s) in the presence of catalyst
A mixture of benzoic acid (1a, 1 mmol) 1-naphthylisocyanides (2a, 1 mmol), and 4-formamido pyridine (3a, 1 mmol) was vigorously stirred in 2 mL water at room temperature for 10 minutes in the presence of 0.02 g of SiO2–H2SO4. Upon completion, the organic layer was separated, and the combined organic phases were concentrated under reduced pressure and the residue was purify by column chromatography using DCM/Hexane (3
:
1) as eluent to afford the desired products. Typical yields range from 83 to 96%. All other products (a–s) were obtained by similar approach.
General procedure for the mechanochemical synthesis of Passerini adducts (4a-b, 4f, 4j, 4n, 4p)
Experiment A. A mixture of benzoic acid (1a, 1 mmol) 1-naphthylisocyanides (2a, 1 mmol), and 4-formamido pyridine (3a, 1 mmol) and additive (SiO2–H2SO4 0.03 g) was milled in a 13.2 mL stainless steel milling vessel contained two balls of the same material (diameter: 6 mm; mass: 0.90 g) at 25 Hz for 20 min. After the milling was complete, the content in the milling vessel was transferred into a beaker using a small amount of organic solvent (DCM). Then, purification was done by column chromatography to afford the corresponding Passerini adducts in high to excellent yield.
General procedure for the mechanochemical synthesis of Passerini adducts (4c–e, 4g–i, 4k–m, 4o, 4s)
Experiment B. A mixture of benzoic acid (1a, 1 mmol) 1-naphthylisocyanides (2a, 1 mmol), and 2-formamidoanthracene-9,10-dione (3o, 1 mmol) and additive (water 75 μL) was milled in a 13.2 mL stainless steel milling vessel contained two balls of the same material (diameter: 6 mm; mass: 0.90 g) at 25 Hz for 30 min. After the milling was complete, the content in the milling vessel was transferred into a beaker using a small amount of organic solvent (DCM). Then, purification was done by column chromatography to afford the corresponding oxindole derivatives in high yield.
Conflicts of interest
There are no conflicts to declare.
References
- H. Bienaymé, Reagent explosion’: An efficient method to increase library size and diversity, Tetrahedron Lett., 1998, 39, 4255–4258 CrossRef.
- A. R. Extance, D. W. M. Benzies and J. J. Morrish, Production of small libraries optimising and examining the scope of aqueous acceleration of the Passerini reaction, QSAR Comb. Sci., 2006, 25, 484–490 CrossRef CAS.
- A. L. Chandgude and A. Dömling, An efficient Passerini tetrazole reaction (PT-3CR), Green Chem., 2016, 18, 3718–3721 RSC.
- B. B. Touré and D. G. Hall, Natural product synthesis using multicomponent reaction strategies, Chem. Rev., 2009, 109, 4439–4486, DOI:10.1021/cr800296p.
- M. A. Fouad, H. Abdel-Hamid and M. S. Ayoup, Two decades of recent advances of Ugi reactions: Synthetic and pharmaceutical applications, RSC Adv., 2020, 10, 42644–42681, 10.1039/d0ra07501a.
- B. Ganem, Strategies for Innovation in Multicomponent Reaction Design, Acc. Chem. Res., 2009, 42, 463–472, DOI:10.1021/ar800214s.
- R. C. Cioc, E. Ruijter and R. V. A. Orru, Multicomponent reactions: Advanced tools for sustainable organic synthesis, Green Chem., 2014, 16, 2958–2975, 10.1039/c4gc00013g.
- O. BZhang and A. Studer, Recent advances in the synthesis of nitrogen heterocycles via radical cascade reactions using isonitriles as radical acceptors, Chem. Soc. Rev., 2015, 44, 3505–3521, 10.1039/c5cs00083a.
- N. P. Tripolitsiotis, M. Thomaidi and C. G. Neochoritis, The Ugi Three-Component Reaction; a Valuable Tool in Modern Organic Synthesis, Eur. J. Org. Chem., 2020, 2020, 6525–6554, DOI:10.1002/ejoc.202001157.
- K. A. Waibel, R. Nickisch, N. Möhl, R. Seim and M. A. R. Meier, A more sustainable and highly practicable synthesis of aliphatic isocyanides, Green Chem., 2020, 22, 933–941, 10.1039/c9gc04070f.
- P. Patil, M. Ahmadian-Moghaddam and A. Dömling, Isocyanide 2.0, Green Chem., 2020, 22, 6902–6911, 10.1039/d0gc02722g.
- I. Ugi, B. Werner and A. Dömling, The chemistry of isocyanides, their multicomponent reactions and their libraries, Molecules, 2003, 8, 53–66, DOI:10.3390/80100053.
- Y. Shen, B. Huang, L. Zeng and S. Cui, Single Reactant Replacement Approach of Passerini Reaction: One-Pot Synthesis of β-Acyloxyamides and Phthalides, Org. Lett., 2017, 19, 4616–4619, DOI:10.1021/acs.orglett.7b02232.
- J. Brioche, G. Masson and J. Zhu, Passerini three-component reaction of alcohols under catalytic aerobic oxidative conditions, Org. Lett., 2010, 12, 1432–1435, DOI:10.1021/ol100012y.
- D. Wu, J. Li and W. Wang, Selective Formation of Monoacylated Diols through a Mild Passerini Reaction, Eur. J. Org. Chem., 2018, 2018, 3022–3030, DOI:10.1002/ejoc.201800499.
- M. Ingold, G. V. López and W. Porcal, Green conditions for passerini three-component synthesis of tocopherol analogues, ACS Sustainable Chem. Eng., 2014, 2, 1093–1097, DOI:10.1021/sc5002116.
- T. Kaicharla, S. R. Yetra, T. Roy and A. T. Biju, Engaging isatins in solvent-free, sterically congested Passerini reaction, Green Chem., 2013, 15, 1608–1614, 10.1039/c3gc40454d.
- B. Kumar, J. Maity, B. Shankar, S. Kumar, Kavita and A. K. Prasad, Synthesis of D-glycopyranosyl depsipeptides using Passerini reaction, Carbohydr. Res., 2021, 500, 108236, DOI:10.1016/j.carres.2021.108236.
- S. T. M. Simila and S. F. Martin, Applications of the Ugi reaction with ketones, Tetrahedron Lett., 2008, 49, 4501–4504, DOI:10.1016/j.tetlet.2008.05.073.
- S. A. Salami, M. Manyeruke, X. Siwe-Noundou and R. W. M. Krause, Immobilized Sulfuric Acid on Silica Gel as Highly Efficient and Heterogeneous Catalyst for the One-Pot Synthesis of Novel α-Acyloxycarboxamides in Aqueous Media, Int. J. Mol. Sci., 2022, 23(17), 9529 CrossRef CAS PubMed.
- M. Cortes-Clerget, J. Yu, J. R. A. Kincaid, P. Walde, F. Gallou and B. H. Lipshutz, Water as the reaction medium in organic chemistry: From our worst enemy to our best friend, Chem. Sci., 2021, 12, 4237–4266, 10.1039/d0sc06000c.
- D. Paprocki, M. Wilk, A. Madej, P. Walde and R. Ostaszewski, Catalyst-free synthesis of α-acyloxycarboxamides in aqueous media, Environ. Chem. Lett., 2019, 17, 1011–1016, DOI:10.1007/s10311-018-0797-5.
- M. Mamaghani, F. Shirini, N. O. Mahmoodi, A. Azimi-Roshan and A. Monfared, ‘on water’ organic synthesis: Three-component one-pot synthesis of novel bis(1-(cyclohexylamino)-1-oxoalkyl or aryl) fumarates, J. Iran. Chem. Soc., 2014, 11, 659–664, DOI:10.1007/s13738-013-0337-y.
- M. C. Pirrung, K. Das Sarma and J. Wang, Hydrophobicity and mixing effects on select heterogeneous, water-accelerated synthetic reactions, J. Org. Chem., 2008, 73, 8723–8730, DOI:10.1021/jo801134r.
- M. Leonardi, M. Villacampa and J. C. Menéndez, Multicomponent mechanochemical synthesis, Chem. Sci., 2018, 9, 2042–2064, 10.1039/c7sc05370c.
- T. Kleine, J. Buendia and C. Bolm, Mechanochemical degradation of lignin and wood by solvent-free grinding in a reactive medium, Green Chem., 2013, 15, 160–166, 10.1039/c2gc36456e.
- J. G. Hernández and T. Friščić, Metal-catalyzed organic reactions using mechanochemistry, Tetrahedron Lett., 2015, 56, 4253–4265, DOI:10.1016/j.tetlet.2015.03.135.
- V. Štrukil, D. Margetic, M. D. Igrc, M. Eckert-Maksic and T. Frišcic, Desymmetrisation of aromatic diamines and synthesis of non-symmetrical thiourea derivatives by click-mechanochemistry, Chem. Commun., 2012, 48, 9705–9707, 10.1039/c2cc34013e.
- T. Carstens, D. A. Haynes and V. J. Smith, Cocrystals: Solution, Mechanochemistry, and Sublimation, Cryst. Growth Des., 2020, 20, 1139–1149, DOI:10.1021/acs.cgd.9b01450.
- L. A. Polindara-García and E. Juaristi, Synthesis of Ugi 4-CR and Passerini 3-CR Adducts under Mechanochemical Activation, Eur. J. Org. Chem., 2016, 2016, 1095–1102, DOI:10.1002/ejoc.201501371.
- J. G. Hernández, M. Turberg, I. Schiffers and C. Bolm, Mechanochemical Strecker Reaction: Access to α-Aminonitriles and Tetrahydroisoquinolines under Ball-Milling Conditions, Chem. – A Eur. J., 2016, 22, 14513–14517, DOI:10.1002/chem.201603057.
- J. G. Hernández, M. Frings and C. Bolm, Mechanochemical Enzymatic Kinetic Resolution of Secondary Alcohols under Ball-Milling Conditions, ChemCatChem, 2016, 8, 1769–1772, DOI:10.1002/cctc.201600455.
- G. Koopmanschap, E. Ruijter and R. V. A. Orru, Isocyanide-based multicomponent reactions towards cyclic constrained peptidomimetics, Beilstein J. Org. Chem., 2014, 10, 544–598, DOI:10.3762/bjoc.10.50.
- M. I. Ansari, M. K. Hussain, N. Yadav, P. K. Gupta and K. Hajela, Silica supported perchloric acid catalyzed rapid N-formylation under solvent-free conditions, Tetrahedron Lett., 2012, 53, 2063–2065, DOI:10.1016/j.tetlet.2012.02.027.
- M. Kaur, S. Sharma and P. M. S. Bedi, Silica supported Brönsted acids as catalyst in organic transformations: A comprehensive review, Chin. J. Catal., 2015, 36, 520–549, DOI:10.1016/S1872-2067(14)60299-0.
- P. M. Price, J. H. Clark and D. J. Macquarrie, Modi fi ed silicas for clean technology, J. Chem. Soc., Dalton Trans., 2008, 2, 101–110 Search PubMed.
- A. Pramanik and S. Bhar, Silica-sulfuric acid and alumina-sulfuric acid: versatile supported Brønsted acid catalysts, New J. Chem., 2021, 45, 16355–16388, 10.1039/d1nj02887a.
- S. A. Salami, X. Siwe-Noundou and R. W. Krause, Catalytic Performance of Immobilized Sulfuric Acid on Silica Gel for N-Formylation of Amines with Triethyl Orthoformate, Molecules, 2022, 27, 4213, DOI:10.3390/molecules27134213.
|
This journal is © The Royal Society of Chemistry 2023 |
Click here to see how this site uses Cookies. View our privacy policy here.