DOI:
10.1039/D2RA05912F
(Paper)
RSC Adv., 2023,
13, 1115-1124
Temporal quantitative profiling of sialyllactoses and sialic acids after oral administration of sialyllactose to mini-pigs with osteoarthritis†
Received
19th September 2022
, Accepted 21st December 2022
First published on 4th January 2023
Abstract
Sialyllactose (SL) is the most abundant acidic oligosaccharide in human breast milk and plays a primary role in various biological processes. Recently, SL has attracted attention as an excellent dietary supplement for arthritis because it is effective in cartilage protection and treatment. Despite the superior function of SL, there are few pharmacological studies of SL according to blood concentrations in arthritis models. In this study, we investigated quantitative changes in SL and sialic acids in the plasma obtained from mini-pigs with osteoarthritis throughout exogenous administration of SL using liquid chromatography-multiple reaction monitoring mass spectrometry. Plasma concentrations of SL and sialic acids in the SL-fed group showed a significant difference compared to the control group. Mini pigs were fed only Neu5Ac bound to SL, but the concentration patterns of the two types of sialic acid, Neu5Ac and Neu5Gc, were similar. In addition, the relative mRNA expression level of matrix metalloproteinases (MMPs), which is known as a critical factor in cartilage matrix degradation, was remarkably decreased in the synovial membrane of the SL-fed group. Consequently, the temporal quantitative profiling suggests that dietary SL can be metabolized and utilized in the body and may protect against cartilage degradation by suppressing MMP expression during osteoarthritis progression.
Introduction
Osteoarthritis (OA) is the most common type of degenerative arthritis caused by the gradual loss and secondary changes in articular cartilage.1 OA is found in more than 3% of the world's population and continues to increase.2 In particular, since cartilage tissue does not regenerate normally once damaged, chronic damage causes fatal osteoarthritis and limits daily life and occupational activities.3 To date, drugs for the treatment of OA have been relatively rare, ineffective, and have problems in efficacy and safety. Non-steroidal anti-inflammatory drugs (NSAIDs), which are representative arthritis drugs, are well known as effective drugs for the treatment of joint pain and inflammation,4 but long-term use can cause various side effects such as cardiovascular risk and gastrointestinal risk.5–7 Therefore, in recent years, there has been an increasing demand for alternative approaches such as safe dietary sources for the treatment of arthritis.
Sialyllactose (SL) is an emerging natural therapeutic agent for OA with the anti-inflammatory property and guaranteed safety.8–12 SL is the most abundant acidic oligosaccharide in human milk oligosaccharides (HMOs),13,14 and is a trisaccharide molecule containing a sialic acid unit bound to a lactose at the 3 and 6 positions.15,16 Interestingly, a recent study demonstrated that 3′-SL leads to suppression of interleukin-1β (IL-1β) induced oxidative stress and inflammation in chondrocyte cells.17 Oxidative stress has been previously reported to be involved in synovial inflammation, chondrocyte death, and signaling pathways in the pathogenesis of OA.18 Several studies have found that free and bound sialic acid (SA) possess anti-oxidative properties19 SA is an important building block with immunomodulatory properties. In particular, sialylation on IgG has been shown to have anti-inflammatory effects as it down-regulates the immune response by binding to the inhibitory IgG receptors on dendritic cells (DCs).20,21 Interestingly, dietary sialylated glycoconjugates may be directly involved in the metabolic and biosynthetic pathway of SA. Previous studies have demonstrated that orally administrated SA or sialylated glycoconjugates are transported to cellular lysosomes via micropinocytosis and then incorporated into cell surface sialo-glycoconjugates.22,23 In addition, it has been reported that the sialylated glycoconjugates form such as SL has higher bioavailability than the free SA form.24
Another study explored the therapeutic impact of SL on OA development in mouse.25 Briefly, the Yang group determined that SL promotes the synthesis of collagen type II alpha 1 chain (Col2a1) involved in cartilage formation and inhibits the expression of cyclooxygenase2 (Cox2) and matrix metalloproteinases (MMPs) that induce cartilage matrix degradation. These results suggest that SL demonstrates potential as a therapeutic agent for OA by modulating key factors involved in the onset and progression of OA. Despite molecular evidence and potential beneficial effects of SL in OA, there are no studies examining the direct effect of SL supplementation on pharmacological changes in biological body fluids.
Mass spectrometry (MS) has been recognized as a powerful tool for composition profiling and structural elucidation of oligosaccharides by providing high sensitivity and high resolution.26 Recently, MS-based absolute quantitation for native HMOs had been developed and well established.27–30 In particular, multiple reaction monitoring (MRM) technique for the triple quadrupole (QqQ) has excellent advantages in removing disturbances that degrade accuracy and low detection limit.31,32 In other words, by eliminating background noise and detecting unique product ions in a sample, target substances can be quantified with very low detection limits, even in complex media. In addition, liquid chromatography (LC) column packed with porous graphitized carbon (PGC) provides excellent isomer separation with high reproducibility for polar compounds.33–36
In the current study, we employed LC-MRM MS and MS/MS combined with PGC-based stationary phase to quantify and evaluate the SLs (3′-SL and 6′-SL) and SAs (Neu5Ac and Neu5Gc) in OA model mini-pig plasma samples. The study included two group: a placebo group (placebo tablet, n = 4) and an SL-intake group (SL tablet, n = 12). SL supplementation group further subdivided by their dose volume and type into those with G2 (3′-SL 200 mg per head, n = 4), G3 (3′-SL 400 mg per head, n = 4), and G4 (3′-SL and 6′-SL 200 mg each per head, n = 4). Structural isomer of SL with same molecular weight was completely separated and successfully quantified the concentration ranged from 153.2 ng mL−1 to 226.5 ng mL−1 for 3′-SL and from 34.4 ng mL−1 to 61.2 ng mL−1 for 6′-SL in mini-pig plasma level. We successfully elucidated the temporal quantitative profile of SLs and SAs according to oral administration of SL in OA model mini-pig plasma for 12 weeks between the placebo and SL-intake groups. It showed dose-dependent profiles according to the ingested dose type and amount, and a significant change in plasma concentration was revealed in the SL-intake group compared to the control group. This study demonstrates the bioavailability of dietary SL in the body through the quantitative profiling of SLs and its metabolite SAs in the plasma, and also suggests the therapeutic potential of SL on OA progression through the changes in inflammatory factors in the joint. Pharmacological changes in blood concentrations are scientific evidence supporting bioavailability predictive of the effectiveness of an active substance (SL in this study), and may serve as molecular evidence for the potential therapeutic effect of SLs in OA. These pharmacological changes in the blood of OA model mini-pigs could be essential reference data in the exploratory clinical trial stage conducted on humans in the future.
Experimental
Materials and reagents
Placebo, 3′-SL, 6′-SL tablets, and standard for 3′-SL and 6′-SL were provided by GeneChem Inc. (Daejeon, Korea). Other standard materials of N-acetylneuraminic acid (Neu5Ac), N-glycolylneuraminic acid (Neu5Gc), and maltotriose were purchased from United States Pharmacopeia (USP). Acetonitrile (HPLC grade) and ethanol (HPLC grade) were purchased from Fisher Scientific (Rockingham, USA). Deionized water (HPLC grade) was purchased from J.T Baker (Center Valley, USA). Sodium borohydride (NaBH4) and formic acid were purchased from Sigma-Aldrich (Saint Louis, USA). Neuraminidase (P0720L) obtained by New England Biolabs (Ipswich, USA).
Mini-pig plasma samples
All experimental protocols were approved by the Animal Care and Use Committee of the Korea Institute of Toxicology (KIT) and complied with the Association for Assessment and Accreditation of Laboratory Animal Care International Animal Care Policies (Approval No. 1908-0276, 1912-0403).
The efficacy evaluation test was conducted via repeated oral administration of SL to Sus scrofa/Yucatan OA model mini-pigs for 12 weeks. Mini-pigs were kept in a cage at constant temperature (23 ± 3 °C) and 30–70% humidity and were maintained on a regular 12 h light/12 h dark cycle and were fed 25 g kg−1 per day of food. Two groups of plasma donors were selected: placebo group (G1, placebo tablet, n = 4) and SL-intake group (n = 12). The mini-pigs of SL-intake group could be further subdivided by their dose volume into those with G2 (3′-SL 200 mg per head, n = 4), G3 (3′-SL 400 mg per head, n = 4), and G4 (3′-SL and 6′-SL 200 mg each per head, n = 4). For the dose setting of SLs administered to mini-pigs, we firstly calculated the equivalent dose per head for human of 60 kg body weight based on the conversion factor according to the guidelines of the US FDA from the dose that showed efficacy in a rodent model.37 The conversion factor of mini-pig and human is almost equal to 1 in accordance with the guidance, so calculated the human equivalent dose was applied to mini-pigs. The average weight of 16 mini-pigs in the present study was 60 kg, which was similar to that of human. OA was induced by anterior cruciate ligament transection (ACLT) surgery that has the same phenotype as degenerative arthritis.38 ACLT surgery was performed 4 weeks after the first dose of the 12 weeks dosing period. The placebo and SL substances were administered orally once a day for 12 weeks. Blood samples were collected at pre-dose (0) as well as 2, 4, 8, 10, and 12 weeks in all groups. All animals were fasted for 16 hours before blood collection. After blood collection, plasma was obtained by centrifugation at 3000 rpm for 10 min at room temperature and thereafter stored at −80 °C.
Preparation of stock and working solution, calibration, and quality control (QC) samples
Stock solutions of 3′-SL and 6′-SL were prepared separately by dissolving each compound in water at a concentration of 0.1 mg mL−1. Before the dilution for working solutions, all oligosaccharides were incubated at 42 °C water baths for 16 h in 1.0 M sodium borohydride to reduce the free oligosaccharides from aldehyde to alditol form.39 The working solutions of 3′-SL and 6′-SL were obtained by serial dilution of the stock solution with water. The stock solution of maltotriose (internal standard: IS) was prepared by dissolving in HPLC grade water at a concentration of 0.1 mg mL−1. Pooled mini-pig plasma at pre-dose was used to prepare the linearity and quality control samples. Linearity samples in the pooled plasma were prepared by serial dilution of stock solution at final concentrations of 40, 100, 200, 400, 1000, and 2000 ng mL−1 for 3′-SL and 6′-SL. This same method was also used to prepare QC samples at final concentrations of 160 (low QC; QL), 800 (medium QC; QM), and 1600 (high QC; QH) ng mL−1.
Sample preparation for 3′-SL and 6′-SL
The reduction from aldehyde to alditol form of 3′-SL and 6′-SL was performed with slight modifications according to optimized procedures.39 IS and 1.0 M sodium borohydride solution were added to 50 μL mini-pig plasma. Double blank, blank, calibration, and QC samples were added deionized water instead of the sodium borohydride solution. All samples were incubated in a water bath at 42 °C for 16 h. The reaction was stopped by adding 1.0 M hydrochloric acid solution until up to pH 5.0–7.0 of the solution was attained. During this step, samples were kept in an ice bath. Then, 80% (v/v) of chilled ethanol was added and stored at −80 °C for about 1 h to precipitate the proteins. After 20 min of centrifugation, the oligosaccharide-rich supernatant was filtered through a 0.22 μm PVDF membrane (Millipore, USA) and transferred into a new tube. Then the residue dried in vacuo and dissolved in mobile phase solution prior to LC-MS analysis.
Sample preparation for Neu5Ac and Neu5Gc
IS added to 50 μL mini-pig plasma were thermally denatured in aqueous buffer containing dithiothreitol and ammonium bicarbonate before digestion. Enzyme digestion reaction of SA residues (both Neu5Ac and Neu5Gc) was performed according to the neuraminidase protocol provided by New England Biolabs (Ipswich, USA). Desialylated proteins were precipitated out with ethanol, and the supernatant was clean up by PVDF membrane. The final collection completely dried under vacuum and reconstituted with mobile phase solution before MS analysis.
UHPLC/QqQ-MS conditions
Quantitation of SLs and SAs was performed using a QqQ MS (triple-quadrupole mass spectrometer 3500, AB Sciex, Framingham, MS, USA) equipped with a Sciex ExionLC AC system and a Hypercarb PGC column (2.1 × 100 mm i.d., 3 μm particle size) (Thermo Scientific). Chromatographic separation and MS analysis for SLs and SAs were performed with slight modification from developed methods.27,30 The aqueous mobile phase (A) was 10 mM ammonium bicarbonate in water and the organic mobile phase (B) was a mixture of acetonitrile and water (80
:
20, v/v) in 10 mM ammonium bicarbonate for the analysis of 3′-SL and 6′-SL. The flow rate was set at 200 μL min−1. The binary gradient was as follows: 1 min of 98% solvent A, increased solvent B from 2% to 45% in 8.2 min and then to 95% solvent B in 0.8 min, stayed at 95% solvent B for 1 min, then re-equilibrate at 98% solvent A for 7 min. For Neu5Ac and Neu5Gc analysis, the mobile phase (A) was 3% acetonitrile + 0.1% formic acid in water, whereas the organic mobile phase (B) was 90% acetonitrile + 0.1% formic acid in water. The flow rate was set at 200 μL min−1. The binary gradient was as follows: 0.5 min of 100% solvent A, increased solvent B from 0% to 10% in 6.0 min and then to 100% solvent B in 2.0 min, then re-equilibrate at 98% solvent A for 4 min. Electrospray ionization was in negative ion mode. The ion source temperature was set at 600 °C with an ion-spray voltage of −4500 V, and the other parameters were optimized as follows: curtain gas = 20 psi; collision gas = 9 psi; nebulizing gas (GS1) = 50 psi; drying gas (GS2) = 50 psi. Extracted ion chromatograms (XICs) of SLs, Neu5Ac, Neu5Gc, and IS were obtained at m/z 634 → 290, m/z 308 → 87, m/z 324 → 116, and m/z 505 → 221, respectively. MRM peak integration was used by Analyst (ver. 1.7.1) and SCIEX OS-MQ (MultiQuant) software (ver. 1.7.0.36606).
Method validation for 3′-SL and 6′-SL in mini-pig plasma
The analytical method was validated following US Food and Drug Administration (FDA) guidelines for the matrix effect, recovery, calibration curve (linearity), selectivity, accuracy, precision, and stability.40
Matrix effect. The matrix effect was evaluated on low- and high-quality control samples using the IS-normalized matrix effect. The equation of the matrix factor (MF) and IS-normalized MF are as follows:
Recovery. The recovery rate is also related to the extraction efficiency of an assay. The recovery rate does not need to be 100%, but the recovery rate of an analyte must be constant, precise, and reproducible. The recovery rate test was evaluated by repeating the analysis of the three concentration quality control samples six times at each concentration. The recovery percentages of the spiked known concentration into blank sample were compared between before and after extraction step.
Linearity. The calibration curve consisted of a blank sample (a biological sample tested without an IS), a zero sample (a biological sample tested with only an IS), and samples at concentrations of 40, 100, 200, 400, 1000, and 2000 ng mL−1. The concentration of at least 75% of the calibration curve concentration, including the minimum and maximum quantification limits, must meet the acceptance criteria within ± 15% of the deviation from the theoretical value (lower limit of quantification, LLOQ is ± 20%).
Selectivity. Selectivity refers to the analytical ability to separate and quantify the analyte in the presence of other substances in a biological sample. When evaluating selectivity, the analysis of the blank sample of a biological sample should be performed using at least six biological samples of different origins. Originally, because SL is an endogenous material, it must be verified by other methods such as ion transition ratio for this purpose, but this does not apply to the analysis method modified with the alditol form developed in this study. It was evaluated at the lowest quantification limit in the blank sample and mini-pig plasma of each of the six animals.
Accuracy and precision. The accuracy of an analysis method refers to the proximity of the average test result obtained by the analysis method to the theoretical value of an analyte. When evaluating the accuracy, four concentrations (LLOQ, QL, QM, and QH) were evaluated by conducting six repeated tests within a day at each concentration for 3 days. The concentration obtained by the back calculation method must be within 15% of the theoretical value (the minimum quantification limit is 20%), and the CV (%) must satisfy 15% or less.
Stability. Stability was evaluated in standard stock solutions as well as low and high concentration quality control samples. The cold-thaw stability test was analyzed after repeating the conditions of freezing for 12–24 h and thawing without an auxiliary device at room temperature three times. Benchtop and standard stock solution stabilities were evaluated after leaving at room temperature for at least 4 h.
Isolation of mRNA and RT-PCR
Total RNA was isolated using Trizol (15596018, Life Technologies, Carlsbad, CA, USA). Total cellular RNA was used to synthesize cDNA using a Quantitect Reverse Transcription Kit (205313, Qiagen, Hilden, Germany) according to the manufacturer's instructions.
Real-time quantitative PCR
Quantitative polymerase chain reaction (qPCR, Power SYBR™ Green PCR Master Mix, 4368702, Applied Biosystems, Foster City, CA, USA) was conducted using porcine primers for GAPDH, MMP1 and MMP12. All the qPCR primers were designed by Primer 3 V0.4.0 (Table S1†). qPCR was as performed as follows: 95 °C for 10 min, 40 cycles of 95 °C for 15 s and 60 °C for 1 min on a PCR machine (A28134, Applied Biosystems). mRNA levels were calculated using GAPDH (ΔCt = Ct gene of interest − Ct GAPDH) and reported as relative mRNA expression (ΔΔCt = 2ΔCt sample−ΔCt control) or the fold-change.
Statistical analysis
Statistical analysis was performed using Microsoft Office Excel and GraphPad Prism version 5 software. One-way ANOVA followed by Dunnett's multiple comparisons test was carried out to determine the significant differences (*P < 0.05, **P < 0.01, ***P < 0.001).
Results and discussion
The ultimate objective of this study was to evaluate the efficacy of SL in the plasma level of OA model mini-pigs between placebo and SL-intake group. Fig. 1A describes the experimental schedules of dietary supplement and blood collection. Information on groups and dosage of SLs is shown in Fig. 1B. All mini-pigs were orally administrated placebo and SL tablets daily during the week for 12 weeks and blood samples were collected six times every 2 weeks except at 6 weeks. In a preliminary study on rodents, efficacy was showed in the 3′-SL administration group (50 mg kg−1 and 100 mg kg−1, data not shown). The dose for rodents was converted into the mini-pig dose, and the low (200 mg per head per day) and high (400 mg per head per day) concentration groups were set in consideration of the human clinical trials. A mixed agent administration group (3′-SL and 6′-SL 200 mg per head per day) was added since the pig has both α-2,3 or α-2,6 linked SA. Quantifying the SLs altered by dietary supplements in biological samples is challenging because they are already present in the body. Moreover, they are linkage isomers containing Neu5Ac residues at the α-2,3 or α-2,6 binding position of lactose. The β-elimination reaction allowed us to obtain temporal and quantitative profiles of 3′-SL and 6′-SL except for intrinsic concentration in plasma of mini-pigs. The aldehyde form of SL is changed to the alditol form through this reaction, resulting in a difference of 2 Da in the m/z value of the precursor (m/z 632 → m/z 634). As shown in Fig. 2, it was confirmed that there was no interference of other substances in the retention time of SLs in the double blank and zero blank in the plasma samples. Chromatographic separation of linkage isomers in which Neu5Ac binds to lactose at the α-2,3 or α-2,6 linkage positions was also successfully achieved based on PGC stationary phase (Fig. 2A). To assess the efficacy of SL supplementation in OA progression, target compounds (3′-SL, 6′-SL, Neu5Ac, and Neu5Gc) were quantitatively profiled in the plasma of OA model mini-pigs.
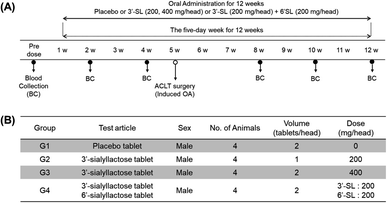 |
| Fig. 1 (A) Experimental schedule of sialyllactoses (SLs) supplementation. (B) Information for group and dosage. | |
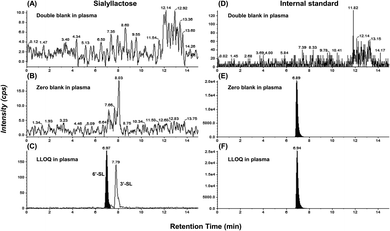 |
| Fig. 2 Extracted ion chromatograms (XICs) monitored at (A–C) m/z 634.2 → m/z 290.0 of 3′-SL and 6′-SL and (D–F) m/z 505.2 → m/z 221.0 of internal standard: double blank, zero blank, and lower limit of quantification (LLOQ) in mini-pig plasma. | |
Calibration curves of 3′-SL and 6′-SL were generated from three replicate LC-MRM MS measurements using 6 concentration points of a standard solution of alditol form. All correlation coefficients were 0.99 from 40 to 2000 ng mL−1 with acceptance criteria in that the accuracy (RE) and precision (CV) were within ± 15% (±20% for LLOQ) as listed in Table S3.† Another target substances, Neu5Ac and Neu5Gc, were measured by neuraminidase to the total (bound and free, respectively) SAs present in plasma. The measured concentrations at each point of calibration curve were calculated from a baseline adjusted by subtracting the pre-dose level as a commonly used method. Linear regression for Neu5Ac and Neu5Gc showed good linearity (R2 = 0.99) in the range of 10 to 500 μg mL−1 (Neu5Ac) and 100 to 5000 ng mL−1 (Neu5Gc) at 6 concentration points of standard solutions within acceptance criteria. The concentration of each sample was calculated using the back-calculation according to each calibration equation. Prior to this study, we performed bioanalytical method validation on mini-pig plasma level in accordance with the USFDA bioanalytical method guidelines. For each point of guidelines, all values satisfied the acceptance criteria (Tables S2–S6†).
Dose-response profile of 3′-SL and 6′-SL in OA model mini-pigs
Relative and absolute concentrations of 3′-SL and 6′-SL in mini-pig plasma samples over 12 weeks between the placebo and SL-intake groups are shown in Fig. 3 and S1.† Relative quantitation was performed for clearer comparisons between two groups by reducing inter-individual variation. Relativity was calculated as the percentage of each point in the sum of the total area of the evaluation period. For 3′-SL, the concentration of the placebo group ranged from 153.2 ± 22.7 to 201.4 ± 23.6 ng mL−1, and that of the SL-intake group was from 169.7 ± 19.2 to 221.7 ± 29.8 ng mL−1 (Fig. S1A–D†). Interestingly, the concentration pattern was reversed after ACLT surgery between the placebo (G1) and SL-intake groups (G2, G3 and G4). 3′-SL in the placebo group decreased from 181.8 ± 40.8 ng mL−1 at 4 weeks to 161.8 ± 11.7 ng mL−1 at 8 weeks after ACLT surgery at 5 weeks. However, all SL-intake groups revealed increasing pattern. In addition, the concentration represented significant differences between the pre-dose and 8 weeks point in relative terms on G2 group (P < 0.05) (Fig. 3B). At the end time point, 3′-SL was significantly increased in the SL-intake G4 group (P < 0.05) (Fig. 3D), whereas there was no significant difference between the pre-dose point and endpoint in the placebo group.
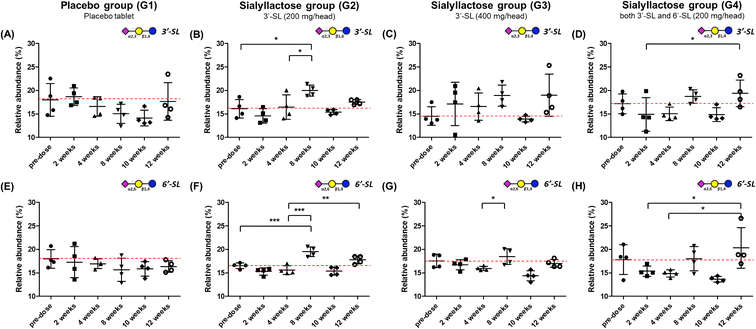 |
| Fig. 3 Relative concentration–time profile of 3′-SL and 6′-SL after oral administration of placebo and SL tablet to OA model mini-pigs. Relative abundances (%) are shown in upper panel for 3′-SL (A–D) and lower panel 6′-SL (E–H), respectively. Placebo group (placebo tablet supplementation), n = 4; G2 group (3′-SL 200 mg per head), n = 4; G3 group (3′-SL 400 mg per head), n = 4; G4 group (both 3′-SL and 6′-SL 200 mg per head), n = 4. Values are means ± SDs, * means differ, P < 0.05; **P < 0.01; ***P < 0.001. | |
Overall, the absolute concentration of 6′-SL in the placebo group was lower than in the SL-intake groups (Fig. S1E†). 6′-SL also showed the greatest difference 8 weeks after surgery, which was same as the above 3′-SL result. 6′-SL in the placebo group decreased from 37.6 ± 14.1 ng mL−1 at 4 weeks to 34.4 ± 12.1 ng mL−1 at 8 weeks, while 6′-SL in all SL-intake groups increased (Fig. S1E–H†). In terms of relative abundance, the concentration of 6′-SL at 8 weeks was significantly different between the groups. 6′-SL in the G2 group increased significantly at 8 weeks (P < 0.001) and the endpoint (P < 0.01) compared to the concentration at 4 weeks before surgery (Fig. 3F). Also, 6′-SL in the G4 group increased at 12 weeks (P < 0.05) compared to the concentration at 2 weeks and 4 weeks before surgery (Fig. 3H). This clearly suggests that dietary SL can complement the concentration of SL in blood level. Moreover, the highest concentration of 3′-SL at the endpoint was found in the G3 group where 3′-SL was administered the most (Fig. S1C†). And the 6′-SL was the highest in the G4 group which is the only SL-intake group including 6′-SL dosage form (Fig. S1H†).
Dose-response profile of Neu5Ac and Neu5Gc in OA model mini-pigs
Fig. 4 illustrate the relative concentration of Neu5Ac and Neu5Gc in mini-pig plasma level for 12 weeks between the placebo and SL-intake groups. The concentration of total Neu5Ac in the placebo group ranged from 156.7 ± 19.2 to 217.3 ± 54.8 μg mL−1, and Neu5Gc was found to be in the range of 171.3 ± 19.0–237.2 ± 55.7 μg mL−1 (Fig. S2A and E†). Both Neu5Ac and Neu5Gc in the placebo group tended to increase until at 4 weeks just before surgery and then steadily decreased after surgery. The concentration of the last point drastically decreased to 156.7 ± 19.2 and 171.3 ± 19.0 μg mL−1 for Neu5Ac and Neu5Gc, respectively, compared with their corresponding at 4 weeks preoperative concentration of 217.3 ± 54.8 and 237.2 ± 55.7 μg mL−1. It was revealed that the relative abundance at the last week significantly decreased in both NeuA5c and Neu5Gc compared with the value at 4 weeks (P < 0.05) (Fig. 4A and E). On the other hand, in the SL-intake groups, the concentration of SAs was maintained without significant change during the 12 weeks. Interestingly, the concentration patterns of the two types of SA, Neu5Ac and Neu5Gc, were similar, although mini-pigs in SL-intake group were fed only Neu5Ac conjugated in SL. These results strongly suggest that dietary SL may affect the endogenous metabolic and biosynthetic pathway of SA. In addition, this is consistent with previous showing that dietary SL or SA affects SA concentration in the brain of mini-pigs and saliva of newborns.41–43
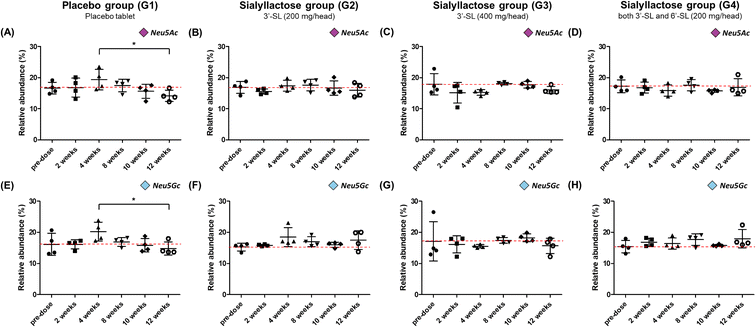 |
| Fig. 4 Relative concentration–time profile of Neu5Ac and Neu5Gc after oral administration of placebo and SL tablet to OA model mini-pigs. Relative abundances (%) are shown in upper panel for Neu5Ac (A–D) and lower panel Neu5Gc (E–H), respectively. Placebo group (placebo tablet supplementation), n = 4; G2 group (3′-SL 200 mg per head), n = 4; G3 group (3′-SL 400 mg per head), n = 4; G4 group (both 3′-SL and 6′-SL 200 mg per head), n = 4. Values are means ± SDs, * means differ, P < 0.05; **P < 0.01; ***P < 0.001. | |
Oral administration of SL reduced expression of MMPs induced by pro-inflammatory cytokines on the synovial membrane of OA model mini-pigs
Interleukin (IL)-1β, IL-6, IL-17 and tumor necrosis factor (TNF)-α are representative pro-inflammatory cytokines implicated in the pathogenesis of OA and have been shown to play important roles in the OA development.44,45 The onset of OA pathogenesis is associated with their inordinate production, which is responsible for a critical factor in joint damage primarily caused by up-regulation of matrix metalloproteinases (MMPs; MMP-1, 2, 3, 12, 13 and 16) and cyclooxygenase2 (Cox2) expression. Interestingly, previous in vitro and in vivo studies using chondrocyte cell and mouse model revealed that SL effectively inhibits matrix degradation and inflammatory activation through regulation of MMPs and Cox2 expression in chondrocytes.25 To investigate the function of SL in cartilage, mRNA expression levels of MMPs (MMP-1 and 12) in the synovial membrane were determined by qRT-PCR (Fig. 5). The mRNA levels of target genes were normalized to those of GAPDH and expressed as relative quantitation to placebo group. As a result, the expression of MMP-1 was markedly decreased in SL-fed group (P < 0.05), and MMP-12 had no significance, but represented a lower expression level than that of the placebo group, showing the same trend as that of MMP-1. Consequently, these results strongly support that inflammatory mediator in the cartilage can be modulated by SL supplementation in the OA model mini-pigs.
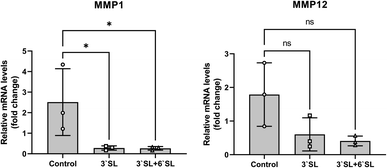 |
| Fig. 5 Relative mRNA level of MMPs on the synovial membrane after SL administration for 12 weeks determined by qRT-PCR. Placebo group (placebo tablet supplementation); 3′-SL and 6′-SL group (SL tablet supplementation). Values are means ± SDs; ns, not significant; * means differ, P < 0.05. | |
While these findings indicate that dietary SLs are might play a role in OA progression of mini-pigs, an important next step must be elucidated the actual dose that elicits a therapeutic effect in human with arthritis.
Conclusions
We investigated the efficacy of SL by quantitative analysis of SL and SA on plasma level in OA model mini-pig following oral administration of SL using an efficient and reliable LC-MRM MS method. During the course of osteoarthritis, the efficacy of SL supplementation could be confirmed through quantitative changes in blood concentration significantly increased in the SL-intake group. In addition, dose-dependent quantitative profiling showed that the intake type and amount of SL affected the 3′-SL and 6′-SL concentration in mini-pig plasma.
Interestingly, dietary sources such as sialylated glycoconjugates and free SA are known to be directly incorporated into this metabolic and biosynthetic pathway for SA production.19 Therefore, based on our vivid data and results, we suggest that dietary SL can be utilized as a source of blood SA via macropinocytosis (Fig. 6). Briefly, ingested SL enters intracellular lysosomes through macropinocytosis, Neu5Ac at the terminal of SL is released by neuraminidase, and the released Neu5Ac is salvaged to synthesize CMP-Neu5Ac in the cell nucleus. SAs (Neu5Ac and Neu5Gc) biosynthesized from CMP-Neu5Ac are delivered to the Golgi apparatus and then utilized at the terminal sites of secreted free oligosaccharides or cell surface glycoconjugates expressed by specific sialyltransferases, respectively. In this study, both Neu5Ac and Neu5Gc were significantly decreased in the placebo group, but remained unchanged in the SL-intake group. These results clearly indicate that dietary SL can be a source of SA in the body.
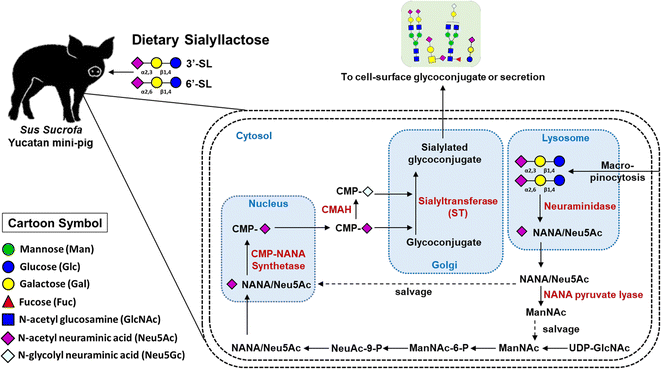 |
| Fig. 6 Illustration of the metabolic and biosynthetic pathway accordance with dietary SL in mini-pig blood level. | |
We observed a trend to decrease the relative mRNA levels of MMPs in the synovial membrane of mini-pigs compared to the placebo group after 12 weeks of SL administration. These pharmacological changes in blood are valid evidence that dietary SL suppresses inflammatory cytokines and matrix degradation during OA progression.
Abbreviations
HMOs | Human milk oligosaccharides |
SL | Sialyllactose |
SA | Sialic acid |
Neu5Ac | N-Acetylneuraminic acid |
Neu5Gc | N-Glycolylneuraminic acid |
OA | Osteoarthritis |
PGC | Porous graphitized carbon |
UHPLC | Ultraperformance liquid chromatography |
FDA | Food and drug administration |
NSAIDs | Non-steroidal anti-inflammatory drugs |
IL-1β | Interleukin-1β |
Col2a1 | Collagen type II alpha 1 chain |
Cox2 | Cyclooxygenase2 |
MMPs | Matrix metalloproteinases |
DCs | Dendritic cells |
IgG | Immunoglobulin G |
MS | Mass spectrometry |
MRM | Multiple reaction monitoring |
QqQ | Triple quadrupole |
QC | Quality control |
IS | Internal standard |
MF | Matrix factor |
LLOQ | Lower limit of quantification |
Author contributions
Dan Bi Park: conceptualization, validation, formal analysis, investigation, writing – original draft, visualization. Lila Kim: funding acquisition, project administration, visualization. Jeong Ho Hwang: resources, methodology. Kyung-Tai Kim: formal analysis, resources. Ji Eun Park: investigation Jong-Soon Choi: writing – review & editing. Hyun Joo An: conceptualization, writing – review & editing, supervision.
Conflicts of interest
There are no conflicts to declare.
Acknowledgements
This research was supported by the Ministry of Trade, Industry & Energy (MOTIE), and the Korea Institute for Advancement of Technology (KIAT) through the Encouragement Program for the Industries of Economic Cooperation Region (P0004751).
References
- A. Latourte, M. Kloppenburg and P. Richette, Emerging pharmaceutical therapies for osteoarthritis, Nat. Rev. Rheumatol., 2020, 16, 673–688 CrossRef PubMed.
- Y. T. Gu, J. Chen, Z. L. Meng, W. Y. Ge, Y. Y. Bian, S. W. Cheng, C. K. Xing, J. L. Yao, J. Fu and L. Peng, Research progress on osteoarthritis treatment mechanisms, Biomed. Pharmacother., 2017, 93, 1246–1252 CrossRef CAS PubMed.
- M. M. Kawano, I. L. Araujo, M. C. Castro and M. A. Matos, Assessment of quality of life in patients with knee osteoarthritis, Acta Ortop. Bras., 2015, 23, 307–310 CrossRef PubMed.
- B. N. Lindler, K. E. Long, N. A. Taylor and W. Lei, Use of Herbal Medications for Treatment of Osteoarthritis and Rheumatoid Arthritis, Medicines, 2020, 7(11) DOI:10.3390/medicines7110067.
- F. Berenbaum, New horizons and perspectives in the treatment of osteoarthritis, Arthritis Res. Ther., 2008, 10(2), S1 CrossRef PubMed.
- A. Mobasheri and M. Batt, An update on the pathophysiology of osteoarthritis, Ann. Phys. Rehabil. Med., 2016, 59, 333–339 CrossRef PubMed.
- N. Veronese, C. Trevisan, M. De Rui, F. Bolzetta, S. Maggi, S. Zambon, E. Musacchio, L. Sartori, E. Perissinotto, G. Crepaldi, E. Manzato and G. Sergi, Association of Osteoarthritis With Increased Risk of Cardiovascular Diseases in the Elderly: Findings From the Progetto Veneto Anziano Study Cohort, Arthritis Rheumatol., 2016, 68, 1136–1144 CAS.
- D. Turck, J. Castenmiller, S. De Henauw, K. I. Hirsch-Ernst, J. Kearney, A. Maciuk, I. Mangelsdorf, H. J. McArdle and A. Naska, Safety of 3′-sialyllactose (3′-SL) sodium salt as a novel food pursuant to regulation (EU) 2015/2283, EFSA J., 2020, 18, e06098 Search PubMed.
- D. Turck, J. Castenmiller, S. De Henauw, K. I. Hirsch-Ernst, J. Kearney, A. Maciuk, I. Mangelsdorf, H. J. McArdle and A. Naska, Safety of 6′-sialyllactose (6′-SL) sodium salt as a novel food pursuant to regulation (EU) 2015/2283, EFSA J., 2020, 18, e06097 CAS.
- S. M. Donovan and S. S. Comstock, Human Milk Oligosaccharides Influence Neonatal Mucosal and Systemic Immunity, Ann. Nutr. Metab., 2016, 69(2), 42–51 Search PubMed.
- D. Kim, R. B. Gurung, W. Seo, A. W. Lee and J. Woo, Toxicological evaluation of 3′-sialyllactose sodium salt, Regul. Toxicol. Pharmacol., 2018, 94, 83–90 CrossRef CAS PubMed.
- R. B. Gurung, D. H. Kim, L. Kim, A. W. Lee, Z. Wang and Y. Gao, Toxicological evaluation of 6′-sialyllactose (6′-SL) sodium salt, Regul. Toxicol. Pharmacol., 2018, 95, 182–189 CrossRef CAS PubMed.
- S. Martin-Sosa, M. J. Martin, L. A. Garcia-Pardo and P. Hueso, Sialyloligosaccharides in human and bovine milk and in infant formulas: variations with the progression of lactation, J. Dairy Sci., 2003, 86, 52–59 CrossRef CAS PubMed.
- G. V. Coppa, P. Pierani, L. Zampini, I. Carloni, A. Carlucci and O. Gabrielli, Oligosaccharides in human milk during different phases of lactation, Acta Paediatr. Suppl., 1999, 88, 89–94 CrossRef CAS PubMed.
- S. J. ten Bruggencate, I. M. Bovee-Oudenhoven, A. L. Feitsma, E. van Hoffen and M. H. Schoterman, Functional role and mechanisms of sialyllactose and other sialylated milk oligosaccharides, Nutr. Rev.Nutr. Rev., 2014, 72, 377–389 CrossRef PubMed.
- B. Wang, Sialic acid is an essential nutrient for brain development and cognition, Annu. Rev. Nutr., 2009, 29, 177–222 CrossRef CAS PubMed.
- A. Baek, S. H. Jung, S. Pyo, S. Y. Kim, S. Jo, L. Kim, E. Y. Lee, S. H. Kim and S.-R. Cho, 3′-Sialyllactose Protects SW1353 Chondrocytic Cells From Interleukin-1β-Induced Oxidative Stress and Inflammation, Front. Pharmacol., 2021, 12, 609817 CrossRef CAS PubMed.
- P. Lepetsos and A. G. Papavassiliou, ROS/oxidative stress signaling in osteoarthritis, Biochim. Biophys. Acta, 2016, 1862, 576–591 CrossRef CAS PubMed.
- C. H. Röhrig, S. S. Choi and N. Baldwin, The nutritional role of free sialic acid, a human milk monosaccharide, and its application as a functional food ingredient, Crit. Rev. Food Sci. Nutr., 2017, 57, 1017–1038 CrossRef PubMed.
- Y. Kaneko, F. Nimmerjahn and J. V. Ravetch, Anti-inflammatory activity of immunoglobulin G resulting from Fc sialylation, Science, 2006, 313, 670–673 CrossRef CAS PubMed.
- S. Böhm, I. Schwab, A. Lux and F. Nimmerjahn, The role of sialic acid as a modulator of the anti-inflammatory activity of IgG, Semin. Immunopathol., 2012, 34, 443–453 CrossRef PubMed.
- C. Oetke, S. Hinderlich, R. Brossmer, W. Reutter, M. Pawlita and O. T. Keppler, Evidence for efficient uptake and incorporation of sialic acid by eukaryotic cells, Eur. J. Biochem., 2001, 268, 4553–4561 CrossRef CAS PubMed.
- N. Yarovaya, R. Schot, L. Fodero, M. McMahon, A. Mahoney, R. Williams, E. Verbeek, A. de Bondt, M. Hampson, P. van der Spek, A. Stubbs, C. L. Masters, F. W. Verheijen, G. M. Mancini and D. J. Venter, Sialin, an anion transporter defective in sialic acid storage diseases, shows highly variable expression in adult mouse brain, and is developmentally regulated, Neurobiol. Dis., 2005, 19, 351–365 CrossRef CAS PubMed.
- K. Banda, C. J. Gregg, R. Chow, N. M. Varki and A. Varki, Metabolism of vertebrate amino sugars with N-glycolyl groups: mechanisms underlying gastrointestinal incorporation of the non-human sialic acid xeno-autoantigen N-glycolylneuraminic acid, J. Biol. Chem., 2012, 287, 28852–28864 CrossRef CAS PubMed.
- J. Jeon, L. J. Kang, K. M. Lee, C. Cho, E. K. Song, W. Kim, T. J. Park and S. Yang, 3′-Sialyllactose protects against osteoarthritic development by facilitating cartilage homeostasis, J. Cell. Mol. Med., 2018, 22, 57–66 CrossRef CAS PubMed.
- S. A. Flowers, C. S. Lane and N. G. Karlsson, Deciphering Isomers with a Multiple Reaction Monitoring Method for the Complete Detectable O-Glycan Repertoire of the Candidate Therapeutic, Lubricin, Anal. Chem., 2019, 91, 9819–9827 CrossRef CAS PubMed.
- Q. Hong, L. R. Ruhaak, S. M. Totten, J. T. Smilowitz, J. B. German and C. B. Lebrilla, Label-free absolute quantitation of oligosaccharides using multiple reaction monitoring, Anal. Chem., 2014, 86, 2640–2647 CrossRef CAS PubMed.
- G. Xu, J. C. Davis, E. Goonatilleke, J. T. Smilowitz, J. B. German and C. B. Lebrilla, Absolute Quantitation of Human Milk Oligosaccharides Reveals Phenotypic Variations during Lactation, J. Nutr., 2017, 147, 117–124 CrossRef CAS PubMed.
- S. Oursel, S. Cholet, C. Junot and F. Fenaille, Comparative analysis of native and permethylated human milk oligosaccharides by liquid chromatography coupled to high resolution mass spectrometry, J. Chromatogr. B: Anal. Technol. Biomed. Life Sci., 2017, 1071, 49–57 CrossRef CAS PubMed.
- O. Csernak, B. Racz, A. Alberti and S. Beni, Quantitative analysis of 3′- and 6′-sialyllactose in human milk samples by HPLC-MS/MS: a validated method for the comparison of two consecutive lactation periods in the same woman, J. Pharm. Biomed. Anal., 2020, 184, 113184 CrossRef CAS PubMed.
- R. A. Yost and C. G. Enke, Triple quadrupole mass spectrometry for direct mixture analysis and structure elucidation, Anal. Chem., 1979, 51, 1251–1264 CrossRef CAS PubMed.
- A. Kulyyassov, M. Fresnais and R. Longuespee, Targeted liquid chromatography-tandem mass spectrometry analysis of proteins: basic principles, applications, and perspectives, Proteomics, 2021, e2100153, DOI:10.1002/pmic.202100153.
- S. Hua, H. J. An, S. Ozcan, G. S. Ro, S. Soares, R. DeVere-White and C. B. Lebrilla, Comprehensive native glycan profiling with isomer separation and quantitation for the discovery of cancer biomarkers, Analyst, 2011, 136, 3663–3671 RSC.
- S. Hua, C. C. Nwosu, J. S. Strum, R. R. Seipert, H. J. An, A. M. Zivkovic, J. B. German and C. B. Lebrilla, Site-specific protein glycosylation analysis with glycan isomer differentiation, Anal. Bioanal. Chem., 2012, 403, 1291–1302 CrossRef CAS PubMed.
- S. Hua, H. N. Jeong, L. M. Dimapasoc, I. Kang, C. Han, J. S. Choi, C. B. Lebrilla and H. J. An, Isomer-specific LC/MS and LC/MS/MS profiling of the mouse serum N-glycome revealing a number of novel sialylated N-glycans, Anal. Chem., 2013, 85, 4636–4643 CrossRef CAS PubMed.
- Y. M. She, R. Y. Tam, X. Li, M. Rosu-Myles and S. Sauve, Resolving Isomeric Structures of Native Glycans by Nanoflow Porous Graphitized Carbon Chromatography-Mass Spectrometry, Anal. Chem., 2020, 92, 14038–14046 CrossRef CAS PubMed.
- US FDA, Guidance for Industry; Estimating the Maximum Safe Starting Dose in Initial Clinical Trials for Therapeutics in Adult Healthy Volunteers.pdf, 2005 Search PubMed.
- B. Wei, M. Zong, C. Yan, F. Mao, Y. Guo, Q. Yao, Y. Xu and L. Wang, Use of quantitative MRI for the detection of progressive cartilage degeneration in a mini-pig model of osteoarthritis caused by anterior cruciate ligament transection, J. Magn. Reson. Imaging, 2015, 42, 1032–1038 CrossRef PubMed.
- S. Ozcan, H. J. An, A. C. Vieira, G. W. Park, J. H. Kim, M. J. Mannis and C. B. Lebrilla, Characterization of novel O-glycans isolated from tear and saliva of ocular rosacea patients, J. Proteome Res., 2013, 12, 1090–1100 CrossRef CAS PubMed.
- R. Meesters and S. Voswinkel, Bioanalytical method development and validation: from the USFDA 2001 to the USFDA 2018 guidance for industry, J. Appl. Bioanal., 2018, 4, 67–73 CrossRef CAS.
- P. McVeagh and J. B. Miller, Human milk oligosaccharides: only the breast, Aust. Paediatr. J., 1997, 33, 281–286 CAS.
- T. H. Tram, J. C. Brand Miller, Y. McNeil and P. McVeagh, Sialic acid content of infant saliva: comparison of breast fed with formula fed infants, Arch. Dis. Child., 1997, 77, 315–318 CrossRef CAS PubMed.
- A. T. Mudd, S. A. Fleming, B. Labhart, M. Chichlowski, B. M. Berg, S. M. Donovan and R. N. Dilger, Dietary Sialyllactose Influences Sialic Acid Concentrations in the Prefrontal Cortex and Magnetic Resonance Imaging Measures in Corpus Callosum of Young Pigs, Nutrients, 2017, 9(12) DOI:10.3390/nu9121297.
- D. Philipot, D. Guerit, D. Platano, P. Chuchana, E. Olivotto, F. Espinoza, A. Dorandeu, Y. M. Pers, J. Piette, R. M. Borzi, C. Jorgensen, D. Noel and J. M. Brondello, p16INK4a and its regulator miR-24 link senescence and chondrocyte terminal differentiation-associated matrix remodeling in osteoarthritis, Arthritis Res. Ther., 2014, 16, R58 CrossRef PubMed.
- Y. Aida, M. Maeno, N. Suzuki, H. Shiratsuchi, M. Motohashi and H. Matsumura, The effect of IL-1beta on the expression of matrix metalloproteinases and tissue inhibitors of matrix metalloproteinases in human chondrocytes, Life Sci., 2005, 77, 3210–3221 CrossRef CAS PubMed.
|
This journal is © The Royal Society of Chemistry 2023 |