DOI:
10.1039/D3QO00457K
(Research Article)
Org. Chem. Front., 2023,
10, 2963-2967
Stereoselective synthesis of five- and six-membered carbocycles via Matteson homologation/ring closing metathesis†
Received
28th March 2023
, Accepted 9th May 2023
First published on 16th May 2023
Abstract
The Matteson homologation is found to be a versatile tool for the stereoselective synthesis of polyunsaturated alkyl boronic esters, which are excellent precursors for the construction of five- and six-membered carbocycles via ring-closing metathesis. The high diversity of the Matteson reaction allows for the preparation of highly substituted cyclic boronic esters, which are also suitable for further homologations.
Introduction
Highly substituted and functionalised carbocycles are widespread in a broad range of natural products, e.g. terpenoids, with interesting biological properties. Common structural motifs are substituted saturated or unsaturated cyclopentanes and -hexanes, as well as combinations of both (Fig. 1).1 Therefore, straightforward and extremely flexible strategies for synthesising these challenging structures are highly desirable. The ring-closing metathesis of functionalised and substituted dienes has been developed as a suitable approach, and many examples have been reported over the last few decades.2
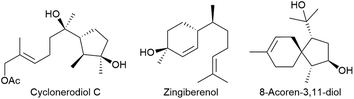 |
| Fig. 1 Selected terpenoid marine natural products. | |
We recently reported the synthesis of allyl boronic esters3via Matteson homologation,4 which could provide suitable candidates for ring-closing metathesis as long as a second double bond could be incorporated during further homologation steps.5 Donald Matteson described this interesting reaction initially in the early 1960s6 and developed it in the 1980s7 as a valuable tool in organic synthesis.8
Typically, a chiral alkyl boronic ester A is reacted with deprotonated dichloromethane in the presence of zinc chloride, generating an α-chloroboronic ester B in a highly stereoselective fashion (Scheme 1A). The addition of a suitable nucleophile onto the boron atom generates a boronate complex, which undergoes a 1,2-shift of the nucleophile to the α-position, replacing the chlorine in a SN2-fashion.5 Ongoing homologation allows the highly stereoselective synthesis of substituted and functionalised carbon chains. Common nucleophiles are alkoxides9 or organometallic compounds, such as alkyl-lithium or -magnesium reagents.10 In addition, allyl zinc reagents are well-suited to introduce unsaturated substituents,11 as well as vinyl organometallics.3,12
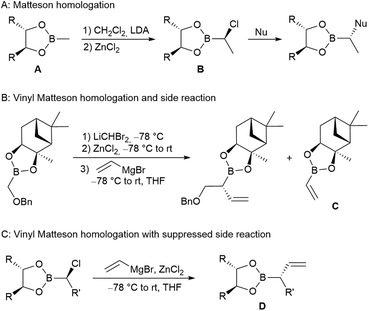 |
| Scheme 1 Matteson homologations. | |
In contrast to alkyl nucleophiles, which are generally not problematic, vinyl nucleophiles tend to undergo various side reactions, such as vinylboronic ester (C) formations (Scheme 1B).13 However, under optimised conditions, where the vinyl Grignard reagent is added in the presence of ZnCl2, these side reactions can be suppressed almost completely, and allyl boronic ester D is obtained in high yields (Scheme 1C).3
We are now interested in such allylboronic esters being further homologated and, in particular, if they allow the introduction of a second double bond, generating suitable substrates for ring-closing metatheses. Hoffmann and others14 have used chiral allyl boronic esters intensively in carbonyl allylations for polyketide syntheses. However, to our knowledge, few examples of Matteson homologations of allyl boronic esters have been reported, probably because of several issues.13,15 For example, Brown et al. reported side reactions, such as allyl migration,16 and Hirschhäuser et al. obtained lowdiastereoselectivity.17
Results and discussion
In recent years, we have applied the Matteson homologation successfully in several syntheses of natural products, in particular, peptides18 and peptide/polyketides conjugates.19 Because of our additional interest in the class of terpenoids, we also investigated methylallylboronic ester 1, which was converted into the corresponding α-chloro boronic ester 2 under our previously optimised conditions (Scheme 2). To this end, dichloromethane was deprotonated with BuLi at −100 °C, and the addition of 1, as well as two equivalents of ZnCl2, resulted in the formation of 2, which was not isolated. The nucleophile, either deprotonated p-methoxybenzylalcohol (at 0 °C) or methyl Grignard reagent (at −78 °C), was added, and the mixture was warmed to room temperature over 24 h. Under these conditions, both desired products 3a and 3b could be obtained in high yields without forming side products.
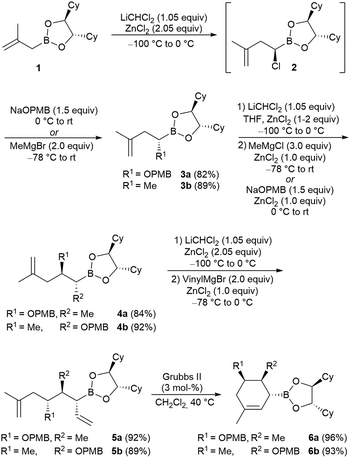 |
| Scheme 2 Synthesis of threefold substituted cyclohexenes 6. | |
The next homologation step proceeded without any problems, and we could obtain the two products 4a and 4b with an inverted substitution pattern. For the introduction of the second double bond, we used vinyl magnesium bromide in the third homologation step.3 In this case, the corresponding α-chloroboronic ester of the first step was isolated and reacted in the presence of exactly one equiv. ZnCl2 with vinyl magnesium bromide. Even with an excess of Grignard reagent, excellent yields of the desired allyl boronic esters 5a and 5b were obtained without the formation of vinylboronic ester as the commonly observed side product. Ring-closing metathesis was performed at 40 °C using second-generation Grubbs catalyst20 generating cyclohexene derivatives 6 containing three stereogenic centres and a threefold substituted double bond. Clearly, the substitution pattern of the linear precursor had no significant influence on the homologation or the cyclisation step.
To also acquire access to fourfold substituted cyclohexenes, we started with the known α-chloroboronic ester 7,3,11 which was reacted with (Z)-1-propenyl magnesium bromide (Scheme 3). The Grignard reagent was freshly prepared from pure (Z)-1-bromopropene, but the desired boronic ester 8 was obtained as a 9
:
1 (Z/E) mixture. During the formation of the Grignard reagent, partial (Z/E)-isomerisation occurred. However, in our case, this phenomenon was completely irrelevant because the methyl substituent would be finally removed in the metathesis step. For this reason, the commercially available E/Z mixture of 1-bromopropene can also be used as an alternative to pure (Z)-1-bromopropene.
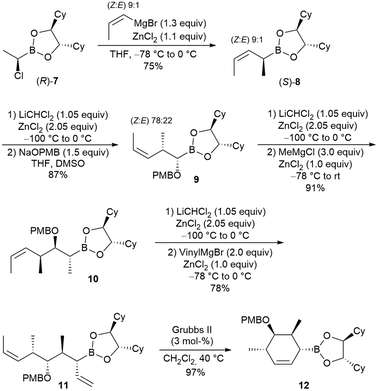 |
| Scheme 3 Synthesis of tetrafold substituted cyclohexene 12. | |
The prolongation of the alkyl chain was performed as described, but interestingly, the (Z/E)-ratio changed slightly in the subsequent homologation step. This result was rather surprising since the reaction conditions should not cause an isomerisation of the double bond.
One explanation might be that the homologation of allylboronic ester 8 does not proceed in a SN2- but a SN′-fashion, at least in part. While SN′ substitution did not change the substitution pattern in this case, it can influence the double-bond geometry via allyl migration. Since the double bond configuration did not matter in our case, we proceeded with the following two homologation steps to boronic ester 11. Its ring-closing metathesis provided the highly substituted cyclohexene 12 as a single stereoisomer in almost quantitative yield.
The corresponding five-membered ring systems also became available by simply reducing the number of homologation steps (Scheme 4). The enantiomer of 9 (ent-9), obtained from the boronic ester (S)-7 containing the enantiomeric chiral ligand, was directly converted into the allylboronic ester 13, which was cyclised to cyclopentene 14 in comparable yield.
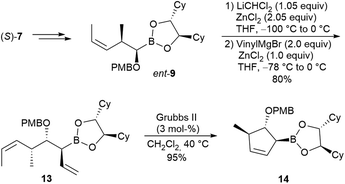 |
| Scheme 4 Synthesis of threefold substituted cyclopentene 14. | |
Finally, we looked more closely at the isomerisation of the double bond in the homologation of allylboronic ester 8. We assumed that at least a partial SN′ might occur during homologation. In principle, this might also happen with boronic ester 1 but, in this case, does not influence product formation.
To investigate this option, we synthesised α-methylated boronic ester 16 starting form known dichloroboronic ester 15 (Scheme 5).14 This smart approach allowed the synthesis of both diastereomeric boronic esters simply by changing the order of nucleophile introduction. We used vinylmagnesium bromide in the first step and methylmagnesium bromide in the second. Therefore, the configuration at the α-position was the opposite of that in the previous example (8), where the methyl group was incorporated first. However, unexpectedly, in the next homologation step, the expected homologation product was not formed at all, but the derivative with a linear side chain and a terminal methyl group at the double bond developed instead. In this case, the homologation proceeded exclusively in a SN′ mode, giving rise to the trans-configured product 17 exclusively. This SN′ reaction was already observed for the formation of the corresponding α-chloroboronic ester intermediate, whereas the second step (nucleophile addition) proceeded in the expected way. Further homologation and ring-closing metathesis provided the double-substituted cyclopentene 19, again in excellent yield.
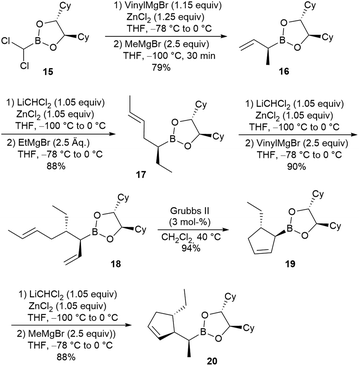 |
| Scheme 5 Synthesis of threefold substituted cyclopentene 19. | |
In all examples investigated, the resulting metathesis products were also allylboronic esters. Therefore, we exemplarily undertook a subsequent homologation step with 19 to ensure the cyclic alkenes could be prolonged predictably. Furthermore, the accustomed high yield could be obtained, indicating that this protocol is well suited for synthesising complex carbocyclic structures. More detailed investigations concerning the allyl migration during the homologation of allylboronic esters are currently underway.
Conclusions
In conclusion, we showed that the high diversity of the Matteson homologation along with the functional group tolerance of the ring-closing metathesis generates an effective tool for the stereoselective synthesis of complex natural products and related structures. No stereoisomers formed in any reaction step, except during allyl migration. However, this isomerisation is not reflected in the final products.
Conflicts of interest
There are no conflicts to declare.
Acknowledgements
Financial support by the Saarland University and the DFG (Ka 880/13-1 and Bruker Neo 500 - 447298507) is gratefully acknowledged.
References
-
(a) Y.-P. Song, X.-H. Liu, Z.-Z. Shi, F.-P. Miao, S.-T. Fank and N.-Y. Ji, Bisabolane, cyclonerane, and harziane derivatives from the marine-alga-endophytic fungus Trichoderma asperellum cf44-2, Phytochemistry, 2018, 152, 45–52 CrossRef CAS PubMed;
(b) Y.-M. Zjou, G.-L. Ju, L. Xiao, X.-F. Zhang and F.-Y. Du, Cyclodepsipeptides and Sesquiterpenes from Marine-Derived Fungus Trichothecium roseum and Their Biological Functions, Mar. Drugs, 2018, 16, 519 CrossRef;
(c) Y.-P. Song, S.-T. Fang, F.-P. Miao, X.-L. Yin and N.-Y. Ji, Diterpenes and Sesquiterpenes from the Marine Algicolous Fungus Trichoderma harzianum X-5, J. Nat. Prod., 2018, 81, 2553–2559 CrossRef CAS PubMed.
-
(a) L. Yet, Metal-Mediated Synthesis of Medium-Sized Rings, Chem. Rev., 2000, 100, 2963–3008 CrossRef CAS PubMed;
(b) K. C. Nicolaou, P. G. Bulger and D. Sarla, Metathesis Reactions in Total Synthesis, Angew. Chem., Int. Ed., 2005, 44, 4490–4527 CrossRef CAS PubMed;
(c) A. Gradillas and J. Pérez-Castells, Macrocyclization by Ring-Closing Metathesis in the Total Synthesis of Natural Products: Reaction Conditions and Limitations, Angew. Chem., Int. Ed., 2006, 45, 6086–6101 CrossRef CAS PubMed.
- T. Kinsinger and U. Kazmaier, Application of Vinyl Nucleophiles in Matteson Homologations, Org. Lett., 2022, 24, 3599–3603 CrossRef CAS PubMed.
- Reviews:
(a) D. S. Matteson, Boronic Esters in Stereodirected Synthesis, Tetrahedron, 1989, 45, 1859–1885 CrossRef CAS;
(b) D. S. Matteson, Boronic Esters in Asymmetric Synthesis, J. Org. Chem., 2013, 78, 10009–10023 CrossRef CAS PubMed;
(c) S. Kirupakaran, H.-G. Korth and C. Hirschhäuser, A Complementary Toolbox of Iterative Methods for Stereoselective Synthesis, Synthesis, 2018, 50, 2307–2322 CrossRef CAS;
(d) D. S. Matteson, B. S. L. Collins, V. K. Aggarwal and E. Ciganik, The Matteson Reaction, Org. React., 2021, 105, 427–860 Search PubMed.
- P. Davoli, R. Fava, S. Morandi, A. Spaggiari and F. Prati, Enantioselective total synthesis of (–)-microcarpalide, Tetrahedron, 2005, 61, 4427–4436 CrossRef CAS.
- D. S. Matteson and R. W. H. Mah, Neighboring Boron in Nucleophilic Displacement, J. Am. Chem. Soc., 1963, 85, 2599–2603 CrossRef CAS.
-
(a) D. S. Matteson and D. Majumdar, α-Chloro Boronic Esters from Homologation of Boronic Esters, J. Am. Chem. Soc., 1980, 102, 7590–7591 CrossRef CAS;
(b) D. S. Matteson and R. Ray, Directed Chiral Synthesis with Pinanediol Boronic Esters, J. Am. Chem. Soc., 1980, 102, 7591–7592 CrossRef.
-
(a) D. S. Matteson and K. M. Sadhu, Boronic ester homologation with 99% chiral selectivity and its use in syntheses of the insect pheromones (3S,4S)-4-methyl-3-heptanol and exo-brevicomin, J. Am. Chem. Soc., 1983, 105, 2077–2078 CrossRef CAS;
(b) D. S. Matteson, H. W. Man and O. C. Ho, Asymmetric synthesis of stegobinone via boronic ester chemistry, J. Am. Chem. Soc., 1996, 118, 4560–4566 CrossRef CAS;
(c) W. C. Hiscox and D. S. Matteson, Asymmetric synthesis of the Japanese beetle pheromone via boronic esters, J. Organomet. Chem., 2000, 614–615, 314–317 CrossRef CAS.
- D. S. Matteson and M. L. Peterson, Synthesis of L-(+)-Ribose via (s)-Pinanediol (αS)-α-Bromo Boronic Esters, J. Org. Chem., 1987, 52, 5116–5121 CrossRef CAS.
- P. B. Tripathy and D. S. Matteson, Asymmetric synthesis of the four stereoisomers of 4-methyl-3-heptanol via boronic esters: Sequential double stereodifferentiation leads to very high purity, Synthesis, 1990, 200–206 CrossRef CAS.
- O. Andler and U. Kazmaier, Application of Allylzinc Reagents as Nucleophiles in Matteson Homologations, Org. Lett., 2021, 23, 8439–8444 CrossRef CAS PubMed.
-
(a) D. S. Matteson and J. J. Yang, Stereoselective chain extension of (R,R)- or (S,S)-1,2-dicyclohexylethane-1,2-diol trityloxymethylboronate to compounds having three stereogenic centers, Tetrahedron: Asymmetry, 1997, 8, 3855–3861 CrossRef CAS;
(b) M. Chen and W. R. Roush, Highly (E)-Selective BF3·Et2O-Promoted Allylboration of Chiral Nonracemic α-Substituted Allylboronates and Analysis of the Origin of Stereocontrol, Org. Lett., 2010, 12, 2706–2709 CrossRef CAS PubMed.
- F. R. Struth and C. Hirschhäuser, A Modular Approach to the Asymmetric Synthesis of Cytisine, Eur. J. Org. Chem., 2016, 958–964 CrossRef CAS.
-
(a) K. Ditrich, T. Bube, R. Stürmer and R. W. Hoffmann, Total Synthesis of Mycinolide V, the Aglycone of a Macrolide Antibiotic of the Mycinamycin Series, Angew. Chem., Int. Ed. Engl., 1986, 25, 1028–1030 CrossRef;
(b) R. W. Hoffmann and A. Schlapbach, Allylboration-reactions, the key to a short synthesis of benzoyl-pedamide, Tetrahedron Lett., 1992, 48, 1959–1968 CrossRef CAS;
(c) S. Breitfelder, A. Schlapbach and R. W. Hoffmann, Stereoselective Synthesis of Alcohols; LII: Synthesis of the C-10 to C-18 Part of the Mycalamides, Synthesis, 1998, 468–478 CrossRef CAS;
(d) M. Wohlfahrt, K. Harms and U. Koert, Asymmetric Allylboration of vic-Tricarbonyl Compounds: Total Synthesis of (+)-Awajanomycin, Angew. Chem., Int. Ed., 2011, 50, 8404–8406 CrossRef CAS.
- D. S. Matteson, R. P. Singh, B. Schafman and J. Yang, Asymmetric synthesis of serricornin via boronic esters, J. Org. Chem., 1998, 63, 4466–4469 CrossRef CAS.
- H. C. Brown and M. V. Rangaishenvi, A simple procedure for the synthesis of three-carbon homologated boronate esters and terminal alkenes via nucleopbilic displacement in α-haloallylboronate ester, Tetrahedron Lett., 1990, 31, 7115–7118 CrossRef CAS.
- K. Bojaryn, C. Hoffmann, F. R. Struth and C. Hirschhäuser, Ambient-Pressure Asymmetric Preparation of S,S-DICHED, a C2-Symmetrical Director for Matteson Reactions, Synlett, 2018, 29, 1092–1094 CrossRef CAS.
-
(a) A. Horn and U. Kazmaier, Synthesis of the Cyclic Heptapeptide Core of Callipeltin A, Org. Chem. Front., 2022, 9, 5213–5218 RSC;
(b) A. Horn and U. Kazmaier, Stereoselective Synthesis of a Protected Sidechain of Callipeltin A, Org. Lett., 2022, 24, 7072–7076 CrossRef CAS PubMed.
-
(a) J. Gorges and U. Kazmaier, Matteson Homologation-based Total Synthesis of Lagunamide A, Org. Lett., 2018, 20, 2033–2036 CrossRef CAS;
(b) O. Andler and U. Kazmaier, A straightforward synthesis of polyketides via ester dienolate Matteson homologation, Chem. – Eur. J., 2021, 27, 949–953 CrossRef CAS PubMed;
(c) O. Andler and U. Kazmaier, Total Synthesis of Apratoxin A using Matteson's Homologation Approach, Org. Biomol. Chem., 2021, 19, 4866–4870 RSC;
(d) M. Tost, O. Andler and U. Kazmaier, A Matteson homologation-based synthesis of doliculide and derivatives, Eur. J. Org. Chem., 2021, 6459–6471 CrossRef CAS;
(e) O. Andler and U. Kazmaier, Stereoselective Synthesis of the Melipomamycin A Side Chain, Org. Lett., 2022, 24, 2541–2545 CrossRef CAS.
- M. Scholl, S. Ding, C. W. Lee and R. H. Grubbs, Synthesis and Activity of a New Generation of Ruthenium-Based Olefin Metathesis Catalysts Coordinated with 1,3-Dimesityl-4,5-dihydroimidazol-2-ylidene Ligands, Org. Lett., 1999, 1, 953–956 CrossRef CAS PubMed.
Footnote |
† Electronic supplementary information (ESI) available: Experimental details, compound characterization, copies of 1H and 13C NMR spectra. See DOI: https://doi.org/10.1039/d3qo00457k |
|
This journal is © the Partner Organisations 2023 |
Click here to see how this site uses Cookies. View our privacy policy here.