DOI:
10.1039/D2QO01421A
(Research Article)
Org. Chem. Front., 2023,
10, 127-132
Divergent synthesis of 5- and 4-(2,1-azaborine) substituted isoxazoles via regioselective [3 + 2] cycloadditions of nitrile oxides and B-ethynyl-1,2-azaborines†
Received
7th September 2022
, Accepted 11th November 2022
First published on 14th November 2022
Abstract
Herein we report the highly regioselective synthesis of 5- and 4-(2,1-azaborine) substituted isoxazoles via [3 + 2] cycloaddition reactions between nitrile oxides and B-ethynyl-1,2-azaborines. 5-(2,1-Azaborine) substituted isoxazoles are favored in the absence of a catalyst, while 4-(2,1-azaborine) substituted isoxazoles are favored in the presence of a ruthenium catalyst. The reaction exhibits excellent regioselectivity, mild reaction conditions, high functional group tolerance and a broad substrate scope.
Introduction
Isoxazoles, especially (hetero)aryl-substituted isoxazoles, are highly important structural units found in drugs and bioactive molecules, such as dopamine D4 receptors, anticancer agents, and antirheumatic drugs (Scheme 1A).1 Therefore, the design and synthesis of structurally diverse isoxazoles2,3 has stimulated a lot of interest in the synthetic community. On the other hand, 1,2-azaborines have garnered considerable attention as isosteres of benzene rings because their aromatic character is preserved and they possess unique electronic properties. These molecules have found potential use in medicinal chemistry, materials science, and catalysis.4 Given that isoxazoles and 1,2-azaborines are two important building blocks in biologically active molecules, we speculate that the merging of isoxazoles with azaborines instead of all-carbon aromatic rings may provide a new opportunity for drug discovery. However, a method to prepare 1,2-azaborine-substituted isoxazoles is extremely rare. To date, the only precedent has been from Molander et al., reporting the synthesis of 4-(2,1-borazaronaphthyl)-isoxazoles via an annulation/aromatization reaction of isoxazolyl trifluoroborate and substituted 2-aminostyrenes under SiCl4 and Et3N, but lower yields and a limited substrate scope have hindered the development of this method.5 Hence, an efficient approach to synthesize 1,2-azaborine-substituted isoxazoles from readily available substrates would facilitate the intensive investigation of the azaborine-isoxazole building blocks for subsequent utilization.
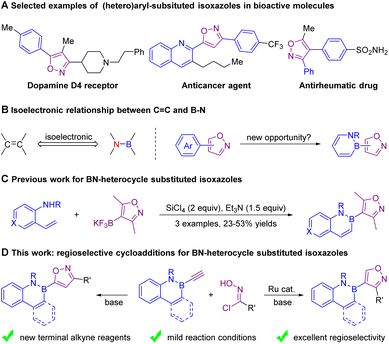 |
| Scheme 1 Regioselective synthesis of 5- and 4-(2,1-azaborine) substituted isoxazoles. | |
The [3 + 2] cycloaddition of nitrile oxides with alkynes has been proved to be an efficient method for the construction of isoxazoles.2,6 In this scenario, we envisioned that the design and synthesis of a class of B-ethynyl-1,2-azaborines (see the ESI† for details) followed by a [3 + 2] cycloaddition with nitrile oxides might achieve the goal of synthesizing structurally diverse 1,2-azaborine-isoxazoles. However, previous [3 + 2] cycloaddition of nitrile oxides with ethynylboronate had exhibited poor regioselectivity;7 therefore, how to control the regioselectivity of such [3 + 2] cycloadditions is a big challenge. Herein we report divergent and highly regioselective [3 + 2] cycloadditions of nitrile oxides and B-ethynyl-2,1-azaborine to afford 5- and 4-(2,1-azaborine) substituted isoxazoles, respectively. This method features excellent regioselectivity, mild reaction conditions, high functional group tolerance and a broad substrate scope.
Results and discussion
We first designed and synthesized a series of B-ethynyl-1,2-azaborines (see the ESI† for details).8 Then, we began our study by using B-ethynyl-2,1-borazaronaphthalene 1a and oxime chloride 2a as the model substrates (Table 1). To our delight, the 3,5-disubstituted isoxazole product 3a was smoothly obtained exclusively in 74% yield using Et3N as the base at room temperature (entry 1). The structure of 3a was determined by X-ray crystallographic analysis (CCDC 2202557†). Further base screening indicated that N,N-diisopropylethylamine (DIPEA) was the best one (entries 2–4), providing 5-(2,1-borazaronaphthyl)-isoxazole 3a in 85% isolated yield (entry 2). Inspired by Fokin's Ru-catalyzed [3 + 2] cycloaddition,9 we then attempted a regioselective synthesis of 3,4-disubstituted isoxazole product 4a by Ru catalysis. When [Cp*RuCl(cod)] was used, 4-(2,1-borazaronaphthyl)-isoxazole 4a was successfully obtained, albeit with poor regioselectivity (the ratio of 4a to 3a is 3.5). This result encouraged us to further study the optimization of the conditions. Investigation of the solvent showed that dichloromethane (DCM) was optimal compared to tetrahydrofuran (THF) and toluene (entries 6–8), and the ratio of 4a to 3a reached 3.8 (entry 6). The regioselectivity was further improved when the loading of 2a and the base was decreased (entry 9). With the addition of additives, the regioselectivity was significantly improved (entries 10–12). When AgOTf was used as the additive, the best regioselectivity (the ratio of 4a to 3a is 39) was achieved with 72% isolated yield (entry 10). Unfortunately, lower catalyst loadings resulted in poor regioselectivity (entry 13).
Table 1 Reaction optimizationa
With the optimized reaction conditions in hand, we first evaluated the compatibility of this regioselective [3 + 2] cycloaddition for 3,5-disubstituted isoxazoles (Scheme 2). In all the cases studied, only 3,5-disubstituted isomers were obtained under the standard conditions. A variety of both electron-donating (3c–3g) and electron-withdrawing (3o) aryl oxime chlorides could be successfully used in this cycloaddition process, affording the desired 5-(2,1-borazaronaphthyl) isoxazoles in good to excellent yields. And remarkably, the 2,4,6-trimethyl substituent (3g) was compatible, with a moderate yield because of steric hindrance. Substrates bearing boronic ester (3i), halogens (3k–3n), and carboxylic ester (3o) functionalities were tolerated well, affording good to excellent yields, which are of utmost value for further structural modifications. 1-Naphth and 2-naphth oxime chlorides were also amenable to this reaction, providing good yields (3o and 3p). It is noted that alkenyl (3q and 3r) and alkyl (3s) oxime chlorides were good substrates for this transformation as well. To our delight, 1,1-dibromoformaldoxime and ethyl-2-chloro-2-(hydroxyimino)acetate as substrates could also successfully furnish the corresponding products 3t and 3u. Furthermore, various B-ethynyl-2,1-borazaronaphthalenes were investigated for 5-(2,1-borazaronaphthyl) isoxazoles (3v–3af). Substituents at the different (4-, 5-, 6-, 7- and 8-) positions of B-ethynyl-2,1-borazaronaphthalenes had minimal influence on the reactivity (3v–3ad), which also shows that the electronic properties of substituents had little effect on the reaction. Notably, N-substituted B-ethynyl-2,1-borazaronaphthalenes (3ae and 3af) were efficient substrates for this regioselective [3 + 2] cycloaddition. Internal alkynes, such as B-(hex-1-yn-1-yl)-1,2-azaborines, do not react under our standard conditions.
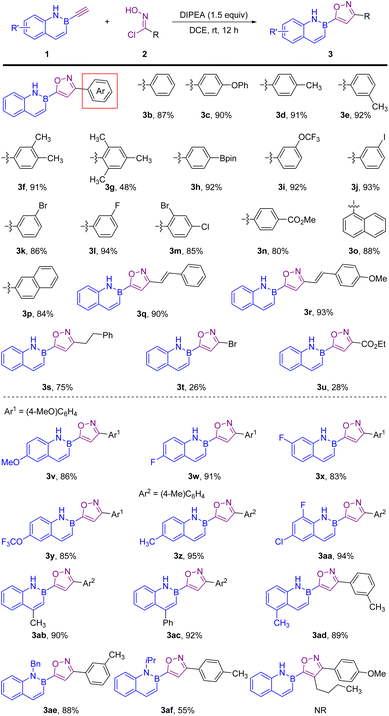 |
| Scheme 2 Substrate scope of regioselective [3 + 2] cycloaddition for 3,5-disubstituted isoxazoles. Reaction conditions: 1 (0.2 mmol, 1 equiv.), 2 (1.5 equiv.), and DIPEA (1.5 equiv.) in 2 mL of DCE at rt for 12 h; isolated yield. | |
We next examined the substrate scope of this regioselective [3 + 2] cycloaddition for 3,4-disubstituted isoxazoles, and we discovered that the Ru-catalyzed process gave a high level of regioselectivity with trace 3,5-disubstituted regioisomers (Scheme 3). Obviously, the electronic properties and the positions of the substituents on the aromatic ring of the oxime chlorides exerted little effect on the reactivity (4b–4j). The structure of 4i was determined by X-ray crystallographic analysis (CCDC 2202558†). Similarly, alkenyl chlorides were also suitable substrates for this transformation (4k). This Ru-catalyzed reaction worked well with different B-ethynyl-2,1-borazaronaphthalenes, producing the desired 3,4-disubstituted products in good to excellent yields. In addition, subsequent efforts expanded the scope of B-ethynyl-1,2-azaborines to B-ethynyl-9,10-phenanthrene 5, providing the corresponding 3,5-disubstituted product 6 (Scheme 4A) and 3,4-disubstituted product 7 (Scheme 4B) in 50 and 80% yield, respectively.
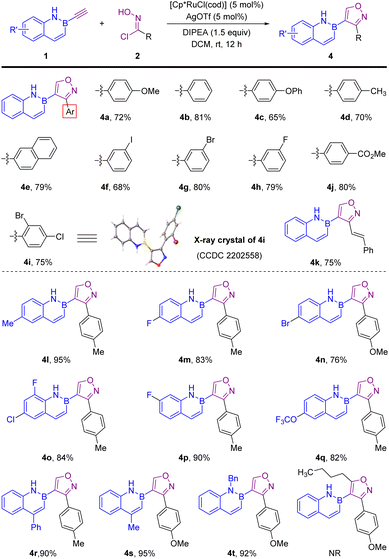 |
| Scheme 3 Substrate scope of regioselective [3 + 2] cycloaddition for 3,4-disubstituted isoxazoles. Reaction conditions: 1 (0.2 mmol, 1 equiv.), 2 (1.2 equiv.), [Cp*RuCl(cod)] (5 mol%), AgOTf (5 mol%), and DIPEA (1.2 equiv.) in 2 mL of DCM at rt for 12 h; isolated yield. | |
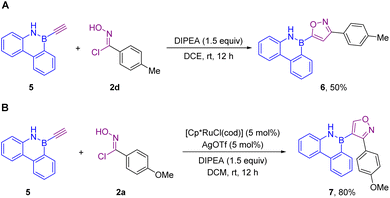 |
| Scheme 4 Regioselective [3 + 2] cycloaddition with B-ethynyl-9,10-phenanthrene. | |
This regioselective [3 + 2] cycloaddition could be easily scaled up to 5 mmol under the standard conditions with the desired 5-(2,1-azaborine) substituted isoxazole 3e in 92% yield (Scheme 5A). In addition, product 3e was smoothly iodinated to give 8 with excellent regioselectivity,10 followed by Sonogashira cross-coupling, Negishi cross-coupling, and reductive coupling11 to obtain structurally diverse products 9, 10, and 11 (Scheme 5B). It was noted that product 3u was easily transformed to the isoxazole amide 12 (Scheme 5C), which is the core structure of many bioactive molecules.12
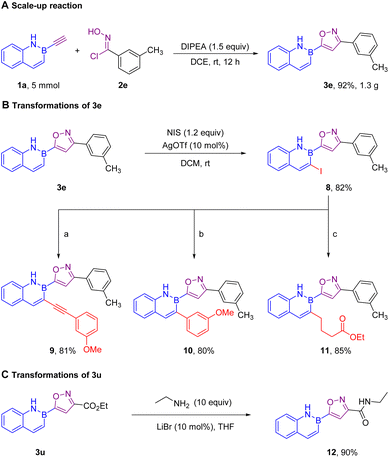 |
| Scheme 5 Scale-up reaction and synthetic transformations. Reaction conditions: (a) (3-MeO)C6H4C CH (3 equiv.), CuI (5 mol%), PdCl2(PPh3) (5 mol%), Et3N/THF = 2 : 1, rt, 12 h. (b) (3-MeO)C6H4ZnBr (1.6 equiv.), Pd2(dba)3 (2 mol%), PCy3 (8 mol%), NMI (1.2 equiv.), THF/NMP = 1 : 1, rt, 16 h. (c) alkyl iodide (1.2 equiv.), NiCl2·glyme (10 mol%), 4,4′-dimethyl-2,2′-bipyridyl (10 mol%), NaBF4 (0.5 equiv.), Mn (2 equiv.), 4-ethylpyridine (1 equiv.), cyclohexane/DMA = 3 : 1, 40 °C, 16 h. | |
Conclusions
In conclusion, we have developed a new class of terminal alkyne reagents, B-ethynyl-1,2-azaborines, and then developed the regioselective [3 + 2] cycloadditions of nitrile oxides with them to access 5- and 4-(2,1-azaborine) substituted isoxazoles. Features such as readily available materials, mild reaction conditions, a broad substrate scope, high functional group tolerance, and excellent regioselectivity illustrate the use of this method.
Conflicts of interest
There are no conflicts to declare.
Acknowledgements
Financial support from the National Natural Science Foundation of China (21931013, 22001038), the Natural Science Foundation of Fujian Province (2022J02009, 2022J05016), Fuzhou University (510578), and the Open Research Fund of School of Chemistry and Chemical Engineering, Henan Normal University is gratefully acknowledged.
References
-
(a) P. Anand and B. Singh, Pyrrolo-isoxazole: A Key Molecule with Diverse Biological Actions, Mini-Rev. Med. Chem., 2014, 14, 623–627 CrossRef CAS PubMed;
(b) G. C. Arya, K. Kaur and V. Jaitak, Isoxazole derivatives as anticancer agent: A review on synthetic strategies, mechanism of action and SAR studies, Eur. J. Med. Chem., 2021, 221, 113511 CrossRef CAS;
(c) A. M. Barmade, R. P. Murumkar, K. M. Sharma and R. M. Yadav, Medicinal Chemistry Perspective of Fused Isoxazole Derivatives, Curr. Top. Med. Chem., 2016, 16, 2863–2883 CrossRef;
(d) A. Sysak and B. Obmińska-Mrukowicz, Isoxazole ring as a useful scaffold in a search for new therapeutic agents, Eur. J. Med. Chem., 2017, 137, 292–309 CrossRef CAS PubMed;
(e) J. Zhu, J. Mo, H.-Z. Lin, Y. Chen and H.-P. Sun, The recent progress of isoxazole in medicinal chemistry, Bioorg. Med. Chem. Lett., 2018, 26, 3065–3075 CrossRef CAS PubMed.
-
(a) S. Das and K. Chanda, An overview of metal-free synthetic routes to isoxazoles: the privileged scaffold, RSC Adv., 2021, 11, 32680–32705 RSC;
(b) X. D. Duc and C. V. Dung, Recent Progress in the Synthesis of Isoxazoles, Curr. Org. Chem., 2021, 25, 2938–2989 CrossRef;
(c) J. Li, Z. Lin, W. Wu and H. Jiang, Recent advances in metal catalyzed or mediated cyclization/functionalization of alkynes to construct isoxazoles, Org. Chem. Front., 2020, 7, 2325–2348 RSC;
(d) T. Morita, S. Yugandar, S. Fuse and H. Nakamura, Recent progresses in the synthesis of functionalized isoxazoles, Tetrahedron Lett., 2018, 59, 1159–1171 CrossRef CAS.
-
(a) J. A. Burkhard, B. H. Tchitchanov and E. M. Carreira, Cascade Formation of Isoxazoles: Facile Base-Mediated Rearrangement of Substituted Oxetanes, Angew. Chem., Int. Ed., 2011, 50, 5379–5382 CrossRef CAS;
(b) C. Chen and S. Cui, Iterative Assembly of Nitrile Oxides and Ynamides: Synthesis of Isoxazoles and Pyrroles, J. Org. Chem., 2019, 84, 12157–12164 CrossRef CAS;
(c) Y. Chen, L. Li, X. He and Z. Li, Four-Component Reactions for the Synthesis of Perfluoroalkyl Isoxazoles, ACS Catal., 2019, 9, 9098–9102 CrossRef CAS;
(d) Y. Chen, D. Zhang, M. Sun and Z. Ding, Divergent synthesis of isoxazoles and 6H-1,2-oxazines via hypervalent iodine-mediated intramolecular oxygenation of alkenes, New J. Chem., 2022, 46, 5663–5667 RSC;
(e) M. Duan, G. Hou, Y. Zhao, C. Zhu and C. Song, Synthesis of Isoxazoles via One-Pot Oxidation/Cyclization Sequence from Propargylamines, J. Org. Chem., 2022, 87, 11222–11225 CrossRef CAS;
(f) X. Guo, G. Xu, L. Zhou, H. Yan, X.-Q. Hao and Q. Wang, Synthesis and application of α-carbonyl nitrile oxides, Org. Chem. Front., 2020, 7, 2467–2473 RSC;
(g) C. Lei, Z. Gao, X. Shao, X. Xu and Z. Li, Synthesis of Trisubstituted Isoxazoles from Nitroenamines and Aromatic Aldehydes, Chin. J. Chem., 2017, 35, 1517–1521 CrossRef CAS;
(h) J. Li, Z. Lin, D. He, W. Wu and H. Jiang, Palladium-Catalyzed Sequential Cyclization/Functionalization of Oxime Ethers with Unactivated Vinyl Ethers for Tunable Assembly of Structurally Diverse Isoxazoles, Chin. J. Chem., 2021, 39, 3285–3291 CrossRef CAS;
(i) Y. Lin, K. Zhang, M. Gao, Z. Jiang, J. Liu, Y. Ma, H. Wang, Q. Tan, J. Xiao and B. Xu, Copper nitrate-mediated synthesis of 3-aryl isoxazolines and isoxazoles from olefinic azlactones, Org. Biomol. Chem., 2019, 17, 5509–5513 RSC;
(j) K. N. Tu, J. J. Hirner and S. A. Blum, Oxyboration with and without a Catalyst: Borylated Isoxazoles via B–O σ-Bond Addition, Org. Lett., 2016, 18, 480–483 CrossRef CAS PubMed;
(k) W. Wu, Q. Chen, Y. Tian, Y. Xu, Y. Huang, Y. You and Z. Weng, Synthesis of polysubstituted 5-trifluoromethyl isoxazoles via denitrogenative cyclization of vinyl azides with trifluoroacetic anhydride, Org. Chem. Front., 2020, 7, 1878–1883 RSC;
(l) Z. Yu, D. Zhang, X. Li, B. Zhang, Z. Yang, Y. Qian and Y. Du, Synthesis of 4-Chalcogenylated Isoxazoles Mediated by PhICl2 and Diorganyl Disulfides/Diselenides, Asian J. Org. Chem., 2021, 10, 3015–3019 CrossRef CAS;
(m) M. Zhao, W. He, L.-H. Zou, D. Wang, T.-Y. Sun and X.-F. Xia, Iron-catalyzed hydrogen atom transfer induced cyclization of 1,6-enynes for the synthesis of ketoximes: a combined experimental and computational study, Org. Chem. Front., 2021, 8, 643–652 RSC.
-
(a) A. Bhattacharjee, G. H. M. Davies, B. Saeednia, S. R. Wisniewski and G. A. Molander, Selectivity in the Elaboration of Bicyclic Borazarenes, Adv. Synth. Catal., 2021, 363, 2256–2273 CrossRef CAS;
(b) Z. X. Giustra and S.-Y. Liu, The State of the Art in Azaborine Chemistry: New Synthetic Methods and Applications, J. Am. Chem. Soc., 2018, 140, 1184–1194 CrossRef CAS PubMed;
(c) C. R. McConnell and S.-Y. Liu, Late-stage functionalization of BN-heterocycles, Chem. Soc. Rev., 2019, 48, 3436–3453 RSC;
(d) F.-D. Zhuang, J.-Y. Wang and J. Pei, Recent progress and challenges in the synthesis of boron-nitrogen fused polycyclic aromatic hydrocarbons, Sci. Sin.: Chim., 2020, 50, 1205–1216 Search PubMed;
(e) G. Bélanger-Chabot, H. Braunschweig and D. K. Roy, Recent Developments in Azaborinine Chemistry, Eur. J. Org. Chem., 2017, 4353–4368 CrossRef;
(f) P. G. Campbell, A. J. V. Marwitz and S.-Y. Liu, Recent Advances in Azaborine Chemistry, Angew. Chem., Int. Ed., 2012, 51, 6074–6092 CrossRef CAS.
- G. H. M. Davies, Z.-Z. Zhou, M. Jouffroy and G. A. Molander, Method for Accessing Nitrogen-Containing, B-Heteroaryl-Substituted 2,1-Borazaronaphthalenes, J. Org. Chem., 2017, 82, 549–555 CrossRef CAS.
-
(a) F. Himo, T. Lovell, R. Hilgraf, V. V. Rostovtsev, L. Noodleman, K. B. Sharpless and V. V. Fokin, Copper(I)-Catalyzed Synthesis of Azoles. DFT Study Predicts Unprecedented Reactivity and Intermediates, J. Am. Chem. Soc., 2005, 127, 210–216 CrossRef CAS PubMed;
(b) W. Chen, J. Zhang, B. Wang, Z. Zhao, X. Wang and Y. Hu, Tandem Synthesis of 3-Chloro-4-iodoisoxazoles from 1-Copper(I) Alkynes, Dichloroformaldoxime, and Molecular Iodine, J. Org. Chem., 2015, 80, 2413–2417 CrossRef CAS;
(c) C. Kesornpun, T. Aree, C. Mahidol, S. Ruchirawat and P. Kittakoop, Water-Assisted Nitrile Oxide Cycloadditions: Synthesis of Isoxazoles and Stereoselective Syntheses of Isoxazolines and 1,2,4-Oxadiazoles, Angew. Chem., Int. Ed., 2016, 55, 3997–4001 CrossRef CAS PubMed;
(d) S. Okusu, E. Tokunaga and N. Shibata, Difluoromethylation of Terminal Alkynes by Fluoroform, Org. Lett., 2015, 17, 3802–3805 CrossRef CAS PubMed;
(e) J.-S. Poh, C. García-Ruiz, A. Zúñiga, F. Meroni, D. C. Blakemore, D. L. Browne and S. V. Ley, Synthesis of trifluoromethylated isoxazoles and their elaboration through inter- and intra-molecular C–H arylation, Org. Biomol. Chem., 2016, 14, 5983–5991 RSC;
(f) R. M. Reja, S. Sunny and H. N. Gopi, Chemoselective Nitrile Oxide–Alkyne 1,3-Dipolar Cycloaddition Reactions from Nitroalkane-Tethered Peptides, Org. Lett., 2017, 19, 3572–3575 CrossRef CAS;
(g) B. C. Sanders, F. Friscourt, P. A. Ledin, N. E. Mbua, S. Arumugam, J. Guo, T. J. Boltje, V. V. Popik and G.-J. Boons, Metal-Free Sequential [3 +
2]-Dipolar Cycloadditions using Cyclooctynes and 1,3-Dipoles of Different Reactivity, J. Am. Chem. Soc., 2011, 133, 949–957 CrossRef CAS PubMed;
(h) X. Ma, W. Qiu, L. Liu, X. Zhang, J. Awad, J. Evans and W. Zhang, Synthesis of tetrahydropyrrolothiazoles through one-pot and four-component N,S-acetalation and decarboxylative [3 + 2] cycloaddition, Green Synth. Catal., 2021, 2, 74–77 CrossRef;
(i) B. A. Chalyk, A. S. Sosedko, D. M. Volochnyuk, A. A. Tolmachev, K. S. Gavrilenko, O. S. Liashuk and O. O. Grygorenko, Regioselective synthesis of isoxazole and 1,2,4-oxadiazole-derived phosphonates via [3 + 2] cycloaddition, Org. Biomol. Chem., 2018, 16, 9152–9164 RSC;
(j) P. Dai, X. Tan, Q. Luo, X. Yu, S. Zhang, F. Liu and W.-H. Zhang, Synthesis of 3-Acyl-isoxazoles and Δ2-Isoxazolines from Methyl Ketones, Alkynes or Alkenes, and tert-Butyl Nitrite via a Csp3–H Radical Functionalization/Cycloaddition Cascade, Org. Lett., 2019, 21, 5096–5100 CrossRef CAS PubMed;
(k) L. De Angelis, A. M. Crawford, Y.-L. Su, D. Wherritt, H. Arman and M. P. Doyle, Catalyst-Free Formation of Nitrile Oxides and Their Further Transformations to Diverse Heterocycles, Org. Lett., 2021, 23, 925–929 CrossRef CAS PubMed;
(l) Q. Feng, H. Huang and J. Sun, Ru-Catalyzed [3 + 2] Cycloaddition of Nitrile Oxides and Electron-Rich Alkynes with Reversed Regioselectivity, Org. Lett., 2021, 23, 2431–2436 CrossRef PubMed.
-
(a) M. W. Davies, R. A. J. Wybrow, C. N. Johnson and J. P. A. Harrity, A regioselective cycloaddition route to isoxazoleboronic esters, Chem. Commun., 2001, 1558–1559 RSC;
(b) J. A. Sáez, M. Arnó and L. R. Domingo, A DFT study for the regioselective 1,3-dipolar cycloadditions of nitrile N-oxides toward alkynylboronates, Tetrahedron, 2003, 59, 9167–9171 CrossRef.
- I. Valencia, P. García-García, D. Sucunza, F. Mendicuti and J. J. Vaquero, 1,10a-Dihydro-1-aza-10a-boraphenanthrene and 6a,7-Dihydro-7-aza-6a-boratetraphene: Two New Fluorescent BN-PAHs, J. Org. Chem., 2021, 86, 16259–16267 CrossRef CAS PubMed.
- S. Grecian and V. V. Fokin, Ruthenium-Catalyzed Cycloaddition of Nitrile Oxides and Alkynes: Practical Synthesis of Isoxazoles, Angew. Chem., Int. Ed., 2008, 47, 8285–8287 CrossRef CAS.
- D. Tian, W. Zhang, G. Shi, S. Luo, Y. Chen, W. Chen, H. Huang, S. Xing and B. Zhu, Synthesis, structure and properties of semi-internally BN-substituted annulated thiophenes, Org. Chem. Front., 2021, 8, 4124–4130 RSC.
- G. A. Molander, S. R. Wisniewski and K. M. Traister, Reductive Cross-Coupling of 3-Bromo-2,1-borazaronaphthalenes with Alkyl Iodides, Org. Lett., 2014, 16, 3692–3695 CrossRef CAS.
-
(a) J. Sun, C. Lin, X. Qin, X. Dong, Z. Tu, F. Tang, C. Chen and J. Zhang, Synthesis and biological evaluation of 3,5-disubstituted-4-alkynylisoxozales as a novel class of HSP90 inhibitors, Bioorg. Med. Chem. Lett., 2015, 25, 3129–3134 CrossRef CAS PubMed;
(b) M. R. Jensen, J. Schoepfer, T. Radimerski, A. Massey, C. T. Guy, J. Brueggen, C. Quadt, A. Buckler, R. Cozens, M. J. Drysdale, C. Garcia-Echeverria and P. Chène, NVP-AUY922: a small molecule HSP90 inhibitor with potent antitumor activity in preclinical breast cancer models, Breast Cancer Res., 2008, 10, R33 CrossRef.
|
This journal is © the Partner Organisations 2023 |
Click here to see how this site uses Cookies. View our privacy policy here.