DOI:
10.1039/D3OB00793F
(Communication)
Org. Biomol. Chem., 2023,
21, 5737-5741
Two-component symmetrical diarylation of ynamides†
Received
21st May 2023
, Accepted 28th June 2023
First published on 28th June 2023
Abstract
The present study describes a method for the dicarbofunctionalization of ynamide via a palladium-catalyzed two-component diarylation with aryl boronic acids. The reaction involves a consecutive transmetalation of the aryl boronic acids with a Pd(II)-complex making the transformation stereoselective. Importantly, the reaction proceeds under mild conditions and tolerates a broad range of functional groups. Control experiments validate the role of the oxidant (useful for catalyst regeneration) in the reaction mechanism.
Introduction
The ubiquitous presence of tetrasubstituted and π-extended olefins in a wide range of drugs, natural products,1–5 and their derivatives, as well as in other biologically active substances,6,7 renders them a topic of great significance. Moreover, their role in materials research cannot be ignored, as the presence of double bonds can impart favourable physical, structural, and electronic properties. This feature makes them particularly appealing for use in electron-transport materials and light-emitting diodes.8–10 A promising approach for accessing polyfunctionalized alkene derivatives involves the transition-metal (TM) catalysed one-pot dicarbofunctionalization of alkynes.11–22 On the other hand, ynamides, a special class of N-substituted alkynes, are of particular interest due to their unique reactivity arising from the polarization of N-lone pair electrons.23–35 The intermediates involved in this process, such as keteniminium ions, and metal carbenoids, are crucial for metal-catalyzed dicarbofunctionalization reactions; the process allows making tetrasubstituted enamides.36 Given the importance of such enamides, development of pathways for the synthesis of substituted enamides attracts attention. The Lam group has developed a chelation-aided dicarbon-functionalization of ynamides using Rh-catalysis in the presence of dialkyl zinc and an electrophile.37,38 The Marek and Hou groups independently revealed the formation of enamides through a syn-alkyl-cupration of ynamides in 2009 and 2015, respectively.39,40 A recent study from our group has showcased the 1,2-diarylation and aryl–alkenylation of ynamides; the transformation involves a syn-1,3-aryl migration in keteniminium species.23,30 Nevertheless, Yoshimura and co-workers have demonstrated an imperative syn-1,2-aryl-palladation of ynamide; this process offers an efficient entry to construct fully substituted-enamides (Fig. 1a).41
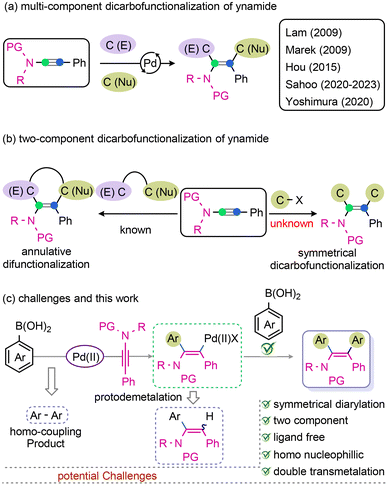 |
| Fig. 1 Background and this work. | |
It is imperative to note that a significant focus on the three-component unsymmetrical dicarbofunctionalization of ynamides has been realized in recent years. While the two-component difunctionalization of ynamides are mostly referred to annulation42 (Fig. 1b, left) or hydroarylation products leading to cyclic and tri-substituted enamides, respectively.43–45 Meanwhile, it is worth emphasizing that the homonucleophilic symmetrical dicarbofunctionalization of ynamide remains unexplored (Fig. 1b, right).
The current investigation of symmetrical diarylation of ynamide is focused on a efficient transmetalation of vinyl palladium(II) complex, obtained in situ via 1,2-syn-aryl palladation of ynamide, with second molecule of aryl-nucleophile. Prior studies have established that transmetalation of vinyl palladium(II) intermediate is rather cumbersome. Mostly, the vinyl palladium(II) species are prone to protodemetalation leading to hydroarylation products.43–45 The aim of this study is to assess the feasibility of utilizing a homo-nucleophilic two-component symmetrical diarylation of ynamides with aryl boronic acid under ligand-free conditions (Fig. 1c). Notably, the reaction pathway involves two sequential transmetalation steps of Pd(II) species (Fig. 1c).
Results and discussion
Our investigation into the symmetrical diarylation of ynamide was commenced by conducting a reaction between N-benzyl-4-methyl-N-(phenylethynyl)benzenesulfonamide (1a) and p-methoxyphenylboronic acid (2a) under the influence of Pd(OAc)2, oxidant, and a base. By subjecting a range of oxidants, bases, and solvents to extensive screening, we successfully optimized the reaction conditions. Specifically, we discovered that a 77% yield of 3a (entry a, Scheme 1) could be achieved through the use of 10 mol% Pd(OAc)2, Ag2CO3 (1.3 equiv.), KH2PO4 (3.0 equiv.) in CH3CN at 60 °C for 6 h. In contrast, alternative oxidants, including Ag2SO4 and PhI(OAc)2, were found to be less effective (entries b and c, Scheme 1), while K2S2O8 proved incapable of yielding 3a (entry d, Scheme 1). Notably, bases NaHCO3 and Na2CO3 exhibited sufficient efficacy to produce 70% 3a (entries e and f, Scheme 1). While the use of solvents, such as toluene, 1,4-dioxane, and THF did not yield 3a (entries g–i, Scheme 1). Furthermore, varying the solvent dilution proved to have a negative impact on yield, as increasing or decreasing the solvent concentration resulted in lower yields (53–65%, entries j–l, Scheme 1).
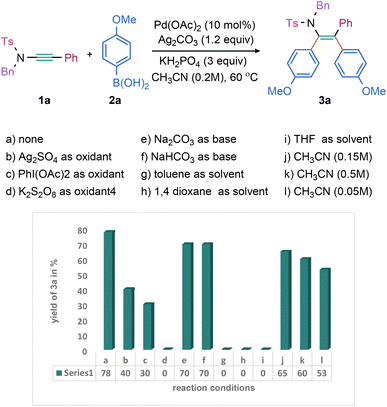 |
| Scheme 1 Optimization graph. All reactions are carried out in 0.3 mmol scale. 1a (0.3 mmol), 2a (0.9 mmol), Pd(OAc)2 (0.03 mmol), oxidant (0.36 mmol), base (0.9 mmol) in 1.5 mL solvent. All isolated yields are provided. | |
Next, the scope and limitations of a reaction with regard to the use of different ynamides were explored (Scheme 2). The simple N(Ts)(Bn)-ynamides having phenyl (1a) and the electron-rich p-tolyl (1b) in alkyne terminus were independently reacted with 2a to yield 3a and 3b in 77% and 53% yield, respectively (Scheme 2). Despite the ynamide bulkiness, the reaction of TsN-[Me]-1-naphthyl ynamide (1c) with 2a was even successful producing the desired diarylated enamide 3c (61%; Scheme 2). Notably, the 3-thienyl bearing tetra-substituted enamide 3d was synthesized from the respective 3-thienyl-substituted ynamide in 48% yield (Scheme 2). In addition, the ynamides with various N-sulfonyl protecting groups, such as BnN-[SO2-1-naphthyl (1e)/SO2C6H4–p-Ph (1f)/SO2C6H4–p-COMe (1g)/SO2C6H4–m-F (1h)/SO2CH2Cl (1i)/SO2–Et (1j)/SO2–Bn (1k)], were well tolerated under the optimized conditions, yielding the respective tetrasubstituted enamides 3e–k (49–70%; Scheme 2). Furthermore, compounds 3l (58%) and 3m (46%) were obtained from electron-rich piperidine and 2-thienyl-based N-sulfonyl-protected ynamides 1l and 1m, respectively (Scheme 2). The structure 3d was unambiguously established by X-ray crystallography.
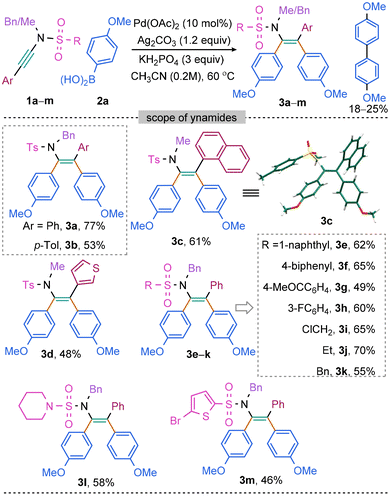 |
| Scheme 2 Scope of ynamide. All reactions are carried out in 0.3 mmol scale. 1a–m (0.3 mmol), 2a (0.9 mmol), Pd(OAc)2 (0.03 mmol), Ag2CO3 (0.36 mmol), KH2PO4 (0.9 mmol) in 1.5 mL CH3CN. All isolated yields are provided. The homocoupling product was obtained in 18–25% yield. | |
Subsequently, the scope of various aryl boronic acids has been investigated (Scheme 3). The 2-oxazolidinone protected ynamide 1n was selected as the model substrate since its reactivity has rarely been showcased in the previous reports of diarylation of ynamides.46 The reaction of 1n with p-tolyl boronic acid 2b under optimized condition for sulfonyl ynamides (entry a, Scheme 1) provided 4a in 20% yield. Subsequent efforts were, therefore, undertaken making the difunctionalization of 1n with 2b effective. Through extensive screening of bases and solvents, a new set of optimized conditions were established [2b (5.0 equiv.), Pd(OAc)2 (10 mol%), Ag2CO3 (2.0 equiv.), K3PO4 (3.0 equiv.), in CH3CN
:
DMF (1
:
1) at room temperature for 6 h]; 4a was isolated in 59% yield (entry a, Scheme 3). Notably, decreasing the stoichiometric quantity of 2a resulted in a further reduction of 4a yield (entry b, Scheme 3). Additionally, various bases, including K3PO4, KF, and a mixture of bases (K3PO4
:
Na2CO3 and K3PO4
:
KH2PO4), were screened (entries c–e, Scheme 3); all these efforts did not significantly improve the product yield of 4a. However, other mixtures of solvents, DME and THF with CH3CN, instead of DMF with CH3CN, were found to be comparatively less effective (entries f and g, Scheme 3). The reaction was unproductive when conducted in the presence of bidentate ligand or at an elevated temperature (entries h–j, Scheme 3).
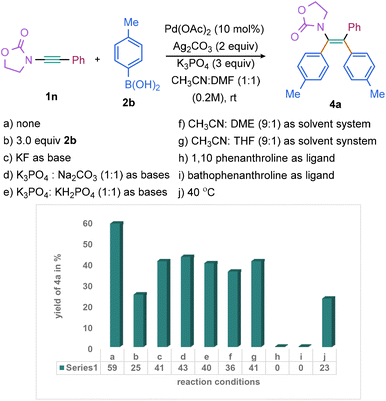 |
| Scheme 3 Optimization graph. All reactions are carried out on a 0.3 mmol scale. 1n (0.3 mmol), 2b (1.5 mmol), Pd(OAc)2 (0.03 mmol), oxidant (0.6 mmol), base (0.9 mmol) in 1.5 mL solvent. All isolated yields are provided. | |
Next, the feasibility of the catalytic conditions was examined for the symmetrical diarylation of 2-oxazolidinone ynamide 1n while exploring the potential of distinct aryl-boronic acid coupling partners. It is noteworthy that several aryl boronic acids were compatible resulting in the successful synthesis of the corresponding target products 4a–j in moderate to low yields (Scheme 4). The reaction of para-substituted (Me, iPr, and F3CO) electron-rich aryl boronic acids 2b–d with 1n yielded the respective tetra-substituted enamides 4a–c in moderate yields. Likewise, compounds 4d and 4e were synthesized independently from the reaction of 1n with 3,4-dimethoxy 2e and 3-methyl 2f phenylboronic acids, respectively. While the reaction of electron-deficient aryl boronic acids 2g and 2h (containing NO2 and CO2Et at the meta-position) separately with 1n produced 4f and 4g in 26% and 43% yield, respectively. Notably, the aryl boronic acids harboring labile and even oxidizable halo groups [m-Cl (2i), p-Cl (2j), p-Br (2k)] were able to withstand under the Pd-catalytic system and the desired products 4h–j were made albeit in poor yield; in no cases the reaction did not produce the dehalogenated product showcasing the robustness of the catalytic conditions.
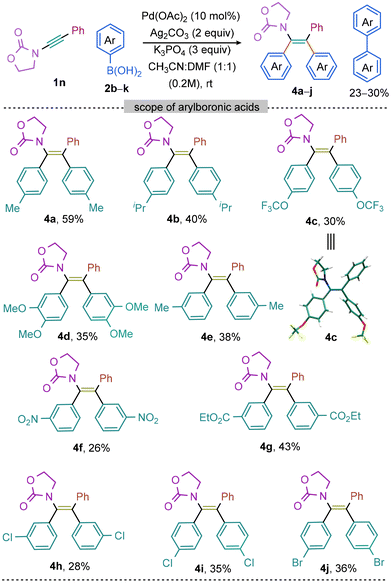 |
| Scheme 4 Scope of ynamide. All reactions are carried out in 0.3 mmol scale. 1n (0.3 mmol), 2b–k (1.5 mmol), Pd(OAc)2 (0.03 mmol), Ag2CO3 (0.6 mmol), K3PO4 (0.9 mmol) in 1.5 mL CH3CN : DMF (1 : 1). All isolated yields are provided. The respective homocoupling biaryls are obtained in 23–30% yield. | |
To gain insights into the role of oxidant, the reaction of 1a and 2a was carried out in the absence of oxidant by removing the dissolved oxygen from the solvent through freeze–thaw-cycle; 3a was obtained in 7.9% yield (Scheme 5a) [the diminished yield is presumably due the unavailability of the active Pd(II) catalyst that is being generated in the presence of oxidant after one catalytic cycle]. Moreover, the reaction in the presence of oxygen instead of Ag2CO3 yielded 16% 3a (Scheme 5b). These observations validate the role of oxidant in regenerating the active Pd(II) catalyst after reductive elimination.
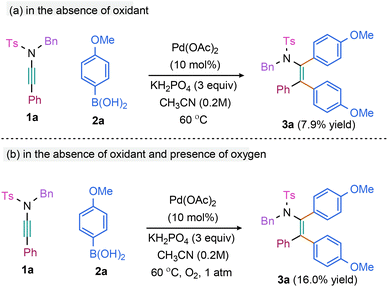 |
| Scheme 5 Control experiments. | |
Based on the control experiments and in accordance with the established mechanistic cycle, the transmetalation of aryl boronic acid with Pd(II) species at first delivers complex A. Next, migratory insertion of A with ynamide 1 led to complex B. The migratory insertion results in the generation of a carbon–carbon bond at the α-position and the carbon–Pd bond at the β-position of the ynamide; the step is regio as well syn-selective. Previous findings on the hydroarylation of ynamide validates that the preferential formation of complex B can be attributed to the steric repulsion of aryl-Pd complex with the sulfonyl-amide and combined with the electron-polarizing effect of the amido group. Following this, the second transmetalation of complex B with an additional equivalent of boronic acid 2 delivers complex E with retention of stereochemistry. Finally, reductive elimination of E affords product 3 and produces the Pd(0). Concomitantly, the regeneration of Pd(II) is facilitated in the presence of an oxidant. While the homocoupling of complex A with aryl boronic acid yields the bi-aryl as a by-product albeit in less amount but the process is inevitable (Fig. 2).
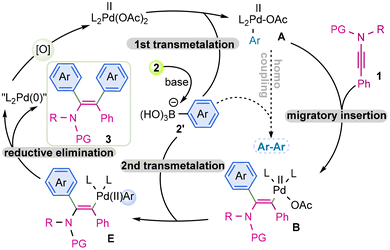 |
| Fig. 2 Plausible mechanism. | |
Conclusions
In summary, we have successfully devised a highly discriminating method for the selective Pd-catalyzed diarylation of unsymmetrical electron-rich-alkynes, utilizing readily available aryl boronic acids as a nucleophilic aryl source and silver carbonate as an oxidant. Notably, our protocol exhibits considerable substrate versatility. Control experiments validate the role of oxidant in facilitating double transmetalation through the regeneration of active Pd(II) catalyst.
Conflicts of interest
There are no conflicts of interest to declare.
Acknowledgements
This research was supported by the SERB-India (grant no.: CRG-2019-1802). We thank the University of Hyderabad (UOH-IoE: UPE-CAS and PURSE-FIST) for its overall facility. AS thanks UGC, India, and SD thanks CSIR, India, for the fellowship. We thank Manash P. Gogoi for his help.
References
- V. Saini, M. O'Dair and M. S. Sigman, J. Am. Chem. Soc., 2015, 137(2), 608 CrossRef CAS PubMed.
- R. B. Williams, A. Norris, C. Slebodnick, J. Merola, J. S. Miller, R. Andriantsiferana, V. E. Rasamison and D. G. I. Kingston, J. Nat. Prod., 2005, 68, 1371 CrossRef CAS PubMed.
- C. Steinborn, R. E. Wildermuth, D. M. Barber and T. Magauer, Angew. Chem., Int. Ed., 2020, 59, 17282 CrossRef CAS PubMed.
- P. Yuan, C. K. G. Gerlinger, J. Herberger and T. Gaich, J. Am. Chem. Soc., 2021, 143, 11934 CrossRef CAS PubMed.
- W.-S. Zhang, D.-W. Ji, Y. Li, X.-X. Zhang, C.-Y. Zhao, Y.-C. Hu and Q.-A. Chen, ACS Catal., 2022, 12, 2158 CrossRef CAS.
- A. S. Levenson and V. C. Jordan, Eur. J. Cancer, 1999, 35, 1628 CrossRef CAS PubMed.
- P. Prasit, Z. Wang, C. Brideau, C. C. Chan, S. Charleson, W. Cromlish, D. Ethier, J. F. Evans, A. W. F. Hutchinson, J. Y. Gauthier, R. Gordon, J. Guay, M. Gresser, S. Kargman, B. Kennedy, Y. Leblanc, S. Leger, J. Mancini, G. P. O'Neill, M. Ouellet, M. D. Percival, H. Perrier, D. Riendeau, I. Rodger, P. Tagari, M. Therien, P. Vickers, E. Wong, L. J. Xu, R. N. Young, R. Zamboni, S. Boyce, N. Rupniak, M. Forrest, D. Visco and D. Patrick, Bioorg. Med. Chem. Lett., 1999, 9, 1773 CrossRef CAS PubMed.
- N. S. Ahmed, N. H. Elghazawy, A. K. ElHady, M. Engel, R. W. Hartmann and A. H. Abadi, Eur. J. Med. Chem., 2016, 112, 171 CrossRef CAS PubMed.
- D. Joly, D. Tondelier, V. Deborde, B. Geffroy, M. Hissler and R. Réau, New J. Chem., 2010, 34, 1603 RSC.
- G.-Y. Ran, M. Gong, J.-F. Yue, X.-X. Yang, S.-L. Zhou, W. Du and Y.-C. Chen, Org. Lett., 2017, 19, 1874 CrossRef CAS PubMed.
- A. Düfert and D. B. Werz, Chem. – Eur. J., 2016, 22, 16718 CrossRef PubMed.
- Z. Li, A. G. Domínguez and C. Nevado, Angew. Chem., Int. Ed., 2016, 55, 6938 CrossRef CAS PubMed.
- Y.-Z. Zhan, H. Menga and W. Shu, Chem. Sci., 2022, 13, 4930 RSC.
- J. Liu, D. Liu, W. Nie, H. Yu and J. Sh, Org. Chem. Front., 2022, 9, 163 RSC.
- C. Zhu, H. Yue and M. Rueping, Nat. Commun., 2022, 13, 3240 CrossRef CAS PubMed.
- L. Guo, F. Song, S. Zhu, H. Li and L. Chu, Nat. Commun., 2018, 9, 4543 CrossRef PubMed.
- S. Dutta, S. Shandilya, S. Yang, M. P. Gogoi, V. Gandon and A. K. Sahoo, Nat. Commun., 2022, 13, 1360 CrossRef CAS PubMed.
- J.-B. Tang, J.-Q. Bian, Y.-S. Zhang, Y.-F. Cheng, H.-T. Wen, Z.-L. Yu, Z.-L. Li, Q.-S. Gu, G.-Q. Chen and X.-Y. Liu, Org. Lett., 2022, 24, 2536 CrossRef CAS PubMed.
- S. Maiti and J. H. Rhlee, Chem. Commun., 2021, 57, 11346 RSC.
- Y.-Z. Zhan, N. Xiao and W. Shu, Nat. Commun., 2021, 12, 928 CrossRef CAS PubMed.
- W. Li, S. Yu, J. Li and Y. Zhao, Angew. Chem., Int. Ed., 2020, 59, 14404 CrossRef CAS PubMed.
- S. Dutta and A. K. Sahoo, Angew. Chem., Int. Ed., 2023, 62, e202300610 CrossRef CAS PubMed.
- S. Dutta, S. Yang, R. Vanjari, R. K. Mallick, V. Gandon and A. K. Sahoo, Angew. Chem., Int. Ed., 2020, 59, 10785 CrossRef CAS PubMed.
- P. Yepes, Á. L. Suárez-Sobrino, M. A. Rodríguez and A. Ballesteros, Org. Lett., 2023, 25, 1020 CrossRef CAS PubMed.
- S. Choudhary, Gayyur and N. Ghosh, Eur. J. Org. Chem., 2023, e202201223 CAS.
- B.-H. Zhu, S.-B. Ye, M.-L. Nie, Z.-Y. Xie, Y.-B. Wang, P.-C. Qian, Q. Sun, L.-W. Ye and L. Li, Angew. Chem., Int. Ed., 2023, 62, e202215616 CAS.
- S. Jos, C. Tan, P. Thilmany, A. Saadane, C. Slebodnick, G. Evano and W. L. Santos, Chem. Commun., 2022, 58, 13751 RSC.
- M. R. Mutra and J.-J. Wang, Nat. Commun., 2022, 13, 2345 CrossRef CAS PubMed.
- Y. Liu, M. C. Dietl, C. Han, M. Rudolph, F. Rominger, P. Krämer and A. S. K. Hashmi, Org. Lett., 2022, 24, 7101 CrossRef CAS PubMed.
- R. Vanjari, S. Dutta, S. Yang, V. Gandon and A. K. Sahoo, Org. Lett., 2022, 24, 1524 CrossRef CAS PubMed.
- P. Hansjacob, F. R. Leroux, V. Gandon and M. Donnard, Angew. Chem., Int. Ed., 2022, 61, e202200204 CrossRef CAS PubMed.
- M. P. Gogoi, R. Vanjari, B. Prabagar, S. Yang, S. Dutta, R. K. Mallick, V. Gandon and A. K. Sahoo, Chem. Commun., 2021, 57, 7521 RSC.
- W.-F. Luo, L.-W. Ye, L. Li and P.-C. Qian, Chem. Commun., 2021, 57, 5032 RSC.
- C. You, M. Sakai, C. G. Daniliuc, K. Bergander, S. Yamaguchi and A. Studer, Angew. Chem., Int. Ed., 2021, 60, 21697 CrossRef CAS PubMed.
- S. Shandilya, M. P. Gogoi, S. Dutta and A. K. Sahoo, Chem. Rec., 2021, 21, 4123 CrossRef CAS PubMed.
- R. Vanjari, S. Dutta, M. P. Gogoi, V. Gandon and A. K. Sahoo, Org. Lett., 2018, 20, 8077 CrossRef CAS PubMed.
- B. Gourdet and H. W. Lam, J. Am. Chem. Soc., 2009, 131, 3802 CrossRef CAS PubMed.
- B. Gourdet, M. E. Rudkin, C. A. Watts and H. W. Lam, J. Org. Chem., 2009, 74, 7849 CrossRef CAS PubMed.
- J. P. Das, H. Chechik and I. Marek, Nat. Chem., 2009, 1, 128 CrossRef CAS PubMed.
- M. Takimoto, S. S. Gholap and Z. Hou, Chem. – Eur. J., 2015, 21, 15218 CrossRef CAS PubMed.
- H. Wakamatsu, A. Takahashi, A. Ishii, Y. Kikuchi, M. Sasaki, Y. Saito, Y. Natori and Y. Yoshimura, Org. Lett., 2020, 22, 5299 CrossRef CAS PubMed.
- S. Dutta, R. K. Mallick and A. K. Sahoo, Angew. Chem., Int. Ed., 2023 DOI:10.1002/anie.202300816.
- B. Gourdet, D. L. Smith and H. W. Lam, Tetrahedron, 2010, 66, 6026 CrossRef CAS.
- Y. Yang, L. Wang, F. Zhang and G. Zhu, J. Org. Chem., 2014, 79, 9319 CrossRef CAS PubMed.
- Y. Yang, L. Wang, J. Zhang, Y. Jinb and G. Zhu, Chem. Commun., 2014, 50, 2347 RSC.
- B. Gourdet, M. E. Rudkin, C. A. Watts and H. W. Lam, J. Org. Chem., 2009, 74, 7849 CrossRef CAS PubMed.
|
This journal is © The Royal Society of Chemistry 2023 |
Click here to see how this site uses Cookies. View our privacy policy here.