DOI:
10.1039/D2NJ04481A
(Paper)
New J. Chem., 2023,
47, 92-96
Rational design of an ortho-vinylhydropyridine-assisted amino-fluorophore as a hypochlorite fluorescent probe†
Received
8th September 2022
, Accepted 12th November 2022
First published on 14th November 2022
Abstract
A novel strategy for developing a hypochlorite fluorescent probe by exploiting the ortho-vinylhydropyridine-assisted amido-to-nitroso oxidation reaction is presented. The designed 1,8-naphthalimide-based derivative (1a) is employed to monitor and identify trace amounts of ClO− with the advantage of fast response (within 1.2 seconds, LOD = 36.0 nM), good selectivity, and high sensitivity in solution and living cells.
Introduction
Reactive oxygen species (ROS) play important roles in cell signaling and homeostasis, such as pathogen response, aging, and anti-inflammation regulation.1 Among various ROS, hypochlorite (ClO−) is endogenously produced from the peroxidation reaction between hydrogen peroxide and chloride ions catalyzed by myeloperoxidase (MPO).2 Excessive levels of ClO− in living organisms could lead to a series of diseases, including atherosclerosis (AS), lung injuries, rheumatoid arthritis, and even cancers.3 Thus, the development of novel chemical tools for highly selective detection of hypochlorite is essential.
Among various analytical methodologies for ClO− determination, fluorescent probes have emerged as robust alternatives to detect ClO− and attracted much attention in recent years due to their unique properties like high selectivity, sensitivity, real-time detection, and good compatibility with bio-samples.4 To date, mainly the sensing mechanism employed is the ClO−-mediated oxidation reaction with specific reaction sites, such as Schiff bases, electron-deficient C
C bonds, sulphides, p-methoxyphenol, p-alkoxyaniline, etc.5 Although the mechanism mentioned above to develop fluorescent probes has achieved considerable recognition, most of these probes display low sensitivity, slow response time, poor water solubility, and biocompatibility. Therefore, strategic design of a novel mechanism for the precise detection of ClO− is still a great challenge.
In general, the direct hydrogenation of nitro compounds is the classical strategy for producing amino compounds. Interestingly, the oxidation of amino compound 1 furnishing the nitro derivative 4 proceeds via the important hydroxylamino intermediate 2 and nitroso compound 3 (Scheme 1).6 Although various types of fluorescent probes for hypochlorite detection have been successfully reported, rare examples regarding the amido-to-nitroso oxidation approach have been explored to date.7 Evidence indicates that vinylhydropyridine (a) could also be oxidized to the stable pyridine derivative (b) by the intramolecular 1,3-hydrogen transfer.8 Inspired by these findings, we envisioned introducing a new concept to develop fluorescent chemosensors for ClO− determination based on ortho-vinylhydropyridine-assisted amido-to-nitroso oxidation.
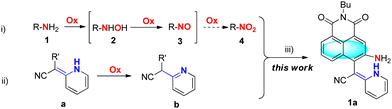 |
| Scheme 1 (i) The classical oxidation route of amino compound 1 to nitro derivative 4; (ii) the vinyl-hydropyridine-containing derivative a and its oxidative product b; (iii) the rational design of ortho-vinylhydropyridine-assisted amino-chemosensor 1a. | |
In this study, we have designed a small molecular fluorescent probe (1a) based on the ClO−-mediated oxidation reaction towards dual potential reaction sites assembling the amino and the vinylhydropyridine into a 1,8-naphthalimide framework. The vinylhydropyridine group is introduced to enhance the conjugation of the naphthalimide fluorophore and improve the hydrophilicity by the potential intramolecular NH⋯NH2 hydrogen bonding. Meanwhile, the prompt oxidation of vinylhydropyridine could improve the sensitivity of the probe and accelerate the transformation of amino to the corresponding nitroso compound along with a remarkable change of the fluorescence signal.
Results and discussion
Optical response of probe 1a towards ClO−
The probe 1a was readily obtained by our previously reported procedure involving the reduction of the nitro substrate 4b.9 With the probe in hand, the essential spectral characteristics of 1a in response to ClO− were investigated in CH3CN/PBS (v/v = 1
:
9, Fig. S1, ESI†) solution. The selectivity of sensor 1a toward ClO− was first investigated through UV-vis spectroscopy by adding the analyte (ClO−, H2O2, ONOO−, 1O2, ˙OH, NO2−, HSO3−, SH−, S2−, CN−, SCN−, F−, Cl−, Br−, I−, HSO4−, ClO4−, H2PO4−, BF4−, P2O74−, OH−, Na+, K+, Mg2+, Ca2+, Zn2+, Al3+, Fe3+ and Ba2+). The free sensor 1a displayed an absorption band at 514 nm, which, upon adding ClO−, shifted to 478 nm along with a decrease in its intensity (Fig. 1a). However, no moderate changes were observed while adding other anions. Thus, 1a showed an excellent selectivity toward ClO− and was not affected and interfered by other anions (Fig. 1c). The UV-vis titration of 1a with ClO− is illustrated in Fig. 1b, where a gradually decreased absorbance signal centered at 505 nm was observed. The blue shift of the wavelength results from the potential interruption in electronic conjugation of the coplanar conjugated π-system of probe 1a by oxidation of pyridomethene to stable pyridylmethane. Further, a linear relationship between the absorbance and ClO− levels from 0 to 500 μM was obtained (Fig. 1d).
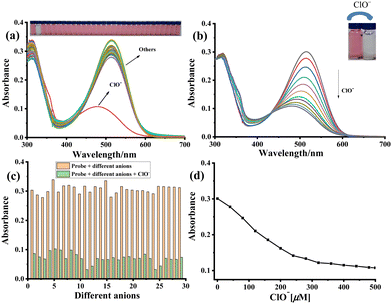 |
| Fig. 1 (a) UV-Vis spectral changes of 1a with various anions (inset: from left to right: 1a only, ClO−, H2O2, ONOO−, 1O2, ˙OH, NO2−, HSO3−, SH−, S2−, CN−, SCN−, F−, Cl−, Br−, I−, HSO4−, ClO4−, H2PO4−, BF4−, P2O74−, OH−, Na+, K+, Mg2+, Ca2+, Zn2+, Al3+, Fe3+ and Ba2+). (b) UV-vis spectral changes of 1a upon the titration with ClO− (0 to 25.0 equiv.) (inset: from left to right: 1a only, 1a + ClO−). (c) Interference experiments of probe 1a for ClO− in the presence of other anions. (d) UV-vis spectral changes of 1a as a function of added ClO− (0 to 25.0 equiv.). All experiments were performed in PBS buffer (1a (20 μM), pH 7.4, with 10% CH3CN, v/v). | |
Furthermore, the fluorescence response of 1a towards various reactive species (H2O2, ONOO−, 1O2, ˙OH, NO2−, HSO3−, SH−, S2−, CN−, SCN−, F−, Cl−, Br−, I−, HSO4−, ClO4−, H2PO4−, BF4−, P2O74−, OH−, Na+, K+, Mg2+, Ca2+, Zn2+, Al3+, Fe3+ and Ba2+) was examined. It was observed that only ClO− could lead to the quenching of the fluorescence signal since it could convert 1a into the oxidative product, further demonstrating the excellent selectivity of the probe 1a towards ClO− (Fig. 2a). The fluorescence response of 1a to variable concentrations of ClO− is presented in Fig. 2b. In the beginning, probe 1a shows a strong fluorescence at 630 nm with dark red emission. However, the fluorescence signal gradually decreased and shifted to 626 nm upon increasing the ClO− concentration and the colour of the solution changed from red to light yellow. Thus, the results evidenced that 1a could specifically distinguish and determine the ClO−. Furthermore, the competition experiment was also performed by acquiring fluorescence emission spectra in the presence of 25.0 equiv. ClO− and 25.0 equiv. of other analytical species, including various reactive species (H2O2, ONOO−, 1O2, ˙OH, NO2−, HSO3−, SH−, S2−, CN−, SCN−, F−, Cl−, Br−, I−, HSO4−, ClO4−, H2PO4−, BF4−, P2O74−, OH−, Na+, K+, Mg2+, Ca2+, Zn2+, Al3+, Fe3+ and Ba2+), further indicating the excellent anti-interference ability of probe 1a. As shown in Fig. S2 (ESI†), the fluorescence intensity was found to be linearly proportional to the concentration of ClO− in the concentration range of 0–200 μM. The LOD by the fluorescence was determined to be 36.0 nM according to the standard method of 3δ/K (Fig. 2d). The probe 1a is superior to a large number of existing reported HClO probes (Table S1, ESI†).
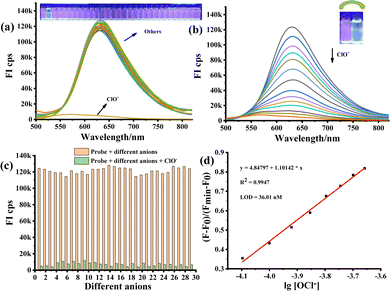 |
| Fig. 2 (a) Fluorescence spectral changes of 1a with various anions (inset: from left to right: 1a only, ClO−, H2O2, ONOO−, 1O2, ˙OH, NO2−, HSO3−, SH−, S2−, CN−, SCN−, F−, Cl−, Br−, I−, HSO4−, ClO4−, H2PO4−, BF4−, P2O74−, OH−, Na+, K+, Mg2+, Ca2+, Zn2+, Al3+, Fe3+ and Ba2+). (b) Fluorescence spectral changes of 1a (λex = 480 nm) upon the titration with ClO− (0 to 25.0 equiv.) (inset: from left to right: 1a only and 1a + ClO−). (c) Interference experiments of 1a for ClO− in the presence of other anions. (d) Linear fluorescence response of sensor 1a to ClO− at concentrations ranging from 0 to 180 μM. All experiments were performed in PBS buffer (1a (20 μM), pH 7.4, with 10% CH3CN, v/v). | |
Mechanism study
Based on the above-mentioned data, the oxidation mechanism of probe 1a with ClO− is proposed in Fig. 3a. As the pyridomethene group is an enamine tautomer of pyridylmethane, it is readily oxidized and exists in the stable pyridine form. Therefore, 1a could rapidly respond to ClO− generating the hydroxylamino (2b) and nitroso derivatives (3b), which coincides with the fluorescence quenching of 1a by ClO− due to the change in the electronic movement in the 1,8-naphthalimide system. Next, the solution of 1a reacted with ClO− was analyzed by LC–MS (Fig. S3, ESI†). A prominent peak at m/z 401.2 was detected in the MS spectra, confirming the generation of trace amounts of the hydroxylamino product 2b (calculated m/z 401.2 [M + 1]+). However, no peak corresponding to the nitroso 3b could be found during MS analysis. We then performed the NMR titration experiments of 1a with different concentrations (0–5.0 equiv.) of ClO− in DMSO-d6 after 5 min of incubation (Fig. 3b). The results revealed that the NH signal (11.8 ppm) rapidly disappears in the initial step (Fig. S4, ESI,† 0–1.0 equiv.), indicating the oxidation of the vinylhydropyridine group to the stable pyridine by intramolecular hydrogen transfer (NH to CH signal, 6.8 ppm). Besides, the signal of amino (6.9 ppm) in 1a also disappeared completely at a high concentration of ClO−, whereas two new signals at 9.8 ppm (NH) and 8.9 ppm (OH) were observed due to a possible formation of the trace amount of hydroxylamino intermediates (2b). Unfortunately, all our attempts to isolate the complex 1a/ClO− were unsuccessful. However, the final NMR spectrum of the titration of 1a with ClO− is quite similar to that of the nitro product 4b in CDCl3 (Fig. S5, ESI†), even the respective UV-vis titration spectrum also matched with the absorption spectrum of the nitro product 4b (Fig. S6 and S7, ESI†). The fluorescence titration on compound 4b was investigated and minimal response was observed (Fig. S8, ESI†). Considering the above evidence, we could confirm that probe 1a was oxidized by ClO− to give mainly the nitroso compound 3b. Thus, the mode of interaction between 1a and ClO− by the ortho-vinylhydropyridine-assisted amido-to-nitroso oxidation mechanism was finally disclosed.
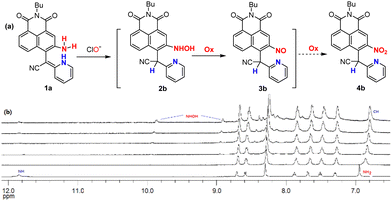 |
| Fig. 3 (a) The proposed mechanism of probe 1a with ClO−; (b) the NMR titration of 1a (30.0 mM) in DMSO-d6 with increasing concentration of ClO− (from bottom to top, 0, 1.0, 2.0, 3.0, 4.0 and 5.0 equiv.). | |
Finally, a time-dependent study of the probe 1a to ClO− was further investigated by fluorescence changes, which showed that 1a interacts with ClO− within just 1.2 s (Fig. 4a). This super-sensitive time is considered beneficial for the probe to monitor variations in a potential real environment. Moreover, the effect of pH on the recognition properties of 1a for ClO− was examined by monitoring the changes in its absorbance and fluorescence intensity upon addition of 25.0 equiv. ClO− within a wide pH range of 3–12. The response of probe 1a and its oxidative product remained stable under physiological pH (Fig. 4b), suggesting the prospective application of the probe in qualitative analysis in the basic subcellular mitochondria of living cells.10
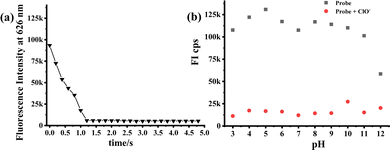 |
| Fig. 4 (a) Time-dependent fluorescence intensity of the probe 1a with ClO− (25.0 equiv.); (b) fluorescence intensity of the probe 1a with different pH values (3–12). All experiments were performed in PBS buffer (1a (20 μM), pH 7.4, with 10% CH3CN, v/v). | |
Fluorescence imaging of ClO− in living cells
To explore the application of probe 1a in cells, the cytotoxicity of 1a was tested. The results indicated that the cytotoxicity of 1a could be ignored under the experimental conditions (5 μM) for PANC cells (Fig. S9, ESI†). Subsequently, to estimate the potential biological applicability, we utilized 1a to detect exogenous ClO− in PANC cells. The cells were first incubated with 5 μM 1a and then treated with ClO− at 37 °C with 5% CO2. As shown in Fig. 5c, 1a-loaded cells displayed a strong fluorescence signal around the cell nucleus. However, significant fluorescence signal quenching was observed after the cells were treated with 25.0 equiv. ClO− (Fig. 5d), indicating that 1a produced a specific response to ClO−. Hence, probe 1a could effectively monitor the exogenous ClO− in living cells. Moreover, the test strip was prepared and the probe 1a working in the solid state for detecting the ClO− anion was observed (Fig. S10, ESI†). It demonstrates a prototype device using probe 1a for detecting ClO− in real-world applications.
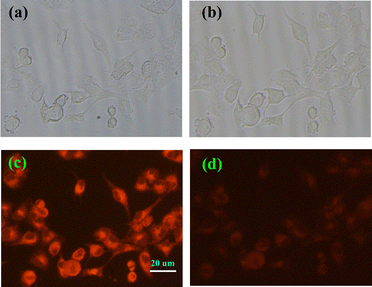 |
| Fig. 5 Fluorescence imaging of exogenous ClO− in PANC cells with 1a (5 μM) at 37 °C. Bright field images of PANC cells incubated with (a) 1a for 5 min and (b) 1a + ClO− (25.0 equiv.) for 10 min; fluorescence images of PANC cells incubated with (c) 1a for 5 min and (d) 1a + ClO− (25.0 equiv.) for 10 min from the red channel. | |
Conclusions
In summary, by designing a novel ortho-vinylhydropyridine reactive intermediate, we have experimentally demonstrated dual oxidative sites that can efficiently transform the amino-fluorophore into a nitroso product via the ClO−-mediated oxidation reaction. This probe features good water solubility, excellent specificity, sensitivity, and rapid response toward ClO− in solution and living cells. A new strategy based on the ortho-vinylhydropyridine-assisted amido-to-nitroso oxidation reaction for fluorescent and colorimetric determination of ClO− is disclosed for the first time. Carefully expanding the fluorophore framework and designing a turn-on fluorescence response by properly positioning the push–pull substitutes, this finding with the ortho-vinylhydropyridine-assisted amino oxidation reaction may serve as a powerful platform for developing novel hypochlorite chemosensors. Further studies on the detailed mechanisms, especially advancements towards more efficient ClO− fluorescent probes, are underway.
Experimental section
Materials and instruments
Unless otherwise noted, solvents and reagents were of analytical grade and used without further purification. UV-visible absorption spectra were obtained on a SHIMADZU UV-1800 spectrophotometer. Fluorescence emission spectra were obtained on a Hitachi F-4600 fluorescence spectrophotometer. NMR spectra were recorded on a Bruker AVANCE III 500 MHz, and chemical shifts are reported in parts per million (ppm, δ) downfield from the internal standard Me4Si (TMS). Multiplicities of signals are described as follows: s – singlet, d – doublet, dd – doublet d, t – triplet, m – multiplet. Coupling constants are reported in hertz (Hz). HRMS spectra were obtained on a solan X 70 FT-MS spectrometer.
Synthesis of 1a
The synthetic route of 1a is presented in Scheme 2. Compound 1a was synthesized according to the literature.94b (0.15 g, 0.36 mmol) and SnCl2 (0.60 g, 3.20 mmol) were dissolved in (20 mL) EA, and the reaction mixture was stirred under N2 at room temperature for 1 h. After the reaction was completed, the solution was filtered and saturated Na2CO3 (aq.) (150 mL) was then added. The resulting suspension was then extracted with ethyl acetate, washed with brine, dried over anhydrous sodium sulfate, concentrated and purified by column chromatography eluting with PE/EA (2
:
1) to give 1a (0.11 g, 79%). 1H NMR (500 MHz, DMSO-d6) δ 11.85 (s, 1H), 8.71 (d, J = 4.8 Hz, 1H), 8.59 (d, J = 8.3 Hz, 1H), 8.30 (t, J = 3.7 Hz, 2H), 7.92–7.85 (m, 1H), 7.69 (d, J = 7.9 Hz, 1H), 7.52 (t, J = 7.9 Hz, 1H), 7.30 (dd, J = 7.5, 5.0 Hz, 1H), 6.95 (s, 2H), 4.06 (t, J = 7.5 Hz, 2H), 1.63 (t, J = 7.4 Hz, 2H), 1.36 (t, J = 7.4 Hz, 2H), 0.94 (t, J = 7.3 Hz, 3H) (Fig. S11, ESI†).
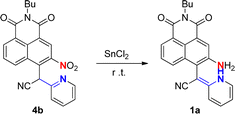 |
| Scheme 2 Synthesis of probe 1a. | |
Conflicts of interest
There are no conflicts to declare.
Acknowledgements
We are grateful for funding from the Postdoctoral Science Foundation of China (No. 2020M683199).
Notes and references
- D. Wu, L. Chen, Q. Xu, X. Chen and J. Yoon, Acc. Chem. Res., 2019, 52, 2158–2168 CrossRef CAS PubMed.
- X. Chen, F. Wang, J. Y. Hyun, T. Wei, J. Qiang, X. Ren, L. Shin and J. Yoon, Chem. Soc. Rev., 2016, 45, 2976–3016 RSC.
-
(a) G. J. Mao, G. Q. Gao, Z. Z. Liang, Y. Y. Wang, L. Su, Z. X. Wang, H. Zhang, Q. J. Ma and G. Zhang, Anal. Chim. Acta, 2019, 1081, 184–192 CrossRef CAS;
(b) Y. Deng, S. Feng, Q. Xia, S. Gong and G. Feng, Talanta, 2020, 215, 120901 CrossRef CAS PubMed.
-
(a) S. Bhatnagar, E. Khera, J. Liao, V. Eniola, Y. Hu, D. E. Smith and G. M. Thurber, Sci. Rep., 2019, 9, 4661 CrossRef PubMed;
(b) S. Liu, Y. Zhu, P. Wu and H. Xiong, Anal. Chem., 2021, 93, 4975–4983 CrossRef CAS PubMed;
(c) D. Soni, N. Duvva, D. Badgurjar, T. K. Roy, S. Nimesh, G. Arya, L. Giribabu and R. Chitta, Chem. – Asian J., 2018, 13, 1594–1608 CrossRef CAS PubMed;
(d) T. Huang, S. Yan, Y. Yu, Y. Xue, Y. Yu and C. Han, Anal. Chem., 2022, 94, 1415–1424 CrossRef CAS PubMed;
(e) P. Lu, X. Zhang, T. Ren and L. Yuan, Chin. Chem. Lett., 2020, 31, 2980–2984 CrossRef CAS;
(f) K. Liu, L. Fan, S. Huang, J. Sun, X. Wang, H. Li, C. Si, W. Zhang, T. Li and Z. Yang, Analyst, 2022, 147, 1976–1985 RSC;
(g) M. Li, W. Fang, B. Wang, Y. Du, Y. Hou, L. Chen, S. Cui, Y. Li and X. Yan, New J. Chem., 2021, 45, 21406–21414 RSC.
-
(a) L. He, H. Xiong, B. Wang, Y. Zhang, J. Wang, H. Zhang, H. Li, Z. Yang and X. Song, Anal. Chem., 2020, 92, 11029–11034 CrossRef CAS PubMed;
(b) L. Liu, P. Wei, W. Yuan, Z. Liu, F. Xue, X. Zhang and T. Yi, Anal. Chem., 2020, 92, 10971–10978 CrossRef CAS;
(c) P. Wei, L. Liu, Y. Wen, G. Zhao, F. Xue, W. Yuan, R. Li, Y. Zhong, M. Zhang and T. Yi, Angew. Chem., Int. Ed., 2019, 58, 4547–4551 CrossRef CAS;
(d) Y. L. Gao, Y. Pan, Y. Y. He, H. Y. Chen and V. N. Nemykin, Sens. Actuators, B, 2018, 269, 151–157 CrossRef CAS;
(e) B. Wang, F. Zhang, S. Wang, R. Yang, C. Chen and W. Zhao, Chem. Commun., 2020, 56, 2598–2601 RSC;
(f) P. Wu, Y. Zhu, L. Chen, Y. Tian and H. Xiong, Anal. Chem., 2021, 93, 13014–13021 CrossRef CAS PubMed;
(g) C. Ma, S. Hou, X. Zhou, Z. Wang and J. Yong, Anal. Chem., 2021, 93, 9640–9646 CrossRef PubMed;
(h) L. K. Li, Y. M. Hou, X. C. Liu, M. J. Tian, Q. J. Ma, N. N. Zhu and S. Z. Liu, New J. Chem., 2022, 46, 6596–6602 RSC;
(i) W. Hu, M. Zhao, K. Gu, L. Xie, M. Liu and D. Lu, RSC Adv., 2022, 12, 777–784 RSC.
-
(a) A. Rajapakse, C. Linder, R. D. Morrison, U. Sarkar, N. D. Leigh, C. L. Barnes, J. C. Daniels and K. S. Gates, Chem. Res. Toxicol., 2013, 26, 555–563 Search PubMed;
(b) B. Priewisch and K. Ruck-Braun, J. Org. Chem., 2005, 70, 2350–2352 CrossRef CAS PubMed.
-
(a) B. Zhu, L. Wu, M. Zhang, Y. Wang, C. Liu, Z. Wang, Q. Duan and P. Jia, Biosens. Bioelectron., 2018, 107, 218–223 CrossRef CAS PubMed;
(b) Z. Wang, Y. Zhang, J. Song, M. Li, Y. Yang, W. Gu, X. Xu, H. Xu and S. Wang, Dyes Pigm., 2019, 161, 172–181 CrossRef CAS;
(c) X. Wang, C. Xu, H. Song, X. Liu, X. Xie, X. Pang and Y. Zhou, J. Lumin., 2019, 210, 472–478 CrossRef CAS;
(d) K. Xiong, F. Huo, C. Yin, Y. Chu, Y. Yang, J. Chao and A. Zheng, Sens. Actuators, B, 2016, 224, 307–314 CrossRef CAS;
(e) K. Zhang, H. Wang, S. Cheng, C. Zhang, X. Zhai, X. Lin, H. Chen, R. Gao and W. Dong, Analyst, 2021, 146, 5658–5667 RSC;
(f) J. Lan, Y. Wang, Y. Qin, Z. Li, R. Zeng, L. Liu, L. Chen, K. Yang, Y. Ding and T. Zhang, Dyes Pigm., 2022, 207, 110706 CrossRef CAS;
(g) W. Qu, T. Guo, B. Yang, R. Tian, S. Qiu, X. Chen, Z. Geng and Z. Wang, Spectrochim. Acta, Part A, 2022, 281, 121649 CrossRef CAS PubMed;
(h) P. Wei, Y. Guo, L. Liu, X. Zhou and T. Yi, J. Mater. Chem. B, 2022, 10, 5211–5217 RSC;
(i) M. W. Afzal, S. Wang, Y. Gao, B. Qin, X. Tian, Y. Zhang, H. Tang and Y. Guo, Dyes Pigm., 2022, 204, 110435 CrossRef CAS;
(j) X. Bao, K. Ai, X. Cao, D. Chen, B. Zhou and C. Huo, Dyes Pigm., 2022, 197, 109928 CrossRef CAS.
- Y. Kubota, T. Tsuzuki, K. Funabiki, M. Ebihara and M. Matsui, Org. Lett., 2010, 12, 4010–4013 CrossRef CAS PubMed.
- C. Zhang, K. Ji, X. Wang, H. Wu and C. Liu, Chem. Commun., 2015, 51, 8173–8176 RSC.
- M. Y. Li, K. Li, Y. H. Liu, H. Zhang, K. K. Yu, X. Liu and X. Q. Yu, Anal. Chem., 2020, 92, 3262–3269 CrossRef CAS.
Footnote |
† Electronic supplementary information (ESI) available: Detailed experimental procedures, synthesis, spectral and characterization data of compounds, and additional spectroscopic data. See DOI: https://doi.org/10.1039/d2nj04481a |
|
This journal is © The Royal Society of Chemistry and the Centre National de la Recherche Scientifique 2023 |