DOI:
10.1039/D3MA00031A
(Paper)
Mater. Adv., 2023,
4, 1769-1776
One-step fabrication of highly stable, durable, adhesion enhanced, flexible, transparent conducting films based on silver nanowires and neutralized PEDOT:PSS†
Received
16th January 2023
, Accepted 6th March 2023
First published on 7th March 2023
Abstract
Silver nanowires (AgNWs) are one of the best material substitutes for indium tin oxide (ITO) in next-generation flexible, foldable, and bendable transparent conducting electrodes (TCEs) due to their very low sheet resistance, good transparency, higher flexibility, and ease of processing. However, they still suffer from some drawbacks such as larger surface roughness, poor stability, high haze, and poor adhesion to substrates. In this work, we report the fabrication of flexible and bendable TCEs with good mechanical stability and optical and electrical properties from the hybrid of AgNWs and neutralized PEDOT:PSS by simple one-step roll-to-roll coating. Imidazole was employed to neutralize the commercial grade PEDOT:PSS for minimal loss of conductivity. Hydroxypropyl methylcellulose (HPMC) was used to improve the hybrid dispersion and as a binder to enhance the adhesion with the substrate. Polysiloxane was used as the overcoating protective layer on the AgNWs/N-PEDOT:PSS TCF to enhance stability against oxidation and corrosion. The AgNW hybrid TCF showed a sheet resistance of 20 Ω sq−1 and optical transmittance over 95% with a low haze value of ∼1. The AgNW hybrid TCFs displayed better adhesion and exceptional mechanical stability with almost no difference in sheet resistance after 5000 repeated bending cycles. This method of hybrid TCF fabrication offering exceptional stability, low sheet resistance, good transparency and lower haze holds great potential in the context of highly flexible electronic applications.
Introduction
Transparent conducting electrodes (TCEs) are extensively used in optoelectronic devices such as displays, touch panels, solar cells, heaters, supercapacitors, organic light-emitting diodes, etc.1–15 Flexible transparent electrode materials are specifically favourable for next-generation electronic applications due to being thin, lightweight, foldable, and bendable.11–20 In the recent past, flexible and foldable TCEs have fascinated much attention due to the increasing demand for flexible and wearable electronics. Flexible TCEs have been extensively explored in various fields including energy storage, display and lighting, wearable electronics, and medical equipment, which have a large impact on human living and, at large, society. At present, indium tin oxide (ITO) TCEs are most commonly used in electronic applications owing to their higher conductivity and optical transmittance. However, the use of ITO in flexible electronics is limited due to drawbacks such as inflexibility (highly brittle), a high-temperature fabrication process, higher costs for production, and shortage of rare earth metals.21–28 Due to the above shortcomings, the production of large-size flexible and bendable devices using ITO is difficult. Hence it is very essential to find alternative materials to ITO with easy TCE fabrication processes and flexibility and bendability characteristics.
In the last decade, there has been growing interest in developing ITO alternatives for flexible electronic devices. Flexible electronics have been widely explored based on carbon nanotubes (CNTs),28–34 graphene,34–39 conducting polymers (PEDOT:PSS),40–43 metal meshes and metal grids,44–49 and silver nanowires (AgNWs).2–6,18–24 Conducting polymers and carbon-based nanomaterials are extremely flexible and allow low-temperature solution processing for the fabrication of electrodes. However, the sheet resistances of conducting polymer and carbon material transparent electrodes are higher in the range between 100 and 500 Ω sq−1 (transparency, 80–95%) when compared with ITO (10–50 Ω sq−1).
AgNWs are considered a suitable replacement material for ITO because of their high electrical conductivity, transparency, and simple and low-cost solution fabrication process. However, AgNWs cannot be used directly as transparent electrodes due to disadvantages such as weak bonding between nanowires, poor adhesion with substrates, high haze (strong light scattering), limited mechanical and electrical stability, large-sized holes and inhomogeneous resistance distribution due to surface roughness.50–56 However, there have been a lot of efforts which include mechanical pressing, thermal annealing, plasmonic welding, introduction of conducting materials (PEDOT:PSS, SWCNTs, graphene, MXenes, etc.), binders (PVA, PDMS, epoxy) and overcoating (conductive polymer/silica binder) which overcame certain drawbacks of AgNW TCFs.7,57–68 However, the reliability and mechanical stability of AgNW TCFs remain an important issue. AgNW TCF fabrication with hybrid approaches and post-processing (thermal annealing, mechanical pressing, and protective layer overcoating) which are done in multiple steps would also increase the manufacturing cost and restrict scalable fabrication for commercial applications.
PEDOT:PSS is a suitable material for hybrid AgNW TCFs due to its exceptionally high electrical conductivity, transparency, and good compatibility for the one-step fabrication process.54–58 However, the highly acidic nature of PEDOT:PSS (sulfonic acid groups of PSS) adversely impacts AgNWs via acidic corrosion.69–73 To the best of our knowledge, only a few reports currently exist on the fabrication of TCFs from the hybrid of AgNWs with neutralized PEDOT:PSS to solve the corrosion stability problem of AgNWs. Chen et al.59 explored the multi-step fabrication of AgNWs/PEDOT:PSS TCFs using neutralized PEDOT:PSS (guanidine as a neutralizing agent) as the overcoating layer. Unfortunately, the neutralized PEDOT:PSS exhibited lower conductivity (∼60% lower compared to pristine) and the resulting AgNW hybrid film showed poor uniformity and high surface roughness. Recently, Bhargavi et al. reported the fabrication of a similar AgNW hybrid based on commercially available neutral PEDOT:PSS.74 In that report, the hybrid film was prepared using an extrusion-based direct write system and tested as a printed heater. The length of the AgNWs was purposefully reduced using ultrasonication for extrusion printing. Kim et al.60 fabricated hybrid AgNW TCFs by a simple roll-to-roll method using neutralized PEDOT:PSS and AgNWs. In that work, imidazole was used as a mild base material to neutralize the PEDOT:PSS. Due to its highly hygroscopic and brittle nature, excessive PSS which was used to adjust the ratio of PEDOT for the fabrication of electrodes resulted in lower stability and durability of the TCFs. These problems need to be solved for practical flexible electronic applications.
In this work, we describe the fabrication of a flexible, bendable, transparent conducting electrode from the hybrid of AgNWs with neutralized PEDOT:PSS by a simple roll-to-roll approach. Hydroxypropyl methylcellulose (HPMC) is used as a binder to improve the compatibility and adhesion properties of the hybrid dispersions.63,64 Neutralized PEDOT:PSS and HPMC improve the adhesion strength between AgNWs and the PET substrate. Also, PEDOT:PSS acts as an interconnector (soldering material) between AgNWs for better charge transport, resulting in enhanced electrical conductivity and higher surface smoothness. In addition, the hybrid film with polysiloxane overcoating can enhance mechanical stability and durability. The mechanical stability of AgNWs hybrid TCFs is investigated with repeated bending and adhesive peel-off tests. The fabricated AgNWs/N-PEDOT:PSS hybrid film displayed a lower sheet resistance of 20 Ω sq−1 with optical transparency over 95% without any post-treatment.
Results and discussion
PEDOT:PSS was utilized as a conducting material for the fabrication of hybrid AgNW TCFs to decrease the haze and roughness while increasing the mechanical stability and durability. Besides, PEDOT:PSS in the AgNWs acts as a soldering material between nanowires, leading to higher conductivity and a homogenous resistance distribution. However, the highly acidic and hygroscopic behaviour of PEDOT:PSS implies inferior stability due to oxidation and acidic corrosion by the excess sulfonic acid groups of PSS. PEDOT:PSS is generally neutralized with base materials including diethanolamine or triethanolamine, guanidine, imidazole derivatives, NaOH, KOH, and ammonia as reported elsewhere.54,55,71 Among them, imidazole as a mild base is the best candidate for neutralization of PEDOT:PSS with a marginal reduction in conductivity. In addition, imidazole acts as a corrosion inhibitor against metals (ITO, copper, aluminium, silver) due to the azole group with free electron pairs of nitrogen atoms which strongly binds with the metallic surface preventing corrosion.56,71 In this work, 1 M imidazole solution was used for neutralization of PEDOT:PSS, which was then utilized for the preparation of the hybrid dispersion of AgNWs.
AgNWs/PEDOT:PSS hybrid dispersion was prepared using AgNWs, neutralized PEDOT:PSS, and HPMC (1.0 wt% aqueous solution) through a roll mixer for 1–2 h. HPMC was used as an adhesive binder to improve the stability/compatibility of the hybrid dispersion and the adhesion between the AgNWs and substrate. HPMC also helps to enhance the extensive contact between the AgNWs with homogenous resistance distribution.64 The AgNWs/N-PEDOT:PSS hybrid dispersion was used to fabricate TCFs via simple one-step roll-to-roll coating (Scheme 1) using a Mayer rod on a PET substrate, followed by drying at 130 °C for 5 min in a hot air oven. Hybrid TCFs were then overcoated with polysiloxane (thanks to Solvay Korea) by the Mayer rod and dried at 130 °C for 5 min. The fabricated hybrid TCFs (Fig. S1, ESI†) showed relatively high Rs values between 85 and 125 Ω sq−1 with haze values in the range of 1.0–2.0. The higher Rs of the hybrid TCFs was due to the higher weight percentage of PEDOT:PSS (1.3 wt%) with AgNWs (0.5 wt%).
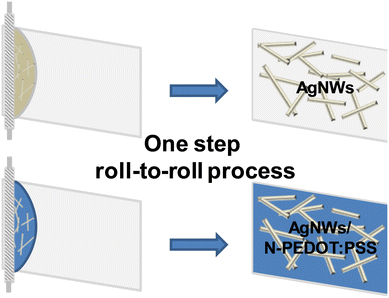 |
| Scheme 1 Schematic illustration of the fabrication of highly conductive flexible, transparent conducting films from pure AgNWs and the AgNWs/N-PEDOT:PSS hybrid by a one-step solution process using Mayer rod coating. | |
Thus, the N-PEDOT:PSS (1.3 wt%) dispersion was adjusted to a weight percentage of ∼0.5 wt% by diluting with isopropyl alcohol (IPA), dimethyl sulfoxide (DMSO) and distilled water to reduce the Rs value. The AgNW hybrid dispersion (Fig. S2, ESI†) was prepared by mixing AgNWs (0.5 wt% in water) and neutralized PEDOT:PSS (0.5 wt%) at varying weight ratios (1
:
0, 1
:
1, 2
:
1, and 0
:
1). Then 5 wt% HPMC (1.0 wt% aqueous solution) was added to the hybrid solution and mixed well for 1–2 h using a roll mixer. Hybrid TCFs (Fig. S3, ESI†) were fabricated by the same coating method mentioned above and used for further characterization. The results are shown in Table S1 (ESI†) and Fig. 1. The developed hybrid TCFs showed lower Rs values in the range of 20–30 Ω sq−1 with haze values of ∼1 and transmittance over 95%. The comparison of TCF results (resistance, transmittance, and haze) between this work and the literature is presented in Table S1 (ESI†) and Fig. 1. It was observed that the developed AgNWs/N-PEDOT:PSS hybrid TCFs showed lower Rs, higher transmittance, and lower haze than previously reported TCFs based on AgNWs, SWCNTs, DWCNTs, graphene, AgNWs/graphene, and AgNWs/PEDOT:PSS.4,5,11,22,24,28,37,50,54,56,62,75–84 This is due to the specific hybrid formulation with an optimal ratio of PEDOT:PSS with AgNWs, and the mild roll mixing and simple roll-to-roll fabrication process. The mild roll mixing and roll-to-roll fabrication process might also have maintained the AgNW structure intact, resulting in higher electrical conductivity, very good transparency and lower haze.
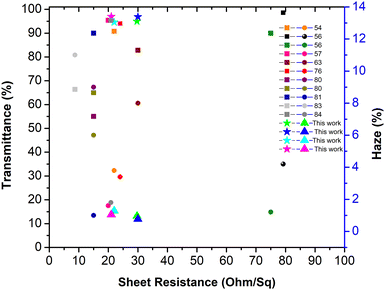 |
| Fig. 1 Sheet resistance, transmittance, and haze of the pristine AgNW film, AgNWs/N-PEDOT:PSS hybrid TCFs and already reported TCFs (■,★ – transmittance and ●,▲ – haze). | |
The surface morphology of the neat AgNW and hybrid AgNW TCFs was investigated using SEM (Fig. 2). As shown in Fig. 2a, the pristine AgNW film showed a very rough surface; each nanowire was loosely in contact with the other one and part of them were even out of contact with the substrate (poor adhesion) which resulted in low electrical conductivity. Thus, the neat AgNW film had less durability under mechanical stress, resulting in a drastic increase in sheet resistance. In contrast, the AgNWs/N-PEDOT:PSS hybrid film (Fig. 2b) showed a comparatively smooth surface. The SEM image suggests that AgNWs were closely embedded and the gaps between AgNWs were completely covered with PEDOT:PSS and HPMC. In the SEM images, the AgNW microstructure remained almost the same as the pure AgNW system. Similar observations are reported elsewhere.59,60 The presence of PEDOT:PSS and HPMC in the hybrid structure substantially improves the adhesion between the AgNWs and the PET. Besides, PEDOT:PSS as a bridge or soldering material between AgNW junctions reduces the contact resistance which results in improved electrical properties. Nonetheless, the high surface roughness of AgNW films usually leads to an acute problem of light scattering (haze) which restricts their use as TCEs. Due to blurriness (less clarity) caused by a high haze value, the TCEs from AgNWs even with high transmittance and low resistance are not suitable for display applications. Thus, a lot of research studies are focused on lowering the haze (higher transparency) to the ITO level (approximately 1–3%) as an essential limit for display applications (OLEDs, OPVs, etc.).64,75,76,84 In AgNW hybrid TCFs, the introduction of PEDOT:PSS and HPMC as interconnecting/filling materials improved the surface roughness with a dramatic reduction in the haze value. The haze value of the AgNWs/N-PEDOT:PSS film was ∼1.0 which was well comparable to ITO.
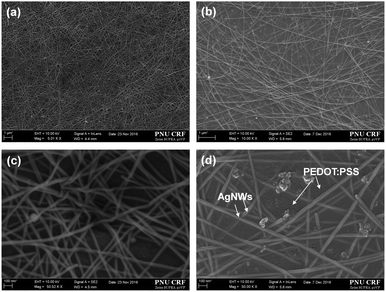 |
| Fig. 2 SEM images of AgNWs (a and c) and AgNWs/N-PEDOT:PSS hybrid (b and d) TCFs. [(a) and (b) - low magnification and (c) and (d) - high magnification images]. | |
The surface topology and roughness of the neat AgNW and AgNWs/N-PEDOT:PSS hybrid films were also studied using AFM (Fig. 3). The roughness (RMS) values of the neat AgNW and AgNWs/N-PEDOT:PSS hybrid (weight ratio of AgNWs to PEDOT
:
PSS of 1
:
1) TCFs were 20.5 and 9.4 nm, respectively. In Fig. 3a, the surface of the pristine AgNW TCF with bulged AgNW clusters can act as electrical shortcuts which may lead to the deterioration of device performance. The large sized holes in the AgNW network restrict the efficient collection of charge carriers and result in an uneven distribution of resistance throughout the entire film surface. These problems could certainly be resolved by the inclusion of PEDOT:PSS into AgNWs (Fig. 3b). The hybrid AgNWs/PEDOT:PSS films showed a much smoother surface with lower surface roughness than the pristine AgNW film. Here, the PEDOT:PSS acted as a reinforcing material to fill the voids between AgNWs, resulting in a smoother surface morphology (reduction of surface roughness) and higher conductivity. Thus, this method is favourable for the fabrication of TCEs with a smoother surface. However, the roughness of AgNW TCFs makes it extremely critical to use them as electrodes in OLEDs, in which the surface roughness is usually needed to be below 2 nm.19 This could be attained by mechanical pressing and other formulation techniques.
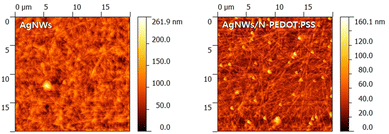 |
| Fig. 3 AFM phase images of AgNWs and AgNWs/N-PEDOT:PSS hybrid TCFs. | |
For long-term stability, AgNWs were hybridized with non-neutralized PEDOT:PSS and N-PEDOT:PSS and their resistance was compared over time (Fig. S5, ESI†). The resistance of non-neutral PEDOT:PSS drastically increased with time in comparison with the negligible resistance change in N-PEDOT:PSS. This is due to the acidic nature of PEDOT:PSS which reduces the stability of AgNWs via corrosion and increases the resistance.55,74 To demonstrate the mechanical durability and flexibility, the pristine AgNW and AgNWs/N-PEDOT:PSS hybrid TCFs were also investigated in terms of changes in resistance against repeated bending cycles and peel-off tape adhesion test (the photo image of the flexible AgNWs/N-PEDOT:PSS hybrid TCF on the PET substrate is shown in Fig. S4, ESI†). The TCFs were tested by bending with a radius curvature of 10 mm for 5000 bending cycles. Fig. 4 shows the Rs of TCFs over 5000 repeated bending cycles. A nominal increase of 10–15% in the Rs value of the hybrid TCF was observed after 5000 repeated bending cycles. In contrast, the pristine AgNW TCF showed a drastic increase in the Rs after repeated bending cycles. Further, AgNW hybrid TCFs were overcoated with polysiloxane to enhance the stability. As a result, the sheet resistance of the overcoated AgNW hybrid TCF remained the same as the initial resistance value after 5000 repeated bending cycles. The polysiloxane overcoating layer improved the mechanical durability of the TCF without affecting the electrical conductivity.
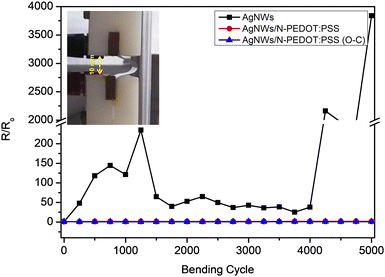 |
| Fig. 4 Mechanical bending test in terms of change in sheet resistance (R/Ro) for pristine AgNW and AgNWs/N-PEDOT:PSS hybrid TCFs with repeated bending cycles. Ro – initial sheet resistance and R – sheet resistance after repeated bending cycles (inset: photograph of TCF for mechanical bending). | |
To check the adhesive strength, the peel-off test was carried out on the neat AgNW and AgNWs/PEDOT:PSS hybrid TCFs. In this test, the ScotchTM tape (3M) was first stuck on the TCF by rubbing the entire surface with constant force, and then the Scotch tape was peeled off from the TCF. In the hybrid TCF, no visible difference was noticed before and after the tape test (Fig. 5). In contrast, the pristine AgNW TCF was detached from the PET substrate during the peel-off tape test. This indicated the poor adhesion of the pristine AgNW film to the substrate. In the hybrid system, PEDOT:PSS and HPMC tightly embedded in the AgNW junction networks increased the adhesion between the substrate and AgNWs, resulting in higher durability of the TCFs. These results reveal that this method is suitable for the fabrication of films as TCEs for application in flexible display electronics, including touch screen, OLEDs, and OPVs.
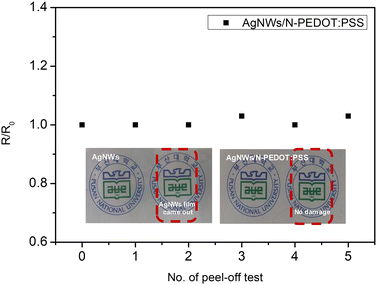 |
| Fig. 5 Adhesive peel-off tape test (3M ScotchTM tape) for pristine AgNW and AgNWs/N-PEDOT:PSS hybrid TCFs. | |
Conclusions
We successfully developed a simple and cost-effective one-step solution fabrication process of a highly transparent, highly conducting, flexible, and durable AgNWs/N-PEDOT:PSS films by roll-to-roll coating without any post-treatment. The Rs value of the resulting TCF was observed to be as low as 20 Ω sq−1, with higher transmittance over 95% and lower haze <1. The neutralization of the acidic PEDOT:PSS dispersion with imidazole was used to prevent acidic corrosion for higher stability. The AgNW hybrid TCF with a much smoother surface and low surface roughness was achieved by filling the holes between AgNWs using N-PEDOT:PSS and HPMC. Besides, HPMC and PEDOT:PSS as soldering materials improved the adhesion of AgNWs with the PET substrate along with the bonding between AgNWs, resulting in lower contact resistance and higher electrical conductivity. In the bending test over 5000 cycles, the polysiloxane overcoating as a protecting layer improved the mechanical stability and durability in terms of almost no variation in the resistance. TCFs were also stable during the tape peel-off test. We believe that this fabrication method will inspire the large-scale production of TCEs with improved properties for flexible optoelectronic applications.
Author contributions
Subramani Devaraju: formal analysis, investigation, methodology, writing – original draft. Aruna Kumar Mohanty: validation, writing – review & editing. Du-hyun Won: formal analysis, project administration. Hyun-jong Paik: funding acquisition, project administration, supervision, writing – review & editing.
Conflicts of interest
There are no conflicts to declare.
Acknowledgements
This work was supported by the Industrial Strategic Technology Development Program (20010566) funded by the Ministry of Trade, Industry and Energy (MOTIE) of Korea. Also, this work was supported by Solvay Chemicals (GBU Special Chem) research project.
Notes and references
- X. Lu, Y. K. Zhang and Z. J. Zheng, Metal-Based Flexible Transparent Electrodes: Challenges and Recent Advances, Adv. Electron. Mater., 2021, 7, 5802 CrossRef.
- K. Ellmer, Past Achievements and Future Challenges in the Development of Optically Transparent Electrodes, Nat. Photonics, 2012, 6, 809–817 CrossRef CAS.
- M.-R. Azani, A. Hassanpour and T. Torres, Benefits, Problems, and Solutions of Silver Nanowire Transparent Conductive Electrodes in Indium Tin Oxide (ITO)-Free Flexible Solar Cells, Adv. Energy Mater., 2020, 10, 2002536 CrossRef CAS.
- W. Gaynor, G. F. Burkhard, M. D. McGehee and P. Peumans, Smooth Nanowire/Polymer Composite Transparent Electrodes, Adv. Mater., 2011, 23, 2905–2910 CrossRef CAS PubMed.
- S. Cho, S. Kang, A. Pandya, R. Shanker, Z. Khan, Y. Lee, J. Park, S. L. Craig and H. Ko, Large-Area Cross-Aligned Silver Nanowire Electrodes for Flexible, Transparent, and Force-Sensitive Mechanochromic Touch Screens, ACS Nano, 2017, 11, 4346 CrossRef CAS PubMed.
- Z. Yu, L. Li, Q. Zhang, W. Hu and Q. Pei, Silver Nanowire-Polymer Composite Electrodes for Efficient Polymer Solar Cells, Adv. Mater., 2011, 23, 4453–4457 CrossRef CAS PubMed.
- H. Tang, H. Feng, H. Wang, X. Wan, J. Liang and Y. Chen, Highly Conducting MXene–Silver Nanowire Transparent Electrodes for Flexible Organic Solar Cells, ACS Appl. Mater. Interfaces, 2019, 11, 25330 CrossRef CAS PubMed.
- P. Wang, M. Jian, C. Zhang, M. Wu, X. Ling, J. Zhang, B. Wei and L. Yang, Highly Stable Graphene-Based Flexible Hybrid Transparent Conductive Electrodes for Organic Solar Cells, Adv. Mater. Interfaces, 2022, 9, 2101442 CrossRef CAS.
- H. J. Yun, S. J. Kim, J. H. Hwang, Y. S. Shim, S.-G. Jung, Y. W. Park and B.-K. Ju, Silver Nanowire-IZO-Conducting Polymer Hybrids for Flexible and Transparent Conductive Electrodes for Organic Light-Emitting Diodes, Sci. Rep., 2016, 6, 34150 CrossRef CAS PubMed.
- S. H. Pham, A. Ferri, A. Da, M. M. S. Mohan, V. D. Tran, D. C. Nguyen, P. Viville, R. Lazzaroni, R. Desfeux and P. Leclère, Nanoscale Electrical Investigation of Transparent Conductive Electrodes Based on Silver Nanowire Network, Adv. Mater. Interfaces, 2022, 9, 2200019 CrossRef CAS.
- N. M. Nair, J. K. Pakkathillam, K. Kumar, K. Arunachalam, D. Ray and P. Swaminathan, Printable Silver Nanowire and PEDOT:PSS Nanocomposite Ink for Flexible Transparent Conducting Applications, ACS Appl. Electron. Mater., 2020, 2, 1000–1010 CrossRef CAS.
- V. Raman, A. R. Selvaraj, S. W. Kim, K. Prabakar and H. K. Kim, Self-Encapsulated Cu Grid for Highly Transparent Conductive Electrode for Transparent Heater and Electrochemical Supercapacitor Applications, Adv. Electron. Mater., 2022, 8, 2200504 CrossRef CAS.
- S. B. Singh, T. Kshetri, T. I. Singh, N. H. Kim and J. H. Lee, Embedded PEDOT:PSS/AgNFs network flexible transparent electrode for solid-state supercapacitor, Chem. Eng. J., 2019, 359, 197–207 CrossRef CAS.
- W.-C. Zhu, P.-Q. He, H.-C. Tien, H.-L. Liu, W.-C. Chen, W. Lv and W.-Y. Lee, Solvent-Enhanced Transparent Stretchable Polymer Nanocomposite Electrode for Supercapacitors, ACS Appl. Energy Mater., 2021, 4, 2266–2274 CrossRef CAS.
- H. B. Lee, W.-Y. Jin, M. M. Ovhal, N. Kumar and J.-W. Kang, Flexible transparent conducting electrodes based on metal meshes for organic optoelectronic device applications: a review, J. Mater. Chem. C, 2019, 7, 1087–1110 RSC.
- S. Yu, X. Ma, X. Li, J. Li, B. Gong and X. Wang, Enhanced adhesion of Ag nanowire based transparent conducting electrodes for application in flexible electrochromic devices, Opt. Mater., 2021, 120, 111414 CrossRef CAS.
- R. Deshmukh, M. Calvo, M. Schreck, E. Tervoort, A. S. Sologubenko and M. Niederberger, Synthesis, Spray Deposition, and Hot-Press Transfer of Copper Nanowires for Flexible Transparent Electrodes, ACS Appl. Mater. Interfaces, 2018, 10, 20748–20754 CrossRef CAS PubMed.
- Y. Wang, Y. Liu, T. Wang, S. Liu, Z. Chen and S. Duan, Low-temperature nanowelding silver nanowire hybrid flexible transparent conductive film for green light OLED devices, Nanotechnology, 2022, 33, 455201 CrossRef PubMed.
- L. Hu, H. S. Kim, J.-Y. Lee, P. Peumans and Y. Cui, Scalable Coating and Properties of Transparent, Flexible, Silver Nanowire Electrodes, ACS Nano, 2010, 4, 2955–2963 CrossRef CAS PubMed.
- J. Lee, P. Lee, H. Lee, D. Lee, S. S. Lee and S. H. Ko, Very Long, Ag Nanowire Synthesis and Its Application in a Highly Transparent, Conductive and Flexible Metal Electrode Touch Panel, Nanoscale, 2012, 4, 6408–6414 RSC.
- J. Lee, P. Lee, H. B. Lee, S. Hong, I. Lee, J. Yeo, S. S. Lee, T. S. Kim, D. Lee and S. H. Ko, Room-Temperature Nanosoldering of a Very Long Metal Nanowire Network by Conducting-Polymer-Assisted Joining for a Flexible Touch-Panel Application, Adv. Funct. Mater., 2013, 23, 4171–4176 CrossRef CAS.
- M. S. Lee, K. Lee, S. Y. Kim, H. Lee, J. Park, K. H. Choi, H. K. Kim, D. G. Kim, D. Y. Lee, S. Nam and J. U. Park, High-Performance, Transparent, and Stretchable Electrodes Using Graphene−Metal Nanowire Hybrid Structures, Nano Lett., 2013, 13, 2814–2821 CrossRef CAS PubMed.
- Y. Li, P. Cui, L. Wang, H. Lee, K. Lee and H. Lee, Highly Bendable, Conductive, and Transparent Film by an Enhanced Adhesion of Silver Nanowires, ACS Appl. Mater. Interfaces, 2013, 5, 9155–9160 CrossRef CAS PubMed.
- Z. Liang and K. R. Graham, Surface Modification of Silver Nanowires for Morphology and Processing Control in Composite Transparent Electrodes, ACS Appl. Mater. Interfaces, 2015, 7, 21652–21656 CrossRef CAS PubMed.
- B. P. Yalagala, S. A. Sankaranarayanan, A. K. Rengan and S. R. K. Vanjari, Biocompatible, Flexible, and High-Performance Nanowelded Silver Nanowires on Silk Fibroin for Transparent Conducting Electrodes toward Biomemristor Application, ACS Sustainable Chem. Eng., 2022, 10, 4473–4485 CrossRef CAS.
- K. S. Cho, S. Kang, Y. J. Oh, J. S. Park, S. Lee, J. S. Wi, J. H. Park, S. Song, K. Kim, Y. J. Eo, J. H. Yun, J. Gwak, J. S. Cho and C. H. Chung, Hierarchical Silver Network Transparent Conducting Electrodes for Thin-Film Solar Cells, ACS Appl. Electron. Mater., 2022, 4, 823–830 CrossRef CAS.
- S. B. Singh, Y. Hu, T. Kshetri, N. H. Kim and J. H. Lee, An Embedded-PVA@Ag Nanofiber Network for Ultra-Smooth, High Performance Transparent Conducting Electrodes, J. Mater. Chem. C, 2017, 5, 4198–4205 RSC.
- Q. Zhang, J. S. Nam, J. Han, S. Datta, N. Wei, E. X. Ding, A. Hussain, S. Ahmad, V. Skakalova and A. T. Khan, Large-Diameter Carbon Nanotube Transparent Conductor Overcoming Performance–Yield Tradeoff, Adv. Funct. Mater., 2021, 32, 2103397 CrossRef.
- B. Dan, G. C. Irvin and M. Pasquali, Continuous and Scalable Fabrication of Transparent Conducting Carbon Nanotube Films, ACS Nano, 2009, 3, 835–843 CrossRef CAS PubMed.
- L. Hu, D. S. Hecht and G. Gruner, Carbon Nanotube Thin Films: Fabrications, Properties and Applications, Chem. Rev., 2010, 110, 5790–5844 CrossRef CAS PubMed.
- T. Lee, B. Kim, S. Kim, J. H. Han, H. B. Jeon, Y. S. Lee and H. J. Paik, Fabrication of flexible, transparent and conductive films from single-walled carbon nanotubes with high aspect ratio using poly((furfuryl methacrylate)-co-(2-(dimethylamino)ethyl methacrylate)) as a new polymeric dispersant, Nanoscale, 2015, 7, 6745–6753 RSC.
- P. Kumar, K. L. Woon, W. S. Wong, M. S. M. Saheed and Z. A. Burhanudin, Hybrid film of single-layer graphene and carbon nanotube as transparent conductive electrode for organic light emitting diode, Synth. Met., 2019, 257, 116186 CrossRef CAS.
- S. Devaraju, T. Lee, A. K. Mohanty, Y. K. Hong, K. H. Yoon, Y. S. Lee, J. H. Han and H.-J. Paik, Fabrication of durable and flexible single-walled carbon nanotube transparent conductive films, RSC Adv., 2017, 7, 19267–19272 RSC.
- D. S. Hecht, L. Hu and G. Irvin, Emerging Transparent Electrodes Based on Thin Films of Carbon Nanotubes, Graphene, and Metallic Nanostructures, Adv. Mater., 2011, 23, 1482–1513 CrossRef CAS PubMed.
- S. Bae, H. Kim, Y. Lee, X. F. Xu, J. S. Park, Y. Zheng, J. Balakrishnan, T. Lei, H. R. Kim, Y. I. Song, Y. J. Kim, K. S. Kim, B. Ozyilmaz, J. H. Ahn, B. H. Hong and S. Iijima, Roll-to-roll production of 30-inch graphene films for transparent electrodes, Nat. Nanotechnol., 2010, 5, 574 CrossRef CAS PubMed.
- K. S. Kim, Y. Zhao, H. Jang, S. Y. Lee, J. M. Kim, K. S. Kim, J.-H. Ahn, P. Kim, J.-Y. Choi and B. H. Hong, Large-scale pattern growth of graphene films for stretchable transparent electrodes, Nature, 2009, 457, 706–710 CrossRef CAS PubMed.
- R. Chen, S. R. Das, C. Jeong, M. R. Khan, D. B. Janes and M. A. Alam, Co-Percolating Graphene-Wrapped Silver Nanowire Network for High Performance, Highly Stable, Transparent Conducting Electrodes, Adv. Funct. Mater., 2013, 23, 5150–5158 CrossRef CAS.
- A. M. Díez-Pascual and A. Rahdar, Graphene-Based Polymer Composites for Flexible Electronic Applications, Micromachines, 2022, 13, 1123 CrossRef PubMed.
- B. Che, D. Zhou, H. Li, C. He, E. Liu and X. Lu, A highly bendable transparent electrode for organic electrochromic devices, Org. Electron., 2019, 66, 86–93 CrossRef CAS.
- S. Shin, M. Yang, L. J. Guo and H. Youn, Roll-to-Roll Cohesive, Coated, Flexible, High-Efficiency Polymer Light-Emitting Diodes Utilizing ITO-Free Polymer Anodes, Small, 2013, 9, 4036–4044 CrossRef CAS PubMed.
- D. J. Lipomi, J. A. Lee, M. Vosgueritchian, R. C. K. Tee, J. A. Bolander and Z. Bao, Electronic Properties of Transparent Conductive Films of PEDOT:PSS on Stretchable Substrates, Chem. Mater., 2012, 24, 373–382 CrossRef CAS.
- D. Alemu, H. Y. Wei, K. C. Ho and C. W. Chu, Highly conductive PEDOT:PSS electrode by simple film treatment with methanol for ITO-free polymer solar cells, Energy Environ. Sci., 2012, 5, 9662 RSC.
- L. Liu, S. Li, L. Wu, D. Chen, K. Cao, Y. Duan and S. Chen, Enhanced flexibility and stability of PEDOT: PSS electrodes through interfacial crosslinking for flexible organic light-emitting diodes, Org. Electron., 2021, 89, 106047 CrossRef CAS.
- H. Wu, D. Kong, Z. Ruan, P. C. Hsu, S. Wang, Z. Yu, T. J. Carney, L. Hu, S. Fan, Y. Cui and A. Transparent, Electrode Based on a Metal Nanotrough Network, Nat. Nanotechnol., 2013, 8, 421–425 CrossRef CAS PubMed.
- J. Ge, H. B. Yao, X. Wang, Y. D. Ye, J. L. Wang, Z. Y. Wu, J. W. Liu, F. J. Fan, H. L. Gao, C. L. Zhang and S. H. Yu, Stretchable Conductors Based on Silver Nanowires: Improved Performance through a Binary Network Design, Angew. Chem., 2013, 52, 1654–1659 CrossRef CAS PubMed.
- Y.-Y. Wang, B.-J. Li, L.-J. Huang and Q. Xu, nFabrication and performances of silver grid transparent conducting films and heaters on glass and PET substrates based on fractal periodic grid pattern design, Surf. Interfaces, 2022, 31, 102072 CrossRef CAS.
- T. Kamijo, S. de Winter, P. Panditha and E. Meulenkamp, Printed Copper Grid Transparent Conducting Electrodes for Organic Light-Emitting Diodes, ACS Appl. Electron. Mater., 2022, 4, 698–706 CrossRef CAS.
- J. Zou, H.-L. Yip, S. K. Hau and A. K. Y. Jen, Metal grid/conducting polymer hybrid transparent electrode for inverted polymer solar cells, Appl. Phys. Lett., 2010, 96, 203301 CrossRef.
- S. Yu, J. Li, L. Zhao, B. Gong and L. Li, Folding-insensitive, flexible transparent conductive electrodes based on copper nanowires, Sol. Energy Mater. Sol. Cells, 2021, 231, 111323 CrossRef CAS.
- M. Menamparambath, C. M. Ajmal, K. H. Kim, D. Yang, J. Roh, H. C. Park, C. Kwak, J. Y. Choi and S. Baik, Silver nanowires decorated with silver nanoparticles for low-haze flexible transparent conductive films, Sci. Rep., 2015, 5, 16371 CrossRef CAS PubMed.
- A. Kim, Y. Won, K. Woo, S. Jeong and J. Moon, All-Solution-Processed Indium-Free Transparent Composite Electrodes based on Ag Nanowire and Metal Oxide for Thin-Film Solar Cells, Adv. Funct. Mater., 2014, 24, 2462 CrossRef CAS.
- B. Zheng, Q. Zhu and Y. Zhao, Flexible silver nanowire transparent conductive films prepared by an electrostatic adsorption self-assembly process, J. Mater. Sci., 2019, 54, 5802–5812 CrossRef CAS.
- S. Arulkumar, T. Senthilkumar, S. Parthiban, G. Dharmalingam, A. Goswami, S. M. Alshehri and M. B. Gawande, AgNWs-a-TiOx: a scalable wire bar coated core–shell nanocomposite as transparent thin film electrode for flexible electronics applications, J. Mater. Sci.: Mater. Electron., 2021, 32, 6454–6464 CrossRef CAS.
- S. Kim, S. Y. Kim, J. Kim and J. H. Kim, Highly reliable AgNW/PEDOT:PSS hybrid films: efficient methods for enhancing transparency and lowering resistance and haziness, J. Mater. Chem. C, 2014, 2, 5636–5643 RSC.
- S. Chen, L. Song, Z. Tao, X. Shao, Y. Huang, Q. Cui and X. Guo, Neutral-pH PEDOT:PSS as over-coating layer for stable silver nanowire flexible transparent conductive films, Org. Electron., 2014, 15, 3654–3659 CrossRef CAS.
- S. Kim, S. Y. Kim, M. H. Chung, J. Kim and J. H. Kim, A one-step roll-to-roll process of stable AgNW/PEDOT:PSS solution using imidazole as a mild base for highly conductive and transparent films: optimizations and mechanisms, J. Mater. Chem. C, 2015, 3, 5859–5868 RSC.
- S. Kim, A. Cho, S. Kim, W. Cho, M. H. Chung, F. S. Kim and J. H. Kim, Multi-purpose overcoating layers based on PVA/silane hybrid composites for highly transparent, flexible, and durable AgNW/PEDOT:PSS films, RSC Adv., 2016, 6, 19280–19287 RSC.
- J. Wang, J. Jiu, T. Sugahara, S. Nagao, M. Nogi, H. Koga, P. He, K. Suganuma and H. Uchida, Highly Reliable Silver Nanowire Transparent Electrode Employing Selectively Patterned Barrier Shaped by Self-Masked Photolithography, ACS Appl. Mater. Interfaces, 2015, 7, 23297–23304 CrossRef CAS PubMed.
- S. K. R. Pillai, J. Wang, Y. Wang, Md. M. Sk, A. B. Prakoso, Rusli and M. B. C. Park, Totally embedded hybrid thin films of carbon nanotubes and silver nanowires as flat homogenous flexible transparent conductors, Sci. Rep., 2016, 6, 38453 CrossRef CAS PubMed.
- J. Han, J. Yang, W. Gao and H. Bai, Ice-Templated, Large-Area Silver Nanowire Pattern for Flexible Transparent Electrode, Adv. Funct. Mater., 2021, 31, 2010155 CrossRef CAS.
- T. Zhao, C. Zhang, Z. Du, H. Li and W. Zou, Functionalization of AgNWs with amino groups and their application in an epoxy matrix for antistatic and thermally conductive nanocomposites, RSC Adv., 2015, 5, 91516–91523 RSC.
- S. Duan, L. Zhang, Z. Wang and C. Li, One-step rod coating of high-performance silver nanowire–PEDOT:PSS flexible electrodes with enhanced adhesion after sulfuric acid post treatment, RSC Adv., 2015, 5, 95280–95286 RSC.
- W.-H. Chung, S.-H. Kim and H.-S. Kim, Welding of silver nanowire networks via flash white light and UV-C irradiation for highly conductive and reliable transparent electrodes, Sci. Rep., 2016, 6(32086), 1–11 Search PubMed.
- A. B. V. K. Kumar, C. W. Bae, L. Piao and S.-H. Kim, Silver nanowire based flexible electrodes with improved properties: High conductivity, transparency, adhesion and low haze, Mater. Res. Bull., 2013, 48, 2944–2949 CrossRef.
- J. Kim, M. S. Kim, Y. Lee, S. Y. Kim, Y. E. Sung and S. H. Ko, Hierarchically Structured Conductive Polymer Binders with Silver Nanowires for High-Performance Silicon Anodes in Lithium-Ion Batteries, ACS Appl. Mater. Interfaces, 2022, 14(15), 17340–17347 CrossRef CAS PubMed.
- C. Liang, X. Sun, W. Su, Y. Hu and J. Duan, Fast welding of silver nanowires for flexible transparent conductive film by spatial light modulated femtosecond laser, Adv. Eng. Mater., 2021, 23, 2100584 CrossRef CAS.
- P. Li, H. Li, R. Wang, R. Miao, L. Qian, X. Feng, W. Wang, J. Li and W. Song, Realization of Highly Foldable Conductive Substrates with 2000 Cyclic Mechanical Stability through Silver Nanowires/Cellulose Structure Design, ACS Appl. Electron. Mater., 2021, 3(5), 2372–2379 CrossRef CAS.
- J. Jiu, J. Wang, T. Sugahara, S. Nagao, M. Nogi, H. Koga, K. Suganuma, M. Hara, E. Nakazawa and H. Uchida, The effect of light and humidity on the stability of silver nanowire transparent electrodes, RSC Adv., 2015, 5, 27657–27664 RSC.
- Y. Ahn, Y. Jeong and Y. Lee, Improved Thermal Oxidation Stability of Solution-Processable Silver Nanowire Transparent Electrode by Reduced Graphene Oxide, ACS Appl. Mater. Interfaces, 2012, 4, 6410–6414 CrossRef CAS PubMed.
- H. Wu, L. Hu, M. W. Rowell, D. Kong, J. J. Cha, J. R. Mcdonough, J. Zhu, Y. Yang, M. D. Mcgehee and Y. Cui, Electrospun Metal Nanofiber Webs as High-Performance Transparent Electrode, Nano Lett., 2010, 10, 4242–4248 CrossRef CAS PubMed.
- A. Cho, S. Kim, S. Kim, W. Cho, C. Park, F. S. Kim and J. H. Kim, Influence of Imidazole-Based Acidity Control of PEDOT:PSS on Its Electrical Properties and Environmental Stability, J. Polym. Sci., Part b: Polym. Phys., 2016, 54, 1530–1536 CrossRef CAS.
- L. Liu, Y. Jiang, J. Jiang, J. Zhou and Z. Xu, Flexible and Transparent Silver Nanowires Integrated with a Graphene Layer-Doping PEDOT: PSS Film for Detection of Hydrogen Sulfide, ACS Appl. Electron. Mater., 2021, 3, 4579–4586 CrossRef CAS.
- Y. Qin, L. Yao, F. Zhang, R. Li and Y. Chen, Highly Stable Silver Nanowires/Biomaterial Transparent Electrodes for Flexible Electronics, ACS Appl. Mater. Interfaces, 2022, 14, 38021–38030 CrossRef CAS PubMed.
- T. Bhargavi, N. M. Nair, A. Belavadi and P. Swaminathan, Fabrication of a Printed Heater using a Composite of Silver Nanowires and neutral PEDOT:PSS, IEEE
J. Flexible Electron., 2022 DOI:10.1109/JFLEX.2022.3224636.
- D. Y. Choi, H. W. Kang, H. J. Sung and S. S. Kim, Annealing-free, flexible silver nanowire–polymer composite electrodes via a continuous two-step spray-coating method, Nanoscale, 2013, 5, 977–983 RSC.
- T. Araki, J. Jiu, M. Nogi, H. Koga, S. Nagao, T. Sugahara and K. Suganuma, Low haze transparent electrodes and highly conducting air dried films with ultra-long silver nanowires synthesized by one-step polyol method, Nano Res., 2014, 7, 236–245 CrossRef CAS.
- X. Zhang, J. Shan, S. Bai, X. Guo, X. Zhao and H. Yang, A robust and flexible silver nanowire/silica sol/poly(3,4-ethylene dioxythiophene)/poly(styrene sulfonate) transparent conductive film for film heater, Thin Solid Films, 2022, 749, 139178 CrossRef CAS.
- Y. Noh, H. Jeong, J. Park and D. Lee, Charge-assisted coating of silver nanowire transparent conductive layer and application to flexible heater, Surf. Interfaces, 2022, 32, 102105 CrossRef CAS.
- H. Dong, S. Yu, L. Song, X. Wang and C. Wu, Fabrication of high-quality flexible transparent conductive thin films with a Nb2O5/AgNWs/Nb2O5 sandwich structure, Ceram. Int., 2022, 48, 15348–15354 CrossRef CAS.
- D. Li, L. Wang, W. Ji, H. Wang, X. Yue, Q. Sun, L. Li, C. Zhang, J. Liu and G. Lu, Embedding Silver Nanowires into a Hydroxypropyl Methyl Cellulose Film for Flexible Electrochromic Devices with High Electromechanical Stability, ACS Appl. Mater. Interfaces, 2021, 13, 1735–1742 CrossRef CAS PubMed.
- Y.-S. Kim, M.-H. Chang, E.-J. Lee, D.-W. Ihm and J.-Y. Kim, Improved electrical conductivity of PEDOT-based electrode films hybridized with silver nanowires, Synth. Met., 2014, 195, 69–74 CrossRef CAS.
- G. Wang, L. Bi, W. Wei, X. Zhang, Y. Gu, L. Huang, H. Yin, Y. Li, G. Chen, Z. Wu and C. Ye, Strongly Adhesive Silver Nanowire Ink Makes Delamination-Free Transparent Conductive Films Possible, ACS Appl. Nano Mater., 2019, 2, 6707–6714 CrossRef CAS.
- Y. Wang, X. Kong, J. Gao, M. Gong, X. Lin, L. Zhang, M. Guo and D. Wang, Customizable Stretchable Transparent Electrodes Based on AgNW/CNT Hybrids via Tailoring Sizes of Building Blocks, ACS Appl. Electron. Mater., 2022, 4, 1186–1195 CrossRef CAS.
- M. M. Menamparambath, K. Yang, H. H. Kim, O. S. Bae, M. S. Jeong, J. Y. Choi and S. Baik, Reduced haze of transparent conductive films by smaller diameter silver nanowires, Nanotechnology, 2016, 27, 465706 CrossRef PubMed.
|
This journal is © The Royal Society of Chemistry 2023 |
Click here to see how this site uses Cookies. View our privacy policy here.