DOI:
10.1039/D2FO02998G
(Paper)
Food Funct., 2023,
14, 1349-1356
Anti-obesity effect of vegetable juice fermented with lactic acid bacteria isolated from kimchi in C57BL/6J mice and human mesenchymal stem cells†
Received
7th October 2022
, Accepted 27th December 2022
First published on 3rd January 2023
Abstract
This study aimed to investigate the effect of fermented vegetable juice (VJ) obtained from a blend of four crops (Brassica oleracea var. capitata, B. oleracea var. italica, Daucus carota L., and Beta vulgaris) on adipogenesis along with the identification of active compounds. Two lactic acid bacteria (LAB) (Companilactobacillus allii WiKim39 and Lactococcus lactis WiKim0124), isolated from kimchi, were used to ferment the VJ and their effectiveness was evaluated in differentiated human mesenchymal stem cells and obese mice. In vitro antibody array analysis was done to understand signaling proteins in adipogenesis. Gene Ontology enrichment analysis showed that differentially expressed proteins are related to biological processes including immunological processes. These were effectively regulated by LAB and fermented VJ. Supplementation of fermented VJ reduced the weight gain, blood biochemical indicators, and liver fat accumulation in mice. Oil Red O staining indicated that the fermentation metabolites of VJ (indole-3-lactic acid, leucic acid, and phenyllactic acid) had an inhibitory effect on lipid accumulation in vitro. Therefore, it can be concluded that LAB-fermented VJ and its metabolites have the potential to counter obesity, and thus can be therapeutically effective.
1. Introduction
Many probiotic lactic acid bacteria (LAB) are reported to alleviate obesity and obesity-associated metabolic disorders. The proposed mechanisms of action include modulation of adipokines and immunometabolic parameters,1,2 increased expression of fatty acid oxidation genes, reduced lipid accumulation, altered serum profile, and changes in the gut microbiome.3 Paraprobiotics are known non-viable microorganisms or bacterial-free extracts that promote health by exhibiting bioactivities.4 Paraprobiotics include peptides, peptidoglycan-derived muropeptides, exopolysaccharides, cell surface-layer proteins, cell-secreted proteins, bacteriocins, and organic acids. The health-promoting effects of paraprobiotics include improved immune response, reduction in host inflammation-induced weight gain, and alleviation of hyperlipidemia.5
Kimchi, prepared from vegetables seasoned with spices, is a well-known fermented food in Korea.6 Several studies have been conducted on the health benefits of the bioactive metabolites of kimchi. LABs, isolated from kimchi, have been reported to exhibit beneficial properties like immunomodulation, antiobesity, antidiabetic, and anti-cancer.7 Moreover, exopolysaccharides synthesized by kimchi LAB show antioxidant activities.8 In addition, recently, we developed LAB fermented vegetable juices (VJ) from four local crops (cabbage (Brassica oleracea var. capitata), broccoli (B. oleracea var. italica), carrots (Daucus carota L.), and beetroot (Beta vulgaris)), preserved from a year prior, on the Jeju island, which had a negative impacting rural industries. LAB fermentation improved the quality of vegetable products by enhancing the nutritional quality and functional properties. VJ fermented with Companilactobacillus allii WiKim39 and Lactococcus lactis WiKim0124, isolated from kimchi, exhibited immunomodulatory functions9 and, improved the antioxidant properties. These health benefits are mainly due to the enhanced production of functional bioactive compounds such as phenolics (3-phenyllactic acid) and other organic acids (D-leucic and indole-3-lactic acids).10
Obesity, a global epidemic, influences more than 700 million people.11 It is associated with cardiovascular complications, diabetes, hypertension, and cancer.12 Probiotics have beneficial effects against obesity-related metabolic complications, including distorted lipid profiles and improve immune modulation.13 Some studies have demonstrated that the supplementation of prebiotics, which stimulates the growth and development of symbiotic microorganisms, is more effective in improving human health than the probiotics.14 Fermented foods and beverages, based on vegetable matrices, are widely reported for their health-promoting properties. However, these approaches and health claims lack scientific evidence due to the absence of relevant data.15
The present study aimed to (i) investigate the effect of fermented VJ on adipogenesis and cellular adipokine profile in mature human adipose-derived mesenchymal stem cells, (ii) exploring the antiobesity potential and its mechanism of action in high-fat diet-induced obese mice, and (iii) identify the active substances in LAB (C. allii WiKim39 and L. lactis WiKim0124) fermentation metabolites.
2. Materials and methods
2.1. Bacterial strains
Companilactobacillus allii WiKim39 (GenBank ID: NR_159087.1) and L. lactis WiKim0124 (GenBank ID: MZ424472.1), isolated from kimchi, were utilized in this study. The LAB strains were cryopreserved in De Man, Rogosa, and Sharpe (MRS) broth, containing 15% (v/v) glycerol, at −80 °C. Ahead of the experiment, the stock cultures were revived in MRS broth at 30 °C for 24 h. The bacterial cells were harvested via centrifugation (6000g, 10 min, 4 °C) and washed twice with phosphate buffered saline (PBS).
2.2. Preparation and fermentation of VJ
VJ was prepared as described in a previous study.10 Briefly, from the harvested crops of the Jeju island (Korea) in 2020, a mixture was prepared to contain 12% cabbage (B. oleracea var. capitata), 12% broccoli (B. oleracea var. italica), 12% carrots (D. carota L.), and 10% beetroot (B. vulgaris), and 54% purified water. The vegetable mixture was extracted with hot water (107 °C for 2 h) and °Brix of VJ samples was adjusted with food-grade glucose for optimal growth conditions. A total of 107 colony forming units (CFU) per mL of each strain (WiKim39 or WiKim0124) were inoculated into the VJ to initiate fermentation (30 °C for 48 h). For in vitro experiments, the fermented VJs were subjected to 95 °C for 10 min to eliminate the bacterial strains. This suspension was freeze-dried and stored at −80 °C. Non-fermented VJ was used as a control.
2.3. Cell culture and adipogenic differentiation
Human adipose-derived mesenchymal stem cells (hMSCs) were obtained from the American Type Culture Collection (PCS-500-011) and cultured at 37 °C and 5% CO2 in basal medium, prepared using the MesenCult proliferation kit (StemCell Technologies, Vancouver, Canada). To assess adipogenic differentiation, cells were harvested at passage three and cultured in MesenCult medium supplemented with the Adipogenic Differentiation Kit (StemCell Technologies, Vancouver, Canada) at a density of 1.5 × 104 cells per mL in 6-well plates, according to the manufacturer's instructions. The medium was changed every 2 d for 21 d. Adipogenesis differentiation was evaluated by Oil Red O staining as previously described.16 Briefly, cells were fixed in 10% formaldehyde in PBS, washed with PBS, stained with Oil Red O solution (Sigma-Aldrich, St Louis, MO, USA) for 10 min, and repeatedly rinsed with distilled water. Lipid droplets were extracted by incubating with 100% isopropanol for 15 min. The optical density (OD) of the solution was measured at 500 nm. D-Leucic acid (LA), indole-3-lactic acid (ILA), and 3-phenyllactic acid (PLA) standards were obtained from Sigma Aldrich (St Louis, MO, USA).
2.4.
In vitro cytotoxicity test
Bacterial cells were harvested, suspended in PBS, sonicated, and centrifuged as previously described.17 Supernatants of bacterial cell extracts were filter-sterilized (0.45 μm), lyophilized, and used to perform the anti-adipogenesis assay in hMSCs. To evaluate the cytotoxicity assay, 104 cells per well were plated in a 96-well plate and incubated for 48 h in a medium containing LAB lysates (concentration range of 0.5 to 5 mg mL−1) and fermented or non-fermented VJ samples (concentration range of 5 to 100 mg mL−1). For estimation of the active substances in fermented VJs, LA, ILA, and PLA were treated at concentration ranges of 0.63 to 40 mM. Cell viability was measured using 2,3-bis[2-methoxy-4-nitro-5-sulfophenyl]-2H-tetrazolium-5-carboxanilide disodium salt (XTT) assay, as described previously.18
2.5. Antibody array analysis
Fully matured hMSCs were collected and lysed. The lysates were centrifuged for 20 min at 15
000g, and supernatants were collected for the experiment. Protein concentration was estimated using the Bradford assay.19 For multiple protein detection, human obesity antibody array membrane (ab169819, Abcam, Cambridge, UK) was used according to the manufacturer's protocol. Briefly, the provided blocking buffer was added to the array membranes for 30 min. The membranes were then incubated with 1 mg of cell lysates/array membrane, followed by the addition of a biotinylated detection antibody. The membranes were incubated on a rocker with horseradish peroxidase-conjugated streptavidin (1
:
100). Spots on the array membranes were scanned and quantified by densitometry (Chemidoc, Bio-Rad, Hercules, CA, USA).
2.6. Bioinformatic analysis
Proteins with a log2 fold change of ≥|1| in the adipogenic differentiation group (CON) versus the pre-adipocytic group (NOR) were assigned as differentially expressed proteins (DEPs). Specific information on all proteins was obtained from the UniProt database (https://www.uniprot.org/). To evaluate functional enrichment, DEPs were searched against the Gene Ontology (GO) (https://geneontology.org/) database. Characteristics of DEPs were examined by GO enrichment analysis using the Biological Networks Gene Ontology (BiNGO) plug-in of Cytoscape.20 Fisher's exact test was used to determine the significance of GO term enrichment for multiple testing and the resulting p-value was adjusted with the false discovery rate (FDR) (cutoff < 0.05). Hierarchical clustering heatmap analysis of DEPs was generated with the “pheatmap” package in R (v3.3.2; https://www.r-project.org).
2.7. Animals
National Academy of Science of the National Institutes of Health (NIH) guidelines for “Guide for the Care and Use of Laboratory Animals” were observed for animal experiments. The animal care and protocols were reviewed and approved by the World Institute of Kimchi's Institutional Animal Care and Use Committee (WIKIM IACUC 202033). Six-week-old male C57BL/6J mice were obtained from Orient Bio (Seongnam, Korea). Mice were kept in ventilated polysulfone cages (200W × 318D × 145H (mm), four mice per cage) in a temperature and humidity-controlled facility with distilled water and food ad libitum. After 1 week, 56 mice were randomly distributed into seven groups (n = 8 per group) as follows: (i) normal control (NOR); mice received a standard pellet feed (Research Diet Inc., D10001, 10 kcal% fat) with distilled water, (ii) obesity-induced group (HFD); received a high-fat diet (Research Diet Inc., D12492, 60 kcal% fat) with distilled water, (iii) HFD with C. allii WiKim39 (WiKim39), (iv) HFD with L. lactis WiKim0124 (WiKim0124), (v) HFD with non-fermented VJ (VJ), (vi) HFD with C. allii WiKim39-fermented VJ (VJ + WiKim39), and (vii) HFD with L. lactis WiKim0124-fermented VJ (VJ + WiKim0124). Food intake and body weight were recorded weekly. All experimental groups were orally administered 10 mL per kg of body weight every second day for 8 w. At the end of the experiment, serum and tissue were collected and samples were stored at −80 °C until further use.
2.8. Dietary dosage information
Companilactobacillus allii WiKim39 and L. lactis WiKim0124 live cells were lyophilized after mass culture. The LAB powder was rehydrated in sterilized distilled water for 1 h at room temperature to a total dose of 1 × 109 CFU 200 μL−1 d−1 before oral gavage. The VJ treated groups (VJ, VJ + WiKim39, VJ + WiKim0124) received a daily dose of 250 mg per kg body weight. The VJ treatment group dose was calculated according to the human equivalent dose as follows: 250 mg kg−1 was estimated to be approximately 1600 mg per 60 kg adult person a day, equal to the consumption of 20 mL VJ when considering the freeze-dried yield.
2.9. Histological analysis
Liver and epididymal fat tissues were fixed in 10% neutral buffered formalin and histology was performed as described previously.9 Tissues stained with hematoxylin and eosin (H&E) were examined under an optical microscope (magnification, 20×; Olympus, Japan).
2.10. Plasma biochemical indicators
Plasma triglycerides (TG), total cholesterol (T-CHO), glucose, aspartate aminotransferase (AST), alanine aminotransferase (ALT), and alkaline phosphatase (ALP) levels were determined using a FUJI DRI-CHEM 7000 (Fujifilm, Tokyo, Japan), following the manufacturer's instructions. The concentration of leptin was measured using the Bio-Plex Pro Mouse Diabetes-Plex Assay (Bio-Rad, USA).
2.11. Quantitative reverse transcription-polymerase chain reaction (qRT-PCR)
Epididymal fat tissue (0.1 g) was homogenized with a bead-beating grinder and lysis system (cycle 2, FastPrep-24; MPBIO, Seoul, Korea). RNA was extracted using the RNeasy kit (Qiagen, Valencia, CA, USA). Approximately 10 ng of RNA was used for qRT-PCR amplification with a QuantiFast SYBR Green RT-PCR Kit (Qiagen, Valencia, CA, USA). The reaction conditions were as follows: reverse transcription at 50 °C for 10 min, activation step at 95 °C for 5 min, denaturation at 95 °C for 10 s, and annealing and extension at 60 °C for 30 s for 35 cycles. The result was normalized to that of beta-actin.
2.12. Statistical analysis
The statistical significance was analyzed using Tukey's honest significant difference (HSD) test performed with the “agricolae” package of R for group comparisons. Results with p < 0.05 were considered statistically significant. All experiments were performed in triplicate, and results were expressed as mean ± standard deviation (SD).
3. Results
3.1. Effects of LAB fermented VJ on the differentiated hMSCs and adipogenic proteins
To investigate the effect of LAB on cell viability, hMSC preadipocytes were incubated with varying concentrations of LAB lysates and fermented VJ products. Treatment with 2 mg mL−1 of LAB lysate and 20 mg mL−1 of VJ did not cause any cytotoxicity (ESI Fig. S3†). To investigate the anti-adipogenic effect, lipid accumulation was measured using the Oil-Red O staining assay. The treatment group showed reduced lipid accumulation in hMSCs indicating the maximum effectiveness of C. allii WiKim39 lysates, followed by a subsequent reduction in the effectiveness after treatment with L. lactis WiKim0124 lysates, C. allii WiKim39 fermented VJ, L. lactis WiKim124 fermented VJ, and non-fermented samples (ESI Fig. S3†). Based on this result, further treatments were administered at these concentrations to investigate the anti-adipogenic mechanism of LAB-fermented VJ. Antibody array analysis was performed on fully matured hMSCs to simultaneously identify 62 proteins related to adipogenesis. We selected 38 DEPs with log2 fold changes ≥|1| between the differentiated hMSCs and experimental groups. The array map and profile are shown in ESI Fig. S1.† The heat map of the DEPs and cluster analysis are shown in Fig. 1a. The expression of each DEP was clustered according to the experimental groups, indicating that DEPs had distinctly different expression patterns. We found that most proteins were upregulated compared to that in NOR and downregulated compared to that CON in the LAB and LAB-fermented VJ treatment groups. C. allii WiKim39 and C. allii WiKim39 fermented VJs were able to suppress the expression of most proteins. Bioinformatic analysis was done to elucidate the change in the DEPs. For GO enrichment analysis, the DEPs of the treatment groups were compared to the CON (Fig. 1b and c). GO enrichment analysis indicated molecular function (MF) and biological process (BP); LAB or LAB-fermented VJ groups were associated with the activation of inflammatory response pathways such as cytokine activity, chemokine activity, immune system process, and positive response to a stimulus. BP related to the immune and stimulation regulation were significantly enriched in VJ + WiKim39 and WiKim39 compared to the HFD group. In contrast, they showed mild enrichment in the VJ group compared to the others.
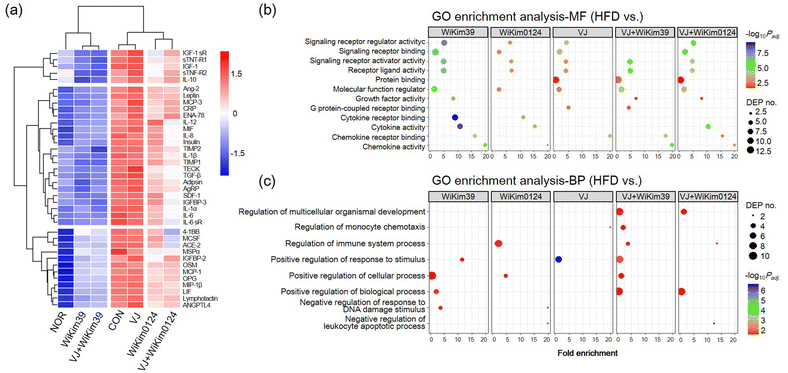 |
| Fig. 1 Analysis of the antibody array. (A) Hierarchical cluster analysis of differentially expressed proteins (DEPs). A total of 38 proteins are visualized on the heat map. Different colors represent the relative abundance of proteins, where red represents a higher intensity and blue represents a lower intensity. (B and C) Scatter plots of enriched GO pathways relevant to biological processes and molecular function. GO classification of DEPs from the control (CON) vs. experimental groups. GO, gene ontology; MF, molecular function; BP, biological process. | |
3.2. Effects of LAB and LAB fermented VJs administration on body weight, plasma lipid profile, and adipocyte size in mice
To investigate the inhibitory effect of LAB and fermented VJ on lipid accumulation, an HFD-induced obesity mouse model was established, as described previously. As shown in Fig. 2 and Table 1: (i) all the treatments resulted in a reduction in the body (Fig. 2a), liver, spleen, and white adipose tissue weight gain (Table 1). Throughout the experiment for 8 w, the weights increased in the HFD mouse group and the weight gain was kept in check by the treatments. However, no significant differences were observed amongst the treatments on the extent of weight gain. The same trend was observed for TG and TCHO (Fig. 2b–d), (ii) in the LAB treatment group, the H&E staining results indicated that epididymal adipose and liver tissue and the adipocyte area looked similar to the NOR group (Fig. 2e–g), and (iii) in glucose treatment, the main differences appeared in fermented VJ treatment group (Fig. 2h), (iv) for all the treatments, the insulin values were equivalent to NOR values for insulin treatment (Fig. 2i) and reduced leptin level during treatment (Fig. 2j). Consistent with these results, an increase in the levels of plasma AST, ALT, ALP, liver-free fatty acids (FFA), TG, and TCHO were observed (ESI Fig. S2†). LAB and fermented VJ administration effectively ameliorated plasma lipid index and hepatic lipid accumulation. This result was like the NOR group but not the VJ-fed group. Plasma ALP, plasma FFA, hepatic TG, and hepatic TCHO showed the lowest levels in the fermented VJ-supplemented groups.
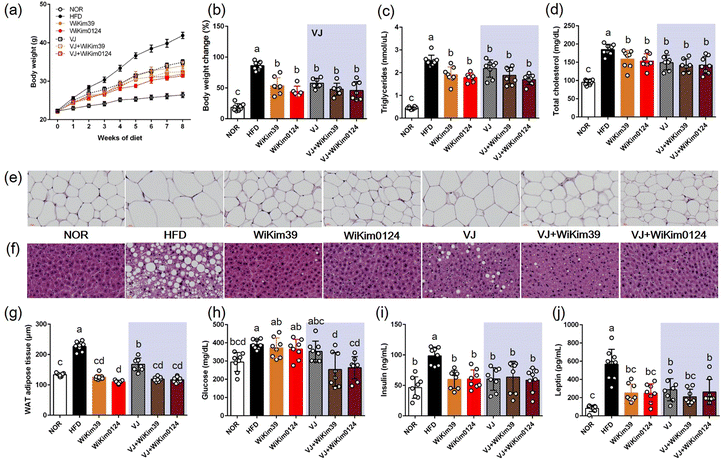 |
| Fig. 2 Effects of WiKim39 and WiKim0124 fermented vegetable juice on high-fat diet-induced obese mice. (a) Body weight, (b) change in body weight over the experiment duration (8 w) (weight at 8 w/weight at 0 w) × 100 − 100), (c) plasma triglycerides, (d) total plasma cholesterol, (e) H&E staining of epididymal adipose tissue (white adipose tissue) sections (40×), (f) H&E staining of liver tissue sections (40×), (g) average white adipose tissue adipocyte area (quantified under microscope, n = 10), (h) plasma glucose, (i) plasma insulin, and (j) plasma leptin. Different letters denote significant differences (p < 0.05, ANOVA, Tukey's HSD). Values are expressed as mean ± SD; H&E is hematoxylin and eosin stain. | |
Table 1 Organ and adipose tissue weights in mice fed with high-fat diet and LAB-fermented VJs for 8 weeks
(g) |
NOR |
HFD |
WiKim39 |
WiKim0124 |
VJ |
VJ + WiKim39 |
VJ + WiKim0124 |
Values are given as mean ± standard deviation (n = 8). Different superscripts in the same row are significantly different (p < 0.05, ANOVA, Tukey-HSD). |
Liver |
1.12 ± 0.06b |
1.44 ± 0.12a |
1.05 ± 0.05b |
0.92 ± 0.02b |
1.09 ± 0.07b |
1.08 ± 0.07b |
1.05 ± 0.07b |
Spleen |
0.06 ± 0.01b |
0.09 ± 0.00a |
0.08 ± 0.01a |
0.08 ± 0.00a |
0.09 ± 0.01a |
0.07 ± 0.01a |
0.08 ± 0.01a |
Epididymal fat |
0.52 ± 0.03c |
2.99 ± 0.06a |
2.13 ± 0.23b |
1.95 ± 0.26b |
2.28 ± 0.12b |
1.92 ± 0.13b |
1.89 ± 0.17b |
Visceral fat |
0.44 ± 0.02c |
2.88 ± 0.21a |
1.38 ± 0.18b |
1.22 ± 0.18b |
1.63 ± 0.14b |
1.33 ± 0.15b |
1.25 ± 0.13b |
3.3. Expression of adipogenic transcription factors and related genes
To elucidate the mechanism of the antiobesity effect of LAB and LAB fermented VJ in obesity induced in mice by a high-fat diet, the expression of transcription factors and the related genes involved in adipogenesis inhibition in epididymal white adipose tissue (Fig. 3) was measured using qRT-PCR. As expected, the mRNA expression of sterol regulatory element binding protein-1c (SREBP-1c), CCAAT/enhancer-binding protein-α (C/EBP-α), proliferator-activated receptor γ (PPAR-γ), fatty-acid-binding protein 4 (FABP4), and fatty acid synthetase (FAS) were significantly increased in HFD compared to NOR. However, dietary administration of LAB and LAB-fermented VJ significantly reduced the expression of adipogenesis regulators. Most of these genes were downregulated in the LAB-administered group compared to the fermented VJ groups. Additionally, the activities of the key lipolytic enzymes, i.e., adipose triglyceride lipase (ATGL) and hormone-sensitive lipase (HSL), were increased in all the treatments except VJ. VJ did not show a significant difference compared to HFD. LAB-fermented VJ showed an increased level of ATGL. VJ + WiKim39 and VJ + WiKim0124 showed efficacy like LAB alone treatment. Therefore, the results indicate that fermented VJ reduced adipogenesis by regulating adipogenic genes, regulators, and lipolytic enzymes, though only VJ treatment was not as effective as fermented VJ treatment.
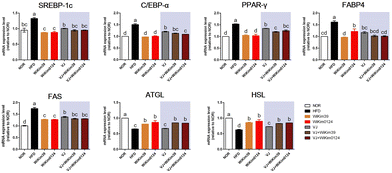 |
| Fig. 3 Effects of WiKim39 and WiKim0124 fermented vegetable juices on the expression of genes related to lipid metabolism in the epididymal adipose tissues of high-fat diet-induced obese mice. The mRNA expression of SREBP-1c, C/EBP-α, PPAR-γ, FABP4, FAS, ATGL, and HSL measured using quantitative real-time polymerase chain reaction. Different letters denote significant differences (p < 0.05, ANOVA, Tukey's HSD). Values were expressed as the mean ± SD (n = 3). | |
3.4. Inhibition of intracellular lipid accumulation of LAB fermented VJ metabolites in hMSCs
To explore functional bioactive ingredients in the fermented VJs, we examined the effects of LA, ILA, and PLA on adipogenic differentiated hMSCs. No significant cytotoxicity was observed when cells were treated with concentrations of up to 20 mM of the three compounds. Matured hMSCs were treated with various concentrations of LA, ILA, and PLA. The lipid droplet accumulation reduced significantly within the cells of all the treatment groups compared to the untreated and differentiated cells. The inhibitory effect was concentration-dependent (Fig. 4). A noticeably higher effect was observed in ILA compared to PLA or LA.
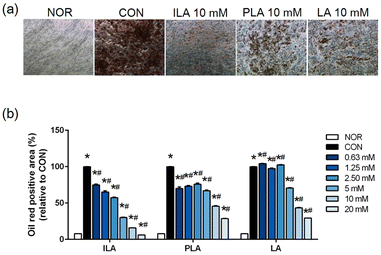 |
| Fig. 4 Effects of potential anti-adipogenic agents present in vegetable juice fermented products on human mesenchymal stem cells. (a) Microscopy after Oil Red O staining and (b) quantified by absorbance at 517 nm. NOR, undifferentiated hMSCs; CON, adipogenic differentiated; 0.63 mM–20 mM, differentiated adipocytes with each single compound according to concentration (ILA: indole-3-lactic acid, PLA: phenyllactic acid, LA: leucic acid). *p < 0.05 indicates statistically significant differences between NOR and experimental groups, and #p < 0.05 indicates statistically significant differences between CON and experimental groups. Values are expressed as the mean ± SD (n = 3). | |
4. Discussion
Adipokines are grouped into a diverse group of proteins that play roles in blood pressure, lipid metabolism, appetite and energy balance, insulin sensitivity, immunity, and inflammation.21 Adipokines released from adipose tissue induce systemic inflammation and this pathway can be a therapeutic target for metabolic diseases.22 Our previous study revealed that LAB fermented VJ has anti-inflammatory potential.9 Consistent with those results, in the current study, treatment of VJ fortified with functional LAB derived from kimchi alleviated lipid accumulation in adipocyte-differentiated hMSCs through cytokine modulation. Antibody array results under LAB lysate and LAB fermented VJ treatment indicated that the majority of DEPs were associated with immunomodulatory response categories. With MF and BP functional groups, GO enrichment analysis further showed that DEPs mainly participated in signaling receptor regulation activity, receptor ligand activity, and G protein-coupled receptor binding in response to negative regulation of DNA damage stimulus and negative regulation of the leukocyte apoptotic process. G protein-coupled receptors induce activation of the mitogen-activated protein kinases (MAPKs).23 MAPKs such as extracellular signal-regulated kinase (ERK), p38, and Jun N-terminal kinase (JNK) are widely-recognized to regulate embryogenesis, cell differentiation, cell proliferation, and cell death. They are also known as enhancers of adipogenesis and lipid accumulation.24 This linkage of G protein-coupled receptors with MAPKs may have a synergistic effect on the regulated production of proinflammatory adipokines.
Considering these results, LAB and VJ fortified with LAB were further tested in HFD-induced obese mice. The daily dosage was determined as 109 CFU per day of LAB or 250 mg per kg body weight of VJ samples. LAB and LAB fermented VJs treatments substantially reduced body weight gain, decreased body fat mass, suppressed the plasma lipid concentration index through down-regulation of SREBP-1c, C/EBPα, PPARγ, FABP4, and FAS adipogenic genes and up-regulation of ATGL, and HSL lipolytic genes. Free fatty acids were subsequently released and adipocyte size decreased. Probiotics and prebiotics are microbiome managing materials for improving host health. They can also impact health-promoting properties of foods through the production of functionally bioactive substances during fermentation.25 In this study, we demonstrated the effect of LAB in mice and cells delivered alone or in a food matrix under different treatment conditions. Inflammation control by surface proteins or cell wall components (exopolysaccharide or lipopolysaccharides) has been previously reported when bacteria were administered into cells or mice.26,27 However, the effects of food matrix are not discussed in-depth in food microbiology and nutritional and physiological science. LAB with appropriate food matrices can improve bacterial cell growth and extend potency. The development of symbiotic combinations is another approach to stimulate LAB growth and efficacy.28 The bioavailability of phenolic compounds can be enhanced by the metabolism of LAB in a food matrix, thus, increasing their functionality.29 In a previous study, we compared the VJ + WiKim39 and VJ + WiKim0124 metabolites to non-fermented VJ and profiled them using ultra-performance liquid chromatography quadrupole time-of-flight tandem mass spectrometry (UPLC-QTOF-MS/MS). Some valid metabolites/molecules such as organic acids, phenols, and amino acids were identified. LA, ILA, and PLA exhibited the most significant antioxidant potential.10 We hypothesized that these molecules present in the VJ could exert a synergistic effect on anti-obesity efficacy through functional LAB fermentation. As expected, LA, ILA, and PLA inhibited lipid accumulation in adipogenic differentiated hMSCs and showed the highest efficacy in ILA treatment. ILA is an indole derived from tryptophan metabolism in the gut microbiota.30 They play critical roles in innate immunity, reducing inflammation, and scavenging free radicals. Indoles can also suppress insulin secretion and appetite, and slow gastric emptying.31 LA (2-hydroxyisocaproic acid) and PLA are derived from L-leucine and L-phenylalanine, respectively. They are LAB-produced metabolites and are gaining interest because of their antimicrobial potential and other pharmacological properties.32 Unlike other natural organic acids, PLA does not have a pronounced effect on food taste. Therefore, PLA is a promising natural additive to extend the shelf life of food.33 Immunomodulatory effects of PLA and LA have been implicated in the consumption of fermented foods.34,35 The contribution of immune cells to metabolic diseases has shifted focus to adipose tissue-associated cells. However, these potential causes affect other metabolic regulatory tissues and promote inflammation and obesity comorbidities.36 VJ fermented with WiKim39 and WiKim0124 suppressed lipid accumulation and improved an anti-obesogenic effect. These results suggest that LAB-fermented VJ metabolites can be a source of functional bioactive substances. Since the functionality of fermented food is determined by the sum of all effective metabolites, the use of a starter can provide the functionality to the fermented foods.37 Future studies should focus on identifying these substances and mechanisms associated with obesity and should consider obesity-related inflammation.
5. Conclusions
VJ fermented with WiKim39 and WiKim0124 effectively ameliorated fat accumulation in vitro and in vivo. We propose ILA, PLA, and LA as potential bioactive compounds that exhibit inhibitory effects on lipid accumulation in vitro. These findings demonstrate that C. allii WiKim39 and L. lactis WiKim0124 isolated from kimchi can be used as functional starters for plant-based foods and lay the foundation for functional probiotic beverages to prevent obesity progression.
Author contributions
M. Lee: Writing – original draft preparation, conceptualization, investigation. Y.-R. Yun: Conceptualization. E. J. Choi: Conceptualization. J. H. Song: Conceptualization. J. Y. Kang: Conceptualization. D. Kim: Conceptualization. K. W. Lee: Conceptualization, writing – reviewing and editing. J. Y. Chang: Project administration, conceptualization, writing – reviewing and editing.
Conflicts of interest
There are no conflicts to declare.
Acknowledgements
This research was supported by a grant from the World Institute of Kimchi (KE2202-1) funded by the Ministry of Science and ICT Republic of Korea.
References
- E. Fabersani, M. C. Abeijon-Mukdsi, R. Ross, R. Medina, S. González and P. Gauffin-Cano, Specific strains of lactic acid bacteria differentially modulate the profile of adipokines in vitro, Front. Immunol., 2017, 8, 266 CrossRef PubMed.
- E. Fabersani, M. Russo, A. Marquez, C. Abeijón-Mukdsi, R. Medina and P. Gauffin-Cano, Modulation of intestinal microbiota and immunometabolic parameters by caloric restriction and lactic acid bacteria, Food Res. Int., 2019, 124, 188–199 CrossRef CAS PubMed.
- D. K. Dahiya, Renuka, M. Puniya, U. K. Shandilya, T. Dhewa, N. Kumar, S. Kumar, A. K. Puniya and P. Shukla, Gut microbiota modulation and its relationship with obesity using prebiotic fibers and probiotics: a review, Front. Microbiol., 2017, 8, 563 CrossRef PubMed.
- P. F. Cuevas-González, A. M. Liceaga and J. E. Aguilar-Toalá, Postbiotics and paraprobiotics: From concepts to applications, Food Res. Int., 2020, 136, 109502 CrossRef PubMed.
- E. Kim, H. G. Lee, S. Han, K. H. Seo and H. Kim, Effect of surface layer proteins derived from paraprobiotic kefir lactic acid bacteria on inflammation and high-fat diet-induced obesity, J. Agric. Food Chem., 2021, 69, 15157–15164 CrossRef CAS PubMed.
- M. Lee, J. H. Song, W. B. Shim and J. Y. Chang, DNAzyme-based quantitative loop-mediated isothermal amplification for strain-specific detection of starter kimchi fermented with Leuconostoc mesenteroides WiKim32, Food Chem., 2020, 333, 127343 CrossRef CAS PubMed.
- M. E. Lee, J. Y. Jang, J. H. Lee, H. W. Park, H. J. Choi and T. W. Kim, Starter cultures for kimchi fermentation, J. Microbiol. Biotechnol., 2015, 25, 559–568 CrossRef CAS PubMed.
- I. S. Choi, S. H. Ko, M. E. Lee, H. M. Kim, J. E. Yang, S. G. Jeong, K. H. Lee, J. Y. Chang, J. C. Kim and H. W. Park, Production, characterization, and antioxidant activities of an exopolysaccharide extracted from spent media wastewater after Leuconostoc mesenteroides WiKim32 fermentation, ACS Omega, 2021, 6, 8171–8178 CrossRef CAS PubMed.
- Y. R. Yun, M. Lee, J. H. Song, E. J. Choi and J. Y. Chang, Immunomodulatory effects of Companilactobacillus allii WiKim39 and Lactococcus lactis WiKim0124 isolated from kimchi on lipopolysaccharide-induced RAW264. 7 cells and dextran sulfate sodium-induced colitis in mice, J. Funct. Foods, 2022, 90, 104969 CrossRef CAS.
- M. Lee, J. H. Song, E. J. Choi, Y. R. Yun, K. W. Lee and J. Y. Chang, UPLC-QTOF-MS/MS and GC-MS characterization of phytochemicals in vegetable juice fermented using lactic acid bacteria from kimchi and their antioxidant potential, Antioxidants, 2021, 10, 1761 CrossRef CAS PubMed.
- L. Li, Y. Zhang, J. R. Speakman, S. Hu, Y. Song and S. Qin, The gut microbiota and its products: Establishing causal relationships with obesity related outcomes, Obes. Rev., 2021, 22, e13341 CAS.
- A. M. Gil-Rodríguez and T. P. Beresford, Lipase inhibitory activity of skim milk fermented with different strains of lactic acid bacteria, J. Funct. Foods, 2019, 60, 103413 CrossRef.
- C. Tang, L. Kong, M. Shan, Z. Lu and Y. Lu, Protective and ameliorating effects of probiotics against diet-induced obesity: A review, Food Res. Int., 2021, 147, 110490 CrossRef CAS PubMed.
- H. S. Ejtahed, P. Angoorani, A. R. Soroush, R. Atlasi, S. Hasani-Ranjbar, A. M. Mortazavian and B. Larijani, Probiotics supplementation for the obesity management; A systematic review of animal studies and clinical trials, J. Funct. Foods, 2019, 52, 228–242 CrossRef CAS.
- I. A. Al Kassaa, M. Hamze, D. Hober, N. E. Chihib and D. Drider, Identification of vaginal lactobacilli with potential probiotic properties isolated from women in North Lebanon, Microb. Ecol., 2014, 67, 722–734 CrossRef PubMed.
- W. Deng, H. Chen, H. Su, X. Wu, Z. Xie, Y. Wu and H. Shen, IL6 receptor facilitates adipogenesis differentiation of human mesenchymal stem cells through activating P38 pathway, Int. J. Stem Cells, 2020, 13, 142–150 CrossRef CAS PubMed.
- J. Lee, J. Y. Jang, M. S. Kwon, S. K. Lim, N. Kim, J. Lee, H. K. Park, M. Yun, M. Y. Shin, H. E. Jo, Y. J. Oh, B. H. Ryu, M. Y. Ko, W. Joo and H. J. Choi, Mixture of two Lactobacillus plantarum strains modulates the gut microbiota structure and regulatory T cell response in diet–induced obese mice, Mol. Nutr. Food Res., 2018, 62, 1800329 CrossRef PubMed.
- S. Kamiloglu, G. Sari, T. Ozdal and E. Capanoglu, Guidelines for cell viability assays, Food Front., 2020, 1, 332–349 CrossRef.
- M. M. Bradford, A rapid and sensitive method for the quantitation of microgram quantities of protein utilizing the principle of protein-dye binding, Anal. Biochem., 1976, 72, 248–254 CrossRef CAS PubMed.
- S. Maere, K. Heymans and M. Kuiper, BiNGO: a Cytoscape plugin to assess overrepresentation of gene ontology categories in biological networks, Bioinformatics, 2005, 21, 3448–3449 CrossRef CAS PubMed.
- A. Aguilar-Valles, W. Inoue, C. Rummel and G. N. Luheshi, Obesity, adipokines and neuroinflammation, Neuropharmacology, 2015, 96, 124–134 CrossRef CAS PubMed.
- V. Z. Rocha and P. Libby, Obesity, inflammation, and atherosclerosis, Nat. Rev. Cardiol., 2009, 6, 399–409 CrossRef CAS PubMed.
- M. Lopez-Ilasaca, P. Crespo, P. G. Pellici, J. S. Gutkind and R. Wetzker, Linkage of G protein-coupled receptors to the MAPK signaling pathway through PI 3-kinase γ, Science, 1997, 275, 394–397 CrossRef CAS PubMed.
- M. J. Seo, Y. J. Lee, J. H. Hwang, K. J. Kim and B. Y. Lee, The inhibitory effects of quercetin on obesity and obesity-induced inflammation by regulation of MAPK signaling, J. Nutr. Biochem., 2015, 26, 1308–1316 CrossRef CAS PubMed.
- M. E. Sanders, D. J. Merenstein, G. Reid, G. R. Gibson and R. A. Rastall, Probiotics and prebiotics in intestinal health and disease: from biology to the clinic, Nat. Rev. Gastroenterol. Hepatol., 2019, 16, 605–616 CrossRef PubMed.
- O. Chipashvili, D. R. Utter, J. K. Bedree, Y. Ma, F. Schulte, G. Mascarin, Y. Alayyoubi, D. Chouhan, M. Hardt, F. Bidlack, H. Hasturk, X. He, J. S. McLean and B. Bor, Episymbiotic Saccharibacteria suppresses gingival inflammation and bone loss in mice through host bacterial modulation, Cell Host Microbe, 2021, 29, 1649–1662 CrossRef CAS PubMed.
- M. Hamidi, O. V. Okoro, G. Ianiri, H. Jafari, K. Rashidi, S. Ghasemi, R. Castoria, D. Palmieri, C. Delattre, G. Pierre, M. Mirzaei, L. Nie, H. Samadian and A. Shavandi, Exopolysaccharide from the yeast Papiliotrema terrestris PT22AV for skin wound healing, J. Adv. Res., 2022, S2090–1232 Search PubMed.
- T. C. Pimentel, W. K. A. D. da Costa, C. E. Barão, M. Rosset and M. Magnani, Vegan probiotic products: A modern tendency or the newest challenge in functional foods, Food Res. Int., 2021, 140, 110033 CrossRef CAS PubMed.
- S. G. G. Morais, G. da Silva Campelo Borges, M. Dos Santos Lima, O. Martín-Belloso and M. Magnani, Effects of probiotics on the content and bioaccessibility of phenolic compounds in red pitaya pulp, Food Res. Int., 2019, 126, 108681 CrossRef CAS PubMed.
- M. Qayed, D. Michonneau, G. Socié and E. K. Waller, Indole derivatives, microbiome and graft versus host disease, Curr. Opin. Immunol., 2021, 70, 40–47 CrossRef CAS PubMed.
- J. Geng, Q. Ni, W. Sun, L. Li and X. Feng, The links between gut microbiota and obesity and obesity related diseases, Biomed. Pharmacother., 2022, 147, 112678 CrossRef CAS PubMed.
- R. V. Rajanikar, B. H. Nataraj, H. Naithani, S. A. Ali, N. R. Panjagari and P. V. Behare, Phenyllactic acid: a green compound for food biopreservation, Food Control, 2021, 128, 108184 CrossRef CAS.
- J. J. Xu, J. Z. Sun, K. L. Si and C. F. Guo, 3-Phenyllactic acid production by Lactobacillus crustorum strains isolated from naturally fermented vegetables, LWT–Food Sci. Technol., 2021, 149, 111780 CrossRef CAS.
- I. F. Maha, X. Xie, S. Zhou, Y. Yu, X. Liu, A. Zahid, Y. Lei, R. Ma, F. Yin and D. Qian, Skin metabolome reveals immune responses in yellow drum Nibea albiflora to Cryptocaryon irritans infection, Fish Shellfish Immunol., 2019, 94, 661–674 CrossRef CAS PubMed.
- A. Peters, P. Krumbholz, E. Jäger, A. Heintz-Buschart, M. V. Çakir, S. Rothemund, A. Gaudl, U. Ceglarek, T. Schöneberg and C. Stäubert, Metabolites of lactic acid bacteria present in fermented foods are highly potent agonists of human hydroxycarboxylic acid receptor 3, PLoS Genet., 2019, 15, e1008145 CrossRef CAS PubMed.
- R. Liu and B. S. Nikolajczyk, Tissue immune cells fuel obesity-associated inflammation in adipose tissue and beyond, Front. Immunol., 2019, 10, 1587 CrossRef CAS PubMed.
- S. J. Lee, H. S. Jeon, J. Y. Yoo and J. H. Kim, Some important metabolites produced by lactic acid bacteria originated from kimchi, Foods, 2021, 10, 2148 CrossRef CAS PubMed.
|
This journal is © The Royal Society of Chemistry 2023 |
Click here to see how this site uses Cookies. View our privacy policy here.