DOI:
10.1039/D2FO02710K
(Communication)
Food Funct., 2023,
14, 1338-1348
β(2 → 1)-β(2 → 6) branched graminan-type fructans and β(2 → 1) linear fructans impact mucus-related and endoplasmic reticulum stress-related genes in goblet cells and attenuate inflammatory responses in a fructan dependent fashion†
Received
13th September 2022
, Accepted 13th January 2023
First published on 16th January 2023
Abstract
Dietary fibers such as fructans have beneficial effects on intestinal health but it is unknown whether they impact goblet cells (GCs). Here we studied the effects of inulin-type fructans (ITFs) and graminan-type fructans (GTFs) with different molecular weights on mucus- and endoplasmic reticulum (ER) stress-related genes in intestinal GCs. To that end, GCs were incubated in the presence of ITFs or GTFs, or ITFs and GTFs + TNFα or the N-glycosylation inhibitor tunicamycin (Tm). IL-8 production by GCs was studied as a marker of inflammation. Effects between ITFs and GTFs were compared. We found a beneficial impact of GTFs especially on the expression of RETNLB. GTF II protects from the TNFα-induced gene expression dysregulation of MUC2, TFF3, GAL3ST2, and CHST5. Also, all the studied fructans prevented Tm-induced dysregulation of GAL3ST2. Interestingly, only the short chain fructans ITF I and GTF I have anti-inflammatory properties on GCs. All the studied fructans except ITF I decreased the expression of the ER stress-related HSPA5 and XBP1. All these benefits were fructan-structure and chain length dependent. Our study contributes to a better understanding of chemical structure-dependent beneficial effects of ITFs and GTFs on gut barrier function, which could contribute to prevention of gut inflammatory disorders.
1. Introduction
The intestinal epithelium is covered by a mucus layer which serves as the first barrier against the harmful content of the lumen.1 This mucus is formed by a film mainly constituted by mucins.2 Mucin-2 (encoded by MUC2) is the most abundant mucin in the gut. This large multimeric protein undergoes posttranslational modifications such as O- and N-glycosylation and sulfation, conferring MUC2 a highly viscous gel-like consistency.3,4 MUC2, along with proteins such as trefoil factor-3 (encoded by TFF3),5 resistin-like molecule β (encoded by RETNLB)6 and other antimicrobial peptides form an organized structure similar to a net and provide a semi-permeable barrier between the intestinal epithelium and the lumen which allows the passage of small molecules while it hampers the translocation of microorganisms.7
Interweaved between enterocytes and the other components of the intestinal epithelium are goblet cells (GCs), which are mucus-synthesizing specialized cells.8 Due to MUC2 dual expression (constitutive and regulated), GCs possess and require a highly active protein trafficking from the endoplasmic reticulum (ER) where its folding occurs, and where glycosylation and sulfation occurs, to its final site of action.9 In these last posttranslational sulfation modifications galactose-3-O-sulfotransferase (encoded by GAL3ST2), and carbohydrate sulfotransferase (encoded by CHST5) are implicated.10,11
In GCs under stress, unfolded protein response (UPR) activation might occur due to inefficient protein folding in the ER.12 The activation of this group of intracellular signaling pathways can drive GCs to stress responses or toward apoptosis when malfunction is irreversible.13,14 Genes such as HSPA5 and XBP1 participate in this UPR.15HSPA5 codes for the ER chaperone BiP, member 5 of the heat shock protein 70 (Hsp70) family. BiP acts mainly as a sensor of unfolded proteins activating the UPR and the subsequent downstream signaling.16 The XBP1 gene codes for the X-box binding protein 1, a transcription factor that belongs to the inositol-requiring enzyme 1α(IRE1α)-signaling axis.17 Inflammation can also activate ER stress.18 Intestinal inflammatory disorders such as inflammatory bowel disease (IBD) have been related to ER stress.19 Under these inflammatory conditions, impairment of GCs function such as aberrant mucin folding has been observed.20
Chronic consumption of Western diet, characterized by low intake of dietary fibers, favor the development of intestinal inflammatory disorders,21–24 whereas, regular consumption of dietary fibers has been associated to a low inflammatory state and to a strengthened barrier function of the mucus layer.25–27
An important group of dietary fibers are fructans. Fructans are mainly plant-derived water-soluble oligo and polysaccharides.28,29 Fructans from chicory plant are broadly used in Europe in the food industry.30 Chicory fructans are composed of fructofuranose molecules linked by β(2 → 1) bonds with a glucose moiety at the reducing end, which confers them a linear structure.31 These chicory fructans are known as inulin-type fructans (ITFs).32 In Latin America, fructans from agave plants are used as fiber supplements in foods.33,34 These agave fructans are structurally different from ITFs since besides β(2 → 1) fructose chains, they also contain fructose units linked by β(2 → 6) bonds.35 These β(2 → 1)-β(2 → 6) bonds gives them a branched complex structure.36,37 This type of fructans are named graminan-type fructans (GTFs).38
It is unknown whether ITFs and GTFs have an impact in GCs on mucus-related genes in presence and absence of inflammatory or ER-stress. Therefore, in this study we aimed to determine whether ITFs and GTFs have an influence on the expression of mucus-related and ER stress-related genes in GCs. We also tested if ITFs and GTFs were capable to protect, and attenuate inflammatory responses of GCs exposed to disruptor agents.
2. Materials & methods
2.1 Fructans
To study the effect of fructans of different sizes with a branched or linear structure, on the expression of mucus-related and ER stress-related genes in gut GCs, β(2 → 1)–β(2 → 6) GTFs and β(2 → 1) ITFs were used. GTFs were extracted from Agave tequilana Weber blue variety and were provided by Nekutli™, Guadalajara, Mexico. ITFs Frutafit™ CLR and Frutafit™ TEX! were studied as well. Both ITFs were provided by Sensus™ (B.V. Roosendaal, The Netherlands). These fructans have been previously studied by our group.39–42
2.2 Cell culture
To study the effects of fructans on the expression of mucus-related and ER stress-related genes in gut GCs, the human colorectal adenocarcinoma-derived LS174T cell line, which exhibits a GC-like phenotype (ATCC® CL188™, Manassas, USA) was used. GCs were maintained in T-75 flasks at 37 °C in humidified air and 5% CO2 and cultured in Eagle Minimum Essential Medium (EMEM, Lonza, MD, USA) supplemented with 10% heat-inactivated fetal bovine Serum (Sigma-Aldrich, Zwijndrecht, The Netherlands), 2 mM L-glutamine (Lonza, MD, USA) and penicillin/streptomycin 50 U mL−1 and 50 μg mL−1 (Gibco, Paisley, UK). When reached ∼80% confluency and after treatment with 10% trypsin-EDTA (Sigma-Aldrich, Zwijndrecht, The Netherlands), GCs were passaged. GCs were used for experiments between passages 30–40.
2.3 Effect of ITFs and GTFs on the expression of intestinal mucus- and ER stress-related genes
Freshly resuspended GCs were seeded at a cell density of 3 × 105 cells per mL in standard 24-well plates (Corning, NY, USA) and cultured at 37 °C and 5% CO2 until they reached ∼80% confluency (2–3 days) (Fig. 1A). At the experiment day, the medium was replaced with 1 mL medium containing 10 mg mL−1 of endotoxin-free GTFs and ITFs, followed by incubation for 72 hours under homeostatic conditions (without any stressor) (Fig. 1B). This incubation time was selected based on previous observations by our group and others that the expression of the studied genes on GCs increased with incubation time up to 72 hours.43,44 Untreated cells (only medium) were used as untreated controls. At the end of experiments, the morphology of controls as well as the cells under the different treatments were studied at light microscope to exclude possible morphological changes.
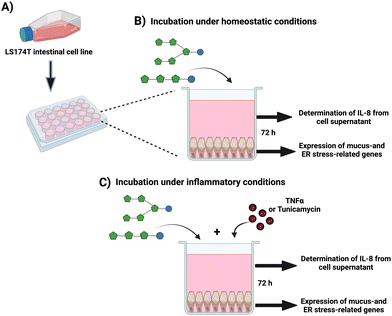 |
| Fig. 1 Experimental design. (A) Confluent goblet cells were seeded in 24-well plates. (B) After a 72-hour incubation of cells in presence of ITFs or GTFs the expression of mucus- and ER stress-related genes was determined by qPCR. The production of IL-8 was quantified from GCs supernatants. (C) GCs were incubated for 72 hours with TNFα or Tm in presence of ITFs or GTFs. Afterwards, expression of mucus- and ER stress-related genes, as well as IL-8 production were determined. | |
2.4 Protective effect of fructans from induced inflammation, mucus barrier disruption and ER stress on GCs
To study whether fructans can protect from induced inflammation, mucus barrier disruption and ER stress, GCs were exposed to 10 ng mL−1 of the pro-inflammatory cytokine TNFα (Immunotools, Friesoythe, Germany), or to 1 μg mL−1 of the N-glycosylation inhibitor tunicamycin (Tm) (Sigma-Aldrich, Zwijndrecht, The Netherlands) for 72 hours, according to the previously standardized and described by our group.44,45 These molecules are known to dysregulate the expression of mucus- as well as ER-stress related genes in gut GCs.46,47 In a second set of experiments, GCs were incubated for 72 hours with TNFα or Tm in presence of 10 mg mL−1 of fructans (Fig. 1C). Untreated cells (only culture medium) were used as negative controls. To calculate the fold-change in these experiments, cells treated with disruptor (i.e., Tm or TNF) where used as reference, thus data was normalized using the disruptors as reference conditions. At least five independent experiments were performed. After the experiments, cells were prepared for RNA isolation with trizol reagent.
2.5 RNA extraction, and quantitative reverse-transcription polymerase chain reaction PCR (qPCR)
We studied the relative mRNA expression of the mucus-related genes MUC2, TFF3, and RETNLB. We also studied the CHST5 and GAL3ST2 genes which are associated to posttranslational modification of mucin. Finally, on these GCs we studied the expression of the genes HSPA5 and XBP1, which are related to control of protein maturation, ER-stress, and UPR.48,49 After incubation under the different experimental conditions, RNA was isolated from GCs by the addition of 500 μL of TRIzol™ reagent (Life Technologies, Bleiswijk, The Netherlands) following the manufacturer's instructions. Followed by RNA quantitation, which was performed by spectrophotometry (Thermo Fisher Scientific, USA). The RNA integrity was determined by gel electrophoresis on a 2% agarose gel. Afterwards, 500 ng of total RNA were used for cDNA synthesis, which was performed with SuperScript II™ reverse transcriptase (Life Technologies, Bleiswijk, The Netherlands) according to the manufacturer's instructions. Briefly, after pre-treatment with DNAse I, RNA samples were used for a retro-transcription reaction with 300 ng of random hexamer primers (Life Technologies, Bleiswijk, The Netherlands),10 mmol L−1 of DNTPs (Thermo Fisher Scientific, USA), 5× first strand buffer, 0.1 mM of DTT, 40 units per μL of recombinant ribonuclease inhibitor (Thermo Fisher Scientific, USA) and 200 units of SuperScript II™ reverse transcriptase. This was followed by incubation at 25 °C for 10 minutes, 42 °C for 50 minutes and 70 °C for 15 minutes using a TProfessional Basic Thermocycler (Biometra, Göttingen, Germany). The total cDNA obtained was diluted 20x with DEPC-treated water (Life Technologies, Bleiswijk, The Netherlands) and 5 μL of this dilution were mixed with 5 μL of FastStart Universal SYBR Green Master (ROX) qPCR Master Mix 2× concentrated (Roche Diagnostics, Basel, Switzerland) including gene specific primers at a final concentration of 300 nmol L−1 (Table S1†).44,45,50 The qPCRs were performed in a Viia 7 Real-Time PCR System (Applied Biosystems, CA, USA). The cycling protocol used is shown in Table S2.† The primer annealing temperature and efficiency were determined with a 5-point standard curve, which was prepared with a pool of all cDNA samples diluted 5–80×. A reaction efficiency of 90–110% was considered acceptable. B2M (beta-2-microglobulin) was used as housekeeping gene.43,46 mRNA fold-change was calculated against untreated controls or against cells treated only with disruptors using the 2−ΔΔCt method.51
2.6 Measurement of GCs IL-8 production
Interleukin-8 (IL-8) from cell supernatants was considered as a marker of inflammation of GCs challenged with the disruptor molecules TNFα and Tm. After the incubation of GCs under the above-mentioned conditions, cells supernatants were collected and centrifuged for removing death cells and cell debris. The IL-8 was quantitated by ELISA from GCs supernatants according to manufacturer's instructions (R&D Systems, Biotechne, Minneapolis, USA). Controls were those GCs without any treatment.
2.7 Statistical analyses
GraphPad Prism version 9.2 was used for statistical analyses. Normal distribution of data was examined by the Shapiro–Wilk test. These data were normally distributed. Therefore, data are expressed as mean ± standard deviation (SD) from least five independent assays. Untreated control and those cells incubated with fructans were compared. Untreated control and cells treated with TNFα or Tm were also compared. Finally, cells treated with TNFα alone and those treated with TNFα in presence of fructans were compared. In all experiments statistical significance was determined using ordinary one-way ANOVA with Dunnett's multiple comparison test. A p-value < 0.05 was considered significant. * or † (p < 0.05), ** or †† (p < 0.01), *** or ††† (p < 0.001), **** or †††† (p < 0.0001).
3. Results
3.1 Chemical characteristics of studied fructans
To investigate the effect of fructans with different structure and size on the expression of mucus-related and USP-related GCs genes, GTFs and ITFs were included. The presence of β(2 → 1) and β(2 → 6) linkages in GTFs confers them a complex branched structure.37 GTF I is a mixture with mostly short chain fructans with a degree of polymerization (DP) between 3–4 but it also has fructans of DP 7–45. GTF II contains a mixture of longer chain fructans with an average DP of 17, but it also has longer chain fructans. These GTFs possess oligosaccharides of fructose (Fn) and glucose-fructose (GFn) series.41 ITFs are constituted only of fructans linked by β(2 → 1) bonds, this gives them a linear structure. ITF I is a short chain fructan mixture with a DP range of 3–10. It has fructans of the Fn and GFn series. ITF II possess a mixture composed of longer chain fructans with a DP range of 10–60 and it only has fructans of the GFn series (Table 1).39
Table 1 Chemical and structural characteristics of fructans included in the study39,41
3.2 Graminan-type fructans enhance mucus-related genes in GCs
To study the effects of ITFs and GTFs on the expression of mucus-related genes, GCs were incubated in presence of these fructans for 72 hours. This provoked a differential gene expression in a fructan structure dependent manner (Fig. 2). The expression of MUC2 was 0.38 ± 0.3-fold decreased (p > 0.01) and 0.48 ± 0.1-fold decreased (p > 0.001) by ITF I and ITF II, while none of the GTFs influenced MUC2 expression (Fig. 2A). This was different for TFF3, where an enhancement of 0.52 ± 0.3-fold (p < 0.05) and 0.52 ± 0.4-fold (p < 0.05) was observed in GCs treated with ITF I and GTF II, respectively (Fig. 2B). The RETNLB expression was strikingly enhanced only by the branched fructans. GTF I enhanced it 6.3 ± 2-fold (p < 0.01) and GTF II enhanced it 9.37 ± 1-fold (p < 0.0001) (Fig. 2C). The CHST5 expression was only enhanced 0.25 ± 0.4-fold (p < 0.05) by GTF II (Fig. 2E). Conversely, the expression of HSPA5 and XBP1 were significantly reduced by all fructans except by ITF I (Fig. 2F and G).
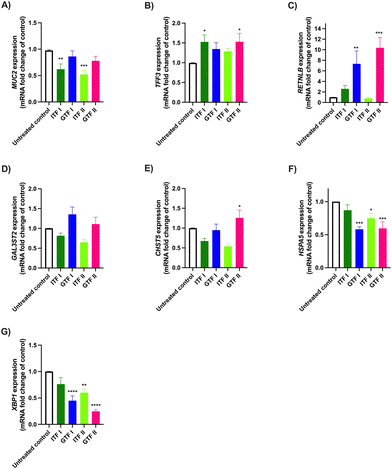 |
| Fig. 2 Effect of ITFs and GTFs with different molecular weight on the expression of mucus- and ER stress-related genes in goblet intestinal cells. GCs were incubated for 72 hours with 10 mg mL−1 of ITFs and GTFs, followed by the determination of mRNA production of MUC2 (A), TFF3 (B), RETNLB (C), GAL3ST2 (D), CHST5 (E), HSPA5 (F), and XBP1 (G) by qPCR. At least five independent experiments were performed. These data were normally distributed. Therefore, data are expressed as mean ± SD. Statistical differences between untreated control and cells incubated with fructans were calculated by ordinary one-way ANOVA with Dunnett's multiple comparison test. * (p < 0.05), ** (p < 0.01), *** (p < 0.001), **** (p < 0.0001). | |
3.3 Long chain GTF prevents TNFα-induced dysregulation of mucus-related genes in GCs
We investigated the potential protective effect of ITFs and GTFs on mucus- and ER stress-related genes in GCs exposed to TNFα. It is known that TNFα alters the expression of secretory proteins in GCs.46 To study the prevention of this impairment, GCs were incubated with TNFα in presence of the studied fructans for 72 hours. Compared with the untreated control MUC2 expression was 1.7 ± 0.7-fold decreased by TNFα (p < 0.01) (Fig. 3A). In cells incubated with TNFα in presence of GTF II this increase was 0.3 ± 1.5-fold attenuated compared with cells challenged with TNFα (p < 0.05). The remaining fructans did not significantly prevent the reduction of MUC2 expression.
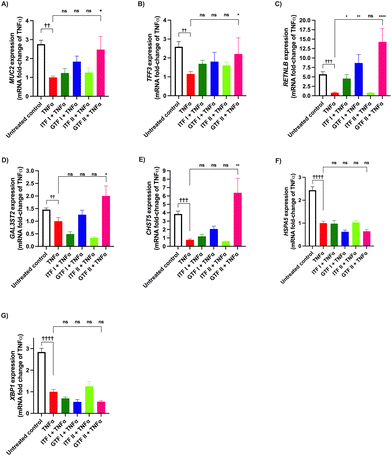 |
| Fig. 3 Prevention by ITFs and GTFs of TNFα-induced mucus- and ER stress-related gene dysregulation in goblet cells. GCs were incubated for 72 hours with TNFα in presence of 10 mg mL−1 of ITFs or GTFs. Expression of MUC2 (A), TFF3 (B), RETNLB (C), GAL3ST2 (D), CHST5 (E), HSPA5 (F), and XBP1 (G) was determined by qPCR. At least five independent experiments were performed. These data were normally distributed. Therefore, data are expressed as mean ± SD. Statistical differences between untreated control and cells treated with TNFα were calculated by ordinary one-way ANOVA with Dunnett's multiple comparison test. Cells treated with TNFα alone and those treated with TNFα in presence of fructans were compared and statistical differences were calculated by ordinary one-way ANOVA with Dunnett's multiple comparison test. * or † (p < 0.05), ** or †† (p < 0.01), *** or ††† (p < 0.001), **** or †††† (p < 0.0001). | |
Compared with the untreated control, TNFα significantly decreased the expression of TFF3 1.5 ± 0.9-fold (p < 0.01) (Fig. 3B). This decrease was 0.3 ± 0.9-fold lower and thus partly prevented by GTF II (p < 0.05). The other studied fructans did not prevent the reduction of TFF3 expression.
The RETNLB expression was 4.6 ± 0.7-fold decreased in cells treated with TNFα compared with the untreated control (p < 0.001). All the studied fructans except ITF II prevented this decrease and they even enhanced RETNLB expression. Cells incubated with TNFα in presence of GTF II had a better prevention of TNFα-induced decrease of RETNLB expression as it was 13.2 ± 3-fold higher compared with cells challenged with TNFα (p < 0.0001) (Fig. 3C).
TNFα decreased the expression of GAL3ST2 and CHST5 0.4 ± 0.1-fold (p < 0.01) and 2.8 ± 0.9-fold (p < 0.001) respectively (Fig. 3D and E). These genes are related to mucin posttranslational modifications. The only fructan that significantly prevented this decrease was GTF II. Neither GTF I nor ITFs prevented the decreased production of GAL3ST2 and CHST5 mRNA.
The expression of the ER stress-related genes HSPA5 and XBP1 was significantly decreased in cells treated with TNFα 1.4 ± 0.5-fold (p < 0.0001) and 1.8 ± 0.4-fold compared with the untreated control (p < 0.0001) (Fig. 3F and G). None of the studied fructans prevented this induced decrease.
3.4 ITFs and GTFs do not prevent Tm-induced changes in mucus-related genes in GCs
The preventive effect of fructans from the deleterious action of the N-glycosylation inhibitor Tm was studied. To that end, GCs were incubated with Tm for 72 hours in presence of fructans (Fig. 4). The MUC2 expression was 1.5 ± 0.6-fold decreased compared with the untreated control (p < 0.01) (Fig. 4A). None of the studied fructans prevented this decrease.
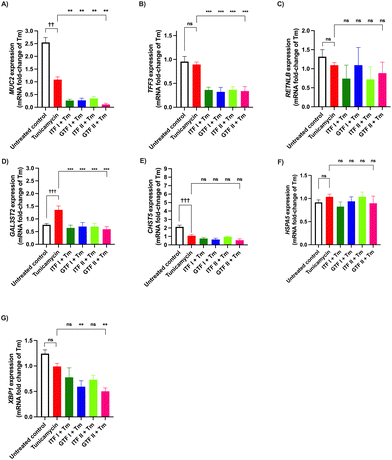 |
| Fig. 4 Prevention by ITFs and GTFs of Tm-induced mucus- and ER stress-related gene dysregulation in goblet cells. GCs were incubated for 72 hours with Tm in presence of 10 mg mL−1 of ITFs or GTFs. Expression of MUC2 (A), TFF3 (B), RETNLB (C), GAL3ST2 (D), CHST5 (E), HSPA5 (F), and XBP1 (G) was determined by qPCR. At least five independent experiments were performed. These data were normally distributed. Therefore, data are expressed as mean ± SD. Statistical differences between untreated control and cells treated with Tm were calculated by ordinary one-way ANOVA with Dunnett's multiple comparison test. Cells treated with Tm alone and those treated with Tm in presence of fructans were compared. Statistical differences were calculated by ordinary one-way ANOVA with Dunnett's multiple comparison test. * or † (p < 0.05), ** or †† (p < 0.01), *** or ††† (p < 0.001), **** or †††† (p < 0.0001) (Tm = Tunicamycin). | |
Compared with the untreated control the expression of TFF3 and RETNLB was not influenced by the Tm challenge (Fig. 4B and C).
Tm treatment provoked a 0.6 ± 0.1-fold increase of the GAL3ST2 expression, compared with the untreated control (p < 0.001) (Fig. 4D). All of the studied fructans provoked a GAL3ST2 expression more likely to that of the untreated control.
Compared with the untreated control a 1.0 ± 0.5-fold decrease of the CHST5 expression was observed in cells treated with Tm (p < 0.001) (Fig. 4E). None of the studied fructans influenced this decrease.
The mRNA production of HSPA5 remained without significant changes after the Tm challenge. The same occurred when incubating GCs with Tm in presence of fructans (Fig. 4F).
Compared with the untreated control the XBP1 expression was not influenced by the Tm challenge alone or in presence of fructans (Fig. 4G).
3.5 GTF I prevent TNFα-induced IL-8 increase in GCs
Next, the effect of fructans on the IL-8 production by GCs was investigated. To that end, GCs were incubated with fructans for 72 hours. Fig. 5A shows that ITF I as well as GTF I significantly decreased the IL-8 production by GCs compared with the untreated control, from 1322 ± 107 pg mL−1 to 885 ± 176 pg mL−1 (p < 0.05) for those cells treated with ITF I and to 888 ± 278 pg mL−1 for GTF I treated cells (p < 0.05).
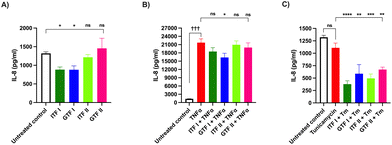 |
| Fig. 5 Production of IL-8 by goblet cells. (A) Cells were incubated with either ITF I, ITF II, GTF I or GTF II for 72 hours, followed by quantification of IL-8 production from cell supernatants. At least five independent experiments were performed. These data were normally distributed. Therefore, data are expressed as mean ± SD. Statistical differences between untreated control and cells incubated with fructans were calculated by ordinary one-way ANOVA with Dunnett's multiple comparison test. (B and C) In a second set of experiments, GCs were incubated for 72 hours with TNFα or tunicamycin alone or with TNFα or tunicamycin in presence of 10 mg mL−1 of ITFs or GTFs. At least five independent experiments were performed. These data were normally distributed. Therefore, data are expressed as mean ± SD. Statistical differences between cells treated only with disruptors and cells incubated with TNFα or tunicamycin in presence of 10 mg mL−1 of ITFs or GTFs were calculated by ordinary one-way ANOVA with Dunnett's multiple comparison test. * or † (p < 0.05), ** or †† (p < 0.01), *** or ††† (p < 0.001), **** or †††† (p < 0.0001) (Tm = tunicamycin). | |
Next the protective effect of fructans on IL-8 production by GCs exposed to TNFα or Tm was studied. To this end, GCs were incubated for 72 hours with TNFα or Tm in presence of 10 mg mL−1 of fructans. The IL-8 production was quantified from the GCs supernatant. The presence of TNFα alone increased IL-8 production from 1322 ± 107 pg mL−1 in the untreated control to 21
716 ± 3100 pg mL−1 (p < 0.001) (Fig. 5B). This increased IL-8 production was decreased to 16
336 ± 3383 pg mL−1 (p < 0.05) by GTF I. The other studied fructans did not influence the over IL-8 production of GCs under TNFα challenge.
The challenge of GCs with Tm did not influence the IL-8 concentration (Fig. 5C). However, incubation of cells with Tm in presence of fructans caused a significant decrease in IL-8 production to values even lower than those of the untreated control. The production of IL-8 decreased from 1109 ± 192 pg mL−1 in cells treated with Tm to 378 ± 135 (p < 0.0001), 588 ± 316 (p < 0.01), 499 ± 174 (p < 0.001) and 671 ± 97 (p < 0.01) in cells pre-incubated with ITF I, GTF I, ITF II and GTF II, respectively.
4. Discussion
In the present study we present, to the best of our knowledge, for the first time the in vitro effect of ITFs and GTFs on the expression of mucus- and ER stress-related genes under homeostatic and inflammatory conditions and their regulatory capacities in gut GCs. We found a remarkable beneficial impact of GTFs especially on the expression of RETNLB. It was unknown whether and to what extend these fructans could protect from the action of TNFα and Tm. Here we demonstrate that the branched long chain GTF II can protect from the gene expression dysregulation induced by TNFα. We also show that all the studied fructans prevent the Tm-induced dysregulation of GAL3ST2. Interestingly, only the short chain fructans have anti-inflammatory properties on GCs under homeostatic conditions. All these benefits found are fructan-structure and chain length dependent.
When tested under homeostatic conditions, ITF I and GTF II significantly increased the TFF3 expression. TFF3 belongs to the trefoil family of secretory peptides whose main function is mucosal protection and repairment of damaged epithelium.52 This integral and constituent mucus protein has shown to possess therapeutic potential, since its interaction with protease activator receptors (PAR) cause downregulation of proinflammatory cytokines such as IL-6 and IL-8 and upregulation of defensin expression in intestinal epithelial cells.53 Moreover, when GCs were challenged with TNFα, the expression of TFF3 significantly decreased. This effect, which is in line with others,54 was prevented by GTF II. Therefore, the prevention of TFF3 downregulation by GTF II could have an application under inflammatory conditions, such as IBD or gastrointestinal infections. Additionally, due to its protective and reparative functions, TFF3 has been proposed as a therapeutic agent for intestinal inflammatory disorders such as colitis and IBD.54,55 Furthermore, the anti-inflammatory capacity of TFF3 goes beyond the gut since it has been found in microglia.56
Interestingly, the expression of RETNLB was the most up regulated among the studied genes, and this was observed only with the branched fructans GTF I and GTF II under homeostatic conditions. When GCs were stimulated with TNFα RETNLB expression downregulation was observed. This was prevented by all fructans, except ITF II. RETNLB codes for RELMβ protein which belongs to the wide repertory of intestinal antimicrobial peptides.57 The intestinal secretion of this cysteine-rich 12.5 kDa protein increases in animal models during helminth infection58,59 and other enteric pathogens60 to promote their expulsion.59 It is also known that RELMβ can inhibit parasite chemotaxis and interferes with their nutrition by directly binding to their chemosensory constituents.61,62 Therefore, the increased RETNLB expression caused by GTFs could be considered as a defensive factor to aid during intestinal infections.
Under homeostatic conditions the CHST5 expression was increased only by GTF II. This is one of the proteins that participate in the decoration of the mucin protein core by O-glycosylations.63 The increased expression of CHST5 has been previously associated with enhancement of the mucosal barrier function in LS174T cells.10 Also, this may imply that cells with higher expression of CHST5 may be able to deal better with ER stress, since an increase in this enzyme may help to relieve the proteins that, due to poor folding or altered posttranslational modifications, got stuck in the ER. This might explain too why cells challenged with Tm in presence of GTF II have lower markers of ER stress. Thus, GTF II could represent a potential molecule for strengthening mucus barrier function.
The expression of the ER stress-related gene HSPA5 was decreased only by GTFs under homeostatic conditions. During homeostasis the protein product of HSPA5 BiP acts as a repressor of the UPR signal transducers inositol-requiring protein-1 (IRE1) and protein kinase RNA (PKR)-like ER kinase (PERK) by forming a complex. Upon ER stress BiP dissociates from the complex, provoking activation of the UPR with subsequent downstream signaling to restore ER homeostasis.49,64 Since the activation of the UPR signal depends on the repressor action of BiP, then BiP overexpression keeps UPR repressed, whereas reduced BiP levels activate the UPR.64 Thus, the decreased expression of HSPA5 that we observed would be indicating a more efficient capacity of counteraction of ER stress. However, herein we are presenting preliminary data about the influence of GTFs on UPR at mRNA level, and additional assessments of BiP protein might further support a complete insight into the role of GTFs in this pathway.
Under homeostatic conditions the expression of the ER stress-related gene XBP1 was decreased by all fructans, except ITF I. The downregulation of these ER-stress- and UPR-related gene has been previously reported by Engevik et al., as an ER-stress modulatory beneficial effect, which was observed when incubating T84 human colonic cells with a probiotic organism.65
The same was observed by Xiong et al. when incubating GCs with a flavonoid molecule.66 It is known that UPR activation is associated with inflammation18 and to failure in immunotolerance with the concomitant development of autoimmune disorders such as IBD and rheumatoid arthritis.67–69 Moreover, genetic sequencing of IBD patients revealed several XBP1 variants as factors of susceptibility for IBD development.19 Thus, the use of these fructans for the prevention of UPR activation could be valuable for the design of therapeutics against these disorders.
Incubation of GCs with Tm and TNFα-induced downregulation of the XBP1 mRNA. Upon ER stress, XBP1 mRNA suffers unconventional splicing to obtain an active XBP1 spliced form.17 Previous studies from Xue et al., who studied the expression of XBP1 on the murine fibrosarcoma L929 cell line, demonstrated that under homeostatic conditions there is a basal expression of both the unspliced and spliced forms of XBP1. When cells are exposed to ER stress by the action of the Tm, then the proportion of the spliced form increases until practically a complete predominance of this spliced form. This leads to the disappearance of the unspliced XBP1 form.70
These findings of Xue, et al., let us hypothesize that in our study we indirectly found that the XBP1 spliced form increases as the observed unspliced form decreases. In other words, under homeostatic conditions we could expect the XBP1 spliced form in the same proportion as the unspliced form (untreated control), and in those cells challenged with Tm we would expect the double proportion of the XBP1 spliced form than the observed unspliced form. Finding the spliced form in major proportion would indicate a more efficient counteraction of ER stress. As to the cells challenged with TNFα, again based on Xue, et al. findings, the predominance of the unspliced and spliced forms of XBP1 change practically in the same manner as the challenge with Tm. Therefore, our findings indicate a more efficient manner to counteract the ER stress induced by TNFα.
It is known that TNFα can induce the UPR in a reactive oxygen species (ROS)-dependent fashion.70 In the present study the measurement of ROS was not determined, however, since it is known that fructans from chicory and from agave possess ROS scavenging capacities,71–73 assessment of ROS after exposure to fructans, in presence or absence of TNFα, along with other inflammatory stressors deserves further studies.
Incubation of GCs in presence of Tm significantly downregulated the expression of MUC2 and CHST5 and increased the expression of GAL3ST2. This is in line with our previous studies.44,45 Tm is a well-known glycosylation inhibitor which induces ER stress.47 None of the fructans protected GCs from the downregulating effect of Tm. This could be indicative of the null capacity of these fructans to protect from the deregulatory effects of Tm under the studied experimental conditions.
The production of IL-8 was considered to be an indicator of inflammation in GCs. When GCs were incubated in presence of fructans, the short chain ITF I and GTF I significantly decreased this IL-8 production. Thus, these fructans could be protecting GCs from inflammation. We have previously observed this protective effect in gut epithelial cells.42 The challenge with TNFα alone caused a 16-fold increase of IL-8 production, while this TNFα challenge in presence of GTF I significantly decreased this elevation. IL-8 is a chemoattractant molecule for immune cells such as neutrophiles, whose excessive production is related to inflammatory disorders.66 This, allow us to suggest that GTF I possess a potential anti-inflammatory effect, that could contribute to lowering symptoms of intestinal inflammatory disorders.
A possible explanation of all these findings (Fig. 6) could be related to the establishment of an interaction between the fructans applied and GCs just as observed in our previous studies,41,42 which may lead to lowering permeability of the mucus layer, due to the saccharide nature of fructans, which resembles the glycans that are added to mucins.
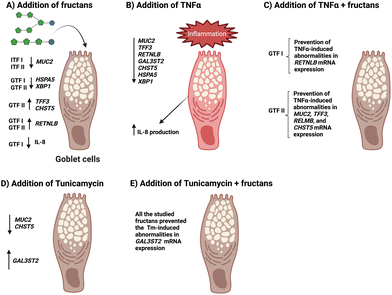 |
| Fig. 6 Schematic summary of the observed effects of ITFs and GTFs and of fructans + disruptors on mucus- and ER stress-related GCs genes. | |
However, a deeper study of the mechanisms by which the studied fructans influence GCs biology is guaranteed. In line with this is the fact that LS174T cells compared to normal GCs synthesize glycans that are truncated compared to normal MUC2 glycosylation.74 This might make these cells prone to enteropathogenic infection, as has been reported in these cells.75 Thus, we speculate that the studied fructans could have a beneficial influence on the barrier function of mucins with truncated glycosylation by replacing the lack of glycans.
Besides the direct protecting and regulatory effects of the studied fructans on GCs presented herein, it is important to acknowledge that there may also be substantial effects that are mediated by the intestinal microbiota and the metabolites they produce from fructans fermentation. This well-known prebiotic effect76–78 remains to be elucidated on GCs.
One limitation of the present study is the absence of complementary assessment of the proteins coded by the studied genes. However, since the influence of fructans derived from agave on intestinal goblet cells has never been studied, we consider our preliminary data on mRNA data is still of value, although further additional assessments of the protein products of the studied genes might provide a wider understanding of the potential beneficial effects of these dietary fibers on cellular homeostasis in GCs. Another limitation is the lack of assessment of the expression of other mucin coding genes than MUC2, such as MUC5AC, MUC6 and MUC1, MUC4 and MUC13, since LS174T cells do produce these mucins which also represent challenges for the ER secretory pathway.20,79
5. Conclusions
Findings of the present study contribute to enhanced knowledge on beneficial effects that dietary fibers such as ITFs and GTFs can exert on the preservation of GC-homeostasis. It is shown that the beneficial effects are dependent on the structure and chain length of the fructan applied. This, knowledge combined with finding from our previous studies39–42 allow us to propose specific fructan molecules as potential candidates for the design of therapies for the reestablishment of health in gut inflammatory disorders.
Author contributions
C.F.L. and P.D.V. designed the study. C.F.L. and M.A.D.S. performed the experiments. L.A.S.L. conceptualization and assisted in the qPCR data analysis. G.L.V. revised and edited the manuscript. P.D.V. Supervised and Administered the project. C.F.L. and P.D.V. wrote the manuscript. All authors have revised and improved the manuscript.
Conflicts of interest
There are no conflicts to declare.
Acknowledgements
This study was partially financed by the “Programa de Recursos Fiscales para Investigación” from Instituto Nacional de Pediatría, Grant Number 2019/062. C. F. L. was financially supported by Abel Tasman Talent Program Sandwich PhD from the University of Groningen-University Medical Center Groningen, UG/UMCG in collaboration with Universidad Nacional Autónoma de México, UNAM and CONACyT (#260625).
References
- M. E. Johansson and G. C. Hansson, Immunological aspects of intestinal mucus and mucins, Nat. Rev. Immunol., 2016, 16, 639–649 CrossRef CAS PubMed.
- E. E. Nyström, B. Martinez-Abad, L. Arike, G. M. Birchenough, E. B. Nonnecke, P. A. Castillo, F. Svensson, C. L. Bevins, G. C. Hansson and M. E. Johansson, An intercrypt subpopulation of goblet cells is essential for colonic mucus barrier function, Science, 2021, 372(6539), 1–29 CrossRef PubMed.
- T. Pelaseyed, J. H. Bergström, J. K. Gustafsson, A. Ermund, G. M. Birchenough, A. Schütte, S. van der Post, F. Svensson, A. M. Rodríguez-Piñeiro and E. E. Nyström, The mucus and mucins of the goblet cells and enterocytes provide the first defense line of the gastrointestinal tract and interact with the immune system, Immunol. Rev., 2014, 260, 8–20 CrossRef CAS PubMed.
- Y. Tobisawa, Y. Imai, M. Fukuda and H. Kawashima, Sulfation of colonic mucins by N-acetylglucosamine 6-O-sulfotransferase-2 and its protective function in experimental colitis in mice, J. Biol. Chem., 2010, 285, 6750–6760 CrossRef CAS PubMed.
- L. Thim and F. May, Trefoil factors, Cell. Mol. Life Sci., 2005, 62, 2956–2973 CrossRef CAS PubMed.
- D. Artis, M. L. Wang, S. A. Keilbaugh, W. He, M. Brenes, G. P. Swain, P. A. Knight, D. D. Donaldson, M. A. Lazar and H. R. Miller, RELMβ/FIZZ2 is a goblet cell-specific immune-effector molecule in the gastrointestinal tract, Proc. Natl. Acad. Sci. U. S. A., 2004, 101, 13596–13600 CrossRef CAS PubMed.
- J. J. Kim and W. I. Khan, Goblet cells and mucins: role in innate defense in enteric infections, Pathogens, 2013, 2, 55–70 CrossRef PubMed.
- K. A. Knoop and R. D. Newberry, Goblet cells: multifaceted players in immunity at mucosal surfaces, Mucosal Immunol., 2018, 11, 1551–1557 CrossRef CAS PubMed.
- S. Cornick, A. Tawiah and K. Chadee, Roles and regulation of the mucus barrier in the gut, Tissue Barriers, 2015, 3(1–2), e982426 CrossRef PubMed.
- N. P. Ponnuraj, S. Bhatia, J. Chow, S. Davis, M. J. Miller and H. R. Gaskins, Direct modulation of goblet cell function by galacto-oligosaccharides, FASEB J., 2013, 27, lb388–lb388 Search PubMed.
- K. Honke, M. Tsuda, S. Koyota, Y. Wada, N. Iida-Tanaka, I. Ishizuka, J. Nakayama and N. Taniguchi, Molecular cloning and characterization of a human β-Gal-3′-sulfotransferase that acts on both type 1 and type 2 (Galβ1–3/1–4GlcNAc-R) oligosaccharides, J. Biol. Chem., 2001, 276, 267–274 CrossRef CAS PubMed.
- M. H. Smith, H. L. Ploegh and J. S. Weissman, Road to ruin: targeting proteins for degradation in the endoplasmic reticulum, Science, 2011, 334, 1086–1090 CrossRef CAS PubMed.
- S. E. Bettigole and L. H. Glimcher, Endoplasmic reticulum stress in immunity, Annu. Rev. Immunol., 2015, 33, 107–138 CrossRef CAS PubMed.
- L. Zhou, H. Shen, X. Li and H. Wang, Endoplasmic Reticulum Stress in Innate Immune Cells-A Significant Contribution to Non-Alcoholic Fatty Liver Disease, Front. Immunol., 2022, 3902 Search PubMed.
- J. Grootjans, A. Kaser, R. J. Kaufman and R. S. Blumberg, The unfolded protein response in immunity and inflammation, Nat. Rev. Immunol., 2016, 16, 469–484 CrossRef CAS PubMed.
- M. C. Kopp, N. Larburu, V. Durairaj, C. J. Adams and M. M. Ali, UPR proteins IRE1 and PERK switch BiP from chaperone to ER stress sensor, Nat. Struct. Mol. Biol., 2019, 26, 1053–1062 CrossRef CAS PubMed.
- H. Yoshida, T. Matsui, A. Yamamoto, T. Okada and K. Mori, XBP1 mRNA is induced by ATF6 and spliced by IRE1 in response to ER stress to produce a highly active transcription factor, Cell, 2001, 107, 881–891 CrossRef CAS PubMed.
- A. Shkoda, P. A. Ruiz, H. Daniel, S. C. Kim, G. Rogler, R. B. Sartor and D. Haller, Interleukin-10 blocked endoplasmic reticulum stress in intestinal epithelial cells: impact on chronic inflammation, Gastroenterology, 2007, 132, 190–207 CrossRef CAS PubMed.
- A. Kaser, A.-H. Lee, A. Franke, J. N. Glickman, S. Zeissig, H. Tilg, E. E. Nieuwenhuis, D. E. Higgins, S. Schreiber and L. H. Glimcher, XBP1 links ER stress to intestinal inflammation and confers genetic risk for human inflammatory bowel disease, Cell, 2008, 134, 743–756 CrossRef CAS PubMed.
- C. K. Heazlewood, M. C. Cook, R. Eri, G. R. Price, S. B. Tauro, D. Taupin, D. J. Thornton, C. W. Png, T. L. Crockford and R. J. Cornall, Aberrant mucin assembly in mice causes endoplasmic reticulum stress and spontaneous inflammation resembling ulcerative colitis, PLoS Med., 2008, 5, e54 CrossRef PubMed.
- M. Martinez-Medina, J. Denizot, N. Dreux, F. Robin, E. Billard, R. Bonnet, A. Darfeuille-Michaud and N. Barnich, Western diet induces dysbiosis with increased E coli in CEABAC10 mice, alters host barrier function favouring AIEC colonisation, Gut, 2014, 63, 116–124 CrossRef PubMed.
- I. A. Myles, Fast food fever: reviewing the impacts of the Western diet on immunity, Nutr. J., 2014, 13, 1–17 CrossRef PubMed.
- A. Agus, J. Denizot, J. Thévenot, M. Martinez-Medina, S. Massier, P. Sauvanet, A. Bernalier-Donadille, S. Denis, P. Hofman and R. Bonnet, Western diet induces a shift in microbiota composition enhancing susceptibility to Adherent-Invasive E. coli infection and intestinal inflammation, Sci. Rep., 2016, 6, 1–14 CrossRef PubMed.
- V. Peters, G. Dijkstra and M. J. Campmans-Kuijpers, Are all dietary fibers equal for patients with inflammatory bowel disease? A systematic review of randomized controlled trials, Nutr. Rev., 2022, 80, 1179–1193 CrossRef PubMed.
- N. Veronese, M. Solmi, M. G. Caruso, G. Giannelli, A. R. Osella, E. Evangelou, S. Maggi, L. Fontana, B. Stubbs and I. Tzoulaki, Dietary fiber and health outcomes: an umbrella review of systematic reviews and meta-analyses, Am. J. Clin. Nutr., 2018, 107, 436–444 CrossRef PubMed.
- E. Salinas, D. Reyes-Pavón, N. G. Cortes-Perez, E. Torres-Maravilla, O. K. Bitzer-Quintero, P. Langella and L. G. Bermúdez-Humarán, Bioactive Compounds in Food as a Current Therapeutic Approach to Maintain a Healthy Intestinal Epithelium, Microorganisms, 2021, 9, 1634 CrossRef CAS PubMed.
- W. Yang, P. Zhao, X. Li, L. Guo and W. Gao, The potential roles of natural plant polysaccharides in inflammatory bowel disease: A review, Carbohydr. Polym., 2022, 277, 118821 CrossRef CAS PubMed.
- M. Shoaib, A. Shehzad, M. Omar, A. Rakha, H. Raza, H. R. Sharif, A. Shakeel, A. Ansari and S. Niazi, Inulin: Properties, health benefits and food applications, Carbohydr. Polym., 2016, 147, 444–454 CrossRef CAS PubMed.
- D. C. Mudannayake, D. D. Jayasena, K. M. Wimalasiri, C. S. Ranadheera and S. Ajlouni, Inulin fructans–food applications and alternative plant sources: a review, Int. J. Food Sci. Technol., 2022, 57, 5764–5780 CrossRef CAS.
- R. Singh, T. Singh and C. Larroche, Biotechnological applications of inulin-rich feedstocks, Bioresour. Technol., 2019, 273, 641–653 CrossRef CAS PubMed.
- L. Vogt, D. Meyer, G. Pullens, M. Faas, M. Smelt, K. Venema, U. Ramasamy, H. A. Schols and P. De Vos, Immunological properties of inulin-type fructans, Crit. Rev. Food Sci. Nutr., 2015, 55, 414–436 CrossRef CAS PubMed.
- G. Kelly, Inulin-type prebiotics–a review: part 1, Altern. Med. Rev., 2008, 13, 15 Search PubMed.
- H. Espinosa-Andrews, J. E. Urias-Silvas and N. Morales-Hernández, The role of agave fructans in health and food applications: A review, Trends Food Sci. Technol., 2021, 114, 585–598 CrossRef CAS.
- W. G. García-Villalba, R. Rodríguez-Herrera, L. A. Ochoa-Martínez, O. M. Rutiaga-Quiñones, J. A. Gallegos-Infante and S. M. González-Herrera, Agave fructans: a review of their technological functionality and extraction processes, J. Food Sci. Technol., 2022, 1–9 Search PubMed.
- E. Mellado-Mojica, L. E. González de la Vara and M. G. López, Fructan active enzymes (FAZY) activities and biosynthesis of fructooligosaccharides in the vacuoles of Agave tequilana Weber Blue variety plants of different age, Planta, 2017, 245, 265–281 CrossRef CAS PubMed.
- M. G. Lopez, N. A. Mancilla-Margalli and G. Mendoza-Diaz, Molecular structures of fructans from Agave tequilana Weber var. azul, J. Agric. Food Chem., 2003, 51, 7835–7840 CrossRef CAS PubMed.
- A. V. Pérez-López, J. Simpson, M. R. Clench, A. D. Gomez-Vargas and J. J. Ordaz-Ortiz, Localization and Composition of Fructans in Stem and Rhizome of Agave tequilana Weber var. azul, Front. Plant Sci., 2021, 11, 2309 Search PubMed.
- N. A. Mancilla-Margalli and M. G. López, Water-soluble carbohydrates and fructan structure patterns from Agave and Dasylirion species, J. Agric. Food Chem., 2006, 54, 7832–7839 CrossRef CAS PubMed.
- L. Vogt, U. Ramasamy, D. Meyer, G. Pullens, K. Venema, M. M. Faas, H. A. Schols and P. de Vos, Immune modulation by different types of β2→ 1-fructans is toll-like receptor dependent, PLoS One, 2013, 8(7), e68367 CrossRef CAS PubMed.
- L. Vogt, D. Meyer, G. Pullens, M. M. Faas, K. Venema, U. Ramasamy, H. A. Schols and P. de Vos, Toll-Like Receptor 2 Activation by b2→ 1-Fructans Protects Barrier Function of T84 Human Intestinal Epithelial Cells in a Chain Length–Dependent Manner, J. Nutr., 2014, 144, 1002–1008 CrossRef CAS PubMed.
- C. Fernández-Lainez, R. Akkerman, M. Oerlemans, M. Logtenberg, H. Schols, L. Silva-Lagos, G. López-Velázquez and P. de Vos, β (2→ 6)-Type fructans attenuate proinflammatory responses in a structure dependent fashion via Toll-like receptors, Carbohydr. Polym., 2022, 277, 118893 CrossRef PubMed.
- C. Fernández-Lainez, M. J. Logtenberg, X. Tang, H. A. Schols, G. López-Velázquez and P. de Vos, β (2→ 1) chicory and β (2→ 1)-β (2→ 6) agave fructans protect the human intestinal barrier function in vitro in a stressor-dependent fashion, Food Funct., 2022, 13, 6737–6748 RSC.
- S. Bhatia, P. N. Prabhu, A. C. Benefiel, M. J. Miller, J. Chow, S. R. Davis and H. R. Gaskins, Galacto–oligosaccharides may directly enhance intestinal barrier function through the modulation of goblet cells, Mol. Nutr. Food Res., 2015, 59, 566–573 CrossRef CAS PubMed.
- L. Cheng, C. Kong, M. T. Walvoort, M. M. Faas and P. de Vos, Human milk oligosaccharides differently modulate goblet cells under homeostatic, proinflammatory conditions and ER stress, Mol. Nutr. Food Res., 2020, 64, 1900976 CrossRef CAS PubMed.
- C. Ren, J. Dokter-Fokkens, S. Figueroa Lozano, Q. Zhang, B. J. de Haan, H. Zhang, M. M. Faas and P. de Vos, Lactic acid bacteria may impact intestinal barrier function by modulating goblet cells, Mol. Nutr. Food Res., 2018, 62, 1700572 CrossRef PubMed.
- J. A. Croix, S. Bhatia and H. R. Gaskins, Inflammatory cues modulate the expression of secretory product genes, Golgi sulfotransferases and sulfomucin production in LS174T cells, Exp. Biol. Med., 2011, 236, 1402–1412 CrossRef CAS PubMed.
- I. Das, C. W. Png, I. Oancea, S. Z. Hasnain, R. Lourie, M. Proctor, R. D. Eri, Y. Sheng, D. I. Crane and T. H. Florin, Glucocorticoids alleviate intestinal ER stress by enhancing protein folding and degradation of misfolded proteins, J. Exp. Med., 2013, 210, 1201–1216 CrossRef CAS PubMed.
- A. S. Lee, The ER chaperone and signaling regulator GRP78/BiP as a monitor of endoplasmic reticulum stress, Methods, 2005, 35, 373–381 CrossRef CAS PubMed.
- O. I. Coleman and D. Haller, ER stress and the UPR in shaping intestinal tissue homeostasis and immunity, Front. Immunol., 2019, 10, 2825 CrossRef CAS PubMed.
- S. Figueroa-Lozano, R. Akkerman, M. Beukema, S. S. van Leeuwen, L. Dijkhuizen and P. de Vos, 2′-Fucosyllactose impacts the expression of mucus-related genes in goblet cells and maintains barrier function of gut epithelial cells, J. Funct. Foods, 2021, 85, 104630 CrossRef CAS.
- K. J. Livak and T. D. Schmittgen, Analysis of relative gene expression data using real-time quantitative PCR and the 2− ΔΔCT method, Methods, 2001, 25, 402–408 CrossRef CAS PubMed.
- N. B. Emidio, W. Hoffmann, S. M. Brierley and M. Muttenthaler, Trefoil factor family: Unresolved questions and clinical perspectives, Trends Biochem. Sci., 2019, 44, 387–390 CrossRef PubMed.
- G. Barrera and G. S. Tortolero, Trefoil factor 3 (TFF3) from human breast milk activates PAR-2 receptors, of the intestinal epithelial cells HT-29, regulating cytokines and defensins, Bratisl. Lek. Listy, 2016, 117, 332–339 CAS.
- M. Loncar, E. Al-Azzeh, P. S. Sommer, M. Marinovic, K. Schmehl, M. Kruschewski, N. Blin, R. Stohwasser, P. Gött and T. Kayademir, Tumour necrosis factor α and nuclear factor κB inhibit transcription of human TFF3 encoding a gastrointestinal healing peptide, Gut, 2003, 52, 1297–1303 CrossRef CAS PubMed.
- B.-H. Zhang, H.-G. Yu, Z.-X. Sheng, H.-S. Luo and J.-P. Yu, The therapeutic effect of recombinant human trefoil factor 3 on hypoxia-induced necrotizing enterocolitis in immature rat, Regul. Pept., 2003, 116, 53–60 CrossRef CAS PubMed.
- P. Arnold, U. Rickert, A.-K. Helmers, J. Spreu, J. Schneppenheim and R. Lucius, Trefoil factor 3 shows anti-inflammatory effects on activated microglia, Cell Tissue Res., 2016, 365, 3–11 CrossRef CAS PubMed.
- D. C. Propheter, A. L. Chara, T. A. Harris, K. A. Ruhn and L. V. Hooper, Resistin-like molecule β is a bactericidal protein that promotes spatial segregation of the microbiota and the colonic epithelium, Proc. Natl. Acad. Sci. U. S. A., 2017, 114, 11027–11033 CrossRef CAS PubMed.
- D. B. R. Herbert, J.-Q. Yang, S. P. Hogan, K. Groschwitz, M. Khodoun, A. Munitz, T. Orekov, C. Perkins, Q. Wang and F. Brombacher, Intestinal epithelial cell secretion of RELM-β protects against gastrointestinal worm infection, J. Exp. Med., 2009, 206, 2947–2957 CrossRef CAS PubMed.
- G. Chen, S. H. Wang, J. C. Jang, J. I. Odegaard and M. G. Nair, Comparison of RELMα and RELMβ single-and double-gene-deficient mice reveals that RELMα expression dictates inflammation and worm expulsion in hookworm infection, Infect. Immun., 2016, 84, 1100–1111 CrossRef CAS PubMed.
- K. S. Bergstrom, V. Morampudi, J. M. Chan, G. Bhinder, J. Lau, H. Yang, C. Ma, T. Huang, N. Ryz and H. P. Sham, Goblet cell derived RELM-β recruits CD4 + T cells during infectious colitis to promote protective intestinal epithelial cell proliferation, PLoS Pathog., 2015, 11, e1005108 CrossRef PubMed.
- Y. S. Kim and S. B. Ho, Intestinal goblet cells and mucins in health and disease: recent insights and progress, Curr. Gastroenterol. Rep., 2010, 12, 319–330 CrossRef PubMed.
- N. Ahmed, E. Heitlinger, N. Affinass, A. A. Kühl, N. Xenophontos, V. H. Jarquin, J. Jost, S. Steinfelder and S. Hartmann, A novel non-invasive method to detect RELM beta transcript in gut barrier related changes during a gastrointestinal nematode infection, Front. Immunol., 2019, 10, 445 CrossRef CAS PubMed.
- J. K. Lee, A. Bistrup, A. Van Zante and S. D. Rosen, Activities and expression pattern of the carbohydrate sulfotransferase GlcNAc6ST-3 (I-GlcNAc6ST): functional implications, Glycobiology, 2003, 13, 245–254 CrossRef CAS PubMed.
- D. Ron and P. Walter, Signal integration in the endoplasmic reticulum unfolded protein response, Nat. Rev. Mol. Cell Biol., 2007, 8, 519–529 CrossRef CAS PubMed.
- M. A. Engevik, B. Herrmann, W. Ruan, A. C. Engevik, K. A. Engevik, F. Ihekweazu, Z. Shi, B. Luck, A. L. Chang-Graham and M. Esparza, Bifidobacterium dentium-derived y-glutamylcysteine suppresses ER-mediated goblet cell stress and reduces TNBS-driven colonic inflammation, Gut Microbes, 2021, 13, 1902717 CrossRef PubMed.
- W. Xiong, H. Ma, Z. Zhang, M. Jin, J. Wang, Y. Xu and Z. Wang, The protective effect of icariin and phosphorylated icariin against LPS-induced intestinal goblet cell dysfunction, Innate Immun., 2020, 26, 97–106 CrossRef CAS PubMed.
- I. Kim, W. Xu and J. C. Reed, Cell death and endoplasmic reticulum stress: disease relevance and therapeutic opportunities, Nat. Rev. Drug Discovery, 2008, 7, 1013–1030 CrossRef CAS PubMed.
- S. S. Cao and R. J. Kaufman, Unfolded protein response, Curr. Biol., 2012, 22, R622–R626 CrossRef CAS PubMed.
- A. Kaser, T. E. Adolph and R. S. Blumberg, The unfolded protein response and gastrointestinal disease, Semin. Immunopathol., 2013, 35, 307–319 CrossRef CAS PubMed.
- X. Xue, J.-H. Piao, A. Nakajima, S. Sakon-Komazawa, Y. Kojima, K. Mori, H. Yagita, K. Okumura, H. Harding and H. Nakano, Tumor necrosis factor α (TNFα) induces the unfolded protein response (UPR) in a reactive oxygen species (ROS)-dependent fashion, and the UPR counteracts ROS accumulation by TNFα, J. Biol. Chem., 2005, 280, 33917–33925 CrossRef CAS PubMed.
- S. G. Sayago-Ayerdi, V. M. Zamora-Gasga and K. Venema, Changes in gut microbiota in predigested Hibiscus sabdariffa L calyces and Agave (Agave tequilana weber) fructans assessed in a dynamic in vitro model (TIM-2) of the human colon, Food Res. Int., 2020, 132, 109036 CrossRef CAS PubMed.
- W. Van den Ende, D. Peshev and L. De Gara, Disease prevention by natural antioxidants and prebiotics acting as ROS scavengers in the gastrointestinal tract, Trends Food Sci. Technol., 2011, 22, 689–697 CrossRef CAS.
- J. r. m. Busserolles, E. Gueux, E. Rock, C. Demigne, A. Mazur and Y. Rayssiguier, Oligofructose protects against the hypertriglyceridemic and pro-oxidative effects of a high fructose diet in rats, J. Nutr., 2003, 133, 1903–1908 CrossRef CAS PubMed.
- K. Lavrsen, S. Dabelsteen, S. Y. Vakhrushev, A. M. Levann, A. D. Haue, A. Dylander, U. Mandel, L. Hansen, M. Frödin and E. P. Bennett, De novo expression of human polypeptide N-acetylgalactosaminyltransferase 6 (GalNAc-T6) in colon adenocarcinoma inhibits the differentiation of colonic epithelium, J. Biol. Chem., 2018, 293, 1298–1314 CrossRef CAS PubMed.
- C. B. Amat, J.-P. Motta, E. Fekete, F. Moreau, K. Chadee and A. G. Buret, Cysteine protease–dependent mucous disruptions and differential mucin gene expression in Giardia duodenalis infection, Am. J. Pathol., 2017, 187, 2486–2498 CrossRef CAS PubMed.
- A. Kapiki, C. Costalos, C. Oikonomidou, A. Triantafyllidou, E. Loukatou and V. Pertrohilou, The effect of a fructo-oligosaccharide supplemented formula on gut flora of preterm infants, Early Hum. Dev., 2007, 83, 335–339 CrossRef CAS PubMed.
- R. Closa-Monasterolo, M. Gispert-Llaurado, V. Luque, N. Ferre, C. Rubio-Torrents, M. Zaragoza-Jordana and J. Escribano, Safety and efficacy of inulin and oligofructose supplementation in infant formula: results from a randomized clinical trial, Clin. Nutr., 2013, 32, 918–927 CrossRef CAS PubMed.
- G. López-Velázquez, M. Parra-Ortiz, I. D. l. M.-D. l. Mora, I. García-Torres, S. Enríquez-Flores, M. A. Alcántara-Ortigoza, A. G.-d. Angel, J. Velázquez-Aragón, R. Ortiz-Hernández and J. M. Cruz-Rubio, Effects of fructans from Mexican agave in newborns fed with infant formula: a randomized controlled trial, Nutrients, 2015, 7, 8939–8951 CrossRef PubMed.
- A. Tawiah, S. Cornick, F. Moreau, H. Gorman, M. Kumar, S. Tiwari and K. Chadee, High MUC2 mucin expression and misfolding induce cellular stress, reactive oxygen production, and apoptosis in goblet cells, Am. J. Pathol., 2018, 188, 1354–1373 CrossRef CAS PubMed.
|
This journal is © The Royal Society of Chemistry 2023 |