DOI:
10.1039/D2FO02722D
(Paper)
Food Funct., 2023,
14, 122-132
Polysaccharides from the seeds of Gleditsia sinensis Lam. attenuate DSS-induced colitis in mice via improving gut barrier homeostasis and alleviating gut microbiota dybiosis†
Received
14th September 2022
, Accepted 24th November 2022
First published on 13th December 2022
Abstract
The seeds from Gleditsia sinensis Lam., a common ecologically and economically useful tree, have high economic and nutritional value. The protective effect of polysaccharides from Gleditsia sinensis Lam. seeds (ZJMP) against dextran sulfate sodium-induced colitis in mice was investigated in this study. ZJMP alleviated weight loss, reduced the disease activity index, prevented colon shortening, alleviated colonic tissue damage, and restored goblet cell secretion in colitic mice. Dietary ZJMP reduced proinflammatory cytokine overproduction in the colonic mucosa and serum, which was accompanied by suppression of NO levels and MPO and SOD activities. The addition of ZJMP increased the expression of Muc2 and tight junction proteins. Furthermore, dietary ZJMP partially reversed the alteration of gut microbiota in colitic mice by boosting the abundance of beneficial bacteria like Akkermansia, Lactobacillus, and Christensenella while lowering the abundance of harmful bacteria like Bacteroides, Prevotella, and Mucispirillum. Additionally, the decreased production of short-chain fatty acids in the colitic mice was recovered by ZJMP administration. The findings demonstrated the anti-inflammatory properties and mechanism of dietary ZJMP in the colon, which is essential for the sensible application of ZJMP in the prevention and amelioration of inflammation-related diseases as a nutritional supplement.
1. Introduction
Inflammatory bowel disease (IBD) including Crohn's disease and ulcerative colitis is a chronic inflammatory dysbiosis in the gastrointestinal tract.1,2 Although the detailed pathogenesis of IBD is poorly understood, multiple studies have indicated that genetic and environmental factors are very crucial in the onset of IBD.3,4 Many studies indicated that the dysbiosis of the gut microbiota is a significant determinant of host susceptibility to colitis.5–7 Diet influences the composition of gut microbiota and can be modified to help manage IBD symptoms.8,9 In fact, dietary polysaccharides have received a lot of attention and have been linked to a lower risk of IBD.10–12
Gleditsia sinensis Lam. (G. sinensis Lam.) is a typical ecologically and economically useful tree species from the genus Gleditisia in the legume family. The seeds of G. sinensis Lam., known as zaojiaomi (ZJM), are an important source of galactomannans and have high economic and nutritional value due to their high carbohydrate and low protein and fat content. Previous research concentrated on the physicochemical properties of polysaccharides derived from G. sinensis, which has been used for the treatment of some diseases.13,14 The anti-inflammatory and immunomodulatory effects of ZJM polysaccharides (ZJMPs) on ulcerative colitis, on the other hand, have been rarely reported. Furthermore, previous research studies had suggested that maintaining intestinal health depends on the cross-talk between the gut microbiota and host's inflammatory response.15–17 However, whether the gut microbiota plays a role in ZJMP-mediated IBD relief remains unknown.
Given the potential of galactomannan as an immunostimulatory or an adjuvant immunotherapy agent and the potential interaction between gut microbiota and ZJMP, we hypothesize that dietary ZJMP could attenuate colitis by primarily modulating the gut microbiota, which leads to the relieving of inflammation in the host. Therefore, in the present study, the immunomodulatory effect and potential mechanism of ZJMP on colitis were investigated and explored in dextran sulfate sodium (DSS)-induced mice colitis.
2.1 Materials
Colitis grade dextran sulfate sodium (DSS, 36
000–50
000 M. wt.) was purchased from MP Biomedicals, LLC (California, USA). Enzyme-linked immunosorbent assay (ELISA) kits for IL-6, IL-1β and TNF-α, nitric oxide (NO), and myeloperoxidase (MPO) assay kits were purchased from Nanjing Jiancheng bioengineering Co., Ltd (Nanjing, China). The bicinchoninic acid (BCA) protein assay kits and Schiff reagent were from Beijing Solarbio Technology Co., Ltd (Beijing, China). Fluorescein isothiocyanate–dextran (FITC, MW 4 kDa) was bought from Shanghai Aladdin Biochemical Technology Co., Ltd (Shanghai, China). DAPI staining solution was purchased from Beyotime Biotechnology (Shanghai, China). Fluorescein (FITC) Affinipure Goat Anti-Rabbit IgG was purchased from ZEN BIO (Chengdu, China). General laboratory chemicals and reagent solutions were of analytical grade and purchased from Sigma-Aldrich (St Louis, MO).
2.2 Preparation of polysaccharides from seeds of Gleditsia sinensis Lam.
The seeds of Gleditsia sinensis Lam. were from a processing base of Maochang Town. The seeds (50 g) were soaked with deionized water in a ratio of 1
:
10 (w/v) overnight and extracted three times at 80 °C (3 h each) with additional 1000 mL of deionized water. Then, the mixture was filtered and the filtrate was concentrated by a rotary evaporator, followed by adding ethanol to a final concentration of 50% (v/v). After precipitation for 24 hours, the precipitate was centrifuged and dissolved in distilled water. The protein was removed by the Sevag reagent method. Finally, the deproteinized residue was dialyzed with a 3000D dialysis bag and lyophilized to obtain crude Zaojiaomi polysaccharides (ZJMPs).
2.3 Molecular weight determination of ZJMPs
ZJMPs were suspended in 10 mM pH 7.0 PBS at a concentration of 2 mg mL−1, and the polysaccharide dispersions were diluted to 0.2, 0.4, 0.6, 0.8, and 1.0 mg mL−1 using the same buffer. Samples were filtered with 0.45 μm filters. Measurements were taken at angles ranging from 30° to 120° with 3D LS (LS Instruments, Fribourg, Switzerland), and data were analyzed using the Zimm Plot software to determine the molecular weight and the radius of gyration (Rg) using the equation below: Kc/I(q) = 1/Mw(1 + q2Rg2/3) + 2A2c, where K = 4π2n2(dn/dc)2/(NAλ04) and q = (4πn/λ0) sin(θ/2), with c, dn/dc, NA, Mw, A2 and λ0 being the concentration of polysaccharide suspension, the specific refractive index increment, Avogadro's number, the weight-average molar mass, the second viral coefficient and the wavelength of light in vacuum, respectively. A value of 0.2 was used as dn/dc.18
2.4 Animal experimental design and sample collection
All specific pathogen-free (SPF) male C57BL/6J mice were obtained from Liaoning Changsheng Biotechnology Co., Ltd (Certificate number: SCXK-2020-0001). The experimental design is shown in Fig. S1.† After one week of acclimation, mice were randomly divided into four groups with two cages of ten mice. The four groups are as follows: (1) control group: distilled water for 21 days; (2) DSS group: distilled water for 14 days, followed by 2.5% DSS for 7 days; (3) DSS + ZJMPL group: oral administration of 10 mg kg−1 ZJMP daily for 14 days, followed by 2.5% DSS and 10 mg kg−1 ZJMP for 7 days; (4) DSS + ZJMPH group: oral administration of 37.5 mg kg−1 ZJMP daily for 14 days, followed by 2.5% DSS and 37.5 mg kg−1 ZJMP for 7 days. All the animal experiments were performed by following the Guidelines for Laboratory Animals of the National Institute of Health and approved by the Animal Ethics Committee of Dalian Polytechnic University (Approval number: DLPU2020013).
Body weight was measured every 3 days before DSS treatment and daily after DSS treatment. Disease activity index (DAI) was used to assess the severity of colitis from the scores of body weight loss, stool consistency, and fecal blood.19 The serum, colon and cecum content, and fecal samples of all mice sacrificed on day 22 were collected and stored at −80 °C for further analysis. The weight and length of the colon were measured.
2.5 Histopathologic evaluation
For morphological analysis, the colon tissues fixed in 4% paraformaldehyde were sectioned and stained with hematoxylin and eosin. The histological score was assessed by the extent of inflammatory infiltration (0–4), villus structure (0–4), and crypt damage (0–4).20 The fixed colon tissues were stained with periodic acid–Schiff to determine the goblet cells under a microscope.21 Neutral mucin-containing goblet cells were counted and compared among different groups. All images were evaluated using the Image J software (US National Institutes of Health, Bethesda, MD).
2.6
In vivo epithelial barrier permeability assay
The gut permeability was assessed using non-digestible 4 kDa dextran conjugated with fluorescein isothiocyanate (FITC-dextran) as previously described.22 The mice were fasted for 4 hours and orally administered with 150 μL of 80 mg mL−1 FITC-dextran. After 4 hours, blood was collected into heparinized tubes by cardiac puncture and the colon tissue was obtained and sampled into tubes with PBS. Plasma was obtained with centrifugation at 2000g for 10 min. The supernatants from colon tissues were obtained by homogenization followed by centrifugation at 1600g for 5 min. The concentration of FITC-dextran in blood and colon was determined using a fluorescence 96-wells plate reader at excitation (485 nm) and emission (525 nm) wavelengths respectively (Infinite 200Pro, Tecan, Austria).
2.7 Colonic myeloperoxidase (MPO), superoxide dismutase (SOD) and nitric oxide (NO) assays
The colon tissues were homogenized in 0.1 M PBS to a homogenate and centrifuged at 2000g for 10 min. Then, the supernatant was collected for MPO, SOD and NO assays. A bicinchoninic acid (BCA) protein kit (Solarbio, Beijing, China) was used to quantify the protein content of colon tissues, and MPO and SOD activity, as well as NO content, were evaluated and determined according to the manufacturer's instructions.
2.8 Measurements of inflammatory cytokines
For inflammatory cytokine analysis, the serum of mice was obtained by centrifuging at 10
000g for 10 min at 4 °C. The concentrations of pro-inflammatory cytokines such as IL-6, IL-1β and TNF-α in the serum were quantified by ELISA kits following the manufacturer's instructions.
2.9 Gene expression analysis by RT-qPCR
Total RNA was isolated and extracted from the colon tissues using RNAeasy animal long RNA isolation kit (Beyotime Biotechnology Co., Ltd, Shanghai, China). The cDNA was synthesized using PrimeScript™ RT reagent kit (Takara Biomedical Technology Co., Ltd, Beijing, China). The primer sequences are listed in Table S1.† Changes in mRNA expression were quantified by RT-qPCR and fold changes in gene expression were calculated using the formula 2−ΔΔCt by normalizing to the reference GAPDH expression.
2.10 Immunofluorescence analysis
The fixed colon tissues were deparaffinized in xylene and rehydrated in graded alcohols. Table S2† shows the primary antibodies and FITC-labeled secondary antibodies used in the study. Fluorescence microscopy was used to obtain DAPI and FITC images from the same area (RVL-100, ECHO, Singapore).
2.11 Short-chain fatty acid (SCFA) analysis
SCFAs in cecum were analyzed with gas chromatography (GC). Briefly, 100 mg of cecum contents were acidified with 50% sulfuric acid and extracted with diethyl ether. The supernatant was obtained by homogenization, followed by ultrasonication in an ice water bath and centrifugation with 12
000g for 15 min at 4 °C. The supernatant was treated with anhydrous sodium sulfate, centrifuged with 12
000g for 15 min at 4 °C, filtered through 0.22 μm sterile membrane, and subjected to SCFA analysis by GC (Shimadzu GC2010-plus, Kyoto, Japan) equipped with an HP-INNOVAX column (30 m × 0.250 mm; 0.25 Micron; Agilent Technologies, Inc.). SCFAs were identified by comparison with chemical standards (Aladdin Bio-Chem Technology Co., Ltd, Shanghai, China) and 2-ethylbutyric acid was used as the internal reference.23
2.12 Fecal microbiota analysis
The genomic DNA of fecal samples was extracted using PowerMax DNA isolation kit (MoBio Laboratories, Carlsbad, CA, USA). DNA concentration was determined with a Nanodrop 2000c spectrophotometer (Thermo Scientific, USA). The V4 hypervariable region of the 16S rRNA gene was amplified with barcoded primers (515F, 5′-GTGCCAGCMGCCGCGGTAA-3′, 806R, 5′-GGACTACHVGGGTWTCTAAT-3′) and Phusion high-fidelity PCR Master Mix with HF buffer (New England Biolabs, USA). PCR products were purified using AXYGEN gel extraction kit (Axygen Biosciences, Santa Clara, CA, USA) and quantified using PicoGreen dsDNA assay kit (Invitrogen, Carlsbad, CA, USA). The sequencing libraries were prepared using Library Quant kit Illumina GA revised primer-SYBR Fast Universal kit (KAPA, Wilmington, MA, USA) and samples were subjected to Hiseq 3000/4000 SBS reagent kit (300 cycles) and sequenced on the Illumina HiSeq4000 platform (Illumina, San Diego, CA, USA). Raw data were qualitatively filtered and assembled, and chimeric sequences were removed to obtain effective data. Operational taxonomic unit (OUT) analysis was performed using Vsearch-1.11.1, including removal of repetitive sequence, clustering, and removal of chimera.24 Sequence data were operated using Quantitative Insights into Microbial Ecology (QIIME) (version 18.0) and R software package (version 3.2.0). Alpha diversity was assessed by the Simpson index, Chao and Shannon indices, and abundance-based richness indices. Beta diversity was assessed by calculating weighted UniFrac distance matrices between samples, which were visualized by PCA, PCoA, and NMDS. Differentially abundant taxa between groups were identified by linear discriminant analysis (LDA) effect size (LEfSe) algorithm with an alpha value of 0.01 and LDA threshold of 2.0.
2.13 Statistical analysis
All data were expressed as means ± SEM and analyzed by the GraphPad Prism 9.0 program (GraphPad Prism Software, San Diego, CA, USA). The statistical significance between different groups was determined by one-way analysis of variance (ANOVA) followed by Tukey's multiple comparison test or Dunnett's multiple comparison test.
3. Results
3.1 Determination of molecular weight of ZJMPs
The total polysaccharide content of ZJMP was determined to be 82.4%. The aggregate-free solution was subjected to static light scattering (SLS) measurements. Fig. 1 depicts the average molecular weight (Mw = 9.71 × 105 g mol−1) and radius of gyration (Rg = 69.7 nm) extracted from the Zimm plots from both samples.
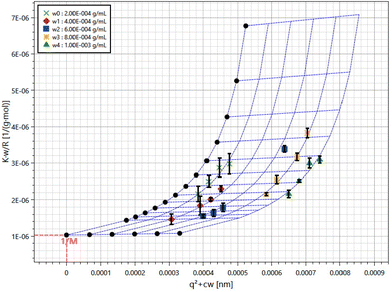 |
| Fig. 1 SLS data presented in Zimm plot obtained from ZJMP in 10 mM pH 7.0 PBS. | |
3.2 The effect of ZJMP on body weight, DAI, and colon length in DSS-induced colitis mice
To investigate the effect of ZJMP on DSS-induced colitis, mice were given 2.5% DSS in water continuously for 7 days to induce colitis. Fig. 2A shows that the weight of mice in all groups increased at a decreasing rate prior to DSS treatment. However, the weight of mice in the DSS group was significantly lower than that of those in the control group, and ZJMPL and ZJMPH administration greatly relieved the DSS-induced weight loss of mice (Fig. 2A and B). DAI in the DSS group was much higher than in the control group, although ZJMPL and ZJMPH successfully reduced DAI (Fig. 2C). Furthermore, colon shortening is an indicator of the severity of colitis. Our findings indicated that ZJMPL and ZJMPH reversed colon shortening in colitic mice (Fig. 2D and E). These findings demonstrated that ZJMP substantially reduced the severity of colitis in DSS-induced colitis mice.
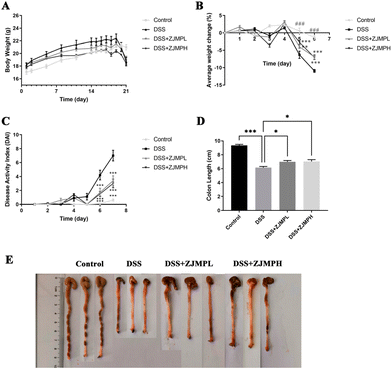 |
| Fig. 2 ZJMP administration alleviated symptoms of DSS-induced experimental colitis in mice. (A) Body weight measurement (n = 10 per group). (B) Average weight change during DSS treatment (n = 10 per group). (C) Disease activity index (DAI) (n = 10 per group). (D) Colon length of mice (n = 7 per group). (E) Representative images of the mouse colon from different groups. All data are presented as mean ± SEM, *p < 0.5, **p < 0.01, ***p < 0.001, ###p < 0.001 vs. DSS group. | |
3.3 ZJMP attenuated DSS-induced colonic injuries
According to hematoxylin and eosin (H&E) staining, DSS treatment caused severe colonic injuries, as revealed by inflammatory cell infiltration, villus structural distortion, and crypt damage, resulting in significantly higher histopathological scores compared to those of the control group. ZJMPL and ZJMPH administration, on the other hand, dramatically decreased the aberrant histological alterations in the colon of DSS-treated animals (Fig. 3A and C). Furthermore, mucin-secreting goblet cells in the colonic epithelia were measured by PAS to assess the effect of ZJMP on colonic injuries. When compared to the control group, the number of goblet cells in colonic tissues of DSS-treated mice was dramatically reduced, but ZJMP administration significantly restored the number of goblet cells (Fig. 3B and D). These findings suggested that dietary ZJMP may protect against DSS-induced colonic injuries.
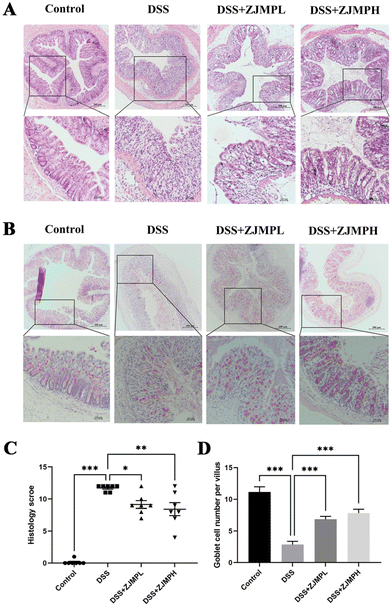 |
| Fig. 3 ZJMP supplementation attenuated colonic injuries in DSS-induced colitis mice. (A) Representative image of H&E staining of the colon tissues (magnification of 40× and 100×), (B) representative image of PAS stained mucus layer of colonic sections (magnification of 40× and 100×), (C) histological scores of colonic abnormality (n = 7 per group), and (D) goblet cell number per villus in colonic tissues (n = 7 per group). All data are presented as mean ± SEM, *p < 0.5, **p < 0.01, ***p < 0.001 vs. DSS group. | |
3.4 ZJMP improved DSS-induced gut barrier function
A FITC-dextran permeability assay was used to evaluate the integrity of the colonic mucosa. As mice were exposed to DSS for 4 hours, there was a significant increase in colonic mucosal permeability when compared to control mice that did not receive DSS. This increase, however, was abolished in mice given DSS followed by supplementary ZJMP (Fig. 4A and B). Muc2 and epithelium tight junction (TJ) proteins play critical roles in gut barrier function. Muc2, occludin, claudin-1, zonula occludens-1 (ZO-1), and Trefoil factor 3 (Tff3) mRNA expression levels were also examined. mRNA expression levels of Muc2, occluadin, claudin-1, ZO-1, and Tff3 in the colon were considerably lower in the DSS group compared to that in the control group. Muc2 and ZO-1 mRNA expression levels were dramatically increased by ZJMPL and ZJMPH treatment (Fig. 4C and F), although occludin and claudin-1 showed no significant difference when compared to that in the DSS group (Fig. 4D and E). ZJMPL treatment dramatically increased Tff3 mRNA expression, but ZJMPH treatment had no significant effect (Fig. 4G). Immunofluorescence assays were used to further investigate the protective effect of ZJMP against DSS-induced alteration of gut barrier function. As shown in Fig. 4H, ZJMP therapy greatly reduced Muc2 and ZO-1 loss and structural alterations in the colonic epithelium's inner layer. These findings suggested that ZJMP may enhance gut barrier function by modulating colonic mucosal permeability and increasing Muc2 and TJ expression.
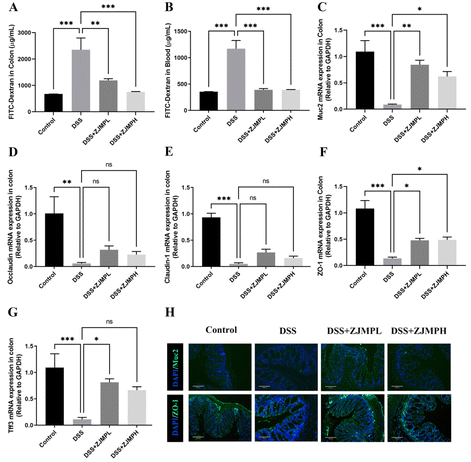 |
| Fig. 4 ZJMP improved gut barrier function in DSS-induced colitis mice. FITC-dextran concentration in colon (A) and blood (B) (n = 3). Relative mRNA expression of Muc2 (C), occludin (D), claudin-1 (E), ZO-1 (F), and Tff3 (G) in the colon tissue (n = 3). (H) Expression of Muc2 and ZO-1 was examined by immunostaining using anti-Muc2 (green) and anti-ZO-1 (green) antibodies in different groups (magnification of 100×). All data are presented as mean ± SEM, *p < 0.5, **p < 0.01, ***p < 0.001 vs. DSS group. | |
3.5 The effect of ZJMP on MPO, SOD, NO, and pro-inflammatory cytokines in DSS-induced colitis
MPO, SOD, NO in serum and colonic tissues were measured to assess the effect of ZJMP on systemic and colonic inflammatory response. MPO activity was measured to assess neutrophil infiltration. As shown in Fig. 5A, DSS significantly increased MPO activity in the colon of mice compared to that in the control group, but this increase was significantly dampened by ZJMP treatment. SOD activity is essential for the control of neutrophil apoptosis. However, no significant difference in SOD activity was found between the DSS and ZJMP groups (Fig. 5B). The level of NO is linked to ulcerative colitis. The NO content in the colon increased significantly after DSS treatment, as expected, and ZJMP supplementation significantly inhibited the increase in NO content (Fig. 5C). Inflammation is marked by abnormal upregulation of proinflammatory cytokines. As shown in Fig. 5D–I, DSS significantly increased the overproduction of proinflammatory cytokines such as IL-6, IL-1β, and TNF-α in the serum and colonic tissues compared to that in the control group. Dietary ZJMP, on the other hand, effectively suppressed the production of these proinflammatory cytokines in DSS-treated mice. These findings suggested that ZJMP's anti-inflammatory effect was at least partially due to reduced neutrophil infiltration and proinflammatory cytokine in the colon.
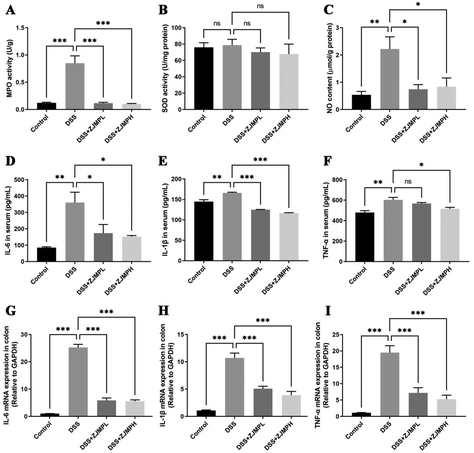 |
| Fig. 5 The effect of ZJMP on systemic and colonic inflammatory response. (A) MPO activity (n = 7), (B) SOD activity (n = 7), (C) NO content (n = 7), (D–F) quantification of proinflammatory cytokines IL-6, IL-1β, and TNF-α in the serum by ELISA kit (n = 7), and (G–I) mRNA expressions of proinflammatory cytokines IL-6, IL-1β, and TNF-α in the colonic tissues by RT-qPCR (n = 3). All data are presented as mean ± SEM, *p < 0.5, **p < 0.01, ***p < 0.001 vs. DSS group. | |
3.6 ZJMP ameliorated DSS-induced gut microbiota dysbiosis
A growing body of evidence suggests that gut microbiota play an important role in colonic inflammation. As a result, we used 16S rRNA gene sequencing to investigate the effect of ZJMP on the gut microbiota composition of DSS-treated mice. The difference in community richness and diversity between groups, as measured by the Chao 1, ACE, Shannon, and Simpson indices, is reflected in the alpha diversity. The alpha diversity of the ZJMPL and ZJMPH groups was significantly lower when compared to that of the DSS group (Fig. 6A and S2A–D†). Principal co-ordinate analysis (PCoA) based on the Bray–Curtis distance revealed a difference in the structure of the gut microbiota between control and colitis mice. The gut microbiota structure was also distinct between the DSS and ZJMP groups, particularly the ZJMPH group (Fig. 6B), indicating that ZJMP treatment had a significant impact on the gut microbiota structure in mice with colitis.
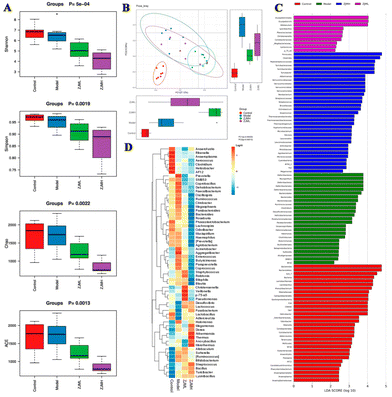 |
| Fig. 6 Effects of ZJMP on gut microbiota in DSS-induced colitis. (A) Effect of ZJMP on alpha diversity of fecal bacteria in different groups, (B) effect of ZJMP on β diversity of fecal bacteria in different groups, (C) analysis of differences in microbial taxa shown by LEfSe, (D) heatmap analysis of microbial taxa based on genus level from different groups. | |
Firmicutes, Bacteroidetes, Proteobacteria, and Verrucomicrobia were the most common phyla in the fecal microbiota (Fig. S3A†). Lactobacillus, Turicibacter, Desulfovibrio, and Prevotella dominated the fecal microbiota at the genus level (Fig. S3B†). LEfSe analysis identified differentially abundant fecal bacterial taxa in mice with different treatments. Furthermore, we discovered that 7 and 18 bacterial genera (e.g., Akkermansia, Lactobacillus, and Christensenella) were enriched in the ZJMPL and ZJMPH groups, respectively, while 10 taxa (e.g., Bacteroides, Prevotella, and Mucispirillum) were enriched in the DSS group (Fig. 6C and D).
3.7 ZJMP regulated the production of SCFAs in the cecum
We measured the cecal concentrations of acetate, propionate, butyrate, isobutyrate, and valerate. The contents of acetate, propionate, butyrate, and valerate were significantly diminished by DSS compared to that in the control group (Fig. 7). Although there was no significant difference on all SCFAs between the DSS and ZJMPH groups, the production of propionate, isobutyrates, valerate, and isovalerate was significantly improved by ZJMPL treatment compared to that in the DSS group (Fig. 7). These results suggested that ZJMPL could restore the production of certain SCFAs in the cecum.
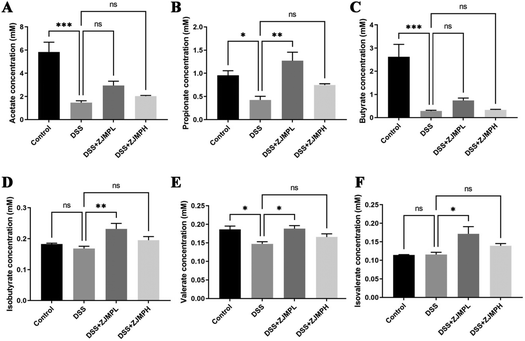 |
| Fig. 7 Effects of ZJMP on the concentrations of (A) acetate, (B) propionate, (C) butyrate, (D) isobutyrate, (E) valerate, and (F) isovalerate in the cecal contents (n = 5). All data are presented as mean ± SEM, *p < 0.5, **p < 0.01, ***p < 0.001 vs. DSS group. | |
4. Discussion
Dietary preference and gut microbiota, in addition to host genetics, have been closely linked to the development and progression of IBD.9,25–27 Various dietary polysaccharides have been studied for their ability to alleviate or treat IBD.10,11 In this study, ZJMP (Mw = 9.71 g mol−1) isolated from the seeds of G. sinensis Lam. Alleviated symptoms in DSS-induced colitis mice by improving body weight, decreasing DAI, restoring colon length, and alleviating colon tissue inflammation.
The inner mucus layer near the epithelium, which is largely devoid of microorganisms, serves as a formidable physical barrier and is also necessary for maintaining intestinal homeostasis.28–30 Histopathological analysis revealed that DSS severely harmed epithelial integrity, while ZJMPL and ZJMPH administration alleviated pathological features such as inflammatory cell infiltration, villus structural distortion, and crypt damage. Mucus secretion by the host limits the access of potentially harmful microorganisms to the mucosal surface.31 The mucus that lines the gut epithelium is primarily made up of highly glycosylated proteins, such as mucin 2 (Muc2), which are secreted by goblet cells.32 As a result, we counted the number of mucin-secreting goblet cells in the colonic epithelia and discovered that ZJMPL and ZJMPH could boost the number of goblet cells. Furthermore, using RT-qPCR and immunostaining, we discovered that ZJMPL and ZJMPH could significantly promote Muc2 secretion in DSS-induced colonic mice. These findings suggest that ZJMP may help to improve the gut barrier by increasing Muc2 secretion. In addition, TJs also play an important role in maintaining intestinal barrier function and are made up of two functional protein categories: integral transmembrane proteins like occludin and claudins and peripheral membrane proteins like ZO-1.33 Occludin and claudin are considered to be important in the assembly and maintenance of TJs because they are highly expressed at the cell–cell contact sites. ZO-1 was the first TJ-specific protein discovered, and it connected junctional proteins like occludin and claudin to the actin cytoskeleton, where these protein interactions keep TJs formed and functioning.34 Tff3 plays a role in the protection of the gastrointestinal mucosa and restoration of normal intestinal permeability, which is closely related to the integrity of the TJ barrier and the TJ associated proteins claudin-1, ZO-1 and occludin.35 In the current study, DSS significantly weakened the intestinal epithelial barrier, whereas ZJMP treatment improved the situation, as evidenced by increased ZO-1 and Tff3 expression and decreased FITC-D mucosal permeability in the serum and colon.
TJs’ ability to maintain the integrity of the intestinal epithelial barrier is critical for protecting the body from inflammatory stress stimuli. Proinflammatory cytokines play an important role in the disruption of TJ homeostasis. As a result, proinflammatory cytokines such as IL-6, IL-1, and TNF-α were significantly increased in DSS-induced mice's colon and serum, whereas ZJMP treatment significantly reduced proinflammatory cytokine secretion. Proinflammatory cytokines frequently activate neutrophils, causing them to infiltrate the colonic mucosa and amplifying the inflammatory response. Increased MPO activity in the circulation is linked to inflammation and increased oxidative stress.36,37 Here, we found that ZJMP could significantly inhibit the MPO activity in DSS-induced colonic mice. To resolve inflammation, activated neutrophils must be safely removed via apoptosis.38 Previous research suggested that SOD could effectively induce neutrophil apoptosis.39 As a result, we measured SOD activity and discovered that there was no significant difference between the DSS and ZJMP groups, implying that ZJMP may not suppress inflammation by increasing SOD activity.
A large body of evidence suggests that the microbiota has a crucial role in triggering IBD and the perturbation of microbiota composition is an important characteristic of IBD.40 In line with previous research, we discovered that DSS reduced the diversity of gut microbiota and altered the composition of microbial communities when compared to the control group.41,42 Furthermore, PCoA analyses revealed that dietary ZJMP had a significant impact on the structure of the gut microbiota in DSS-induced colitic mice. The presence of symbiotic microorganisms is very important for IBD pathogenesis. Many host physiological processes are aided by resident symbionts, including metabolic functions, epithelial barrier regulation, host immune regulation, and pathogen colonization protection.43Akkermansia has also been shown to improve gut barrier function in the context of intestinal inflammation.44 Indeed, Akkermansia has been shown to be significantly reduced in patients with Crohn's disease and ulcerative colitis.45 Furthermore, several studies have found that Akkermansia muciniphila improved DSS-induced colitis by restoring gut barrier function and decreasing weight loss, colon length shortening, and histopathology scores.46Lactobacillus, as a recognized beneficial bacterium, has been used to regulate the immune system and treat gastrointestinal disease.47,48 Previous studies have indicated that various Lactobacillus strain supplementation could attenuate DSS-induced colitis.49–51Christensenella is from the family Christensenellaceae, which is closely associated with IBD and were reported among the main predictive taxa significantly decreased in a cohort of CD patients. Thus, Christensenella plays a major role in gut health.52–54 As expected, the relative abundance of Akkermansia, Lactobacillus, and Christensenella was significantly higher in the ZJMPL and ZJMPH groups than in the DSS group.
SCFAs are an important class of gut microbiota bioproducts that are produced primarily through fermentation of non-digestible carbohydrates. SCFAs, such as acetate, propionate, butyrate, and valerate play an important role in intestinal homeostasis. Several studies have found that fecal SCFA levels are lower in people with active IBD.55–57 The current study found that acetate, propionate, butyrate, isobutyrate, and valerate levels were 74.9, 55.5, 89.2, 8.2, and 20.9% lower in DSS-induced colitic mice than in the control group. Dietary ZJMPL restored the decreased SCFAs in the cecum caused by DSS-induced colitis, but ZJMPH did not. According to the evidence, SCFAs are closely related to the type and dose of polysaccharide intake,11,58 which might explain why ZJMPL and ZJMPH have different effects on SCFA production. However, why ZJMPL could promote SCFA production while ZJMPH did not warrants further investigation.
5. Conclusion
These findings demonstrated for the first time that dietary ZJMP could attenuate inflammation in DSS-induced colitis mice, which was closely related to dietary ZJMP's restoration of immune homeostasis, as evidenced by the fact that dietary ZJMP reduced inflammatory cell infiltration, promoted Muc2 secretion, increased tight junction protein expression, and decreased the overproduction of proinflammatory cytokines in the colitic mice. Dietary ZJMP's anti-inflammatory effects were also linked to alleviated dysbiosis of gut microbiota in colitic mice, as evidenced by the increased abundance of beneficial bacteria, the decreased abundance of harmful bacteria, and the increased production of SCFAs in the colon. In conclusion, the present results establish a scientific basis for the use of ZJMP in the prevention of colonic inflammation. However, further research is required to elucidate the detailed mechanisms before its application.
Author contributions
Ningbo Qin: conceptualization, methodology, writing – original draft, and funding acquisition. Hongxu Liu: conceptualization, project administration, and writing – original draft. Yu Cao: investigation, data curation, and formal analysis. Zhen Wang: investigation and data curation. Xiaomeng Ren: review of draft. Xiaodong Xia: supervision and review of draft.
Conflicts of interest
The authors declare that they have no conflict of financial interest.
Acknowledgements
The authors are grateful for the support provided by the National Natural Science Foundation of China (32101963) and Basic Scientific Research Foundation in Higher Education Institutions of Liaoning Province, China (LJKMZ20220890).
References
- G. G. Kaplan, The global burden of IBD: from 2015 to 2025, Nat. Rev. Gastroenterol. Hepatol., 2015, 12, 720–727 CrossRef PubMed.
- S. C. Ng, H. Y. Shi, N. Hamidi, F. E. Underwood, W. Tang, E. I. Benchimol, R. Panaccione, S. Ghosh, J. C. Y. Wu, F. K. L. Chan, J. J. Y. Sung and G. G. Kaplan, Worldwide incidence and prevalence of inflammatory bowel disease in the 21st century: a systematic review of population-based studies, Lancet, 2017, 390, 2769–2778 CrossRef PubMed.
- R. J. Xavier and D. K. Podolsky, Unravelling the pathogenesis of inflammatory bowel disease, Nature, 2007, 448, 427–434 CrossRef CAS PubMed.
- C. Chen, Y. M. Shah, K. Morimura, K. W. Krausz, M. Miyazaki, T. A. Richardson, E. T. Morgan, J. M. Ntambi, J. R. Idle and F. J. Gonzalez, Metabolomics reveals that hepatic stearoyl-CoA desaturase 1 downregulation exacerbates inflammation and acute colitis, Cell Metab., 2008, 7, 135–147 CrossRef CAS PubMed.
- E. A. Franzosa, A. Sirota-Madi, J. Avila-Pacheco, N. Fornelos, H. J. Haiser, S. Reinker, T. Vatanen, A. B. Hall, H. Mallick, L. J. McIver, J. S. Sauk, R. G. Wilson, B. W. Stevens, J. M. Scott, K. Pierce, A. A. Deik, K. Bullock, F. Imhann, J. A. Porter, A. Zhernakova, J. Fu, R. K. Weersma, C. Wijmenga, C. B. Clish, H. Vlamakis, C. Huttenhower and R. J. Xavier, Gut microbiome structure and metabolic activity in inflammatory bowel disease, Nat. Microbiol., 2019, 4, 293–305 CrossRef CAS PubMed.
- D. Gevers, S. Kugathasan, L. A. Denson, Y. Vazquez-Baeza, W. Van Treuren, B. Ren, E. Schwager, D. Knights, S. J. Song, M. Yassour, X. C. Morgan, A. D. Kostic, C. Luo, A. Gonzalez, D. McDonald, Y. Haberman, T. Walters, S. Baker, J. Rosh, M. Stephens, M. Heyman, J. Markowitz, R. Baldassano, A. Griffiths, F. Sylvester, D. Mack, S. Kim, W. Crandall, J. Hyams, C. Huttenhower, R. Knight and R. J. Xavier, The treatment-naive microbiome in new-onset Crohn's disease, Cell Host Microbe, 2014, 15, 382–392 CrossRef CAS PubMed.
- K. Honda and D. R. Littman, The microbiome in infectious disease and inflammation, Annu. Rev. Immunol., 2012, 30, 759–795 CrossRef CAS PubMed.
- C. A. Chapman-Kiddell, P. S. Davies, L. Gillen and G. L. Radford-Smith, Role of diet in the development of inflammatory bowel disease, Inflamm. Bowel Dis., 2010, 16, 137–151 CrossRef PubMed.
- P. M. Miranda, G. De Palma, V. Serkis, J. Lu, M. P. Louis-Auguste, J. L. McCarville, E. F. Verdu, S. M. Collins and P. Bercik, High salt diet exacerbates colitis in mice by decreasing Lactobacillus levels and butyrate production, Microbiome, 2018, 6, 57 CrossRef PubMed.
- J. Sun, H. Chen, J. Kan, Y. Gou, J. Liu, X. Zhang, X. Wu, S. Tang, R. Sun, C. Qian, N. Zhang, F. Niu and C. Jin, Anti-inflammatory properties and gut microbiota modulation of an alkali-soluble polysaccharide from purple sweet potato in DSS-induced colitis mice, Int. J. Biol. Macromol., 2020, 153, 708–722 CrossRef CAS.
- Q. Zou, X. Zhang, X. Liu, Y. Li, Q. Tan, Q. Dan, T. Yuan, X. Liu, R. H. Liu and Z. Liu, Ficus carica polysaccharide attenuates DSS-induced ulcerative colitis in C57BL/6 mice, Food Funct., 2020, 11, 6666–6679 RSC.
- S. R. Llewellyn, G. J. Britton, E. J. Contijoch, O. H. Vennaro, A. Mortha, J. F. Colombel, A. Grinspan, J. C. Clemente, M. Merad and J. J. Faith, Interactions Between Diet and the Intestinal Microbiota Alter Intestinal Permeability and Colitis Severity in Mice, Gastroenterology, 2018, 154, 1037–1046.e2 CrossRef.
- H. Jian, C. Cristhian, W. Zhang and J. Jiang, Influence of dehulling pretreatment on physicochemical properties of Gleditsia sinensis Lam. gum, Food Hydrocolloids, 2011, 25, 1337–1343 CrossRef CAS.
- Y. Liu, Z. Xu, W. Zhang, J. Duan, J. Jiang and D. Sun, Characterization of Fractional Polysaccharides from Gleditsia sinensis and Gleditsia microphylla Gums, Molecules, 2016, 21, 1745 CrossRef PubMed.
- A. Larabi, N. Barnich and H. T. T. Nguyen, New insights into the interplay between autophagy, gut microbiota and inflammatory responses in IBD, Autophagy, 2020, 16, 38–51 CrossRef CAS PubMed.
- G. Aviello, A. K. Singh, S. O'Neill, E. Conroy, W. Gallagher, G. D'Agostino, A. W. Walker, B. Bourke, D. Scholz and U. G. Knaus, Colitis susceptibility in mice with reactive oxygen species deficiency is mediated by mucus barrier and immune defense defects, Mucosal Immunol., 2019, 12, 1316–1326 CrossRef CAS PubMed.
- Y. Lee, K. Sugihara, M. G. Gillilland 3rd, S. Jon, N. Kamada and J. J. Moon, Hyaluronic acid-bilirubin nanomedicine for targeted modulation of dysregulated intestinal barrier, microbiome and immune responses in colitis, Nat. Mater., 2020, 19, 118–126 CrossRef CAS PubMed.
- J. Wang, W. B. Navicha, X. Na, W. Ma, X. Xu, C. Wu and M. Du, Preheat-induced soy protein particles with tunable heat stability, Food Chem., 2021, 336, 127624 CrossRef CAS PubMed.
- Y. Han, M. Song, M. Gu, D. Ren, X. Zhu, X. Cao, F. Li, W. Wang, X. Cai, B. Yuan, T. Goulette, G. Zhang and H. Xiao, Dietary intake of whole strawberry inhibited colonic inflammation in Dextran-Sulfate-Sodium-Treated mice via restoring immune homeostasis and alleviating gut microbiota dysbiosis, J. Agric. Food Chem., 2019, 67, 9168–9177 CrossRef CAS PubMed.
- Z. Wu, S. Huang, T. Li, N. Li, D. Han, B. Zhang, Z. Z. Xu, S. Zhang, J. Pang, S. Wang, G. Zhang, J. Zhao and J. Wang, Gut microbiota from green tea polyphenol-dosed mice improves intestinal epithelial homeostasis and ameliorates experimental colitis, Microbiome, 2021, 9, 184 CrossRef CAS PubMed.
- A. Kasprzak, A. Seraszek-Jaros, J. Jagielska, C. Helak-Lapaj, E. Siodla, J. Szmeja and E. Kaczmarek, The histochemical alterations of mucin in colorectal carcinoma quantified by two efficient algorithms of digital image analysis, Int. J. Mol. Sci., 2019, 20, 4580 CrossRef CAS.
- A. Woting and M. Blaut, Small intestinal permeability and gut-transit time determined with low and high molecular weight fluorescein isothiocyanate-dextrans in C3H mice, Nutrients, 2018, 10, 685 CrossRef PubMed.
- Y. Cao, J. Gao, L. Zhang, N. Qin, B. Zhu and X. Xia, Jellyfish skin polysaccharides enhance intestinal barrier function and modulate the gut microbiota in mice with DSS-induced colitis, Food Funct., 2021, 12, 10121 RSC.
- T. Rognes, T. Flouri, B. Nichols, C. Quince and F. Mahé, VSEARCH: a versatile open source tool for metagenomics, PeerJ, 2016, 4, e2584 CrossRef PubMed.
- D. Piovani, S. Danese, L. Peyrin-Biroulet, G. K. Nikolopoulos, T. Lytras and S. Bonovas, Environmental risk factors for inflammatory bowel diseases: An umbrella review of meta-analyses, Gastroenterology, 2019, 157, 647–659.e4 CrossRef.
- D. B. Graham and R. J. Xavier, Pathway paradigms revealed from the genetics of inflammatory bowel disease, Nature, 2020, 578, 527–539 CrossRef CAS PubMed.
- M. Lee and E. B. Chang, Inflammatory bowel diseases (IBD) and the microbiome-searching the crime scene for clues, Gastroenterology, 2021, 160, 524–537 CrossRef CAS.
- M. E. Johansson, M. Phillipson, J. Petersson, A. Velcich, L. Holm and G. C. Hansson, The inner of the two Muc2 mucin-dependent mucus layers in colon is devoid of bacteria, Proc. Natl. Acad. Sci. U. S. A., 2008, 105, 15064–15069 CrossRef CAS PubMed.
- M. Herath, S. Hosie, J. C. Bornstein, A. E. Franks and E. L. Hill-Yardin, The Role of the Gastrointestinal Mucus System in Intestinal Homeostasis: Implications for Neurological Disorders, Front. Cell. Infect. Microbiol., 2020, 10, 248 CrossRef CAS PubMed.
- G. C. Hansson, Role of mucus layers in gut infection and inflammation, Curr. Opin. Microbiol., 2012, 15, 57–62 CrossRef CAS.
- Y. Belkaid and T. W. Hand, Role of the microbiota in immunity and inflammation, Cell, 2014, 157, 121–141 CrossRef CAS.
- L. Arike, J. Holmen-Larsson and G. C. Hansson, Intestinal Muc2 mucin O-glycosylation is affected by microbiota and regulated by differential expression of glycosyltranferases, Glycobiology, 2017, 27, 318–328 CAS.
- B. Lee, K. M. Moon and C. Y. Kim, Tight junction in the intestinal epithelium: its association with diseases and regulation by phytochemicals, J. Immunol. Res., 2018, 2018, 2645465 Search PubMed.
- J. Haskins, L. Gu, E. S. Wittchen, J. Hibbard and B. R. Stevenson, ZO-3, a novel member of the MAGUK protein family found at the tight junction, interacts with ZO-1 and occludin, J. Cell Biol., 1998, 141, 199–208 CrossRef CAS PubMed.
- E. Aihara, K. A. Engevik and M. H. Montrose, Trefoil factor peptides and gastrointestinal function, Annu. Rev. Physiol., 2017, 79, 357–380 CrossRef CAS PubMed.
- M. J. Davies and C. L. Hawkins, The role of myeloperoxidase in biomolecule modification, chronic inflammation, and disease, Antioxid. Redox Signal., 2020, 32, 957–981 CrossRef CAS PubMed.
- G. Ndrepepa, Myeloperoxidase - A bridge linking inflammation and oxidative stress with cardiovascular disease, Clin. Chim. Acta, 2019, 493, 36–51 CrossRef CAS PubMed.
- K. Yasui and A. Baba, Therapeutic potential of superoxide dismutase (SOD) for resolution of inflammation, Inflammation Res., 2006, 55, 359–363 CrossRef CAS PubMed.
- K. Yasui, N. Kobayashi, T. Yamazaki, K. Agematsu, S. Matsuzaki, S. Ito, S. Nakata, A. Baba and K. Koike, Superoxide dismutase (SOD) as a potential inhibitory mediator of inflammation via neutrophil apoptosis, Free Radic. Res., 2005, 39, 755–762 CrossRef CAS PubMed.
- D. N. Frank, A. L. St Amand, R. A. Feldman, E. C. Boedeker, N. Harpaz and N. R. Pace, Molecular-phylogenetic characterization of microbial community imbalances in human inflammatory bowel diseases, Proc. Natl. Acad. Sci. U. S. A., 2007, 104, 13780–13785 CrossRef CAS PubMed.
- Y. Peng, Y. Yan, P. Wan, D. Chen, Y. Ding, L. Ran, J. Mi, L. Lu, Z. Zhang, X. Li, X. Zeng and Y. Cao, Gut microbiota modulation and anti-inflammatory properties of anthocyanins from the fruits of Lycium ruthenicum Murray in dextran sodium sulfate-induced colitis in mice, Free Radicals Biol. Med., 2019, 136, 96–108 CrossRef CAS PubMed.
- H. Zhao, N. Cheng, W. Zhou, S. Chen, Q. Wang, H. Gao, X. Xue, L. Wu and W. Cao, Honey polyphenols ameliorate DSS-induced ulcerative colitis via modulating gut microbiota in rats, Mol. Nutr. Food Res., 2019, 63, 1900638 CrossRef CAS PubMed.
- J. M. Pickard, M. Y. Zeng, R. Caruso and G. Nunez, Gut microbiota: Role in pathogen colonization, immune responses, and inflammatory disease, Immunol. Rev., 2017, 279, 70–89 CrossRef CAS PubMed.
- A. Everard, C. Belzer, L. Geurts, J. P. Ouwerkerk, C. Druart, L. B. Bindels, Y. Guiot, M. Derrien, G. G. Muccioli, N. M. Delzenne, W. M. de Vos and P. D. Cani, Cross-talk between Akkermansia muciniphila and intestinal epithelium controls diet-induced obesity, Proc. Natl. Acad. Sci. U. S. A., 2013, 110, 9066–9071 CrossRef CAS PubMed.
- C. W. Png, S. K. Linden, K. S. Gilshenan, E. G. Zoetendal, C. S. McSweeney, L. I. Sly, M. A. McGuckin and T. H. Florin, Mucolytic bacteria with increased prevalence in IBD mucosa augment in vitro utilization of mucin by other bacteria, Am. J. Gastroenterol., 2010, 105, 2420–2428 CrossRef CAS PubMed.
- X. Bian, W. Wu, L. Yang, L. Lv, Q. Wang, Y. Li, J. Ye, D. Fang, J. Wu, X. Jiang, D. Shi and L. Li, Administration of Akkermansia muciniphila ameliorates dextran sulfate sodium-induced ulcerative colitis in mice, Front. Microbiol., 2019, 10, 2259 CrossRef PubMed.
- B. Le and S. H. Yang, Efficacy of Lactobacillus plantarum in prevention of inflammatory bowel disease, Toxicol. Rep., 2018, 5, 314–317 CrossRef CAS PubMed.
- X. Tian, Z. Yu, P. Feng, Z. Ye, R. Li, J. Liu, J. Hu, A. Kakade, P. Liu and X. Li, Lactobacillus plantarum TW1-1 alleviates diethylhexylphthalate-induced testicular damage in mice by modulating gut microbiota and decreasing inflammation, Front. Cell. Infect. Microbiol., 2019, 9, 221 CrossRef CAS PubMed.
- H. Wang, C. Zhou, J. Huang, X. Kuai and X. Shao, The potential therapeutic role of Lactobacillus reuteri for treatment of inflammatory bowel disease, Am. J. Transl. Res., 2020, 12, 1569–1583 CAS.
- R. Curciarello, K. E. Canziani, I. Salto, E. B. Romero, A. Rocca, I. Doldan, E. Peton, S. Brayer, A. M. Sambuelli, S. Goncalves, P. Tirado, G. J. Correa, M. Yantorno, L. Garbi, G. H. Docena, M. L. Á. Serradell and C. I. Muglia, Probiotic Lactobacilli isolated from kefir promote down-regulation of inflammatory lamina propria T cells from patients with active IBD, Front. Pharmacol., 2021, 12, 658026 CrossRef CAS PubMed.
- W. K. Kim, D. H. Han, Y. J. Jang, S. Park, S. J. Jang, G. Lee, H. S. Han and G. Ko, Alleviation of DSS-induced colitis via Lactobacillus acidophilus treatment in mice, Food Funct., 2021, 12, 340–350 RSC.
- C. Kropp, K. Le Corf, K. Relizani, K. Tambosco, C. Martinez, F. Chain, G. Rawadi, P. Langella, S. P. Claus and R. Martin, The Keystone commensal bacterium Christensenella minuta DSM 22607 displays anti-inflammatory properties both in vitro and in vivo, Sci. Rep., 2021, 11, 11494 CrossRef CAS PubMed.
- M. T. Alam, G. C. A. Amos, A. R. J. Murphy, S. Murch, E. M. H. Wellington and R. P. Arasaradnam, Microbial imbalance in inflammatory bowel disease patients at different taxonomic levels, Gut Pathog., 2020, 12, 1 CrossRef CAS PubMed.
- K. Relizani, K. Le Corf, C. Kropp, R. Martin-Rosique, D. Kissi, G. Dejean, L. Bruno, C. Martinez, G. Rawadi, F. Elustondo, W. Mazier and S. P. Claus, Selection of a novel strain of Christensenella minuta as a future biotherapy for Crohn's disease, Sci. Rep., 2022, 12, 6017 CrossRef CAS PubMed.
- P. Portincasa, L. Bonfrate, M. Vacca, M. De Angelis, I. Farella, E. Lanza, M. Khalil, D. Q. Wang, M. Sperandio and A. D. Ciaula, Gut Microbiota and Short Chain Fatty Acids: Implications in Glucose Homeostasis, Int. J. Mol. Sci., 2022, 23, 1105 CrossRef CAS PubMed.
- S. Deleu, K. Machiels, J. Raes, K. Verbeke and S. Vermeire, Short chain fatty acids and its producing organisms: An overlooked therapy for IBD?, EBioMedicine, 2021, 66, 103293 CrossRef CAS PubMed.
- D. P. Venegas, M. K. De la Fuente, G. Landskron, M. J. González, R. Quera, G. Dijkstra, H. J. M. Harmsen, K. N. Faber and M. A. Hermoso, Short chain fatty acids (SCFAs)-mediated gut epithelial and immune regulation and its relevance for inflammatory bowel diseases, Front. Immunol., 2019, 10, 277 CrossRef CAS PubMed.
- Z. Xu, W. Chen, Q. Deng, Q. Huang, X. Wang, C. Yang and F. Huang, Flaxseed oligosaccharides alleviate DSS-induced colitis through modulation of gut microbiota and repair of the intestinal barrier in mice, Food Funct., 2020, 11, 8077–8088 RSC.
|
This journal is © The Royal Society of Chemistry 2023 |
Click here to see how this site uses Cookies. View our privacy policy here.