DOI:
10.1039/D2FO02233H
(Paper)
Food Funct., 2023,
14, 250-261
Chemical composition and anti-inflammatory activities of Castanopsis honey†
Received
30th July 2022
, Accepted 4th November 2022
First published on 23rd November 2022
Abstract
Castanopsis is diffusely spread in tropical and subtropical regions and is an important nectar source plant in China. The Castanopsis honey (CH) is characterized by its bitter taste. However, its composition and functions remain unclear. In this study, the physicochemical parameters, chemical composition, and antioxidant capacity of CH were comprehensively investigated, with the anti-inflammatory effects of the Castanopsis honey extract (CHE) evaluated based on the RAW 264.7 cell inflammatory model. The results revealed a high level of quality in CH based on the quality standards. Among a total of 84 compounds identified in CH, 5 high response compounds and 29 phenols were further quantified by UPLC-Q/TOF-MS. The high content of phenylethylamine (117.58 ± 64.81 mg kg−1) was identified as a potential marker of CH. Furthermore, the CH showed evident antioxidant activities, and the anti-inflammatory activities of CHE were observed to inhibit the release of nitric oxide (NO) and reduce the content of tumor necrosis factor alpha (TNF-α) and improve the content of interleukin-10 (IL-10) by regulating the NF-κB pathway. Our study indicates that CH has sound physicochemical properties and biological activities with a high level of quality, providing strong experimental evidence to support the further economic and agricultural development and application of CH.
1. Introduction
As a natural sweet substance and healthy food, honey has been widely favored and is consumed by consumers worldwide with the global consumption of honey increasing for decades.1 The main chemical composition of honey generally contains fructose and glucose as well as small amounts of phenols, proteins, amino acids, organic acids, and volatiles with phenols generally considered the main active substances of honey.2 As a function food, honey has already been revealed to possess antioxidant, anti-inflammatory, anti-tumor, and other biological activities3,4 and has been diffusely applied in medical, nutrition, clinical, and pharmaceutical industries.2,5
Inflammation is the fundamental mechanism used by the immune system to protect the organism from inflammatory factors and harmful factors.6 However, if it is not controlled, inflammation may develop into chronic disorders, leading to tissue damage and illnesses such as cancer, obesity, and cardiovascular diseases; moreover, oxidative stress and inflammation are closely related and both synergistically play a crucial role in many chronic diseases.6 Considerable studies have focused on the antioxidant capacity as well as anti-inflammatory effects of antioxidant-rich foods.8 Especially, various types of honey are derived from different plant sources due to their high contents of phenolic acids and flavonoids. Studies have shown that both chestnut honey and eucalyptus honey can improve the inflammation of lipopolysaccharide (LPS) induced RAW 264.7 cells by decreasing the content of nitric oxide (NO) and regulating the expression of inducible NO synthase (iNOS), tumor necrosis factor alpha (TNF-α), nuclear factor kappa-light-chain-enhancer of activated B cells (NF-κB), and interleukin-10 (IL-10).7 Furthermore, manuka honey can reduce the levels of iNOS and inflammatory factors, i.e., TNF-α, interleukin-1β (IL-1β), and interleukin-6 (IL-6), through the TLR4/NF-κB pathway, with anti-inflammatory and antioxidant activities revealed in RAW 264.7 cells.6 In past decades, numerous studies have confirmed the anti-inflammatory and antioxidant activities of honey. However, among more than 300 types of unifloral honey worldwide, only a few types of honey, such as manuka honey, eucalyptus honey, rape honey, and safflower honey, have been investigated for their anti-inflammatory and antioxidant effects.6,7,9 Further studies are necessary to verify the findings in these previous investigations based on different types of honey to identify the chemical composition and reveal the relationships between the chemical composition of different types of honey and their anti-inflammatory effects.
The Castanopsis honey (CH) is produced from the nectar of plants in the genus Castanopsis of Fagaceae, with C. eyrei, C. sclerophylla, and C. carlesii as the common nectar sources. These plants diffusely spread in tropical and subtropical regions of Asia with a flowering period ranging from March to June10 and are an important nectar source in southern China. Plants in Castanopsis have been found to possess antioxidant, anti-inflammatory, and antibacterial capabilities and are routinely used in traditional medicines to treat stomach diseases, headaches, and other diseases.11,12 However, as far as we know, there are few research studies done on CH. The CH is a kind of honey with dark color and a bitter taste, suggesting potentially strong biological activities with significant economic and agricultural prospects. For example, dark honey usually has a higher content of phenols and stronger biological activities than light honey,5 while bitter honey is generally not only of higher economic value, with a market price four to eight times higher than that of regular honey,13 but also of significant potential in terms of biological activities and therapeutic effects.14 Furthermore, chestnut honey, which is also derived from plant sources belonging to Fagaceae, has been confirmed to be rich in active substances such as phenols with a variety of biological activities, including anti-inflammatory and antioxidant properties.7 However, studies about the physicochemical properties, chemical composition, and therapeutic effects of CH are lacking.
In this study, the CH collected from Jiangxi, China, was used to study its physicochemical properties, and the high response compounds, as well as phenolic acids and flavonoid components were characterized on account of UPLC-Q/TOF-MS. Furthermore, the antioxidant capacity and anti-inflammatory effects of CH and Castanopsis honey extract (CHE) were investigated in vitro, respectively. The results of our study revealed the significant potential of CH to develop high quality honey products, providing strong experimental evidence to support the further economic and agricultural development and application of CH.
2. Materials and methods
2.1. Honey samples
CH samples were harvested from six colonies of Apis cerana L. with the flowering period of Castanopsis spp. from 2020 to 2021 in Jiangxi, China. All samples were collected directly by different professional beekeepers and preserved at 4 °C in the dark until further analysis. All of plant sources of honey were identified by Prof. Xueyong Huang (Nanchang University, Nanchang, China).
The type and frequency of pollen (n ≥ 200) in the honey samples were counted and used as a basis for determining the kind of honey.15 Honey (1 g) was vortex mixed with 4 mL water and centrifuged at 3500 rpm for 15 min. The supernatant was discarded and 1 mL water was added to re-suspend and then observed with a microscope (Nikon Eclipse Ci). As shown in Fig. S1,† the main pollen in CH was Castanopsis pollen, which accounted for 70.38 ± 6.94% of the total pollens, and met the requirement of monofloral honey (>45%).15
2.2. Chemicals and reagents
Suger (fructose, glucose, sucrose), 2,2-diphenyl-1-picrylhydrazyl (DPPH), 3,4-dihydroxybenzoic acid, phenethylamine, tricoumaroyl spermidine, gallic acid, salicylic acid, syringic acid, p-coumaric acid, ellagic acid, trans-ferulic acid, genistein 7-O-glucoside, luteolin, hesperidin, p-hydroxybenzoic acid, myricetin, resveratrol, 3,4-dimethoxycinnamic acid, fisetin, biochanin A, daidzein, chrysin, 5-hydroxymethylfurfural (5-HMF), vanillic acid, and caffeic acid were obtained from Aladdin (Shanghai, China). Kynurenic acid, phenethyl caffeate, 4-hydroxyquinoline, rutin, quercetin, naringenin, kaempferol, apigenin, kaempferide, abscisic acid, and hesperetin were obtained from J&K Scientific. The purity of all chemicals was higher than 98%. The formic acid and acetonitrile used in UPLC-Q/TOF-MS were obtained from Thermo Fisher Scientific (Shanghai, China). Water used in the experiments was derived from an integrated ultra-pure water machine (Hkech-Master, Hetai Instruments, Shanghai, China).
Dulbecco's Modified Eagle Medium (DMEM), heat inactivated fetal bovine serum (FBS), dexamethasone (DEX), and antibiotics were obtained from the Gibco Invitrogen™ (Grand Island, NY, USA).
2.3. Determination of physicochemical properties
The physicochemical properties of honey were determined on account of the method of the International Honey Committee (IHC)16 and compared with the requirements of the Codex Alimentarius Commission, 2001 (CAC).17 In a word, abbe refractometer, pH meter, and electrical conductivity meter were used to measure the moisture, pH, and EC of honey. The free acidity and diastase activity of honey were determined by acid–base titration and spectrophotometry.
The 5-HMF and sugar composition (fructose, glucose, and sucrose) in honey were determined by the methods of IHC16 and Wang et al.5 The honey solution of 0.1 g mL−1 and 7.5 mg mL−1 were used to detect 5-HMF and sugar composition with HPLC, respectively.
The color of honey was determined by methods of Demir et al.18 with chromameter. All of CH with being homogenized completely were tested under a white background. The results were shown as the values of L* a* b*. The colour values were expressed as L* (0 and 100 indicated the degree of blackness and whiteness), a* (−a and +a indicated the degree of greenness and redness), and b* (−b and +b indicated the degree of blueness and yellowness).
2.4. Total phenolics and total flavonoids determination
The total phenolics content (TPC) was determined according to the method of Al et al.19 Briefly, 2.0 mL of 0.2 g mL−1 of honey solution was admixed with 2.0 mL of Folin–Ciocalteu reagent. 8.0 mL of 7.5% of Na2CO3 was added after 5 min. Finally, a total of mixed samples were incubated in the dark at 25 °C for 1 h. The absorbance was recorded at 760 nm.
The total flavonoid content (TFC) was determined according to the method of Bouayed et al.20 Briefly, 2.0 mL of 0.5 g mL−1 of honey was admixed with 0.30 mL of 5% of NaNO2 solution. 0.30 mL of 10% of Al(NO3)3 solution was mixed after 5 min. All of the mixed solutions were reacted with 2.0 mL of 4% of NaOH solution after 6 min. All solutions were incubated in the dark at 25 °C for 15 min. The absorbance was recorded at 510 nm.
Gallic acid and rutin were applied as a reference standard (20–1000 mg L−1). The results were denoted as mg gallic acid equivalent (GAE) per 100 g honey and mg rutin equivalent (RE) per 100 g honey.
2.5. UPLC-Q/TOF-MS analysis of CH
2.5.1. Pretreatment of honey.
All honey samples were dissolved at 35 °C and were homogenized before treatment. A total of all of the honey were prepared by the method of Zhu et al.21 to remove most of the sugar from honey. The honey (1 g) was added with 2 mL of 10% NaCl solution (pH regulated to 2 by hydrochloric acid). Then, the solution was mixed with 2 mL of acetonitrile, vortexed for 60 s to mix thoroughly, and centrifuged at 4000 rpm for 5 min to obtain the upper layer of organic matter. The extraction was repeated twice, and the organic matter was collected to fix to 10 mL.
The organic phase obtained by pretreatment of honey was collected, vacuum dried at 35 °C, and then redissolved in 0.1% dimethyl sulfoxide (DMSO) to obtain CHE of different concentrations. The different concentrations of LPS and DEX were dissolved in PBS. The 0.22 μm membrane filters were applied in all solutions in the experiment.
2.5.2. UPLC-Q/TOF-MS analysis.
The analysis was performed in light of our previous methods.5 The samples were detected by an HSS T3 column (2.1 × 100 mm, 1.8 μm) with 0.02% formic acid water (A) and 0.02% formic acid acetonitrile (B), with the following primary parameters: flow rate, 0.4 mL min−1; injection volume, 5 μL; column temperature, 40 °C.
2.6. Antioxidant capacities of CH
The determination of DPPH free radical scavenging capacity (DPPH+) of honey was according to the methodology of Scherer et al.22 The honey samples were dissolved at 0.008 to 0.2 g mL−1 with water. The honey group contained 80 μL honey solution added with 120 μL DPPH ethanol solution (25 μg mL−1). The control group contained 80 μL ultrapure water mixed with 120 μL DPPH solution. After mixing, all groups were reacted in the dark at 25 °C for 90 min. The absorbance was recorded at 517 nm. The inhibition rate of DPPH was calculated with the following equation:
Inhibition rate (%) = [(A1 − A2)/A1] × 100. A1 and A2 were the absorbance of the control group and the sample group, respectively. The results were displayed as 50% inhibition concentration (IC50) of DPPH.
Both ABTS free radical scavenging capacity (ABTS+) and ferric ion reducing antioxidant power (FRAP) of honey were determined using kits (Nanjing Jianceng Institute of Bioengineering, Nanjing, China) and concerning the methods of Chen et al.23 The results of ABTS+ and FRAP were presented as μmol Trolox per 100 g and μmol FeSO4·7H2O per 100 g of honey, respectively.
2.7. Cellular experiments
The RAW 264.7 macrophages were used in the inflammation experiments. The cells at an appropriate density were seeded on a Petri dish and were cultured with DMEM high glucose medium containing 10% FBS, streptomycin (100 μg mL−1), and penicillin (100 IU mL−1), and were incubated at 37 °C and 5% CO2 condition up till the adherence rate reached 70–80% to obtain the stable growth of the cells for further study.
2.7.1. Effects of CHE and LPS treatments on relative cell viability.
The viability of RAW 264.7 cells was measured by using the CCK-8 method (WST-8 solution). After adding different concentrations of LPS (1, 0.5, and 0.25 μg mL−1) and CHE (500, 250, and 125 μg mL−1) for 24 h, respectively, all samples were added with 10 μL of the WST-8 solution and incubated for another 1 h. The absorbance was recorded at 450 nm. The results were displayed as a percentage of the absorbance ratio of the treated cells to that of the control group (cells treated with growth medium only).
2.7.2. Cellular treatments.
The cells were separated into four groups: (a) the control group (no inflammatory cells) was untreated cells; (b) the negative control group (inflammatory cells) was cells treated with LPS (0.5 μg mL−1); (c) the positive control group (anti-inflammatory drug treatment) was cells treated with LPS (0.5 μg mL−1) after pretreatment with DEX (1 μg mL−1); (d) the experimental group (honey extract treatment) was cells treated with LPS after pretreatment with CHE (500, 250 and 125 μg mL−1). With 2 h of pretreatment with the drug and honey extract, LPS stimulation was applied for 24 h. The samples were centrifuged to collect the supernatants and cell precipitates for further study.
2.7.3. Determination of NO and inflammatory factors.
Based on the method of Green et al.,24 the effects of CHE and DEX on the relative concentration of NO in RAW 264.7 cells after LPS stimulation were determined by measuring the NO accumulation in the supernatants. The absorbance was recorded at 540 nm. In four different groups of RAW 264.7 cells as described above, 100 μL of supernatant and the same equivalent amounts of Griess A reagent (1% sulfanilamide with 5% phosphoric acid) and Griess B reagent (0.1% N-(1-naphthyl) ethylenediamine dihydrochloride) were mixed in a 96-well microtiter plate. All samples were reacted in the dark at 25 °C for 10 min.
The enzyme linked immunosorbent assay (ELISA) kits were used to detect the cytokines, including TNF-α, IL-1β, and IL-10 (item numbers 88-724-88, 88-7013-88, 88-7105-88, Thermo Fisher Scientific, Shanghai, China). All results were expressed as a percentage of the absorbance of the negative control group.
2.7.4. Effects of CHE on NF-κB p65 after the LPS treatment.
After centrifugation, the four groups of treated cell precipitate were washed twice and lysed with cell lysate for 30 min on ice. The protein concentration was measured with the bicinchoninic acid protein concentration assay kit (Servicebio, G2026). The protein concentration was adjusted, and the samples were boiled and then analyzed by electrophoresis. The gel was transferred to a polyvinylidene fluoride (PVDF) membrane, which was kept in ice water with 5% bovine serum albumin for 1 h. Then, the primary antibody was added to the membrane and incubated overnight at 4 °C. The membrane was washed by Tris Buffered Saline Tween (TBST), and the secondary antibody was added for incubation. After 30 min, the color was developed with the enhanced chemiluminescence (ECL) reagent. The images were collected by scanning and archiving the film, sorting out the color, and analyzing the optical density value of the target band by Alpha software (alphaEaseFC) processing system. The results were expressed as the ratio of the gray value of the target band to that of the internal reference band (β-actin).
2.8. Statistical analysis
All data were from more than three repeated tests. Data were exported to Microsoft Excel and expressed as mean ± standard deviation (SD). One-way ANOVA and Tukey's test were performed using IBM SPSS Statistics 19 (Armonk, New York, USA) to analyze the significance of differences with p < 0.05 considered statistically significant.
3. Results and discussion
3.1. Physicochemical properties, TPC, and TFC of CH
The results of the physicochemical properties, TPC, and TFC are shown in Table 1. The moisture content of CH was 17.50% to 19.70%. The climatic conditions, collection methods, and the maturity of honey are essential factors affecting the moisture content of honey.25 The moisture content of all samples was lower than 20%, meeting the requirements of the CAC. The free acidity content of CH ranged from 14.00 meq kg−1 to 32.00 meq kg−1, which was lower than the requirement (50 meq kg−1) by the CAC. Studies show that as an essential parameter in the honey fermentation process, the free acidity content of honey increases with the fermentation of honey.25 The pH values of CH samples ranged from 4.17 to 4.41, indicating the acidic environments in the honey samples, which was beneficial in inhibiting microbial growth.26 The 5-HMF is a significant standard to evaluate the freshness of honey. The content of 5-HMF in fresh honey is usually low but will be increased if the honey is in long-term storage or heated.25 The average content of 5-HMF in CH was 6.83 ± 8.91 mg kg−1, which was lower than the requirement of CAC (40 mg kg−1), indicating that CH samples were in fresh conditions. The average diastase activity of CH was 9.72 ± 4.24 Schade units, which was higher than the standard (8.00 Schade units) specified by the CAC. However, the diastase activity of some samples was lower than 8.00 Schade units, probably caused by the plant sources of honey, species of bee, nectar harvesting period, nectar flow, and nectar composition, as reported previously.27 The EC is related to the plant origins of the honey and can be applied in distinguishing honeydew honey from blossom honey. For example, the EC of honeydew honey is higher than 0.8 mS cm−1,28 while the blossom honey or a mixture of blossom honey and honeydew honey would show EC values lower than 0.8 mS cm−1. Our results showed that the EC values of CH samples were 0.43 mS cm−1 to 0.65 mS cm−1, indicating that these CH samples were either blossom honey or mixtures of honeydew honey and blossom honey, meeting the requirement of CAC (≤0.8 mS cm−1).
Table 1 The physicochemical properties, i.e., moisture, free acidity, pH, 5-hydroxymethylfurfural (5-HMF), diastase activity, electrical conductivity (EC), sugar composition, color, and total phenolic content (TPC) and total flavonoid content (TFC) of Castanopsis honey
Parameter |
Average ± SD |
Minimum |
Maximum |
Standard value |
Standard value: the parameters in honey required by the Codex Alimentarius Commission (2001). “nd” indicates not detected. |
Moisture (%) |
18.56 ± 0.97 |
17.50 |
19.70 |
≤20 |
Free acidity (meq kg−1) |
25.20 ± 6.90 |
14.00 |
32.00 |
≤50 |
pH |
4.28 ± 0.10 |
4.17 |
4.41 |
|
5-HMF (mg kg−1) |
6.83 ± 8.91 |
0.23 |
23.22 |
≤40 |
Diastase activity (Schade units) |
9.72 ± 4.24 |
4.43 |
14.29 |
≥8 |
EC (mS cm−1) |
0.54 ± 0.10 |
0.43 |
0.65 |
≤0.8 |
Sugar composition
|
|
|
|
|
Fructose (%) |
31.91 ± 3.23 |
27.56 |
36.59 |
≥60 |
Glucose (%) |
25.80 ± 2.58 |
23.45 |
29.32 |
|
Sucrose (%) |
1.14 ± 0.76 |
nd |
2.13 |
≤5 |
Fructose/glucose ratio |
1.24 ± 0.10 |
1.18 |
1.41 |
|
Color CIE L* a* b*
|
|
|
|
|
L* |
43.88 ± 3.21 |
41.29 |
47.47 |
|
a* |
5.54 ± 2.25 |
2.98 |
7.21 |
|
b* |
15.80 ± 5.96 |
10.39 |
22.18 |
|
TPC (mg GAE per 100 g honey) |
115.25 ± 26.35 |
72.59 |
139.89 |
|
TFC (mg RE per 100 g honey) |
12.36 ± 2.19 |
9.53 |
15.41 |
|
Sugar is the major component of honey, about 60–80%, with both fructose and glucose as the main components, among other sugars like sucrose, maltose, and trehalose were also included.29,30 Our results showed that the content of both fructose and glucose was 57.71 ± 5.41%, which was lower than the value (60%) required by CAC. The sugar composition of honey is related to its plant and geographical sources.2 For example, studies have found that the sugar content of chestnut honey (same belonging to Fagaceae) is also lower than 60%.30 These results suggested that the low sugar content might be a characteristic of CH, causing its taste to be less sweet than other types of honey. As the honey ripens, sucrose is continuously converted to fructose and glucose, resulting in generally lower sucrose content in mature honey.29 The results showed that the sucrose content of CH was 1.14 ± 0.76%, which was lower than the standard (5%) required by CAC. The ratio of fructose/glucose is used to evaluate the crystallization state of honey, while the honey crystallizes more easily when the fructose/glucose ratio is less than 1.31 Our results revealed the fructose/glucose ratio of 1.24 ± 0.10, indicating that the CH crystallized not easily at room temperature.
The color of honey is related to its plant origin, geographical origin, and elemental composition. Dark honey generally contains higher contents of phenolic acids and flavonoids than those light honey.32 In our study, the L* value of CH was 43.88 ± 3.21 (Table 1), which is near to the value of chestnut honey (43.53 ± 3.34)32 and lower than those of the black locust honey (87.20 ± 1.60) and asphodel honey (86.70 ± 4.30).33 A lower L* value suggested that CH was a kind of dark honey, similar to chestnut honey, and darker than both locust honey and asphodel honey. The a* and b* values of CH were 5.54 ± 2.25 and 15.80 ± 5.96, respectively, indicating that CH was reddish and yellowish.
Phenolic acids and flavonoids are the main active substances in honey. Studies showed that honey with high TPC and TFC generally has high nutritional functions.2 As shown in Table 1, our results showed that the TPC of CH was 115.25 ± 26.35 mg GAE per 100 g honey, which was much higher than those reported for some other plant sources of honey, such as black locust honey (14.28 ± 1.60 mg GAE per 100 g) and goldenrod (17.34 ± 3.67 mg GAE per 100 g).34 CH was also rich in flavonoids; the TFC of CH was 12.36 ± 2.19 mg RE per 100 g, which was higher than eucalyptus honey (6.91 ± 0.17 mg RE per 100 g) and some commercial honey (8.09 ± 0.15 mg RE per 100 g).35 These results suggested the potent biological activities in CH samples due to their high TPC and TFC.
3.2. Analysis of compounds in CH by UPLC-Q/TOF-MS
The chemical composition of CH was detected by UPLC-Q/TOF-MS. Five high response compounds were identified among many high response peaks observed in the chromatograms (Fig. 1). The fragmentation behavior of these substances in honey and those of the corresponding standard substance in the positive ion mode are shown in Fig. 2. Compound A was identified as phenethylamine with a parent ion of m/z 122.0958, which mainly formed fragment of C8H8 (m/z 105.0697) by losing NH3. The result was consistent with the fragmentation behavior of the standard. The main fragment of compound B (C10H12N2O3, m/z 190.0494) was C9H6NO (m/z 144.0444), which lost the CH2O2 fragment during the fragmentation behavior process, as reported previously by Wang et al.5 These results were consistent with the fragmentation behavior of the standard, supporting the identification of compound B as kynurenic acid. Compound C was identified as 4-hydroxyquinoline by lost NO to form a fragment of C9H6N (m/z 128.0498) and compound D was identified as abscisic acid according to the main fragment ions C15H19O3 (m/z 247.1338) and C15H17O2 (m/z 229.1228) as well as the standard. Compound E was identified as tricoumaramide spermidine based on fragments of C25H32N3O4 (m/z 438.2381), C25H30N3O3 (m/z 420.2276), and C12H14NO2 (m/z 204.1018).
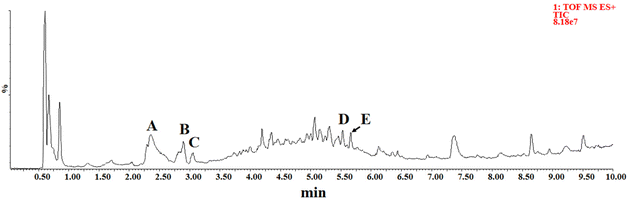 |
| Fig. 1 UPLC-Q/TOF-MS spectrum of Castanopsis honey in positive ion mode showing five high response peaks representing. (A) Phenethylamine, (B) kynurenic acid, (C) 4-hydroxyquinoline, (D) abscisic acid, and (E) tricoumaroyl spermidine. | |
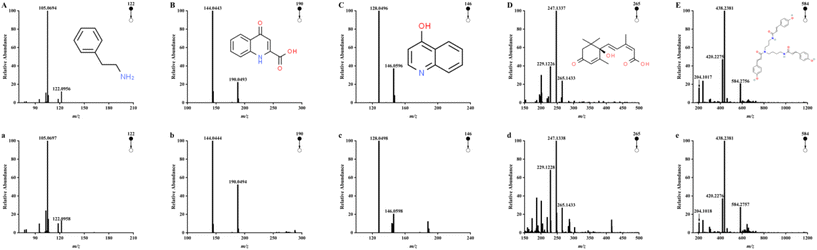 |
| Fig. 2 The mass spectrometry and fragment spectra of 5 high response compounds (a–e) from Castanopsis honey and the fragment spectra of their corresponding standards (A–E) in positive ion mode. (A and a) phenethylamine; (B and b) kynurenic acid; (C and c) 4-hydroxyquinoline; (D and d) abscisic acid; (E and e) tricoumaroyl spermidine. | |
These five high response compounds were quantified with the standard (Table 2). The content of phenylethylamine in CH was 117.58 ± 64.81 mg kg−1. Phenethylamine is a neuroactive biogenic amine, generally formed by organisms through the decarboxylation of phenylalanine and found in ham (182 mg kg−1), fish products (180 mg kg−1), cheese (61 mg kg−1), and vegetables (9.3 mg kg−1).36 As a well-known neuroactive amine, phenethylamine is gaining popularity because of its multiple functions such as heart rate increase, euphoria, and antidepressant, while it can be used as a nutritional supplement to improve mood, enhance well-being, increase concentration, lose weight, and enhance athletic performance.37 Phenethylamine is generally considered to be produced with the process of microbial fermentation in foods (e.g., ham and cheese).36 However, as an antimicrobial food, honey is rarely fermented and is unsuitable for microbial growth,4 suggesting that the high content of phenethylamine in CH is more probably from the nectar of Castanopsis plants. This conclusion is closely related to the studies of Zhang et al., who found phenethylamine in the flowers of Castanopsis plants and suggested that it may contribute to the attraction of flower visitors.38 Although biogenic amines exist widely in foods, they usually present a low concentration in honey (lower than 11.32 mg kg−1).39 This study was the first to reveal the highest content of phenethylamine in monofloral honey among the studies reported previously. Therefore, phenethylamine could be assigned as a potential marker for CH, and high content of phenethylamine may provide stronger potential activity to it.
Table 2 Contents of five high response compounds (mg kg−1 honey) detected in Castanopsis honey and their retention time (RT), regression equation, and R2
Compound name |
RT (min) |
Average concentration |
Concentration ranges |
Regression equation y = ax + b |
R
2
|
Phenethylamine |
2.35 |
117.58 ± 64.81 |
29.89–236.73 |
y = 917.15x + 1860.7 |
0.9974 |
Kynurenic acid |
2.87 |
5.00 ± 1.98 |
3.26–7.16 |
y = 118.32x − 18 061 |
0.9952 |
4-Hydroxyquinoline |
3.01 |
2.03 ± 0.47 |
1.49–2.32 |
y = 7044.3x + 10 426 |
0.9975 |
Abscisic acid |
5.51 |
3.57 ± 1.99 |
1.44–5.39 |
y = 470.01x + 5429.2 |
0.9907 |
Tricoumaroyl spermidine |
5.63 |
1.69 ± 1.20 |
0.69–3.03 |
y = 2004.5x − 22 530 |
0.9964 |
As shown in Table 2, the contents of kynurenic acid and 4-hydroxyquinoline in CH were 5.00 ± 1.98 mg kg−1 and 2.03 ± 0.47 mg kg−1, respectively. Both kynurenic acid and 4-hydroxyquinoline were also detected in Dendropanax dentiger honey and chestnut honey,5,40 with higher contents than those of the CH revealed in our study. The content of abscisic acid in CH was 3.57 ± 1.99 mg kg−1, which is lower than that in several other types of unifloral honey, such as Dendropanax dentiger honey, acacia honey, and linden honey.5,41 The content of tricoumaroyl spermidine in CH was 1.69 ± 1.20 mg kg−1. Although there are few reports on the contents of tricoumaroyl spermidine in honey, our unpublished data showed evidently that tricoumaroyl spermidine was found in a variety of honey, while studies have indicated that the tricoumaroyl spermidine may be derived from bee pollen.42
Other compounds identified in CH were also analyzed by UPLC-Q/TOF-MS (Table S1†). In the aggregate, 84 compounds were identified in CH, including 22 organic acids, 17 flavonoids, 12 phenolic acids, 13 amino acids and nucleotides, 11 nitrogen-containing organic compounds, and 9 other substances. The organic acids detected in CH mainly included abscisic acid, D-gluconic acid, and 2-hydroxybutyric acid, with abscisic acid showing the highest response. The amino acids and nucleotides detected in CH mainly included proline, phenylalanine, and adenine. The nitrogen-containing organics mainly included alkaloids such as kynurenic acid, 4-hydroxyquinoline, and phenethylamine, with kynurenic acid showing the highest response. In addition, other substances such as 5-HMF, salicylic alcohol, and p-hydroxybenzaldehyde were also detected in CH.
3.3. Quantification of phenolic compounds in CH
Phenolic compounds are the main active substances in honey, and different phenolic compounds make their unique contributions to the activity, color, taste, and flavor of honey.2 A total of 29 phenolic compounds identified in CH were further quantified by UPLC-Q/TOF-MS (Table 3).
Table 3 Contents of 29 phenolic compounds (μg per 100 g honey) detected in Castanopsis honey by UPLC-Q/TOF-MS and their retention time (RT), regression equation, and R2
Compound name |
RT (min) |
Average concentration |
Concentration ranges |
Regression equation (y = ax + b) |
R
2
|
“nd” indicates not detected. |
Gallic acid |
1.44 |
2.27 ± 0.17 |
2.12–2.46 |
y = 115.75x − 1720.6 |
0.9879 |
3,4-Dihydroxybenzoic acid |
2.36 |
2.20 ± 0.75 |
1.33–2.56 |
y = 235.91x + 159.08 |
0.9998 |
Salicylic acid |
3.21 |
5.35 ± 0.75 |
4.65–5.14 |
y = 148.13x − 527.7 |
0.9987 |
Chlorogenic acid |
3.36 |
0.87 ± 0.23 |
0.67–1.12 |
y = 180.87x − 773.65 |
0.9988 |
Vanillic acid |
3.59 |
1.73 ± 0.28 |
1.43–1.99 |
y = 31.897x + 77.563 |
0.9999 |
Caffeic acid |
3.70 |
0.59 ± 0.19 |
0.46–0.81 |
y = 497.08x − 344.32 |
0.9999 |
Syringic acid |
3.76 |
0.64 ± 1.11 |
nd–1.92 |
y = 100.85x − 691.44 |
0.9995 |
Rutin |
4.12 |
2.95 ± 2.64 |
nd–5.01 |
y = 1747.5x + 26 310 |
0.9901 |
p-Coumaric acid |
4.20 |
7.75 ± 1.72 |
7.70–9.74 |
y = 345.73x + 11 865 |
0.9979 |
Ellagic acid |
4.37 |
25.86 ± 10.85 |
17.85–38.20 |
y = 193.32x − 69.029 |
0.9997 |
trans-Ferulic acid |
4.52 |
1.71 ± 0.08 |
1.64–1.79 |
y = 456.32x + 6585.8 |
0.9970 |
Genistein 7-O-glucoside |
4.53 |
2.32 ± 2.01 |
nd–3.56 |
y = 684.56x + 10 742 |
0.9971 |
Luteolin |
4.58 |
1.07 ± 0.18 |
0.88–1.25 |
y = 166.81x − 310.86 |
0.9999 |
Hesperidin |
4.73 |
0.22 ± 0.38 |
nd–0.65 |
y = 167.87x + 2436.6 |
0.9998 |
p-Hydroxybenzoic acid |
4.92 |
0.37 ± 0.64 |
nd–1.11 |
y = 429.43x + 5119.9 |
0.9958 |
Myricetin |
4.96 |
1.20 ± 0.03 |
1.16–1.23 |
y = 806.81x − 8826.8 |
0.9976 |
Resveratrol |
5.20 |
0.02 ± 0.04 |
nd–0.07 |
y = 763.94x + 1318.4 |
0.9981 |
3,4-Dimethoxycinnamic acid |
5.32 |
1.56 ± 1.36 |
nd–2.45 |
y = 181.24x − 3752.4 |
0.9949 |
Fisetin |
5.51 |
1.83 ± 0.98 |
0.70–2.44 |
y = 2141.1x − 4015.3 |
0.9990 |
Quercetin |
5.54 |
6.16 ± 0.51 |
5.59–6.56 |
y = 1255.6x + 711.61 |
0.9980 |
Naringenin |
6.00 |
4.83 ± 1.55 |
3.48–6.53 |
y = 1954.9x + 357.64 |
0.9997 |
Kaempferol |
6.11 |
2.86 ± 0.06 |
2.80–2.93 |
y = 3050.6x − 9228.6 |
0.9987 |
Apigenin |
6.12 |
1.58 ± 0.76 |
0.98–2.44 |
y = 1907x + 1913.3 |
0.9979 |
Kaempferide |
6.18 |
42.96 ± 32.47 |
10.74–75.68 |
y = 15.839x − 4.9083 |
0.9992 |
Hesperetin |
6.22 |
0.23 ± 0.09 |
0.13–0.30 |
y = 2328.6x − 1106.1 |
0.9999 |
Biochanin A |
7.13 |
44.94 ± 12.50 |
31.05–55.28 |
y = 9.5705x − 1447.6 |
0.9899 |
Daidzein |
7.49 |
7.77 ± 3.65 |
3.60–10.37 |
y = 739.24x + 210.49 |
0.9997 |
Phenethyl caffeate |
7.77 |
0.32 ± 0.21 |
0.09–0.50 |
y = 2600.8x + 8087.5 |
0.9893 |
Chrysin |
7.79 |
69.80 ± 24.11 |
55.51–97.64 |
y = 148.13x − 527.7 |
0.9987 |
The climate, environment, and storage time all affected the phenolic composition of honey,2 which were probably the causes for slight variations in the phenolic composition of different CH samples. Among the total of 29 phenols detected, 22 phenolic compounds were detected in all honey, including gallic acid, 3,4-dihydroxybenzoic acid, salicylic acid, chlorogenic acid, vanillic acid, caffeic acid, p-coumaric acid, ellagic acid, trans-ferulic acid, luteolin, myricetin, fisetin, quercetin, naringenin, kaempferol, apigenin, kaempferide, hesperetin, biochanin A, daidzein, phenethyl caffeate, and chrysin. The highest content of phenols detected is chrysin (69.80 ± 24.11 μg per 100 g), followed by biochanin A (44.94 ± 12.50 μg per 100 g), kaempferol (42.96 ± 32.47 μg per 100 g), and ellagic acid (25.86 ± 10.85 μg per 100 g). Chrysin is a flavonoid compound with various biological activities like antioxidant, anti-inflammatory, and neuroprotective. Honey is rich in chrysin (10.00–530.00 μg per 100 g), which can provide honey with antioxidant and anti-inflammatory activities.43 Biochanin A is an antioxidant compound commonly found in honey (0.70 μg per 100 g), honeycombs, and various dietary supplements.44–46 Kaempferol is an antioxidant with free radical scavenging ability, which can effectively prevent oxidative stress.47 Malaysian honey is rich in ellagic acid (626.74 ± 56.2 μg per 100 g), which has been found to possess anti-inflammatory activity in vitro.48 In a word, these results of UPLC-Q/TOF-MS showed that CH is rich in biological compounds such as various phenolic acids and flavonoids, suggesting the potential biological activities of CH.
3.4. Antioxidant capacity of CH in vitro
DPPH+, ABTS+, and FRAP are widely applied to quantitatively determine the antioxidant capacity of natural products, plant extracts, and honey. DPPH free radical is a kind of stable nitrogen center free radical, reflecting the substance's antioxidant capacity by capturing the free radical. The oxidation of ABTS, generating the blue-green ABTS free radical, is inhibited in the presence of antioxidants. The ability of honey samples to reduce Fe3+ directly reflected the antioxidant capacity of the samples, and they can reflect the antioxidant capacity of the samples from different levels.5,49
As shown in Table 4, the DPPH+ IC50 value of CH was 25.64 ± 7.38 mg mL−1 honey. The result revealed a stronger DPPH radical scavenging ability in CH than several other types of unifloral honey reported previously, like loquat honey (98–103 mg mL−1 honey) and Dendropanax dentiger honey (71.91 ± 19.10 mg mL−1 honey).5,41 The ABTS+ value of CH was 62.84 ± 20.79 μmol Trolox per 100 g honey, which was close to that of Dendropanax dentiger honey (56.48 ± 14.56 μmol Trolox per 100 g honey).5 The FRAP value of CH was 118.06 ± 17.49 μmol FeSO4·7H2O per 100 g honey, which was more potent than some other types of unifloral honey such as Dendropanax dentiger honey (58.38 ± 10.20 μmol FeSO4·7H2O per 100 g honey).5 These results showed that CH contained a stronger antioxidant capacity than that of some other types of monofloral honey. Because the antioxidant capacity of honey depends on the active compounds, therefore, the higher antioxidant capacity of CH could be caused by the active compounds with high contents, mainly phenolic acids and flavonoids.
Table 4 Antioxidant activity of Castanopsis honey based on in vitro assays of DPPH free radical-scavenging activity (DPPH+), ABTS + cation radical-scavenging activity (ABTS+), and ferric reducing antioxidant power (FRAP)
Parameter |
Average |
Minimum |
Maximum |
DPPH IC50 (mg mL−1) |
25.64 ± 7.38 |
21.82 |
38.81 |
ABTS+ (μmol Trolox per 100 g) |
62.84 ± 20.79 |
39.07 |
79.47 |
FRAP (μmol FeSO4·7H2O per 100 g) |
118.06 ± 17.49 |
96.80 |
144.20 |
3.5. Anti-inflammatory activities by CHE treatment in vitro
3.5.1. Effects of CHE and LPS on the survival of RAW 264.7 cells.
It is known that LPS stimulates macrophages to produce cytokines, which interact with multiple target cells through receptors as endogenous mediators of inflammation.50 In this study, the anti-inflammatory effects of CHE were measured by the LPS induced RAW 264.7 cell model. The highest concentration of LPS (1 μg ml−1) showed a significant effect on cell viability (p < 0.05), so concentrations of 500, 250, and 125 μg mL−1 of CHE and 0.5 μg ml−1 of LPS were used for subsequent experiments (Fig. 3).
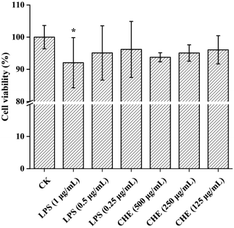 |
| Fig. 3 Viability of RAW 264.7 macrophages after treatments of lipopolysaccharide (LPS) and Castanopsis honey extract (CHE) with different concentrations. CK is the control group without inflammation. “*” represents a significant difference between groups (p < 0.05). The results are expressed as the percentage of viable cells (CK). | |
3.5.2. Effects of CHE and LPS on NO and inflammatory cytokines.
NO is a biologically active free radical and an essential biological regulatory molecule in the immune system as well as a signal molecule in various physiological and pathological processes. Excessive production of NO can promote the occurrence of inflammation.6 As shown in Fig. 4A, the anti-inflammatory drug DEX was the most prominent in decreasing the production of NO by 37.7%. CHE with different concentrations could also significantly decrease the level of NO, and the higher the dose of CHE, the stronger the inhibitory effect on NO, suggesting a significant dose-dependent effect. It was reported previously that phenolic compounds in honey reduced NO production, and the effect was closely related to its concentration.50 Furthermore, both manuka honey and stingless honey can significantly reduce the production of NO due to the phenolic compounds in honey.6 Therefore, these results suggested that CHE reduced the level of NO due to the multiple types of phenolic compounds.
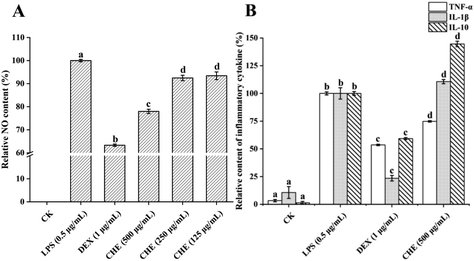 |
| Fig. 4 Relative contents of nitric oxide (NO) (A) and TNF-α, IL-1β, and IL-10 levels (B) of RAW 264.7 macrophages treated with lipopolysaccharide (LPS), dexamethasone (DEX), and Castanopsis honey extract (CHE). CK is the control group without inflammation. Different letters a, b, c, and d represent the significant difference between groups (p < 0.05). The results were expressed as a percentage of the LPS-treatment group. | |
TNF-α is one of the pro-inflammatory cytokines produced by macrophages, playing a multifunctional role in congenital reactions by up-regulating the production of several other types of pro-inflammatory cytokines.51 As shown in Fig. 4B, LPS conspicuously increased the TNF-α level in cells, while the CHE treatment significantly reduced the TNF-α level in inflammatory cells (p < 0.05). These results were consistent with studies reported previously on the effect of honey, such as manuka honey, chestnut honey, and eucalyptus honey, on LPS-induced inflammation.52 More and more research has shown that a variety of phenolic compounds in honey, such as chrysin, can reduce the level of TNF-α, thus playing an anti-inflammatory effect. The CHE reduced the TNF-α level due to its various types of phenols, in particular, the chrysin with high content.
IL-1β is one of the inflammatory cytokines with a large pro-inflammatory activity and can induce a variety of cytokines and chemokines.9 The IL-1β levels were significantly increased by both the LPS and CHE treatments but were significantly reduced by the DEX treatment. The result was different from those reported by other studies, such as manuka honey lowered the IL-1β levels and thus exhibited anti-inflammatory effects.6 However, other studies have found that honey promoted IL-1β expression in mice.53 This could be due to the complex chemical composition in honey, not only being a pure anti-inflammatory agent but also acting on different cellular pathways of macrophages.50
IL-10 is an anti-inflammatory factor that reduces inflammation levels by reducing the production of pro-inflammatory cytokines and enzymes like TNF-α, interferon-γ, and iNOS.7 In our study, the IL-10 levels were conspicuously strengthened by the CHE treatment contrasted with the LPS-treated group, suggesting that CHE could play a significant part in anti-inflammatory activities by increasing the IL-10 levels. Studies have shown that several types of honey, such as chestnut honey, eucalyptus honey, and manuka honey, have shown anti-inflammatory effects by boosting the IL-10 levels, similar effects caused by a variety of natural compounds such as kaempferol and resveratrol.6,7,54 In conclusion, CHE showed a strong anti-inflammatory effect due to its rich phenolic compounds, which could play a significant part in anti-inflammatory activities by reducing the levels of NO and pro-inflammatory factor TNF-α as well as increasing the level of IL-10.
3.5.3. Effect of CHE on the nuclear localization of NF-κB p65.
Transcription factor NF-κB plays a significant part in inflammation by adjusting the gene expression of multiple inflammatory cytokines. In the presence of pro-inflammatory factors (physical, chemical, and biological), inhibitor of kappa B (IκB) is phosphorylated and rapidly degraded. The NF-κB subunit is transferred to the nucleus to stimulate the transcription of various genes coding chemokines, cytokines, and inflammatory factors.55 As shown in Fig. 5, the protein levels of subunit NF-κB p65 were increased significantly by the LPS induction, while the levels of NF-κB p65 were reduced remarkably after the CHE treatment. The NF-κB pathway is associated with a variety of biochemical processes as a key inflammatory regulatory pathway, such as oxidative stress, which plays an important role in the process of inflammation. The LPS can cause oxidative stress in RAW 264.7 cells by stimulating the mitogen-activated protein kinase (MAPK) pathway.56 Oxidative stress induces various modifications in lipids, generating several oxidized products and promoting the production of cytokines, such as TNF-α, IL-6, and IL-1, which will then induce phosphorylation of NF-κB and stimulate inflammation generation.56 Furthermore, reactive oxygen species promote inflammation through the activation of redox-sensitive factors, such as NF-κB and activator protein 1 (AP-1).57 Therefore, the good antioxidant capacity exhibited by CH may contribute to its ability to reduce inflammation. Besides, studies have shown that LPS stimulation is able to decrease the production of a variety of inflammatory compounds, while a variety of plant derived bioactive compounds and honey can inhibit the inflammation of LPS-stimulated cells.2 For example, studies have shown that both chestnut honey and eucalyptus honey can improve the inflammation of LPS-induced RAW 264.7 cells by decreasing the content of NO and regulating the expression of iNOS, TNF-α, and IL-10, through the NF-κB pathway.7 Furthermore, the manuka honey can reduce the levels of iNOS and inflammatory factors, i.e., TNF-α, IL-1β, and IL-6, through the TLR4/NF-κB pathway, with anti-inflammatory and antioxidant activities revealed in RAW 264.7 cells.6 These results were consistent with our findings, indicating that CHE could adjust the expression of multiple inflammatory factors by restraining the protein expression of NF-κB p65, thus playing an anti-inflammatory effect. Both chrysin and ellagic acid significantly down-regulated the NF-κB expression, reducing the iNOS biosynthesis, and ultimately inhibiting the NO production.48 Vanillic acid showed anti-inflammatory and analgesic effects by restraining the manufacture of pro-inflammatory cytokines related to NF-κB. Gallic acid can reduce LPS-induced activation of TLR4/NF-κB to reduce inflammation in RAW 264.7 cells.9 Because CH was rich in these phenols, which could significantly contribute to the anti-inflammatory effects of CHE.
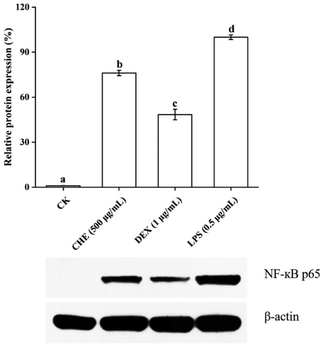 |
| Fig. 5 The effect of lipopolysaccharide (LPS), dexamethasone (DEX), and Castanopsis honey extract (CHE) on the regulatory protein NF-κB p65 of inflammation. β-Actin is the internal reference. CK is the control group without inflammation. Different letters a, b, c, and d represent the significant difference between groups (p < 0.05). The results were expressed as a percentage of LPS-treatment group. | |
4. Conclusions
On the whole, we characterized the physicochemical properties of CH samples from Jiangxi, China, showing that most of the indicators were in line with the requirements of the CAC (2001), except for the slightly lower sugar content. A total of 84 compounds in CH were identified by UPLC-Q/TOF-MS analysis, with 29 phenolic compounds further quantified. The high content of phenylethylamine suggested that it could be used as a potential marker of CH. The CH showed a strong scavenging ability of DPPH+, ABTS+ radical, and ferric ion. The CHE was found to activate the NF-κB signaling pathway and plays a significant part in anti-inflammatory activity by inhibiting the levels of NO and TNF-α and increasing the levels of anti-inflammatory factor IL-10. This study provided strong experimental evidence to support the economic and agricultural development and application of CH and to improve public understanding of the health benefits and commercial value of CH.
Author contributions
Wenjie Yu: Conceptualization, methodology, validation, investigation, writing – original draft, visualization. Fengjie Sun: Writing – review & editing, visualization. Ruixin Xu: Conceptualization, writing – review & editing, methodology. Meng Cui: Writing – review & editing, visualization. Yongquan Liu: Writing – review & editing, visualization. Quanyuan Xie: Conceptualization, writing – review & editing, methodology. Limin Guo: Validation, investigation. Chenxian Kong: Validation, investigation. Xin Li: Validation, investigation. Xiali Guo: Validation, investigation. Liping Luo: Conceptualization, methodology, visualization, resources, writing – review & editing, supervision, project administration, funding acquisition.
Conflicts of interest
There are no conflicts to declare.
Acknowledgements
This work was supported by Jiangxi Agriculture Research System (No. JXARS-14) and the Science and Technology Department of Jiangxi Province (20212AEI91010).
References
- S. Yan, K. Wang, Y. Al Naggar, Y. Vander Heyden, L. Zhao, L. Wu and X. Xue, Natural plant toxins in honey: An ignored threat to human health, J. Hazard. Mater., 2022, 424, 127682 CrossRef CAS PubMed.
- P. M. Da Silva, C. Gauche, L. V. Gonzaga, A. C. O. Costa and R. Fett, Honey: Chemical composition, stability and authenticity, Food Chem., 2016, 196, 309–323 Search PubMed.
- R. K. Kishore, A. S. Halim, M. S. N. Syazana and K. N. S. Sirajudeen, Tualang honey has higher phenolic content and greater radical scavenging activity compared with other honey sources, Nutr. Res., 2011, 31, 322–325 CrossRef CAS PubMed.
- S. Nikhat and M. Fazil, History, phytochemistry, experimental pharmacology and clinical uses of honey: A comprehensive review with special reference to Unani medicine, J. Ethnopharmacol., 2022, 282, 114614 Search PubMed.
- L. Wang, F. Ning and T. Liu,
et al., Physicochemical properties, chemical composition, and antioxidant activity of Dendropanax dentiger honey, LWT – Food Sci. Technol., 2021, 147, 111693 Search PubMed.
- M. Gasparrini, S. Afrin and T. Y. Forbes-Hernández,
et al., Protective effects of Manuka honey on LPS-treated RAW 264.7 macrophages. Part 2: Control of oxidative stress induced damage, increase of antioxidant enzyme activities and attenuation of inflammation, Food Chem. Toxicol., 2018, 120, 578–587 CrossRef CAS PubMed.
- D. Cianciosi, T. Y. Forbes-Hernandez and J. M. Alvarez-Suarez,
et al., Anti-inflammatory activities of Italian Chestnut and Eucalyptus honeys on murine RAW 264.7 macrophages, J. Funct. Foods, 2021, 87, 104752 Search PubMed.
- M. Battino, F. Giampieri and D. Cianciosi,
et al., The roles of strawberry and honey phytochemicals on human health: A possible clue on the molecular mechanisms involved in the prevention of oxidative stress and inflammation, Phytomedicine, 2021, 86, 153170 Search PubMed.
- L. Sun, F. Shi, W. Zhang, Z. Zhang and K. Wang, Antioxidant and anti-inflammatory activities of safflower (Carthamus tinctorius L.) honey extract, Foods, 2020, 9, 1039 CrossRef CAS PubMed.
- C. Bishan, L. Bing and C. Chixin,
et al., Relationship between airborne pollen assemblages and major meteorological parameters in Zhanjiang, South China, PLoS One, 2020, 15, e240160 CrossRef PubMed.
- N. Dolai, I. Karmakar, R. S. Kumar, B. Kar, A. Bala and P. K. Haldar, Free radical scavenging activity of Castanopsis indica in mediating hepatoprotective activity of carbon tetrachloride intoxicated rats, Asian Pac. J. Trop. Biomed., 2012, 2, S243–S251 CrossRef.
- Y. Gao, J. Wang, Y. Fu, J. Yin, J. Shi and Y. Xu, Chemical composition, sensory properties and bioactivities of Castanopsis lamontii buds and mature leaves, Food Chem., 2020, 316, 126370 CrossRef CAS PubMed.
- C. I. G. Tuberoso, E. Bifulco, P. Caboni, F. Cottiglia, P. Cabras and I. Floris, Floral markers of strawberry tree (Arbutus unedo L.) honey, J. Agric. Food Chem., 2010, 58, 384–389 CrossRef CAS PubMed.
- M. G. Lorente, C. D. L. Carretero and R. A. P. Martín, Sensory attributes and antioxidant capacity of Spanish honeys, J. Sens. Stud., 2008, 23, 293–302 CrossRef.
- T. Zhao, L. Zhao, M. Wang, S. Qi, X. Xue, L. Wu and Q. Li, Identification of characteristic markers for monofloral honey of Astragalus membranaceus var. mongholicus Hsiao: A combined untargeted and targeted MS-based study, Food Chem., 2023, 404, 134312 CrossRef CAS PubMed.
-
S. Bogdanov, Harmonised methods of the international honey commission. (https://www.bee-hexagon.net/en/network.htm).
- Codex Alimentarius Commission. Revised Codex standards for honey, Codex standard 12-1981, 2001, p. 2.
- E. Demir, T. Yuksek, V. Atamov and A. E. Ozcelik, A comparison of the physicochemical properties of chestnut and highland honey: The case of Senoz Valley in the Rize province of Turkey, Food Chem., 2020, 345, 128864 CrossRef PubMed.
- M. L. Al, D. Daniel, A. Moise, O. Bobis, L. Laslo and S. Bogdanov, Physico-chemical and bioactive properties of different floral origin honeys from Romania, Food Chem., 2009, 112, 863–867 Search PubMed.
- J. Bouayed, L. Hoffmann and T. Bohn, Total phenolics, flavonoids, anthocyanins and antioxidant activity following simulated gastro-intestinal digestion and dialysis of apple varieties: bioaccessibility and potential uptake, Food Chem., 2011, 128, 14–21 Search PubMed.
- Z. Zhu, Y. Zhang, J. Wang, X. Li, W. Wang and Z. Huang, Sugaring-out assisted liquid-liquid extraction coupled with high performance liquid chromatography-electrochemical detection for the determination of 17 phenolic compounds in honey, J. Chromatogr. A, 2019, 1601, 104–114 Search PubMed.
- R. Scherer and H. T. Godoy, Antioxidant activity index (AAI) by the 2,2-diphenyl-1-picrylhydrazyl method, Food Chem., 2009, 112, 654–658 Search PubMed.
- L. Chen, G. J. T. Tan, X. Pang, W. Yuan, S. Lai and H. Yang, Energy regulated nutritive and antioxidant properties during the germination and sprouting of broccoli sprouts (Brassica oleracea var. italica), J. Agric. Food Chem., 2018, 66, 6975–6985 CrossRef CAS PubMed.
- L. C. Green, D. A. Wagner, J. Glogowski, P. L. Skipper, J. S. Wishnok and S. R. Tannenbaum, Analysis of nitrate, nitrite, and [15N]nitrate in biological fluids, Anal. Biochem., 1982, 126, 131–138 CrossRef CAS PubMed.
- M. F. Silvano, M. S. Varela, M. A. Palacio, S. Ruffinengo and D. K. Yamul, Physicochemical parameters and sensory properties of honeys from Buenos Aires region, Food Chem., 2014, 152, 500–507 Search PubMed.
- I. Mato, J. F. Huidobro, J. Simal-Lozano and M. T. Sancho, Rapid determination of nonaromatic organic acids in honey by capillary zone electrophoresis with direct ultraviolet detection, J. Agric. Food Chem., 2006, 54, 1541–1550 CrossRef CAS PubMed.
- K. Matović, J. Ćirić, V. Kaljević, N. Nedić, G. Jevtić, N. Vasković and M. Ž. Baltić, Physicochemical parameters and microbiological status of honey produced in an urban environment in Serbia, Environ. Sci. Pollut. Res., 2018, 25, 14148–14157 CrossRef PubMed.
- A. Belay, W. K. Solomon, G. Bultossa, N. Adgaba and S. Melaku, Physicochemical properties of the Harenna forest honey, Bale, Ethiopia, Food Chem., 2013, 141, 3386–3392 CrossRef CAS PubMed.
- M. Eyer, P. Neumann and V. Dietemann, A look into the cell: honey storage in honey bees, Apis mellifera, PLoS One, 2016, 11, e161059 Search PubMed.
- V. León-Ruiz, S. Vera, A. V. González-Porto and M. P. S. Andrés, Vitamin C and sugar levels as simple markers for discriminating Spanish honey sources, J. Food Sci., 2011, 76, C356–C361 CrossRef PubMed.
- I. Dobre, L. A. Georgescu, P. Alexe, O. Escuredo and M. C. Seijo, Rheological behavior of different honey types from Romania, Food Res. Int., 2012, 49, 126–132 CrossRef CAS.
- O. Bobis, A. R. Moise and I. Ballesteros,
et al., Eucalyptus honey: Quality parameters, chemical composition and health-promoting properties, Food Chem., 2020, 325, 126870 CrossRef CAS PubMed.
- C. I. G. Tuberoso, I. Jerković, G. Sarais, F. Congiu, Z. Marijanović and P. M. Kuś, Color evaluation of seventeen European unifloral honey types by means of spectrophotometrically determined CIE L* C*abhab chromaticity coordinates, Food Chem., 2014, 145, 284–291 CrossRef CAS PubMed.
- P. M. Kuś, F. Congiu, D. Teper, Z. Sroka, I. Jerković and C. I. G. Tuberoso, Antioxidant activity, color characteristics, total phenol content and general HPLC fingerprints of six Polish unifloral honey types, LWT – Food Sci. Technol., 2014, 55, 124–130 Search PubMed.
- M. Z. Aumeeruddy, Z. Aumeeruddy-Elalfi and H. Neetoo,
et al., Pharmacological activities, chemical profile, and physicochemical properties of raw and commercial honey, Biocatal. Agric. Biotechnol., 2019, 18, 101005 Search PubMed.
- B. Del Rio, B. Redruello, M. Fernandez, M. C. Martin, V. Ladero and M. A. Alvarez, The biogenic amine tryptamine, unlike β-phenylethylamine, shows in vitro cytotoxicity at concentrations that have been found in foods, Food Chem., 2020, 331, 127303 CrossRef CAS PubMed.
- R. S. Pawar and E. Grundel, Overview of regulation of dietary supplements in the USA and issues of adulteration with phenethylamines (PEAs), Drug Test. Anal., 2017, 9, 500–517 Search PubMed.
- X. Zhang, K. Chingin, D. Zhong, L. Luo, V. Frankevich and H. Chen, Deciphering the chemical origin of the semen-like floral scents in three angiosperm plants, Phytochemistry, 2018, 145, 137–145 CrossRef CAS PubMed.
- V. Pereira, M. Pontes, J. S. Câmara and J. C. Marques, Simultaneous analysis of free amino acids and biogenic amines in honey and wine samples using in loop orthophthalaldeyde derivatization procedure, J. Chromatogr. A, 2008, 1189, 435–443 CrossRef CAS PubMed.
- P. Truchado, I. Martos, L. Bortolotti, A. G. Sabatini, F. Ferreres and F. A. Tomas-Barberan, Use of quinoline alkaloids as markers of the floral origin of chestnut honey, J. Agric. Food Chem., 2009, 57, 5680–5686 Search PubMed.
- A. Boussaid, M. Chouaibi, L. Rezig, R. Hellal, F. Donsì, G. Ferrari and S. Hamdi, Physicochemical and bioactive properties of six honey samples from various floral origins from Tunisia, Arabian J. Chem., 2018, 11, 265–274 CrossRef CAS.
- C. Elejalde-Palmett, T. D. de Bernonville and G. Glevarec,
et al., Characterization of a spermidine hydroxycinnamoyltransferase in Malus domestica highlights the evolutionary conservation of trihydroxycinnamoyl spermidines in pollen coat of core Eudicotyledons, J. Exp. Bot., 2015, 66, 7271–7285 CrossRef CAS PubMed.
- M. Talebi, M. Talebi, T. Farkhondeh and S. Samarghandian, Molecular mechanism-based therapeutic properties of honey, Biomed. Pharmacother., 2020, 130, 110590 CrossRef CAS PubMed.
- S. Magiera, I. Baranowska and A. Lautenszleger, UHPLC–UV method for the determination of flavonoids in dietary supplements and for evaluation of their antioxidant activities, J. Pharm. Biomed., 2015, 102, 468–475 Search PubMed.
- J. Rusko, P. Vainovska, B. Vilne and V. Bartkevics, Phenolic profiles of raw mono- and polyfloral honeys from Latvia, J. Food Compos. Anal., 2021, 98, 103813 Search PubMed.
- H. Zhao, M. Zhu and K. Wang,
et al., Identification and quantitation of bioactive components from honeycomb (Nidus Vespae), Food Chem., 2020, 314, 126052 CrossRef CAS PubMed.
- M. Takashima, K. Ichihara and Y. Hirata, Neuroprotective effects of Brazilian green propolis on oxytosis/ferroptosis in mouse hippocampal HT22 cells, Food Chem. Toxicol., 2019, 132, 110669 CrossRef CAS PubMed.
- M. Kassim, M. Achoui, M. R. Mustafa, M. A. Mohd and K. M. Yusoff, Ellagic acid, phenolic acids, and flavonoids in Malaysian honey extracts demonstrate in vitro anti-inflammatory activity, Nutr. Res., 2010, 30, 650–659 Search PubMed.
- S. Dudonné, X. Vitrac, P. Coutière, M. Woillez and J. Mérillon, Comparative study of antioxidant properties and total phenolic content of 30 plant extracts of industrial interest using DPPH, ABTS, FRAP, SOD, and ORAC assays, J. Agric. Food Chem., 2009, 57, 1768–1774 CrossRef PubMed.
- F. C. Biluca, B. Da Silva and T. Caon,
et al., Investigation of phenolic compounds, antioxidant and anti-inflammatory activities in stingless bee honey (Meliponinae), Food Res. Int., 2020, 129, 108756 Search PubMed.
- A. B. Lagha and D. Grenier, Tea polyphenols protect gingival keratinocytes against TNF-α-induced tight junction barrier dysfunction and attenuate the inflammatory response of monocytes/macrophages, Cytokine, 2019, 115, 64–75 Search PubMed.
- S. Afrin, M. Gasparrini and T. Y. Forbes-Hernández,
et al., Protective effects of Manuka honey on LPS-treated RAW 264.7 macrophages. Part 1: Enhancement of cellular viability, regulation of cellular apoptosis and improvement of mitochondrial functionality, Food Chem. Toxicol., 2018, 121, 203–213 CrossRef CAS PubMed.
- N. Kalantari, M. Ghasemi, M. Bayani and S. Ghaffari, Effect of honey on mRNA expression of TNF-α, IL-1β and IL-6 following acute toxoplasmosis in mice, Cytokine, 2016, 88, 85–90 Search PubMed.
- M. Palacz-Wrobel, P. Borkowska, M. Paul-Samojedny, M. Kowalczyk, A. Fila-Danilow, R. Suchanek-Raif and J. Kowalski, Effect of apigenin, kaempferol and resveratrol on the gene expression and protein secretion of tumor necrosis factor alpha (TNF-α) and interleukin-10 (IL-10) in RAW-264.7 macrophages, Biomed. Pharmacother., 2017, 93, 1205–1212 CrossRef CAS PubMed.
- T. Lawrence, The Nuclear Factor NF-κB Pathway in Inflammation, Cold Spring Harbor Perspect. Biol., 2009, 1, a1651 Search PubMed.
- E. Parra-Ortiz, K. L. Browning, L. S. E. Damgaard, R. Nordström, S. Micciulla, S. Bucciarelli and M. Malmsten, Effects of oxidation on the physicochemical properties of polyunsaturated lipid membranes, J. Colloid Interface Sci., 2019, 538, 404–419 CrossRef CAS PubMed.
- M. Battino, F. Giampieri and D. Cianciosi,
et al., The roles of strawberry and honey phytochemicals on human health: A possible clue on the molecular mechanisms involved in the prevention of oxidative stress and inflammation, Phytomedicine, 2021, 86, 153170 Search PubMed.
|
This journal is © The Royal Society of Chemistry 2023 |
Click here to see how this site uses Cookies. View our privacy policy here.