Electrolysed water (hypochlorous acid) generation and efficacy against food-borne pathogens
Received
4th April 2023
, Accepted 18th June 2023
First published on 4th July 2023
Abstract
The present study was conducted to investigate the efficacy of electrochemically generated hypochlorous acid (HOCl) against Salmonella enterica, Pseudomonas aeruginosa and Staphylococcus aureus under clean and soiled conditions. Electrolysed water was produced using a high purity sodium chloride salt solution (90% saturation) and 25–35 A of current supplied to the electrolytic cell and diluted to 165 mg L−1 (P165) and 200 mg L−1 (P200) HOCl. P165 was highly potent against Salmonella enterica and Staphylococcus aureus under clean conditions while P200 showed >99% anti-microbial activity when tested in the presence of 5% foetal bovine serum albumin for a contact time of 10 min. Assuming a fail-threshold of 3/60 as set by the USEPA, the results suggest that HOCl is a good alternative for hard surface disinfection.
Sustainability spotlight
Different chemicals have established their efficacy and suitability for hard surface disinfection, however synthetic disinfectants pose a complex environmental, health and safety risk. There is a dire need to find alternatives that not only have a good efficacy against mutating microbes but also minimise the risk to the environment and the health of people. Hypochlorous acid (HOCl) is one such potent alternative whose neutral charge allows it to be effective at low concentrations without posing any threat to the environment and human health. The on-site generation option for such solution eliminates the use of unnecessary plastic packaging and is in line with the United Nations Sustainable development goals (SDG 3, 6, 7, 9, 11, 13).
|
Introduction
Different forms of chlorine species have been exploited to harness their microbicidal properties. Among them, sodium hypochlorite (NaOCl) and chlorine dioxide (ClO2) have been explored substantially primarily due to their ease of application, excellent disinfection properties and cost-effectivity.1,2 Both NaOCl and ClO2 have been observed to express a broad-spectrum antimicrobial activity and their efficacy is shown to be influenced by temperature, concentration, surface characteristics, and organic load.3,4 Although effective, repeated exposure to high concentrations of NaOCl and ClO2 is often associated with hazards like skin irritation, skin sensitization and cytotoxicity to mammalian cells.5
Over the past decade, hypochlorous acid (HOCl) has garnered tremendous interest for its use as an antimicrobial agent in a variety of applications.6–8 HOCl is a non-irritating, non-toxic form of chlorine that has powerful oxidizing properties and is primarily produced by the neutrophils in the human body through a series of chemical reactions catalyzed by the enzyme myeloperoxidase.9In vitro, HOCl has been demonstrated to have a higher oxidizing power than OCl− primarily due to the ease of penetration of HOCl molecules into the microbial cell.10 When produced via electrolysis of sodium chloride solution, the pH of the solution plays a very critical role in HOCl manufacture. For instance, HOCl can be harnessed to its maximum capacity when the pH of the solution is within the range of 5–6.5, whereas at pH > 6.5 the presence of OCl− in the solution increases and at pH < 5.0 the presence of chlorine gas in the solution increases,10 which subsequently influences the germicidal power of the solution.
Staphylococcus aureus, Pseudomonas aeruginosa and Salmonella enterica are the most common food-borne pathogens that exist within the food chain. It has been documented that these organisms are found to contaminate food items like meat, poultry, dairy, eggs and freshly harvested fruits and vegetables.11 Ingestion of contaminated food sources is responsible for serious illnesses, particularly in children, the elderly, and people with compromised immunity.12 In some cases, Staphylococcus and Pseudomonas spp. have been argued to exist in complex polymicrobial biofilm communities thus, giving rise to the idea of “super bugs” that has raised several important concerns in antimicrobial resistance.13 Hence, most of the disinfectants are tested against these pathogens to review their bactericidal efficacy.14
In recent years, NaOCl and ClO2 are reported to have reduced efficacy against pathogenic micro-organisms. For instance, Almatroudi et al.15 reported that while sodium hypochlorite exposure reduced the plate counts of S. aureus by 7
log10, the organisms regrew and formed biofilms upon prolonged incubation. In addition, Hatanaka et al. reported reduced potency of sodium hypochlorite in the presence of organic matter.16 The incidents of resistance of chlorine-based disinfectants to pathogenic microbes have been surfacing in recent years17,18 and it is critical to evaluate disinfectants with good antimicrobial performance with reduced risk to health and the environment.
The present study was conducted to investigate the antimicrobial effect of HOCl on Staphylococcus aureus, Pseudomonas aeruginosa and Salmonella enterica under clean and dirty conditions at different available chlorine concentrations (ACC) using the AOAC use-dilution method as prescribed by the USEPA. The study is a preliminary approach to establish HOCl as an alternative disinfectant for cleaning and sanitisation of hard surfaces.
Methods
Reagent preparation
High purity sodium chloride (NaCl) salt crystals containing (w/w) NaCl 99.9%, calcium sulfate 0.001%, sodium sulfate 0.04%, insoluble 0.00% and moisture 0.04% were obtained from United Salt Corporation, Texas, USA. RO water (TdS: 3 ppm) at 15.9 °C was used for brine preparation and was produced in-house using the RO system (Culligan G2 series, Indiana, USA).
Analytical substance references potassium iodide, sodium thiosulfate, potassium iodate, concentrated sulfuric acid, and starch were purchased from Sigma Aldrich, IL, USA and were used as received.
Equipment description and operation
Fresh hypochlorous acid (HOCl) was produced by electrochemical activation of a saturated brine solution using an electrochemical solution generator (model: EGS6020, PathoSans, Spraying Systems Co., IL, USA). The system comprises a brine tank (containing the electrolytic cell), a control panel, water softener and collection tanks. The brine tank is subdivided into two compartments with the help of a perforated plastic wall to allow segregation between the cell and the salt crystals. The cell in the system is composed of a dual ion exchange membrane that allows segregation of ions between the two electrodes during electrolysis and prevents the passage of unionised salt in the final solution to maintain purity of the output solution (Fig. 1).
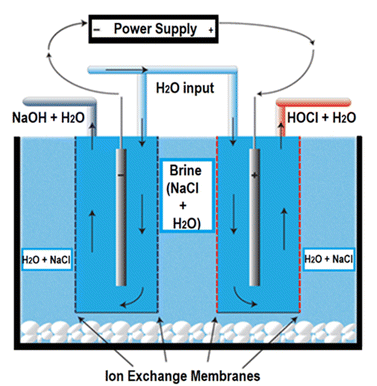 |
| Fig. 1 Schematic representation of the electrochemical cell. | |
A total current of 25–35 A was supplied to the electrolytic cell through power supplies to the cell. Specific combinations were utilized to result in a series of reactions as shown in eqn (1)–(4). In the initial reaction, the applied current splits the deionised water into the following half reactions:
At the anode:
| 2H2O → O2(g) + 4H+(aq) + 4e− | (1) |
At the cathode:
| 2H2O + 2e− → H2(g) + 2OH−(aq) | (2) |
The accumulation of H+ ions in the anode causes an influx of chloride (Cl−) from the saturated brine tank, through the anion exchange membrane, and lowers the pH of the anode chamber. The chloride is oxidised to give chlorine gas which is then rapidly hydrolyzed in the water stream to form HOCl eqn (3) and (4).
| Cl2 + H2O ↔ HOCl + H+ + Cl− | (4) |
The resulting hypochlorous acid was collected at a rate of 2.3 L per min and diluted further using RO water to result in 165 mg L−1 and 200 mg L−1 HOCl solutions. The machine operation was carried out under ambient conditions of 20 ± 2 °C and the solution was analyzed immediately after production to prevent degradation of the active component.
Chemical analysis
pH.
The pH of the disinfectant solution was measured using a pre-calibrated pH meter (HI 98121, Hanna Instruments, USA) at 20 ± 2 °C.
Hypochlorous acid measurement.
The strength of HOCl was measured in terms of the ACC using the enforcement analytical method under the United States Environmental Protection Agency (USEPA) guidelines OPPTS 830.1800. Approximately 10 mL of each 10% potassium iodide (KI) solution and 10% sulphuric acid solution were added to 50 mL of sample and mixed vigorously for 20 s. Subsequently the mixture was titrated against standardized 0.1 N sodium thiosulfate solution till the end point (pale yellow colour) was observed. A few drops of 0.5% starch indicator were then added, and the titration was continued until a clear end point was achieved. The resulting concentration of the active component was determined using eqn (5) |  | (5) |
where A is the volume of the sodium thiosulfate solution used (mL), B is the normality of the sodium thiosulfate solution (N) and C is the weight of the sample used (g).
Immediately after analysis, the samples were transferred to an ISO/IEC 17025:2017 accredited local microbiological laboratory where they were analysed within 24 h of reception.
Data validation
The analytical method used for testing the HOCl concentration in the sample was validated for linearity, precision, and accuracy. Linearity was determined by analyzing 5 concentrations of the standard with concentrations ranging 70–110% of the target analyte concentration by preparing respective dilutions of the sample. The specification for linearity was set at a correlation coefficient (R2) ≥0.98 for the resulting line of best fit. Precision was evaluated by preparing a set of 6 uniform test substance sample replicates and individually evaluating them for the active ingredient concentration. The specification was set at ≤5% relative standard deviation in the data set. Accuracy validation was performed at 3 levels of 80 ± 5%, 100 ± 5%, and 110 ± 5% of the target concentration of the active component and each level was evaluated in triplicate. The specification for accuracy was a target sample recovery of 95–105%.
AOAC use-dilution assay
The experimental design for the use-dilution study followed the general protocol as described by AOAC Official Method 964.02, 955.14, 955.15.19–21 Strains of Salmonella enterica (ATCC 10708), Staphylococcus aureus (ATCC 6538), and Pseudomonas aeruginosa (ATCC 15442) were obtained from American Type Culture Collection, Manassas, Virginia, USA.
Preparation of samples
Briefly, 10 mL aliquots of the HOCl samples were transferred to sterile test tubes placed in a water-bath at 20 ± 1 °C and allowed to equilibrate for 10 min prior to testing.
Preparation of test organisms
A loopful of stock slant culture was transferred to an initial 10 mL tube of growth medium (synthetic broth). After mixing, the tube was incubated for 24 hours at 37 ± 1 °C. Following incubation, without vortex mixing the culture, a 10 μL aliquot of culture was transferred to a 20 × 150 mm Morton Closure tube containing 10 mL of synthetic broth (daily transfer 1). The final test culture was incubated for 48–54 h at 37 ± 1 °C.
For testing under clean conditions, 10 mL of the inoculum was transferred into 20 × 150 mm Morton Closure tubes while to simulate dirty conditions, 5% foetal bovine serum was added to the inoculum, adequately mixed before transfer to the 20 × 150 mm tubes. Each test culture was vortex mixed for 3 to 4 seconds and allowed to stand for more than 10 minutes prior to use. Subsequently, the upper portion of the culture was removed, leaving behind any clumps or debris and was pooled in a sterile vessel and mixed. Care was taken while harvesting P. aeruginosa cultures in order to avoid disrupting the pellicle by not shaking the test cultures. The P. aeruginosa culture was visually inspected to ensure no pellicle fragments were present.
Carrier contamination and inoculation
Carriers were screened according to the AOAC Official Method of Analysis,22 and all carriers positive for growth were discarded. The prepared culture was transferred to the penicylinders (length: 10 mm, ID: 6 mm, OD: 8 mm) after siphoning off the water, and the carriers were immersed for 15 ± 2 min in a prepared suspension at a ratio of one carrier per one ml of culture. The carriers were completely covered by culture. A maximum of 60 carriers were inoculated per vessel and each vessel inoculated was considered a part of one total inoculation run per test organism. The inoculated carriers were transferred to sterile Petri dishes matted with Whatman no. 2 filter paper after tapping the carrier against the side of the container to remove excess inoculum. No more than twelve carriers were placed in the Petri dish. Thereafter, the carriers were dried for 38 minutes at 37 ± 1 °C and at 48.3–54.1% relative humidity. Carriers (inoculated penicylinders) were used in the test procedure within 2 hours of drying. The carrier count procedure was adapted from Tomasino et al.22 to a final bacterial load of not less than 105 cfu mL−1.
Exposure conditions
Each contaminated and dried carrier was placed into a separate tube containing 10.0 mL of the HOCl samples. Immediately after placing each test carrier in the test tube, the tube was swirled using approximately 2–3 gentle rotations to release any air bubbles trapped in or on the carrier. The carriers were exposed for 10 minutes at 20 ± 1 °C. Care was taken to avoid touching the sides of the tubes. The carrier was placed into the test substance within ±5 seconds of the exposure time following a calibrated timer.
Test system recovery, incubation and observation
Following the exposure time, each medicated carrier was transferred to 10 mL of neutralizing subculture medium (Letheen broth + 0.1% sodium thiosulphate) and incubated for 48 ± 2 hours at 37 ± 1 °C. Following incubation and storage, the subcultures were visually examined for the presence or absence of growth. The tubes showing growth were sub-cultured to Tryptic Soy Agar containing 5% Sheep's blood and incubated at 37 ± 1 °C for 24 h. The resultant growth was visually examined, gram stained and biochemically assayed to confirm or rule out the presence of the test organism.
Statistical analysis
All experiments were performed in triplicate. Two-way analysis of variance (ANOVA) was conducted to determine statistical significance among samples by using SPSS 25.0 software (IBM SPSS statistics 25, Australia) using the type of micro-organism and conditions of testing as the factors. A significance level of 0.05 (α = 0.05) was used.
Results and discussion
Data validation for hypochlorous acid (HOCl)
Electrochemically generated HOCl solutions of 165 mg L−1 and 200 mg L−1 were used in the present study. The data generated to quantify the HOCl content in the sample was validated according to Section 2.3 and the results are shown in Table 1 and Fig. 2, respectively. During electrolysis, the dominant form of chlorine species that exists in the solution is determined by its pH10 which subsequently affects the antimicrobial efficacy of the solution.23 For instance, while the majority of chlorine is present as hypochlorite (OCl−) at a pH > 8, studies have shown that more than 95% chlorine can be harnessed as HOCl within pH 5–6.5 at room temperature below which other chlorine species may become dominant in the solution.24 In the present study, HOCl solution was generated electrolytically at a pH of 5.40 ± 0.05 indicating that the concentrations of molecular chlorine and trichloride ions are negligible,10 thus demonstrating that the disinfecting property of the solution is a result of the presence of the HOCl. The strength of HOCl solution was chosen in accordance with the studies that have been conducted on the disinfectants containing available chlorine as the active ingredient.25,26
Table 1 Validation of the analytical enforcement method (parameters: precision and accuracy)
Precision |
Sample |
No. of replicates |
Available chlorine concentration (mg L−1)a |
RSD (%) |
Values are shown as mean ± std dev.; n ≥ 3.
|
1 |
6 |
200 ± 0.00 |
0.00 |
2 |
6 |
165 ± 0.00 |
0.00 |
Accuracy |
Sample variation (%) |
No. of replicates |
Recovery (%)a |
80 ± 5 |
3 |
99.67 ± 0.58 |
100 ± 5 |
3 |
98 ± 0.00 |
110 ± 5 |
3 |
100 ± 0.00 |
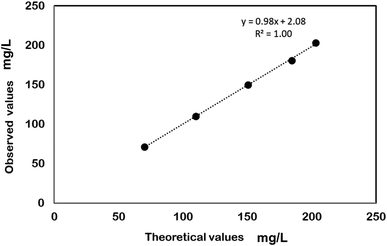 |
| Fig. 2 Validation of the analytical enforcement method for HOCl determination (parameter: linearity). | |
Microbiological results
The purity control and carrier population control results are shown in Tables 2 and 3. The type of micro-organism and the testing conditions influenced the antimicrobial efficacy of HOCl solution at the tested concentration. In the absence of a protein source, no carrier growth was observed for S. enterica, and S. aureus exposed to 165 mg L−1 HOCl solution for 10 min, while growth was observed in 2 carriers out of 60 for P. aeruginosa under the same conditions. In the presence of 5% foetal bovine albumin, none of the micro-organisms except for S. enterica showed growth in the carrier exposed to 200 mg L−1 HOCl for 10 min (Table 4). Different strengths of HOCl solution were chosen to test under the presence and absence of 5% bovine serum albumin. This was because the presence of organic load has been shown to decrease the oxidising power of chlorine-based solutions.25 For instance, Bloomfield et al.27 demonstrated a reduction in the bactericidal effect of 250 ppm chlorine solution from 6 log units to 0.3–1.9 log units after addition of 0.5–1.0% (w/v) albumin while Ayyildiz et al.28 also showed an inverse relationship between water organic load and bacterial inactivation by chlorine dioxide. In addition, Virto et al.29 suggested that the presence of organic load may increase the resistance of bacteria to chlorine disinfection by stabilizing the bacterial membranes while simultaneously reducing chlorine access to cellular components, thus reducing its efficacy. Therefore, it was assumed that a higher concentration of 200 mg L−1 of HOCl solution in the present study would be required as the organic soil load was 5% foetal bovine serum.
Table 2 Purity control and test population control results for the test organisms
Test organism |
Purity control |
Viability |
Neutralizer sterility control |
Organic soil sterility control |
Carrier sterility |
Salmonella enterica (ATCC 10708) |
Pure |
Growth |
No growth |
No growth |
No growth |
Staphylococcus aureus (ATCC 6538) |
Pure |
Growth |
No growth |
No growth |
No growth |
Pseudomonas aeruginosa (ATCC 15442) |
Pure |
Growth |
No growth |
No growth |
No growth |
Table 3 Carrier population control results for test organisms
Test organism |
Carrier set |
Resultsa |
CFU/carrier |
Log10 |
Average log10 |
a, b – values within a column for a specific micro-organism not sharing a common lowercase superscript differ significantly (p < 0.05).
|
Salmonella enterica (ATCC 10708) |
Pre-testing |
7.8 × 105 |
6.65a |
6.62 |
Post-testing |
8.8 × 105 |
6.58a |
Staphylococcus aureus (ATCC 6538) |
Pre-testing |
4.1 × 106 |
5.89b |
5.92 |
Post-testing |
6.4 × 106 |
5.94b |
Pseudomonas aeruginosa (ATCC 15442) |
Pre-testing |
4.5 × 106 |
6.61a |
6.71 |
Post-testing |
3.8 × 106 |
6.81a |
Table 4 AOAC Use dilution method testing for HOCl containing 165 and 200 mg L−1 available chlorine against test organisms Salmonella enterica, Staphylococcus aureus and Pseudomonas aeruginosa under clean and dirty conditions for 10 min at 20 °C
Test organism |
Sample dilution |
No. of carriersab |
Exposed |
Showing growthc |
Confirmed as the test organism |
P165 |
P200 |
P165 |
P200 |
a, b – values within a column for a specific micro-organism not sharing a common lowercase superscript differ significantly (p < 0.05).
A, B – values within a row under a specified column not sharing a common uppercase superscript differ significantly (p < 0.05).
Number of carriers showing growth of the test organism.
|
Salmonella enterica (ATCC 10708) |
Ready-to-use |
60 |
0aA |
1bB |
0aA |
1bB |
Staphylococcus aureus (ATCC 6538) |
Ready-to-use |
60 |
0aA |
0bA |
0aA |
0aA |
Pseudomonas aeruginosa (ATCC 15442) |
Ready-to-use |
60 |
2bB |
0bA |
2bB |
0aA |
On comparing the results with those of Bloomfield et al.,27 it was found that 5% foetal bovine serum did not significantly impact the germicidal action of 200 mg L−1 HOCl solution against the tested micro-organisms. In addition, it was seen that the inhibitory effect of 5% foetal bovine serum on the efficacy of HOCl at 200 mg L−1 was much lower than that reported by Pappen et al.30 on 300 mg L−1 sodium hypochlorite suggesting the influence of pH on the microbicidal activity of the chlorine species. Teng et al.31 studied the chlorine depletion profile at pH 3.0, 5.0 and 6.5 and reported that the depletion rate increased significantly at low pH in the presence of organic load. This finding agrees with the observations reported by Takehara32 where degradation of 100 ppm chlorine solution in the presence of 0.3% (w/v) bovine serum albumin was the least at pH 4.5 while the degradation was maximum at pH 9.5 indicating that HOCl is the least affected chlorine species in the presence of a protein molecule.3
HOCl solution showed 100% antimicrobial activity against S. aureus regardless of the presence or absence of organic load. Generally, Gram positive bacteria like S. aureus have been reported to offer higher resistance to antimicrobial agents in comparison to Gram negative bacteria like S. enterica because of the difference in their cell wall structure and composition33 and this has also been confirmed by Tango et al.34 and Issa-Zacharia et al.35 for their studies on the effect of slightly acidic electrolysed water on inactivation of different gram-staining organisms. However, the difference in observation in the present study from the literature could be due to the concentration of the solution tested. It has been argued that the mechanism of action of HOCl is not like any other anti-microbial agent. HOCl has been shown to target the membrane structures of microbial cells directly as opposed to NaOCl and ClO2 that causes microbial inactivation by damaging the intracellular protein and enzyme structures.36 In addition, HOCl causes a higher rate of peroxidation of lipid present in the bacterial cell membrane, thus giving it increased access to the microbial structures as compared to the other chlorine counterparts. It is hypothesized that the electrolytical process induces changes in the structure of the water molecule which causes it to transform into simple monomeric structures that can readily pass through the bacterial cell resulting in faster deactivation, possibly explaining the least resistance to inactivation shown by S. aureus.
Although some growth was observed under the tested conditions, given that the USEPA fail threshold is 3/60, HOCl solution in the present study qualifies as a hard surface disinfectant at both the concentrations investigated.
Conclusion
HOCl at 165 mg L−1 and 200 mg L−1 concentrations is effective as a hard surface disinfectant when left in contact with hard surfaces for 10 min in the absence and presence of 5% foetal serum albumin, respectively. While S. aureus was expected to have a higher resistance than S. enterica and P. aeruginosa due to difference in their microbial structures, no such difference in susceptibility was observed, suggesting that HOCl has a different mechanism of disinfection as compared to other antimicrobial agents. This work is a preliminary approach to suggest the use of HOCl as an alternative disinfectant that has a relatively high anti-microbial efficacy than other chlorine-based sanitisers even in the presence of organic load. HOCl can be used for a variety of antimicrobial applications with the added advantage of being non-toxic to the handling personnel, thus eliminating the need for personal protective equipment.
Conflicts of interest
The authors declare that they have no known competing financial interests or personal relationships that could have appeared to influence the work reported in this paper.
Acknowledgements
The authors acknowledge the funding provided by Spraying Systems Co., IL, USA to conduct the present study.
References
- R. Van Houdt and C. W. Michiels, Biofilm formation and the food industry, a focus on the bacterial outer surface, J. Appl. Microbiol., 2010, 109(4), 1117–1131 CrossRef CAS PubMed.
- J. N. Tan, C. A. Hwang, L. Huang, V. C. Wu and H. I. Hsiao, A p ilot-scale evaluation of using gaseous chlorine dioxide for decontamination of foodborne pathogens on produce and l ow-moisture foods, J. Food Saf., 2023, 43(2), e12937 CrossRef CAS.
- S. Fukuzaki, Mechanisms of actions of sodium hypochlorite in cleaning and disinfection processes, Biocontrol Sci., 2006, 11(4), 147–157 CrossRef CAS PubMed.
- K. H. Byun, S. H. Han, J. W. Yoon, S. H. Park and S. D. Ha, Efficacy of chlorine-based disinfectants (sodium hypochlorite and chlorine dioxide) on Salmonella Enteritidis planktonic cells, biofilms on food contact surfaces and chicken skin, Food Control, 2021, 123, 107838 CrossRef CAS.
- F. K. Cobankara, H. B. Ozkan and A. Terlemez, Comparison of organic tissue dissolution capacities of sodium hypochlorite and chlorine dioxide, J. Endod., 2010, 36(2), 272–274 CrossRef PubMed.
- B. Zhang, L. K. Ma, S. G. Deng, C. Xie and X. H. Qiu, Shelf-life of pacific white shrimp (Litopenaeus vannamei) as affected by weakly acidic electrolyzed water ice-glazing and modified atmosphere packaging, Food Control, 2015, 51, 114–121 CrossRef CAS.
- H. L. Degala, J. R. Scott, F. I. Rico Espinoza, A. K. Mahapatra and G. Kannan, Synergistic effect of ozonated and electrolyzed water on the inactivation kinetics of Escherichia coli on goat meat, J. Food Saf., 2020, 40(1), e12740 CrossRef.
- P. Li, Z. Chen, M. Tan, J. Mei and J. Xie, Evaluation of weakly acidic electrolyzed water and modified atmosphere packaging on the shelf life and quality of farmed puffer fish (Takifugu obscurus) during cold storage, J. Food Saf., 2020, 40(3), e12773 CAS.
- A. L. Severing, J. D. Rembe, V. Koester and E. K. Stuermer, Safety and efficacy profiles of different commercial sodium hypochlorite/hypochlorous acid solutions (NaClO/HClO): antimicrobial efficacy, cytotoxic impact and physicochemical parameters in vitro, J. Antimicrob. Chemother., 2019, 74(2), 365–372 CrossRef CAS PubMed.
-
G. C. White, White's Handbook of Chlorination and Alternative Disinfectants, Wiley, 2010 Search PubMed.
- C. B. Penha, E. Bonin, A. F. da Silva, N. Hioka, É. B. Zanqueta, T. U. Nakamura, B. A. de Abreu Filho, P. A. Campanerut-Sá and J. M. Mikcha, Photodynamic inactivation of foodborne and food spoilage bacteria by curcumin, LWT–Food Sci. Technol., 2017, 76, 198–202 CrossRef CAS.
-
T. A. Taylor and C. G. Unakal, Staphylococcus aureus, StatPearls, 2021 Search PubMed.
- Z. Xu, J. Xie, T. Soteyome, B. M. Peters, M. E. Shirtliff, J. Liu and J. M. Harro, Polymicrobial interaction and biofilms between Staphylococcus aureus and Pseudomonas aeruginosa: an underestimated concern in food safety, Curr. Opin. Food Sci., 2019, 26, 57–64 CrossRef.
- W. A. Rutala, E. C. Cole, C. A. Thomann and D. J. Weber, Stability and bactericidal activity of chlorine solutions, Infect. Control Hosp. Epidemiol., 1998, 19(5), 323–327 CrossRef CAS PubMed.
- A. Almatroudi, I. B. Gosbell, H. Hu, S. O. Jensen, B. A. Espedido, S. Tahir, T. O. Glasbey, P. Legge, G. Whiteley, A. Deva and K. Vickery, Staphylococcus aureus dry-surface biofilms are not killed by sodium hypochlorite: implications for infection control, J. Hosp. Infect., 2016, 93(3), 263–270 CrossRef CAS PubMed.
- N. Hatanaka, B. Xu, M. Yasugi, H. Morino, H. Tagishi, T. Miura, T. Shibata and S. Yamasaki, Chlorine dioxide is a more potent antiviral agent against SARS-CoV-2 than sodium hypochlorite, J. Hosp. Infect., 2021, 118, 20–26 CrossRef CAS PubMed.
- S. Speck, C. Wenke, A. T. Feßler, J. Kacza, F. Geber, A. D. Scholtzek, D. Hanke, I. Eichhorn, S. Schwarz, M. Rosolowski and U. Truyen, Borderline resistance to oxacillin in Staphylococcus aureus after treatment with sub-lethal sodium hypochlorite concentrations, Heliyon, 2020, 6(6), e04070 CrossRef PubMed.
- H. Yu, Y. Liu, F. Yang, Y. Xie, Y. Guo, Y. Cheng and W. Yao, Synergistic efficacy of high-intensity ultrasound and chlorine dioxide combination for Staphylococcus aureus biofilm control, Food Control, 2021, 122, 107822 CrossRef CAS.
-
AOAC, Official Method of Analysis of AOAC Intl. Method 964.02. Association of Official Analytical Chemists, Arlington, VA, USA, 2013 Search PubMed.
-
AOAC, Official Method of Analysis of AOAC Intl. Method 955.15. Association of Official Analytical Chemists, Arlington, VA, USA, 2013 Search PubMed.
-
AOAC, Official Method of Analysis of AOAC Intl. Method 955.14, Association of Official Analytical Chemists, Arlington, VA, USA, 2013 Search PubMed.
- S. F. Tomasino, R. M. Fiumara and M. P. Cottrill, Enumeration procedure for monitoring test microbe populations on inoculated carriers in AOAC use-dilution methods, J. AOAC Int., 2006, 89(6), 1629–1634 CrossRef CAS PubMed.
- S. V. Len, Y. C. Hung, M. Erickson and C. Kim, Ultraviolet spectrophotometric characterization and bactericidal properties of electrolyzed oxidizing water as influenced by amperage and pH, J. Food Prot., 2000, 63(11), 1534–1537 CrossRef CAS PubMed.
- M. Yaqub, C. Woo and W. Lee, Optimization of hypochlorous acid generation by HCl electrolysis through response surface methodology and artificial neural networks, J. Environ. Chem. Eng., 2021, 9(5), 105826 CrossRef CAS.
- M. Ishihara, K. Murakami, K. Fukuda, S. Nakamura, M. Kuwabara, H. Hattori, M. Fujita, T. Kiyosawa and H. Yokoe, Stability of weakly acidic hypochlorous acid solution with microbicidal activity, Biocontrol Sci., 2017, 22(4), 223–227 CrossRef CAS PubMed.
- M. Kuwabara, Y. Sato, M. Ishihara, T. Takayama, S. Nakamura, K. Fukuda, K. Murakami, H. Yokoe and T. Kiyosawa, Healing of Pseudomonas aeruginosa-infected wounds in diabetic db/db mice by weakly acidic hypochlorous acid cleansing and silver nanoparticle/chitin-nanofiber sheet covering, Wound Medicine, 2020, 28, 100183 CrossRef.
- S. F. Bloomfield, M. Arthur, E. Looney, K. Begun and H. Patel, Comparative testing of disinfectant and antiseptic products using proposed European suspension testing methods, Lett. Appl. Microbiol., 1991, 13(5), 233–237 CrossRef CAS.
- O. Ayyildiz, B. Ileri and S. Sanik, Impacts of water organic load on chlorine dioxide disinfection efficacy, J. Hazard. Mater., 2009, 168(2–3), 1092–1097 CrossRef CAS PubMed.
- R. Virto, P. Manas, I. Alvarez, S. Condon and J. Raso, Membrane damage and microbial inactivation by chlorine in the absence and presence of a chlorine-demanding substrate, Appl. Environ. Microbiol., 2005, 71(9), 5022–5028 CrossRef CAS PubMed.
- F. G. Pappen, W. Qian, J. Aleksejūnienė, R. de Toledo Leonardo, M. R. Leonardo and M. Haapasalo, Inhibition of sodium hypochlorite antimicrobial activity in the presence of bovine serum albumin, J. Endod., 2010, 36(2), 268–271 CrossRef PubMed.
- Z. Teng, Y. Luo, S. Alborzi, B. Zhou, L. Chen, J. Zhang, B. Zhang, P. Millner and Q. Wang, Investigation on chlorine-based sanitization under stabilized conditions in the presence of organic load, Int. J. Food Microbiol., 2018, 266, 150–157 CrossRef CAS PubMed.
- A. Takehara, Relationship between the pH and decrease in available chlorine of sodium hypochlorite in the presence of organic compounds or during freezing treatment, Rep. Ind. Technol. Cent. Okayama Prefect., 2006, 32, 10–13 Search PubMed.
- P. Foladori, B. Laura, A. Gianni and Z. Giuliano, Effects of sonication on bacteria viability in wastewater
treatment plants evaluated by flow cytometry—fecal indicators, wastewater and activated sludge, Water Res., 2007, 41(1), 235–243 CrossRef CAS PubMed.
- C. N. Tango, A. R. Mansur and D. H. Oh, Fumaric acid and slightly acidic electrolyzed water inactivate gram positive and gram negative foodborne pathogens, Microorganisms, 2015, 3(1), 34–46 CrossRef CAS PubMed.
- A. Issa-Zacharia, Y. Kamitani, K. Morita and K. Iwasaki, Sanitization potency of slightly acidic electrolyzed water against pure cultures of Escherichia coli and Staphylococcus aureus, in comparison with that of other food sanitizers, Food Control, 2010, 21(5), 740–745 CrossRef CAS.
- Z. G. Ersoy, O. Dinc, B. Cinar, S. T. Gedik and A. Dimoglo, Comparative evaluation of disinfection mechanism of sodium hypochlorite, chlorine dioxide and electroactivated water on Enterococcus faecalis, LWT, 2019, 102, 205–213 CrossRef CAS.
|
This journal is © The Royal Society of Chemistry 2023 |
Click here to see how this site uses Cookies. View our privacy policy here.