In situ construction of a magnesium foliar fertilizer with pH-controlled release and high adhesion capacity†
Received
9th September 2022
, Accepted 22nd November 2022
First published on 1st December 2022
Abstract
To solve the worldwide problems caused by magnesium deficiency in modern agriculture, a pompon-like magnesium foliar fertilizer (PMFF) was developed via an ammonia-assisted sacrificial nano silica template method. The ultra-thin curled nanosheets are distributed radially on the shell of the PMFF with an obvious hollow structure, which endows the PMFF with high adhesion capacity on the surface of plant leaves without being washed away by rainwater. Moreover, the release of nutrient element magnesium from the PMFF could be sensitively controlled by changing the pH of the solution. Unexpectedly, compared to the traditional magnesium foliar fertilizer (TMFF), the unique structure of the PMFF makes it have a good sustained-release ability, and its magnesium release during 48 h is 88.0 mg g−1. Benefiting from the rough morphology of the PMFF, the foliar adhesion efficiency, rainfastness capacity, and the fertilization utilization efficiency of magnesium were promoted by 10.4, 2.2, and 9.0 times in comparison with those of the TMFF (MgSO4 and Mg(NO3)2), respectively. This work provides a novel strategy to solve the problem of magnesium deficiency in crops worldwide and offers new opportunities and approaches for the application of nanotechnology in agriculture.
Environmental significance
As a ‘forgotten element’ in global crop production, magnesium deficiency significantly affects the crop yield and nutritional quality. The misuse of traditional magnesium foliar fertilizers (TMFFs) has resulted in serious economic losses and terrible environmental pollution. Here, we developed a nano pompon-like magnesium foliar fertilizer (PMFF) via a method of directly in situ constructing the nutrient element magnesium on a nano silica template. The foliar adhesion efficiency, rainfastness capacity and the fertilization utilization efficiency of magnesium were promoted by 10.4, 2.2 and 9.0 times in comparison with those of the TMFF. The novel PMFF and its design concept provide a new inspiration for solving the agricultural problems caused by magnesium deficiency in the world and offers a reference path for realizing the sustainable development of nano-agriculture.
|
Introduction
According to the report from the Food and Agriculture Organization of the United Nations (FAO), the global population is predicted to reach 10 billion by 2050, and thus the food production is required to increase by 60–70% to adapt to this population explosion.1,2 In the past few decades, huge amounts of macro-element fertilizers (nitrogen (N),3,4 phosphorus (P),5,6 and potassium (K)7) have been used to improve the quality and yield of grain around the world.8 Despite the fact that massive inputs of macro-element fertilizers have increased the total output,9 the medium and trace elements in the soil have been continuously consumed, limiting crop yield and nutritional quality improvement.10–14 As one of the most important elements for plant growth in addition to N, P, and K, magnesium (Mg) is not only an important component of polyribosomes, but also promotes the formation of vitamins in crops.15 Therefore, the application of Mg fertilizers can enhance the photosynthesis of crops, increase the yield and improve the quality of agricultural products.16–19 Mg is actually an essential nutrient element for plant growth that is a ‘forgotten element’ in crop production worldwide.20,21 With the increase in the multiple cropping index of crops and the cultivation of new varieties, although crop yields and biomass continue to increase, the amount of Mg extracted by crops from the soil is also increasing, simultaneously.13,22–25 More seriously, farmers do not pay enough attention to the medium and trace fertilizers including Mg, leading to ineffective supplementation of Mg in the soil.20 Therefore, the phenomenon of crop Mg deficiency has gradually appeared in different regions and different crops, which not only significantly affects the increase in crop production and nutritional quality, but also is not conducive to the sustainable development of modern agriculture.13,24
The reported average value of yield loss owing to severe magnesium deficiency in soil was 9.4%.25 In order to solve the problems caused by Mg deficiency in crops, two effective strategies have been proposed: applying Mg fertilizers to the roots or leaves of crops.25–27 In the process of root fertilization, the excessive content of the other cations (especially excessive K ions caused by K fertilizer abuse21,28) in the soil will inhibit the absorption of Mg ions by the crops, resulting in low absorption efficiency of Mg by the roots, and ultimately causing poor utilization of Mg fertilizers and soil contamination.25 Recent studies have proved that crop leaves can effectively absorb Mg fertilizers and avoid the antagonistic effects between the Mg ions and other cations in the soil.26 Traditionally, the application of the TMFF in the market is to simply spray water-soluble magnesium salts (e.g., magnesium sulfate (MgSO4)27,29 or magnesium nitrate (Mg(NO3)2)30 by mixing with water) on the foliage of crops. In the process of spraying the foliar fertilizer, due to the inherent lotus effect of crop leaves,31 the commercial TMFF on the foliar surface easily slips during the spraying process, which ultimately leads to poor utilization of the Mg fertilizer. To guarantee the effectiveness of the TMFF, farmers have to spray a great deal of Mg fertilizers with high concentrations.32 Moreover, the commercial foliar Mg fertilizers are generally instantaneous release fertilizers, in which the Mg is almost completely released within a few minutes and easily causes Mg poisoning of crop leaves. Besides that, foliar fertilization is significantly affected by the soil environment. Generally, spraying foliar fertilizers does not work in windy weather and during rainy days.33 These unsatisfactory weather conditions severely restricted spraying fertilizers on crop leaves in time, causing serious economic losses.34,35 In brief, TMFFs have many shortcomings: low fertilization utilization efficiency (FUE), no controlled-release effect of nutrients, and ultra-low foliar adhesion, leading to serious fertilizer waste and fearful soil pollution.33,36
With the success of nanotechnology in the fields of pharmaceuticals and health sciences,37,38 the application of nanotechnology in the agricultural revolution has also been attempted.1,39,40 The excellent performance of engineered nanomaterials (ENMs) in agricultural practices provides the most promising new opportunities and approaches for a novel agricultural revolution to sustainably meet future food demands.41,42 Several published reports suggest that foliar application of Mg-based nanomaterials is far more efficient than TMFFs. For example, Kanjana43 confirmed that foliar spraying of MgO nanoparticles improved fibre quality parameters such as fibre length and fibre strength in cotton compared to foliar spraying of the sulfate form of Mg fertilizers. Khordadi Varamin et al.44 reported that foliar fertilization of nano-magnesium chelates increased the seed yield and oil content of sesame. Wang et al.45 reported that foliar applications of 35 nm MgO nanoparticles can effectively supplement Mg for watermelon. They also mentioned that foliar application of MgO nanoparticles by foliar spraying may have advantages over soil irrigation since the potential ecological risks (e.g., soil contamination by metals) are eliminated by avoiding direct soil interaction. However, these newly reported foliar magnesium-based nano-fertilizers are prone to slipping from the foliage due to the action of wind and rain causing fertilizer waste and soil contamination. Therefore, it is urgent to develop a new type of Mg foliar fertilizer, which could be applied under unfavorable weather conditions and have good controlled-release ability and high foliar adhesion efficiency (FAE) to solve the above-mentioned problems.
Nanosilica as an environmentally friendly carrier has been confirmed to be used on the surface of plant leaves without causing changes in plant photosynthesis and respiration.46 In this study, a pompon-like Mg foliar fertilizer (PMFF) was developed via an ammonia-assisted sacrificial nano silica template method, which directly in situ constructed nutrient element magnesium on a nano silica template (Scheme 1a). The unique surface roughness structure makes the PMFF have a super-high foliar adhesion ability and high rainfastness property (Scheme 1c). Thanks to its unique hollow structure, the PMFF has a slow-release ability of Mg. In vitro experiments demonstrate that the PMFF can automatically adapt to changes in acidity caused by evaporation of fertilizer droplets on the leaf surface to effectively release Mg. The pH value of normal rainwater is 5.6, which is conducive to the release of Mg from the PMFF. It is noteworthy that the releasing rate of Mg from the PMFF could be modulated by adjusting the pH of solution to meet the demand for the Mg fertilizer in different growth cycles of crops (Scheme 1b). Furthermore, our experiment systematically compared the adhesion and rain resistance of two TMFFs (MgSO4 and Mg(NO3)2) and the PMFF on the leaf surface. The contact angle experiment and microscopic morphology analysis explained the reason for the super-high adhesion and excellent resistance of the PMFF to rain erosion. Finally, a pot experiment was conducted to compare the growth status of the three foliar Mg fertilizers on the growth of Mg-deficient tomato seedlings, and the Mg utilization efficiency of the PMFF and traditional Mg fertilizers was further evaluated.
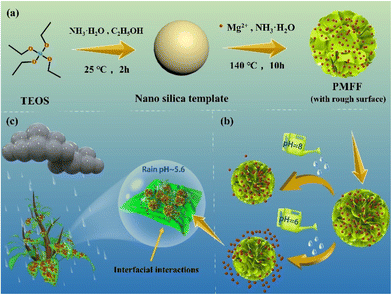 |
| Scheme 1 (a) Schematic illustration for the synthesis of the PMFF via a facile hydrothermal method using nano silica as a sacrificial template. (b) Schematic diagram of magnesium release from the PMFF in different acid–base environments on the leaf surface. (c) Schematic representation of the PMFF with ultra-high adhesion capacity and high rainfastness property on leaves. | |
Experimental section
Preparation of the PMFF
The template nano silica was fabricated according to the well-known Stöber method.47 Briefly, TEOS (4.2 mL) was promptly added to the mixed solution of NH3·H2O (24 mL) and C2H5OH (81 mL) under magnetic stirring at 25 °C for 2 h. Subsequently, the white product (nano silica with a particle diameter of about 500 nm) was collected and washed with deionized water and anhydrous ethanol several times. Subsequently, 0.08 g nano silica was ultrasonically dispersed in 20 mL of deionized water to form solution A, while MgCl2·6H2O (0.20 g), NH4Cl (0.54 g) and 1 mL NH3·H2O were dissolved in 30 mL of deionized water in sequence, and then mixed uniformly under magnetic stirring to form solution B. Subsequently, solution A and solution B were transferred into an autoclave and heated at 140 °C for 10 h. The white product was collected by centrifugation and washed with anhydrous ethanol and deionized water at least three times. Finally, the white product was dried in a vacuum oven at 60 °C overnight and was denoted as the PMFF.
Adhesion performance
At room temperature, 1 mL of MgSO4 solution (1%), Mg(NO3)2 solution (1%), PMFF solution (1.5 g L−1) and deionized water were sonicated (500 W, 40 kHz) for 5 min, respectively, and then evenly sprayed on the leaves of tomato at an angle of 30° to the horizontal, respectively. After the droplets on tomato leaves were naturally air-dried, 5 mL of deionized water was sprayed on the tomato leaf surface to simulate the effect of rain rinsing. After the droplets dried on the leaf surface naturally, once again, the simulated rainwater rinsing was performed under the same conditions. After natural air drying, the leaf was submersed in 20 mL of deionized water under shaking in a constant temperature shaker for 6 h at 250 rpm to fully separate the Mg fertilizer from the leaf surface into the deionized water. Finally, the concentration of Mg in solution was measured using an inductively coupled plasma-atomic emission spectrometer (ICP-AES, ICP-7000, Thermo Fisher Scientific, USA).
The foliar adhesion efficiency (FAE) of the magnesium foliar fertilizer was calculated according to the equation:
|  | (1) |
where
Nadhesion is the total Mg content adhered on the leaf surface (3 cm
2);
Nspraying is the total magnesium content in the sprayed Mg foliar fertilizer (1 mL).
The rainfastness capacity (RC) of the Mg foliar fertilizer was calculated using the following formula:
| 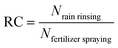 | (2) |
where
Nrain rinsing is the Mg content on the leaf surface after two-times simulated rain rinsing;
Nfertilizer spraying is the total Mg content of the first-time spray of the PMFF on the leaves.
Controlled-release behavior of magnesium
The PMFF (0.3 g) was immersed in 200 mL of deionized water under different pH conditions, in which 0.1 M HCl and NaOH were used to adjust the pH value of the solution to 5–8. Then, 5 mL of the resulting suspension was taken out for filtration at different times to measure the Mg concentration.
Pot experiments
Tomato seeds with full and uniform particle size were sterilized using 2% hydrogen peroxide solution for 30 min, and then washed with deionized water, repeatedly. Next, the tomato seeds were soaked in deionized water for 6 h, and then transferred to a Petri dish (diameter of 12 cm) covered with sterile moist filter paper (1 cm or larger distance was kept away from each seed). After germination and growth for 5 days under dark conditions at 26 °C, uniform seedlings were transplanted into a black light-proof incubation box (length 12.7 cm, width 8.7 cm, height 11.4 cm). A total of four experimental groups were designed in the pot experiments, with three pots in each group and six tomato plants in each pot. After transplanting, 1 L of Hoagland nutrient solution containing major nutrients (15 mM NH4NO3, 5 mM KH2PO4, 5 mM K2SO4, 10 mM Ca(NO3)2, and 10 mM MgSO4) and micronutrients (8 μM Na2MoO4, 8 μM ZnSO4, 90 μM MnSO4, 3 μM CuSO4, 0.75 M EDTA–Fe and 0.46 M H3BO3) were replaced to each pot every five days. And 0.1 M HCl and NaOH were used to adjust the pH value of the nutrient solution to about 6.5. All the plants after transplanting were cultured in a plant growth chamber (CONVIRON ADAPTIS-A1000, Canada) at a temperature of 26 °C/21 °C (14 h/10 h), a light–dark cycle of 14 h/10 h, a relative humidity of 60%, and a light intensity of 300 mol photons m−2 s−1.
On the 25th day after transplanting, the tomato seedlings were treated with Mg deficient Hoagland nutrient solution without MgSO4. By the 36th day, the tomato seedlings have shown more obvious symptoms of Mg deficiency. At this time, 10 mL PMFF (1.5 g L−1), MgSO4 solution (1%), Mg(NO3)2 solution (1%), and deionized water were sprayed on the four groups of tomato leaves, respectively. After that, 50 mL deionized water was sprayed onto the leaves of the four groups of tomato seedlings every five days (spray two-times) to simulate rainwater rinsing. The chlorophyll content, plant height, and stem diameter of the tomato seedlings in each group were measured every day. Tomato seedlings were harvested on the 50th day, and then washed with deionized water 3 times, and the root length were measured. The shoots and roots were separated, and their fresh weights were measured, respectively. The growth status of tomato seedlings at various stages was recorded using a digital camera. Subsequently, the tomato seedlings were vacuum-dried at a temperature of 60 °C for 36 h until they were thoroughly dried, and then their dry weight was measured. The dried roots, stems and leaves of tomato seedlings (each about 0.25 g) were digested with 6 mL concentrated HNO3 (65% w/w) and 2 mL H2O2 (30% w/w) overnight. After that, 1 mL of HF was added to the sample and the reaction was kept in a microwave oven (Mars 6, CEM, Matthews, NC, USA) at a power of 1000 W and 180 °C for 3 h. Whereafter, the above digestion solution was concentrated to less than 1 mL (160 °C). Finally, the digest was diluted to 10 mL with deionized water and the magnesium content of dried roots, stems, and leaves was measured by ICP-AES.
The fertilization utilization efficiency (FUE) of the Mg foliar fertilizer was calculated according to the following equations:
| 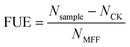 | (3) |
where
Nsample is the total Mg content in tomato seedlings after applying the Mg foliar fertilizer;
NCK is the total Mg content of tomato seedlings after applying deionized water;
NMFF is the total Mg content in the Mg foliar fertilizer.
Biosafety evaluation
At 25 °C, red zebrafish (n = 8) were kept in a fish tank (150 mm × 110 mm × 130 mm) containing 1 L of PMFF solution with different concentrations (0, 0.2, 0.4, 0.6, 0.8, and 1.0 g L−1). The same solution was replaced every 3 days during the feeding process of each fish tank. Digital photos recorded the number of alive fish and calculated the viability of the red zebrafish after 14 days. All animal procedures were performed in accordance with the Guidelines for Care and Use of Laboratory Animals of Hefei Institutes of Physical Science and approved by the Animal Ethics Committee of Hefei Institutes of Physical Science (Approval ID: SWYX-DW-2022-51).
Results and discussion
Synthesis and characterization of the PMFF
Firstly, a nano silica template with uniform particle size and good dispersibility was synthesized according to the classic Stöber method.46 The surface morphology and particle size distribution of nano silica templates are shown in Fig. S1,† in which the synthesized template has a smooth surface morphology and the size distribution is in the range of 450–550 nm. Subsequently, the PMFF was successfully prepared in a one-pot reaction via an ammonia-assisted sacrificial nano silica template method. Under the heating conditions of 140 °C, the nano silica template is dissolved by hydroxide ions in an alkaline solution with suitable concentration to generate silicate ions. The silicate ions react with the Mg ions in the solution, and gradually deposit on the surface of the nano silica template to form a magnesium silicate shell, and finally generate the hollow PMFF. It is worth mentioning that in the hydrothermal reaction, Mg ions easily form magnesium hydroxide precipitates in ammonia solution. The addition of ammonium chloride can adjust the form of Mg ions and the rate of hydroxide release in the solution. In this way, the release rate of silicate ions is further controlled, so that they can react with enough surrounding Mg ions to form a magnesium silicate shell. As shown in Fig. 1a, the crystal structure of magnesium silicate is a talc structure composed of layered substances, and each layer is a T–O–T symmetrical structure composed of a silicon–oxygen tetrahedron (T) and a magnesium–oxygen octahedron (O). The Mg ions in the talc structure are easily replaced by the hydrogen ions in the acidic solution, resulting in an ion exchange reaction. And this special reaction also endows the PMFF with predictable pH sensitivity. Furthermore, the XRD patterns of the nano silica template and PMFF are presented in Fig. 1b. It showed that the diffraction peaks of the PMFF at 19.5°, 28.8°, 36.2°, 53.2°, 60.9°, 71.4°, and 78.1° were well-consistent with the (020), (
15), (
33), (
41), (
32), and (335) planes (JCPDS card no. 03-0174, Mg3Si4O13H4), which are completely different from the diffraction peaks of the nano silica template (JCPDS card no. 01-0438). This demonstrated that the Mg nutrient elements are successfully in situ loaded and grown on the nano silica template. The low crystallinity of the PMFF can be inferred from the broadened XRD peaks, which also signifies that the Mg in the PMFF is easily replaced by hydrogen ions. In order to further illustrate the final state of the PMFF after the complete release of magnesium, we set an extreme condition experiment to explore the release content of Mg from the PMFF at pH 0, and the PMFF after the release of Mg under these conditions was named PMFF-D. The XRD (Fig. 1c) pattern of PMFF-D was exactly the same as that of the nano silica template, indicating that the PMFF could eventually become nano silica after complete Mg release. Therefore, the release behavior of the PMFF in an acidic environment could be described by the following chemical equation: | Mg3Si4O13H4 + 6H+ → 3Mg2+ + 4SiO2 + 5H2O | (4) |
Besides, we determined that the magnesium content entirely released by the PMFF in the solution of pH 0 is 155.5 mg g−1. To clearly understand the surface structure of the PMFF, nitrogen adsorption and desorption isotherms of the PMFF and nano silica template are shown in Fig. 1d. Conspicuously, the isotherm behavior of the PMFF could be classified as a type-IV isotherm with a H3 type weak hysteresis loop in the IUPAC classification. The specific surface area (SSA) of the PMFF was measured to be 336.8 m2 g−1 from a multipoint Brunauer–Emmett–Teller (BET) plot and the pore size of the PMFF was mainly distributed in the range from 2 to 2.4 nm. In fact, such a high specific SSA of the PMFF could be caused by the special rough morphology and hollow structure of the PMFF. Furthermore, we found that the SSA of the nano silica template is only 4.1 m2 g−1, which is much smaller than that of the PMFF. This is attributed to a large number of ultra-thin fold-like curled nanosheet units and the hollow structure being formed in the PMFF during the synthesis of the Mg fertilizer.
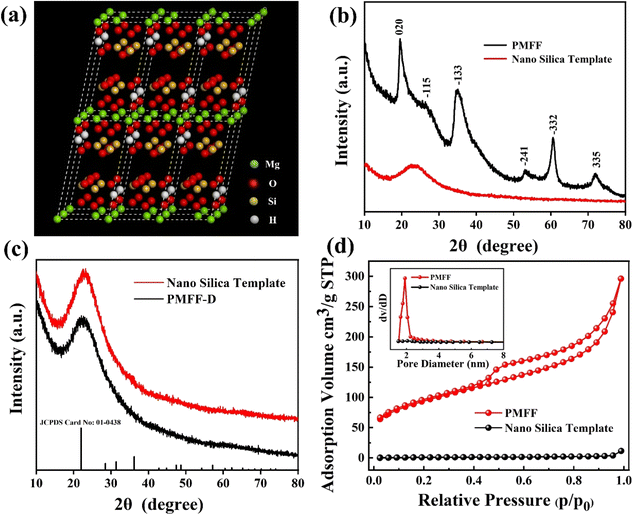 |
| Fig. 1 (a) Three-dimensional images of the magnesium silicate layered structure. (b) XRD patterns of the PMFF and nano silica template. (c) XRD patterns of the nano silica template and PMFF-D. (d) N2 adsorption–desorption isotherms and pore size distribution of the PMFF and nano silica template. | |
To further understand the microstructure, the morphology of the PMFF was investigated by scanning electron microscopy (SEM) and transmission electron microscopy (TEM). As shown in Fig. 2a, the PMFF has excellent monodispersity and the average particle diameter of the PMFF is about 800 nm. The morphology of the PMFF is a pompon mum-like shape with a highly rough surface. Densely packed ultra-thin fold-like curled nanosheet units are distributed across the PMFF surface, in which the thickness of the nanosheet is only 15 nm. The unique structure is attributed to the talc structure of the synthesized PMFF, the talc structure consists of layered substances, which are bound by van der Waals forces. In the process of combining Mg ions with silicate ions to form magnesium silicate, it preferentially grows along the layered direction, and finally forms an ultra-thin fold-like curled nanosheet structure. Fig. 2b shows that the ultra-thin curled nanosheets are distributed radially on the shell of the PMFF with an obvious hollow structure. Specifically, an obvious shell layer with a thickness of 160 nm is shown in the inset of Fig. 2b. Thanks to this rough structure, the PMFF can be efficiently adhered onto the tomato leaf. To further determine the elemental composition of the PMFF, the elemental mapping images of the samples from SEM are shown in Fig. 2c–e. The results revealed the homogeneous distribution of Mg, O, and Si elements throughout the whole structure of the PMFF. The abundant Mg elements in the shell indicated that the Mg nutrient elements are in situ constructed on the nano silica template.
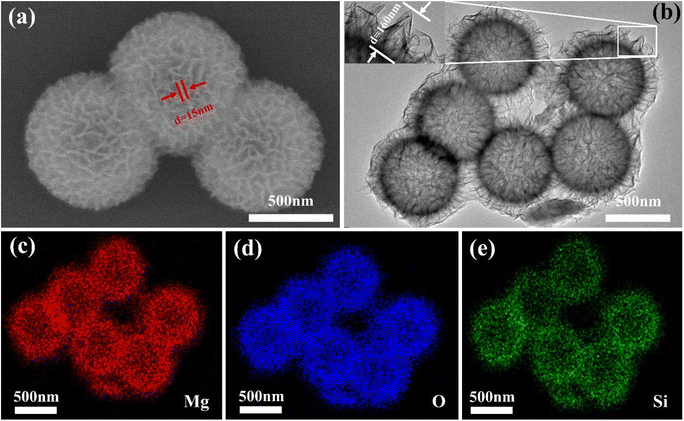 |
| Fig. 2 (a) SEM image and (b) TEM image of the PMFF (the inset is the high magnification of the fold-like nanosheets on the surface of the PMFF). Corresponding elemental mapping of (c) Mg, (d) O, and (e) Si elements in the PMFF, respectively. | |
Controlled-release behavior of magnesium in the PMFF
To assess the release behavior of Mg in the PMFF, the release amount of fertilizer at different times was explored in deionized water. As shown in Fig. 3a, in contrast to the instantaneous release of nutrients in the TMFF, Mg in the PMFF was a continuous release process with obvious slow-release characteristics. The amount of magnesium released from the PMFF in 5 minutes was only 55.2 mg g−1, but its cumulative release over 30 days was 112 mg g−1, accounting for 72% of the total magnesium load. This is mainly due to the different release rates of Mg from the inner and outer shell layers of the PMFF with a hollow structure. Moreover, the release behavior of Mg in aqueous solutions with different pH values from 5.0 to 8.0 is presented in Fig. 3b. The release amount of Mg gradually increased with decreasing pH value. As the pH value decreased, the hydrogen ions in the solution gradually increased, and the Mg from the PMFF was replaced by the hydrogen ions accordingly. It is a remarkable fact that the release amount of Mg at pH 8.0 is only 61.1 mg g−1, which is lower than the release amount of 92.8 mg g−1 at pH 5.0. This result revealed that the pH of the PMFF solution can be adjusted to control the release of Mg, which makes it possible to regulate the demand for Mg in different growth periods of crops. More interestingly, the SEM image of PMFF-D in Fig. S2† shows that PMFF-D still maintains the same rough surface as the PMFF. This indicated that during the application of the PMFF, the sustained release of Mg will not change the surface morphology of the PMFF, that is, it will not reduce the foliar adhesion capacity of the PMFF. Furthermore, the XRD patterns of the nano silica template and PMFF-D were exactly the same, indicating that the PMFF will eventually become environmentally friendly nano silica after complete Mg release, and the carrier materials will not bring the risk of environmental pollution. Furthermore, it is significant to understand the release behavior of the PMFF on the foliar surface with changes in the environment. In particular, the volume of PMFF droplets that remain on the foliage of crops will gradually decrease with water evaporation, which will cause a pH change in droplets and ultimately affect the release of Mg. To this end, the in vitro experiments simulate the pH changes of droplets on the foliar surface with evaporation in the natural environment. The solution with an initial pH of 6 was naturally evaporated at 30 °C to verify the change in pH as the water evaporates. As shown in Fig. S3,† under the premise of an initial pH of 6, the decrease in solution volume due to water evaporation reduces the pH of the solution. This interesting experimental result also suggested that the pH-sensitive PMFF could effectively release magnesium during the evaporation of foliar PMFF droplets.
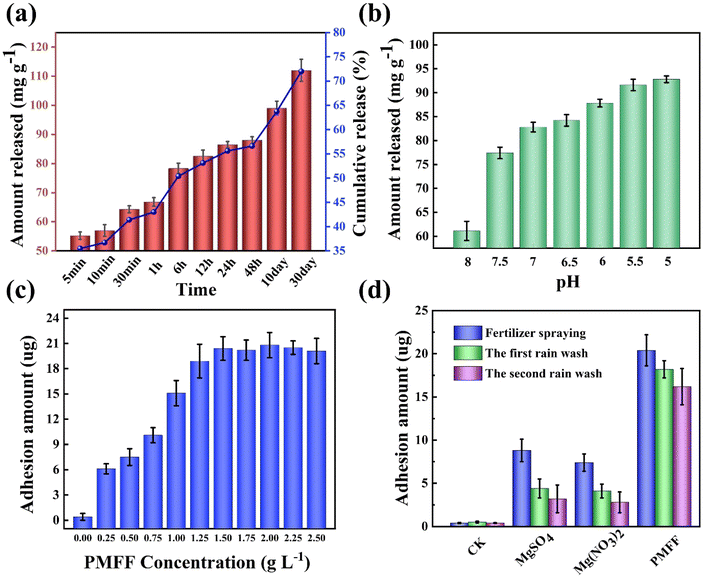 |
| Fig. 3 Controlled-release behavior of Mg in the PMFF at (a) different times and (b) different pH values. (c) Adhesion performance of the PMFF on the tomato leaf surface with different PMFF concentrations. (d) Adhesion performance of deionized water, MgSO4 solution, Mg(NO3)2 solution, and PMFF solution on the tomato leaf surface after the fertilizer spraying and two-times simulated rain rinsing. | |
Adhesion capacity of the magnesium foliar fertilizer
The most appropriate concentration of PMFF on the tomato foliar surface was determined by the Mg content adhered on the tomato leaf surface, as shown in Fig. 3c. With the increase of PMFF concentration, the adhesion amount of Mg firstly increased, and then reached balance gradually. When the concentration of PMFF was 1.5 g L−1, the adhesion amount of Mg on the foliar surface achieved the maximum value of 6.8 μg cm−2. When the concentration of PMFF was lower than 1.5 g L−1, it has been found that the PMFF can be effectively dispersed on the surface of tomato leaves during the spraying process. When the concentration of PMFF was higher than 1.5 g L−1, however, the PMFF will agglomerate, causing some of the agglomerates to slip off from the crop leaves, resulting in fertilizer loss. Therefore, the optimized spray concentration of PMFF should be 1.5 g L−1.
In the practical application of foliar fertilizers, the duration of the fertilizing effect is largely influenced by weather conditions, especially the rinsing of the rain on the foliar fertilizer. Therefore, we further compared the foliar adhesion efficiency and rainfastness capacity of two kinds of TMFFs and the PMFF. In Fig. 3d, during the first-time spraying of the foliar Mg fertilizers, the foliar adhesion of the PMFF is much higher than that of the TMFFs (MgSO4 and Mg(NO3)2), and its FAE was 9.1 and 10.4 times higher than that of the TMFFs, respectively. After two-times simulated rain rinsing, the residual amount of PMFF on the leaf surface is 5.1 and 5.8 times higher than that of MgSO4 and Mg(NO3)2, respectively, and the rainfastness capacity (RC) is 2.2 and 2.1 times, respectively. The rough surface allows the PMFF to be firmly stuck in the fold structure of the tomato leaf surface, resulting in effective resistance to rain erosion. However, the TMFFs tended to slip off from the surface of the tomato leaves due to the inherent lotus effect of the crop leaves. The excellent foliar adhesion ability of the PMFF could provide a feasible way to solve the problem of poor foliar adhesion of TMFFs.
Wettability performance of MgSO4, Mg(NO3)2 and PMFF on leaf surfaces
In order to further clarify the adhesion of the foliar Mg fertilizers on tomato leaves, the contact angles of the PMFF, Mg(NO3)2, MgSO4, and deionized water with different concentrations on tomato leaves were investigated. Fig. 4 reveals the average contact angle of the four solutions on the tomato leaf surface. The contact angle of deionized water on the tomato leaf surface was about 120°, indicating that the tomato leaf surface has a hydrophobic structure. When the solution concentration was 1.5 g L−1, the average contact angle of the PMFF on the tomato leaves was much lower than that of deionized water, Mg(NO3)2 and MgSO4 solutions, revealing the best wettability of the PMFF on the surface of crop leaves. It greatly reduced the slip rate of the Mg foliar fertilizer on the surface of leaves. Moreover, the contact angle for different concentrations of MgSO4 and Mg(NO3)2 on the tomato leaf surface did not change significantly (the range of change was 112.1–120.1° and 119.2–126.8°, respectively). However, as the concentration of the solution increased, the contact angle value of the PMFF on the surface of the tomato leaves decreased significantly. This result also supported that when the concentration of the solution was less than 1.5 g L−1, the adhered amount of Mg on the tomato leaf surface increased with increasing concentration of PMFF solution.
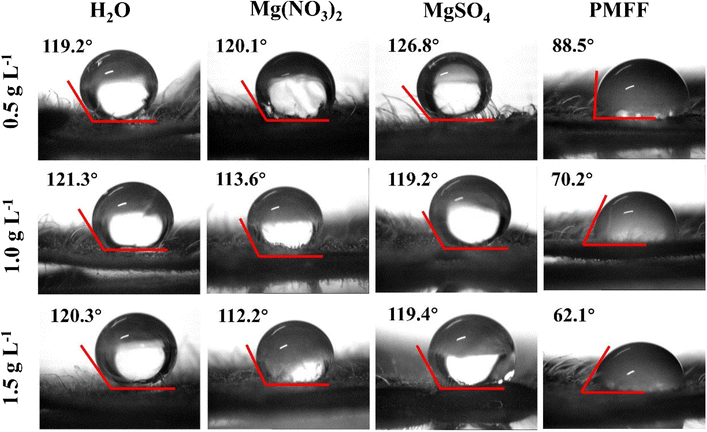 |
| Fig. 4 Contact angles of deionized water, MgSO4 solution, Mg(NO3)2 solution, and PMFF solution with different concentrations on the surface of leaves. | |
Microscopic investigation of PMFF adhesion on the leaf surface
To further understand the attachment state of the PMFF on the surface of tomato leaves, the SEM images of the PMFF on the tomato leaves are shown in Fig. 5. By exploring the microscopic morphology of the tomato leaves, it was clearly found that there is a mass of stomata on the surface of the leaves (marked by white dotted circles in Fig. 5a). Due to unevenly distributed cuticle, epidermal cells, and stomata guard cells on the tomato leaf surface, many irregular fold structures form on the tomato leaves. This rough structure could provide feasible conditions for the efficient attachment of the PMFF. Fig. 5b shows the adhesion of the PMFF on the surface of tomato leaves after two-times simulated rainwater rinsing. It is observed that a huge amount of PMFF was attached on the leaf surface of tomato, revealing the superhigh foliar adhesion ability of the PMFF. Since the shell layer on the PMFF surface has many ultra-thin curled nanosheets, this unique shape allows the PMFF to be firmly embedded between the folds of tomato leaves. Therefore, the PMFF can very effectively attach to the rough surface of tomato leaves and resist rain rinsing without slipping off. Fig. 5c is the corresponding EDS mapping of Mg, Si, and O elements for the adhered PMFF on the tomato leaf surface after two-times simulated rainwater rinsing. This indicated that the PMFF still holds some of the Mg content after two-times simulated rainwater rinsing. This phenomenon also demonstrated that the adhesion behavior of the PMFF on the leaf surface can not only resist rain rinsing, but also have the ability to continuously release Mg.
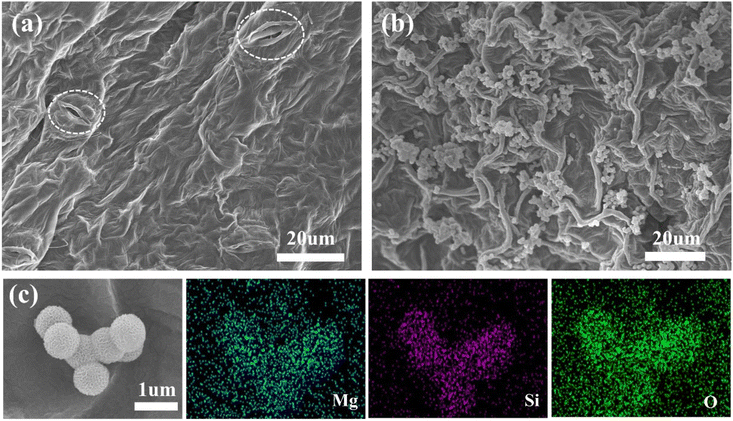 |
| Fig. 5 (a) SEM image of the tomato leaf surface. (b) SEM image of the tomato leaf surface with PMFF after two-times simulated rainwater rinsing. (c) Corresponding elemental mapping of Mg, Si, and O elements for PMFF adhered on the tomato leaf surface. | |
Pot experiments
Mg is the center atom of the chlorophyll molecule and is placed in the middle of the porphyrin ring of the chlorophyll molecular structure. Plants deficient in magnesium will be unable to manufacture chlorophyll normally, resulting in a considerable decrease in chlorophyll content.48 Mg not only promotes the active participation of nutrients in photosynthesis, but also helps phosphate metabolism and plant respiration. In addition, Mg can improve the drought, cold, and disease resistance of crops, and promote the growth and development of plants.18,48 When plants lacked Mg, the older leaves at the bottom will be affected firstly. The color of the tip and edge of the leaf will change from green to light yellow, and gradually extend to the leaf vein.49 In severe cases, the leaves will wilt and fall off. In order to explore the fertilizer effect of the PMFF, pot experiments were further performed to evaluate the effects of the different Mg foliar fertilizers on the growth of tomato seedlings. Fig. 6a shows the influence of the chlorophyll in tomato seedlings by spraying different Mg foliar fertilizers. When the tomato seedlings grew to the 25th day (stage I), all tomato seedlings were treated with Mg deficient Hoagland nutrient solution, resulting in the decrease in the chlorophyll content of the leaves due to the Mg deficiency of the tomato seedlings. When the tomato seedlings grew to the 34th day, different Mg foliar fertilizers were sprayed on different groups of tomato seedlings (stage II). In the following 5 days, the chlorophyll content of the tomato seedlings sprayed with deionized water drops sharply, owing to no timely Mg supplementation. However, spraying the PMFF, MgSO4, and Mg(NO3)2 onto the tomato seedlings prevents the chlorophyll content from further decreasing due to Mg supplementation. Among them, the chlorophyll recovery effect of the PMFF was the best, which is attributed to its better foliar adhesion ability than the other two traditional foliar Mg fertilizers. When the tomato seedlings were grown to the 40th day (stage III) and the 45th day (stage IV), all tomato seedlings were washed by two-times simulated rainwater rinsing. With increasing the plant growth period, the chlorophyll content of tomato seedlings sprayed with deionized water still keeps dropping continuously. Most of the traditional Mg fertilizers slip off from the leaves because of simulated rainwater rinsing, resulting in poor fertilizer effects of MgSO4 and Mg(NO3)2, and gradually reducing the chlorophyll content of tomato seedlings. More seriously, tomato seedlings sprayed with the two kinds of TMFFs have a more obvious chlorophyll decline rate after the second-time rainwater rinsing than after the first-time rainwater rinsing. Dramatically, the chlorophyll content of tomato seedlings sprayed with the PMFF is still continuously recovered even after two-times rainwater rinsing. Finally, the chlorophyll content of tomato seedlings sprayed with the PMFF gradually returns to the normal level before Mg deficiency. This is mainly because the PMFF attached on the tomato leaves can effectively resist rain erosion and sustain the release of Mg in the process. After spraying different foliar Mg fertilizers, the plant height (Fig. 6b) and stem diameter (Fig. 6c) of the tomato seedlings were recorded daily. Compared with the control CK (sprayed with deionized water), MgSO4, Mg(NO3)2, and PMFF could all promote the growth of plant height and stem diameter of magnesium-deficient tomato seedlings to a certain extent. Nevertheless, the plant height and stem diameter of tomato seedlings applied with the PMFF are the highest, indicating that the PMFF has the most obvious effect of Mg supplementation on the growth of tomato seedlings. After the tomato seedlings were harvested, the root length (Fig. 6d), dry weight (Fig. 6e), and fresh weight (Fig. S4†) of the tomato seedlings were measured. Obviously, compared with spraying deionized water, MgSO4 and Mg(NO3)2, the PMFF is more effective in promoting the growth of tomato seedling roots. Similarly, the roots and stems of tomato seedlings sprayed with the PMFF have the highest dry weight and fresh weight, suggesting that the PMFF promotes the accumulation of tomato seedling biomass. To better understand the accumulation of magnesium in crops, the content of magnesium in different parts of the crop is shown in Fig. 6f. The Mg content of tomato seedlings sprayed with the PMFF is 0.14%, 0.096%, and 0.614% in the dry weight of the roots, stems and leaves of the plants, respectively. The Mg fertilization utilization efficiency (FUE) of tomato seedlings sprayed with the PMFF is 7.7 and 9.0 times that of the TMFFs, respectively.
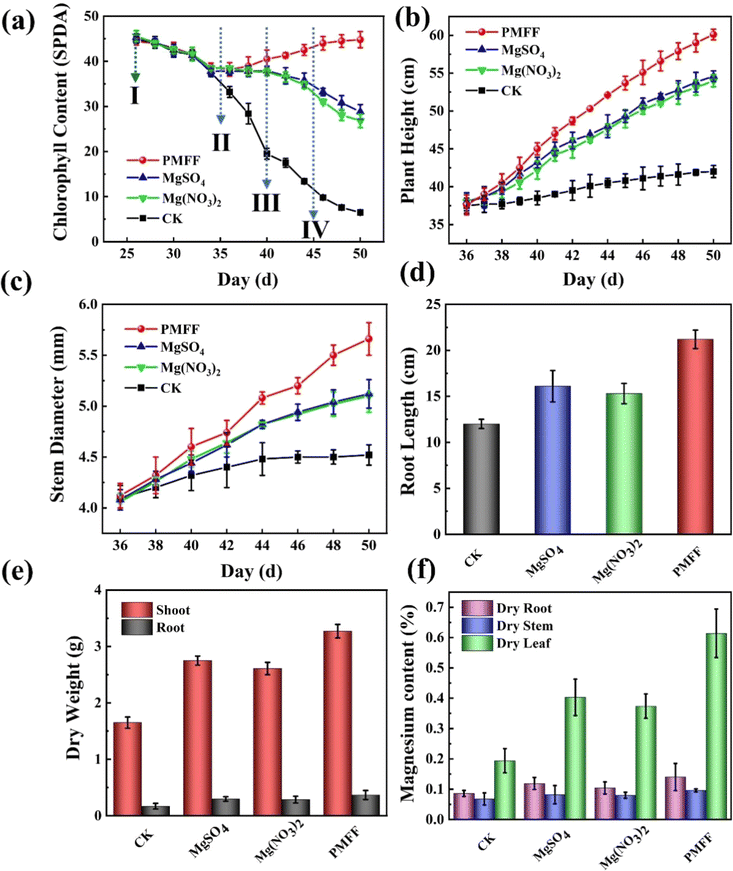 |
| Fig. 6 (a) Chlorophyll content (I–IV represent the different processing stages of tomato seedlings). (b) Plant height, (c) stem diameter, (d) root length, (e) dry weight, and (f) Mg content of tomato seedlings sprayed with deionized water, MgSO4, Mg(NO3)2, and PMFF solutions. | |
To investigate the effects of different Mg foliar fertilizers on the growth of magnesium-deficient tomato seedlings, digital photographs recorded the growth status of tomato seedlings in different periods. Fig. 7a presents the symptoms of Mg deficiency in tomato seedlings. The bottom individual mature leaves in the four groups of tomato seedlings with the same growth period showed that the leaf veins turn yellow and exhibit chlorosis on the edge, which are the typical symptoms of Mg deficiency. Fig. 7b shows the tomato seedlings after spraying with different foliar Mg fertilizers. Since there is no timely Mg supplementation, most of the bottom mature leaves of the tomato seedlings sprayed with deionized water exhibit serious chlorosis and even some of the leaves have withered, showing very serious symptoms of Mg deficiency. For tomato seedlings sprayed with Mg(NO3)2 and MgSO4, some bottom mature leaves veins turn yellow. However, the symptoms of Mg deficiency are only milder than those of tomato seedlings sprayed with deionized water. The symptoms of Mg deficiency in tomato seedlings sprayed with the PMFF have been a remarkable improvement, and some leaves gradually returned to green. This indicated that spraying with the PMFF could effectively remedy the symptoms of Mg deficiency in tomatoes. Fig. 7c shows the digital photograph of tomato seedlings after two-times simulated rainwater rinsing. The bottom leaves of tomato seedlings in the control group all withered and their growth stagnated. Since most of the Mg(NO3)2 and MgSO4 were washed off by simulated rainwater rinsing, tomato seedlings sprayed with Mg(NO3)2 and MgSO4 still have severe Mg deficiency symptoms, in which large areas of bottom mature leaves exhibited chlorosis, and even some leaves withered. However, tomato seedlings sprayed with the PMFF showed a remarkable improvement for Mg deficiency symptoms. This directly confirmed that the PMFF can effectively resist rain erosion and continuously release Mg on the tomato leaves. The digital photographs of tomato seedlings after harvesting are shown in Fig. 7d. Compared with tomato seedlings sprayed with the TMFFs, tomato seedlings sprayed with the PMFF have longer roots, taller plants and more vigorous growth. This indicates that the PMFF has the most obvious effect on the growth of tomato seedlings.
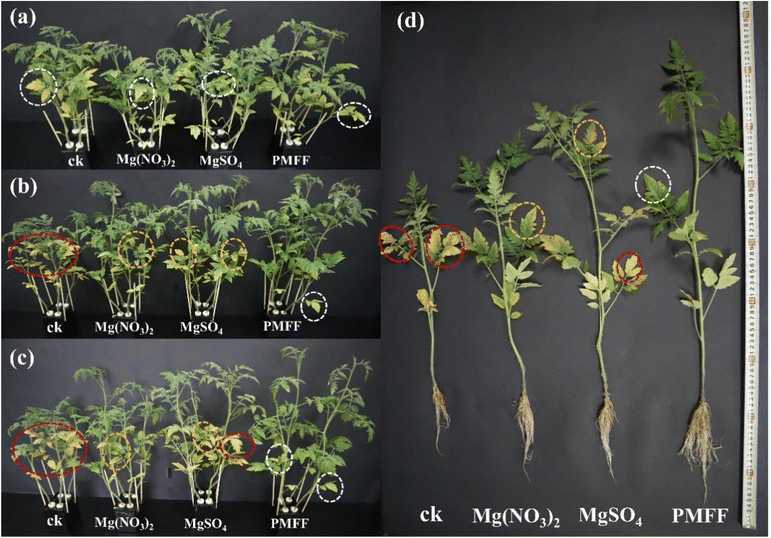 |
| Fig. 7 Digital photographs of tomato seedlings of different growth periods ((a) 35 days, (b) 40 days, (c) 50 days, and (d) harvest) sprayed with deionized water, MgSO4 solution, Mg(NO3)2 solution, and PMFF solution. Different colored circles represent different degrees of Mg deficiency (red circles: severe symptoms; yellow circles: medium symptoms; white circles: mild symptoms). | |
Biosafety evaluation
As a new type of ENM, although the PMFF is constructed based on environmentally friendly nano-silica, it is worth exploring the biosafety of the PMFF. Zebrafish are 87% genetically homologous to humans, making them an excellent model organism, and have been used in a wide variety of studies as a target for biotoxicity research.50–56 In this study, we evaluated the biological safety of the PMFF by studying the survival rate of red zebrafish in PMFF solutions with different concentrations (0, 0.2, 0.4, 0.6, 0.8, and 1.0 g L−1). The survival status of red zebrafish in different solutions is shown in Fig. 8. Comparing the survival status of zebrafish for 0 days and 14 days, it could be found that the survival rate of red zebrafish is 100% in different concentrations of PMFF solution (Fig. S5†). This indicated that the PMFF has no significant toxicity to red zebrafish, which has high biological safety.
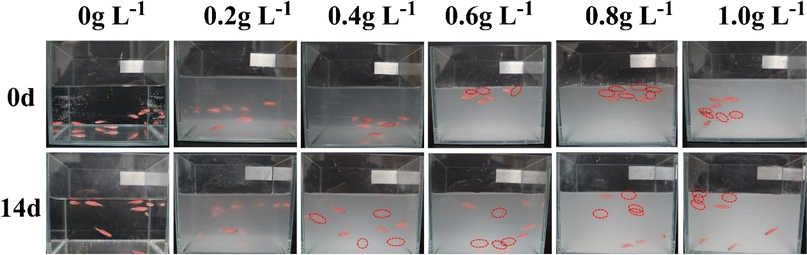 |
| Fig. 8 Digital photographs of red zebrafish in water with different concentrations of PMFF. The red circle represents the fuzzy position of the red zebrafish. | |
Conclusions
In summary, a pompon-like Mg foliar fertilizer (PMFF) was developed via an ammonia-assisted sacrificial nano silica template method, which directly in situ constructed magnesium on a nano silica template to release the nutrient element Mg. The unique hydrotalcite crystal structure endows the PMFF with pH sensitivity and the release amounts of Mg could be modulated by adjusting the pH of solution to meet the demand for the PMFF in different growth cycles of crops. The PMFF has a particle size of 800 nm and a distinct hollow structure. 15 nm ultra-thin convoluted nanosheets are radially distributed on a 160 nm thick shell of the PMFF. Thanks to the unique rough surface structure and peculiar hollow structure, the PMFF possesses ultra-high adhesion capacity and sustained-release ability (sustained Mg release of 112 mg g−1 over 30 days), which could be an ideal choice to solve the problems of ultra-low adhesion, poor fertilizer efficiency, serious soil pollution and Mg poisoning of seedlings for TMFFs in the market. Compared with MgSO4 and Mg(NO3)2, the PMFF can effectively increase the FAE, RC and FUE by 10.4, 2.2, and 9.9 times at most, respectively. The novel PMFF and its design concept provide a new inspiration for solving the agricultural problems caused by magnesium deficiency in the world and offer a reference path for realizing the sustainable development of nano-agriculture.
Conflicts of interest
There are no conflicts to declare.
Acknowledgements
This work was financially supported by the National Natural Science Foundation of China (Grant No. 51772298 and 51872291), the Research Fund of State Key Laboratory of Soil and Sustainable Agriculture, Nanjing Institute of Soil Science, Chinese Academy of Science (Grant No. Y812000007), and the Presidential Foundation of Hefei Institutes of Physical Science, Chinese Academy of Sciences (No. YZJJZX202019).
References
- G. V. Lowry, A. Avellan and L. M. Gilbertson, Opportunities and challenges for nanotechnology in the agri-tech revolution, Nat. Nanotechnol., 2019, 14(6), 517–522 CrossRef CAS.
- M. Kah, N. Tufenkji and J. C. White, Nano-enabled strategies to enhance crop nutrition and protection, Nat. Nanotechnol., 2019, 14(6), 532–540 CrossRef CAS PubMed.
- J. K. Ladha, H. Pathak, T. J. Krupnik, J. Six and C. van Kessel, Efficiency of fertilizer nitrogen in cereal production: Retrospects and prospects, Adv. Agron., 2005, 87, 85–156 CAS.
- L. Liu, X. Y. Zhang, W. Xu, X. J. Liu, Y. Li, J. Wei, M. Gao, J. Bi, X. H. Lu, Z. Wang and X. D. Wu, Challenges for Global Sustainable Nitrogen Management in Agricultural Systems, J. Agric. Food Chem., 2020, 68(11), 3354–3361 CrossRef CAS PubMed.
- D. L. Lopez-Arredondo, M. A. Leyva-Gonzalez, S. I. Gonzalez-Morales, J. Lopez-Bucio and L. Herrera-Estrella, Phosphate Nutrition: Improving Low-Phosphate Tolerance in Crops, Annu. Rev. Plant Biol., 2014, 65, 95–123 CrossRef CAS.
- D. Cordell, J. O. Drangert and S. White, The story of phosphorus: Global food security and food for thought, Glob. Environ. Change, 2009, 19(2), 292–305 CrossRef.
- C. Zorb, M. Senbayram and E. Peiter, Potassium in agriculture - Status and perspectives, J. Plant Physiol., 2014, 171(9), 656–669 CrossRef.
- G. S. Khush, Green revolution: preparing for the 21st century, Genome, 1999, 42(4), 646–655 CrossRef CAS PubMed.
- W. M. Stewart, D. W. Dibb, A. E. Johnston and T. J. Smyth, The contribution of commercial fertilizer nutrients to food production, Agron. J., 2005, 97(1), 1–6 CrossRef.
- D. L. Jones, P. Cross, P. J. A. Withers, T. H. DeLuca, D. A. Robinson, R. S. Quilliam, I. M. Harris, D. R. Chadwick and G. Edwards-Jones, REVIEW: Nutrient stripping: the global disparity between food security and soil nutrient stocks, J. Appl. Ecol., 2013, 50(4), 851–862 CrossRef.
- D. D. Miller and R. M. Welch, Food system strategies for preventing micronutrient malnutrition, Food Policy, 2013, 42, 115–128 CrossRef.
- A. Rashid and J. Ryan, Micronutrient constraints to crop production in soils with Mediterranean-type characteristics: A review, J. Plant Nutr., 2004, 27(6), 959–975 CrossRef CAS.
- W. L. Guo, H. Nazim, Z. S. Liang and D. F. Yang, Magnesium deficiency in plants: An urgent problem, Crop J., 2016, 4(2), 83–91 CrossRef.
- M. S. Fan, F. J. Zhao, S. J. Fairweather-Tait, P. R. Poulton, S. J. Dunham and S. P. McGrath, Evidence of decreasing mineral density in wheat grain over the last 160 years, J. Trace Elem. Med. Biol., 2008, 22(4), 315–324 CrossRef CAS.
- N. Farhat, A. Elkhouni, W. Zorrig, A. Smaoui, C. Abdelly and M. Rabhi, Effects of magnesium deficiency on photosynthesis and carbohydrate partitioning, Acta Physiol. Plant., 2016, 38(6), 145 CrossRef.
- I. Cakmak, Magnesium in crop production, food quality and human health, Plant Soil, 2013, 368(1), 1–4 CrossRef CAS.
- J. Gerendas and H. Fuhrs, The significance of magnesium for crop quality, Plant Soil, 2013, 368(1), 101–128 CrossRef CAS.
- I. Cakmak and P. J. White, Magnesium in crop production and food quality, Plant Soil, 2020, 457(1), 1–3 CrossRef CAS.
- J. Li, Q. H. Li, X. Y. Zhang, L. Y. Zhang, P. L. Zhao, T. Wen, J. Q. Zhang, W. L. Xu, F. Guo, H. Zhao, Y. Wang, P. Wang, D. J. Ni and M. L. Wang, Exploring the Effects of Magnesium Deficiency on the Quality Constituents of Hydroponic-Cultivated Tea (Camellia sinensis L.) Leaves, J. Agric. Food Chem., 2021, 69(47), 14278–14286 CrossRef CAS PubMed.
- I. Cakmak and A. M. Yazici, Magnesium: A Forgotten Element in Crop Production, Better Crops Plant Food, 2010, 94, 23–25 Search PubMed.
- A. Gransee and H. Fuhrs, Magnesium mobility in soils as a challenge for soil and plant analysis, magnesium fertilization and root uptake under adverse growth conditions, Plant Soil, 2013, 368(1), 5–21 CrossRef CAS.
- B. P. Meena, A. K. Biswas, M. Singh, R. S. Chaudhary, A. B. Singh, H. Das and A. K. Patra, Long-term sustaining crop productivity and soil health in maize-chickpea system through integrated nutrient management practices in Vertisols of central India, Bull. - Commonw. Bur. Pastures Field Crops, 2019, 232, 62–76 CrossRef.
- G. L. Miner, J. A. Delgado, J. A. Ippolito and C. E. Stewart, Soil health management practices and crop productivity, Agric. Environ. Lett., 2020, 5(1), e20023 CAS.
- A. Rosanoff, Changing crop magnesium concentrations: impact on human health, Plant Soil, 2013, 368(1), 139–153 CrossRef CAS.
- Z. Wang, M. Ul Hassan, F. Nadeem, L. Q. Wu, F. S. Zhang and X. X. Li, Magnesium Fertilization Improves Crop Yield in Most Production Systems: A Meta-Analysis, Front. Plant Sci., 2020, 10, 1727 CrossRef.
- Y. Ceylan, U. B. Kutman, M. Mengutay and I. Cakmak, Magnesium applications to growth medium and foliage affect the starch distribution, increase the grain size and improve the seed germination in wheat, Plant Soil, 2016, 406(1), 145–156 CrossRef CAS.
- M. Jezek, C. M. Geilfus, A. Bayer and K. H. Muhling, Photosynthetic capacity, nutrient status, and growth of maize (Zea mays L.) upon MgSO4 leaf-application, Front. Plant Sci., 2015, 5, 781 Search PubMed.
- M. E. Maguire and J. A. Cowan, Magnesium chemistry and biochemistry, BioMetals, 2002, 15(3), 203–210 CrossRef CAS.
- R. Karimi, A. Saberi and A. Khadivi, Effects of foliar spray of agricultural grade mineral oil in springtime, in combination with potassium and calcium sulfates on the phenological and biophysical indices of clusters, and foliar nutritional levels in seedless, Sultanina, Biol. Res., 2021, 54(1), 28 CrossRef CAS PubMed.
- S. Nigar, S. Nazneen, S. Khan, N. Ali and T. Sarwar, Response of Vigna radiata L. (Mung Bean) to Ozone Phytotoxicity Using Ethylenediurea and Magnesium Nitrate, J. Plant Growth Regul., 2021, 1–13 Search PubMed.
- W. C. Li, R. Y. Fan, H. J. Zhou, Y. F. Zhu, X. Zheng, M. Y. Tang, X. S. Wu, C. Z. Yu and G. Z. Wang, Improving the utilization rate of foliar nitrogen fertilizers by surface roughness engineering of silica spheres, Environ. Sci.: Nano, 2020, 7(11), 3526–3535 RSC.
- N. K. Fageria, M. P. Barbosa, A. Moreira and C. M. Guimaraes, Foliar Fertilization of Crop Plants, J. Plant Nutr., 2009, 32(6), 1044–1064 CrossRef CAS.
- V. Fernandez and P. H. Brown, From plant surface to plant metabolism: the uncertain fate of foliar-applied nutrients, Front. Plant Sci., 2013, 4, 289 Search PubMed.
- V. Fernandez and T. Eichert, Uptake of Hydrophilic Solutes Through Plant Leaves: Current State of Knowledge and Perspectives of Foliar Fertilization, Crit. Rev. Plant Sci., 2009, 28(1–2), 36–68 CrossRef CAS.
- R. A. Meurer, S. Kemper, S. Knopp, T. Eichert, F. Jakob, H. E. Goldbach, U. Schwaneberg and A. Pich, Biofunctional Microgel-Based Fertilizers for Controlled Foliar Delivery of Nutrients to Plants, Angew. Chem., Int. Ed., 2017, 56(26), 7380–7386 CrossRef CAS.
- M. Wang, X. Sun, N. Q. Zhong, D. Q. Cai and Z. Y. Wu, Promising Approach for Improving Adhesion Capacity of Foliar Nitrogen Fertilizer, ACS Sustainable Chem. Eng., 2015, 3(3), 499–506 CrossRef CAS.
- S. T. Xie, L. L. Ai, C. Cui, T. Fu, X. D. Cheng, F. L. Qu and W. H. Tan, Functional Aptamer-Embedded Nanomaterials for Diagnostics and Therapeutics, ACS Appl. Mater. Interfaces, 2021, 13(8), 9542–9560 CrossRef CAS.
- P. Horcajada, T. Chalati, C. Serre, B. Gillet, C. Sebrie, T. Baati, J. F. Eubank, D. Heurtaux, P. Clayette, C. Kreuz, J. S. Chang, Y. K. Hwang, V. Marsaud, P. N. Bories, L. Cynober, S. Gil, G. Ferey, P. Couvreur and R. Gref, Porous metal-organic-framework nanoscale carriers as a potential platform for drug delivery and imaging, Nat. Mater., 2010, 9(2), 172–178 CrossRef CAS.
- M. Kah, R. S. Kookana, A. Gogos and T. D. Bucheli, A critical evaluation of nanopesticides and nanofertilizers against their conventional analogues, Nat. Nanotechnol., 2018, 13(8), 677–684 CrossRef CAS PubMed.
- L. M. Gilbertson, L. Pourzahedi, S. Laughton, X. Y. Gao, J. B. Zimmerman, T. L. Theis, P. Westerhoff and G. V. Lowry, Guiding the design space for nanotechnology to advance sustainable crop production, Nat. Nanotechnol., 2020, 15(9), 801–810 CrossRef CAS PubMed.
- L. J. Zhao, L. Lu, A. D. Wang, H. L. Zhang, M. Huang, H. H. Wu, B. S. Xing, Z. Y. Wang and R. Ji, Nano-Biotechnology in Agriculture: Use of Nanomaterials to Promote Plant Growth and Stress Tolerance, J. Agric. Food Chem., 2020, 68(7), 1935–1947 CrossRef CAS.
- S. Dhiman, A. Yadav, N. Debnath and S. Das, Application of Core/Shell Nanoparticles in Smart Farming: A Paradigm Shift for Making the Agriculture Sector More Sustainable, J. Agric. Food Chem., 2021, 69(11), 3267–3283 CrossRef CAS.
- D. Kanjana, Foliar application of magnesium oxide nanoparticles on nutrient element concentrations, growth, physiological, and yield parameters of cotton, J. Plant Nutr., 2020, 43(20), 3035–3049 CrossRef CAS.
- J. Khordadi Varamin, F. Fanoodi, J. Masoud Sinaki, S. Rezvan and A. Damavandi, Foliar application of chitosan and nano-magnesium fertilizers influence on seed yield, oil content, photosynthetic pigments, antioxidant enzyme activities of sesame (Sesamum indicum L.) under water-limited conditions, Not. Bot. Horti Agrobot. Cluj-Napoca, 2020, 48(4), 2228–2243 CrossRef CAS.
- W. N. Wang, J. C. Tarafdar and P. Biswas, Nanoparticle synthesis and delivery by an aerosol route for watermelon plant foliar uptake, J. Nanopart. Res., 2013, 15(1), 1–13 Search PubMed.
- T. K. Barik, B. Sahu and V. Swain, Nanosilica—from medicine to pest control, Parasitol. Res., 2008, 103(2), 253–258 CrossRef CAS.
- W. Stöber, A. Fink and E. Bohn, Controlled growth of monodisperse silica spheres in the micron size range, J. Colloid Interface Sci., 1968, 26(1), 62–69 CrossRef.
- I. Cakmak and E. A. Kirkby, Role of magnesium in carbon partitioning and alleviating photooxidative damage, Physiol. Plant., 2008, 133(4), 692–704 CrossRef CAS PubMed.
- C. Hermans and N. Verbruggen, Physiological characterization of Mg deficiency in Arabidopsis thaliana, J. Exp. Bot., 2005, 56(418), 2153–2161 CrossRef CAS PubMed.
- Y. F. Lu, Y. Zhang, Y. F. Deng, W. Jiang, Y. P. Zhao, J. J. Geng, L. L. Ding and H. Q. Ren, Uptake and Accumulation of Polystyrene Microplastics in Zebrafish (Danio rerio) and Toxic Effects in Liver, Environ. Sci. Technol., 2016, 50(7), 4054–4060 CrossRef CAS PubMed.
- A. V. Kalueff, A. M. Stewart and R. Gerlai, Zebrafish as an emerging model for studying complex brain disorders, Trends Pharmacol. Sci., 2014, 35(2), 63–75 CrossRef CAS PubMed.
- Y. J. Gao, C. W. Chen, D. F. Wang, L. H. Zhang, D. Q. Cai and Z. Y. Wu, TiO2/Biochar with Light-Switchable Wettability as a Herbicide Safener and Foliar Fertilizer Adhesive, ACS Sustainable Chem. Eng., 2020, 8(2), 1121–1128 CrossRef CAS.
- M. Zheng, J. G. Lu and D. Y. Zhao, Effects of starch-coating of magnetite nanoparticles on cellular uptake, toxicity and gene expression profiles in adult zebrafish, Sci. Total Environ., 2018, 622, 930–941 CrossRef PubMed.
- X. L. Zhang, Q. X. Zhou, W. Zou and X. G. Hu, Molecular Mechanisms of Developmental Toxicity Induced by Graphene Oxide at Predicted Environmental Concentrations, Environ. Sci. Technol., 2017, 51(14), 7861–7871 CrossRef CAS PubMed.
- Q. Fang, X. J. Shi, L. P. Zhang, Q. W. Wang, X. F. Wang, Y. Y. Guo and B. S. Zhou, Effect of titanium dioxide nanoparticles on the bioavailability, metabolism, and toxicity of pentachlorophenol in zebrafish larvae, J. Hazard. Mater., 2015, 283, 897–904 CrossRef CAS PubMed.
- T. Wu, X. L. Fang, Y. Yang, W. Y. Meng, P. J. Yao, Q. Liu, B. Zhang, F. Liu, A. H. Zou and J. G. Cheng, Eco-friendly Water-Based lambda-Cyhalothrin Polydopamine Microcapsule Suspension with High Adhesion on Leaf for Reducing Pesticides Loss, J. Agric. Food Chem., 2020, 68(45), 12549–12557 CrossRef CAS PubMed.
|
This journal is © The Royal Society of Chemistry 2023 |