Validation of a modified IDEXX defined-substrate assay for detection of antimicrobial resistant E. coli in environmental reservoirs†
Received
3rd May 2022
, Accepted 26th November 2022
First published on 1st December 2022
Abstract
The emergence of antimicrobial resistant (AMR) bacteria has been identified as one of the principal public health threats of the 21st century. The World Health Organization (WHO) has long recognized the threat of AMR bacteria and highlights environmental surveillance as a key step in understanding and combating the global rise of antimicrobial resistance. Here, we modified and validated an IDEXX defined-substrate assay commonly used for recreational water quality monitoring of E. coli to enumerate cefotaxime resistant E. coli in environmental reservoirs. We then applied this method to understand AMR trends in multiple environmental matrices over time. This modified IDEXX assay performed highly similarly to two widely accepted plating methods (TBX and MacConkey agar) for enumerating AMR bacteria in pure culture samples and environmental matrices, indicating it is a valid method for enumerating AMR E. coli in the environment. We detected AMR E. coli in urban surface water (63%, 15/24 samples), surface soil (35%, 8/23), and waterfowl feces (43%, 3/7). Sampling around a heavy rain event also revealed that concentrations of AMR E. coli and total E. coli co-vary over time in both surface water and surface soil. This novel method can reliably be performed outside of a laboratory setting and has very low equipment requirements, meaning it has tremendous potential to bolster global monitoring efforts, particularly in resource-restricted and highly rural settings.
Environmental significance
Escherichia coli has one of the highest rates of resistance in bacteria. The World Health Organization has classified antimicrobial resistant (AMR) E. coli as a public health threat that requires prompt and sustained action due to its ability to spread rapidly and cause or complicate infections in otherwise healthy individuals. The large number of AMR E. coli present in the environment, along with the interactions of humans and animals, quicken and sustain the proliferation and spread of AMR genes. This research validates a novel method for enumerating AMR E. coli in the environment (e.g., water, soil, feces). This technique can aid in global surveillance as it does not require advanced lab equipment and can be employed in low-resource settings.
|
Introduction
Antimicrobials have been crucial to achievements in modern medicine, however, the emergence of antimicrobial resistant (AMR) bacteria has been identified as one of the principal public health threats of the 21st century.1 Annual deaths attributable to drug-resistance are predicted to increase from an estimated 4.95 million in 2019
2 to 10 million deaths annually by 2050, representing a cumulative 100 trillion USD of economic output at risk.3
Global data on the scale of antibiotic resistance in environmental reservoirs that is both geographically explicit and timely is currently missing and inhibits an effective global surveillance system.4,5 The WHO has long recognized the need for coordinated global action to avert a global healthcare crisis and has highlighted environmental surveillance as a key step in understanding and combating the global rise of AMR bacteria.17 Standard fecal bacteria monitoring already forms much of the global regulatory fabric, and researchers have suggested an effective method for integrating AMR surveillance is to adapt an existing standard method to focus on resistant strains of concern.6 The IDEXX Quanti-Tray system is an US EPA approved semiautomated quantification method based on the Most Probable Number (MPN) model7 and is widely used in recreational water,8,9 wastewater,10,11 and drinking water12–14 quality monitoring for quantifying coliforms, E. coli, enterococci, and Heterotrophic Plate Counts. One prior study confirmed that a modified version of the IDEXX assay could detect antimicrobial resistant organisms in wastewater and surface water.15 However, this study did not compare the enumeration accuracy of the modified IDEXX system to traditional plating methods or evaluate its efficacy with other environmental reservoirs. Using the IDEXX system for AMR testing could fill a crucial gap in global monitoring of AMR organisms in the environment, particularly in highly rural and resource restricted settings, and inform effective management.
This study seeks to validate a modified IDEXX defined-substrate assay for detection and enumeration of cefotaxime resistant E. coli in multiple environmental reservoirs. Cefotaxime was used in this study since testing for cefotaxime-resistance has been proposed as a lower cost alternative for quantifying presumptive ESBL-producing E. coli,6 a multi-drug resistant strain of particular global concern.1 Enumeration and detection accuracy were compared to accepted membrane filtration and plating methods with Tryptone Bile X-glucuronide (TBX) and MacConkey agar.16 Experiments were performed on pure culture samples and environmental samples (surface water, surface soil, waterfowl feces) then used to understand temporal trends and distributions of AMR E. coli in environmental matrices in an urban setting.
Materials and methods
Experimental design
Three distinct control strains were used in this analysis: EC NC11 (cefotaxime resistant E. coli strain), EC25922 (ATCC reference strain of antibiotic susceptible E. coli), and Klebsiella pneumoniae (ATCC reference strain of non-E. coli total coliform). The three enumeration methods (IDEXX, MacConkey, TBX) were compared with varying sample input material. Pure Culture Samples were comprised of deionized (DI) water spiked with liquid pure cultures of EC NC11 (n = 9), EC25922 (n = 9), and K. pneumoniae (n = 9). Matrix Control Samples were comprised of environmental samples (surface water (n = 3), surface soil (n = 3), waterfowl feces (n = 3)) spiked with liquid pure cultures of EC NC11 (n = 6) and EC 25922 (n = 6). Environmental Samples include surface water (n = 27), surface soil (n = 27), and waterfowl feces (n = 5) without any introduction of control strains. Environmental samples were collected from various public locations around Raleigh, NC between June and November of 2020. Table S1† further describes the experimental design. Sample collection details can be found in the ESI† (pages 2 and 3).
Enumeration using IDEXX defined substrate assay
Samples were processed using the standard protocol for the IDEXX defined-substrate assay with Colilert-18 media and Quanti-Tray/2000.5 A second aliquot of each sample was processed using an amended IDEXX protocol to detect cefotaxime resistant E. coli, where 80 μL of filter-sterilized cefotaxime antibiotic (Sigma Aldrich, St. Louis, MO) solution at a concentration of 5 mg mL−1 (resulting in a final concentration of 4 μg mL−1 in the 100 mL sample solution)6 was pipetted into each sample bottle after Colilert-18 media was completely dissolved (see protocol.io for a full SOP, https://dx.doi.org/10.17504/protocols.io.5qpvorkz9v4o/v1). Standard methods were followed for membrane filtration and plating with MacConkey (BD, Franklin Lakes, NJ) and TBX (Sigma Aldrich, St. Louis, MO) agar. Additional details can be found in the ESI† (pages 4 and 5).
Confirmation testing
Cefotaxime resistant isolates from a subset of environmental samples were recovered from each assay (IDEXX, TBX, MacConkey). ESBL production amongst the archived cefotaxime-resistant isolates was confirmed phenotypically by using a combination disk diffusion test.18 See ESI† for additional details (pages 5 and 6).
Data analysis
One-way ANOVA tests with Tukey multiple-pairwise comparisons were used to compare the 3 assays, unless a non-parametric alternative (i.e., Kruskal–Wallis rank sum test) was needed. GEE (generalized estimating equation) models were fitted to compare detection (presence/absence) of cefotaxime resistant E. coli in different environmental matrices (surface water, surface soil) with assay and sample date as predictors while accounting for clustering by sample site. Additional bivariate GEE models were then fitted to explore predictors of cefotaxime resistant E. coli concentrations in surface water and surface soil over time.
Results
Pure culture experiment
As expected, E. coli was not detected in samples spiked with K. pneumoniae across all assays (n = 9), demonstrating the specificity of the assays to E. coli. Additionally, all samples spiked with EC25922 (a non-resistant strain) and treated with cefotaxime resulted in no growth (See Table S2† for more details).
Fig. 1 illustrates how IDEXX and MacConkey performed highly similarly, whereas TBX consistently enumerated at a lower level across the pure culture treatment conditions. Tukey multiple pairwise comparisons revealed TBX performed significantly differently than IDEXX for pure cultures of EC NC11 treated and not treated with cefotaxime (p < 0.05) (see ESI† for more details). TBX was also significantly different than IDEXX (p = 0.049) when spiked with EC25922 and not treated with cefotaxime, however, TBX and MacConkey were not significantly different (p = 0.110) and MacConkey and IDEXX were not significantly different (p = 0.801).
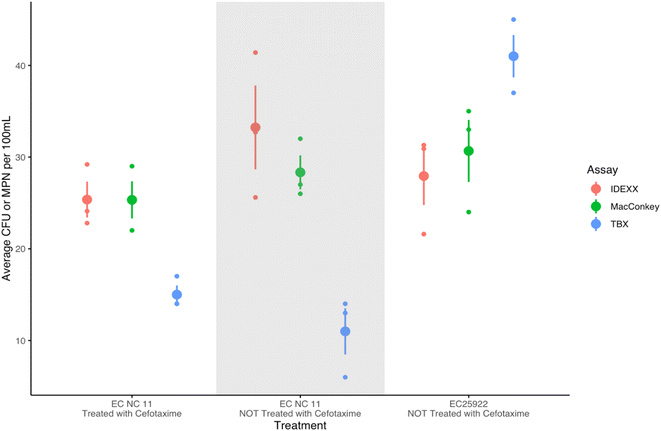 |
| Fig. 1 Mean, standard deviation, and scatter of three treatment conditions processed by IDEXX, MacConkey, and TBX in triplicate. Large dots in the center of each line represent the mean value of the three samples processed which are shown as smaller dots. Each line represents the standard deviation of three observations, and all measurements have units of MPN or colony forming unit (CFU) per 100 mL of sample according to assay. Three treatment conditions of interest are shown along the x-axis: (1) a control strain spike of EC NC11 (a resistant strain) treated with cefotaxime, (2) a control strain spike of EC NC11 with no cefotaxime added, and (3) a control strain spike of EC25922 (a non-resistant strain) with no cefotaxime added. | |
Matrix control experiments
The three assays resulted in similar concentrations of cefotaxime resistant E. coli in surface water samples spiked with EC NC11 and treated with cefotaxime (see Fig. 2), with means of 20.6 MPN E. coli per 100 mL (SD = 3.7 MPN E. coli per 100 mL) for the IDEXX assay, 23.1 CFU E. coli per 100 mL (SD = 8.2 CFU E. coli per 100 mL) for MacConkey, and 23.3 CFU E. coli per 100 mL (SD = 4.6 CFU E. coli per 100 mL) for TBX. A Kruskal–Wallis rank sum test indicated no statistically significant differences between the performance of these assays on spiked surface water samples. A one-way ANOVA test indicated no statistically significant differences between the performance of these assays on surface soil samples as well, shown in the shaded region of Fig. 2. For the waterfowl feces, MacConkey performed significantly differently than IDEXX (p = 0.010) and TBX (p = 0.041), but TBX and IDEXX did not perform significantly differently (p = 0.803) (Tukey post hoc tests).
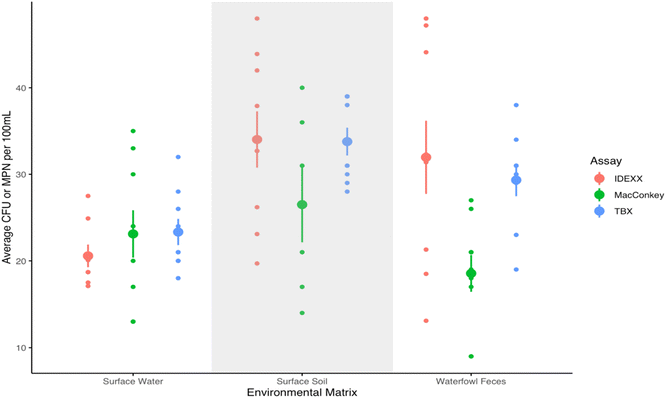 |
| Fig. 2 Enumeration of EC NC11 in environmental matrices displayed by assay. Data represent three water, three soil, and three fecal samples taken from various locations around Raleigh, NC over four months of sampling. Samples were spiked with EC NC11 and treated with the prescribed dose of cefotaxime then processed in triplicate by the IDEXX, MacConkey, and TBX. Large dots in the center of each line represent the mean value of the samples processed which are shown as smaller dots. Each line represents the standard deviation of all observations, and all measurements have units of MPN or CFU per 100 mL of sample according to assay. | |
Environmental samples
IDEXX, MacConkey, and TBX assays were compared in detecting naturally occurring cefotaxime resistant E. coli in environmental surface water, surface soil, and waterfowl feces, and this was done based on presence/absence since a large fraction of samples (>30%) were non-detects.
Individual GEE models for surface water (n = 60; see Table S3†) and surface soil (n = 58; see Table S3†) were fitted to predict the presence/absence of cefotaxime resistant E. coli with assay as the key predictor (and IDEXX as the reference category) in order to determine if resistant E. coli detection was influenced by assay. Neither MacConkey nor TBX were significant predictors in the model for surface water samples, indicating similar performances between the three assays. Only the MacConkey assay was a significant predictor in the model for surface soil samples, but once again the IDEXX and TBX assays performed similarly.
Contingency tables (see Table S4†) were also created to compare detection of cefotaxime resistant E. coli with the three protocols for the environmental samples. Despite the presence of discordant pairs which represent inconsistencies in detection between assays, the McNemar's exact tests for surface water and surface soil were not statistically significant (p > 0.05), indicating there was not a pattern among the discordant pairs.
Trends of cefotaxime resistant E. coli in the environment
We detected cefotaxime resistant E. coli in 63% (15/24) of surface water samples, 35% (8/23) of surface soil samples, and 43% (3/7) of waterfowl fecal samples. Among samples where we detected resistant E. coli, concentrations ranged from 1.0–13.1 MPN E. coli per 100 mL in surface water, 0.3–8.0 MPN E. coli per gram wet surface soil, and 1.73 × 105–2.42 × 107 MPN E. coli per gram waterfowl feces.
Rainfall during the 24 hours prior to sample collection was not a statistically significant predictor for the concentration of cefotaxime resistant E. coli in surface water (p = 0.069, β = −0.965, Table S5† Model 1) or surface soil (p = 0.416, β = −0.968, Table S5† GEE Model 1). However, our data may have been too sparse to detect significant differences since only one period of sampling intentionally captured a heavy rainfall event. We observed an apparent spike in concentrations of total E. coli and cefotaxime resistant E. coli in surface water at all sample sites directly after an intense rainfall event (see Fig. S1†).
Based on a separate GEE model, concentration of total E. coli was found to be a significant predictor of the concentration of cefotaxime resistant E. coli in both the surface water (p < 0.001) and surface soil (p = 0.013) models, indicating concentrations of total E. coli and resistant E. coli co-vary (see Table S5,† Model 2). However, the incremental increase in cefotaxime resistant E. coli was small. A 1000 MPN increase in total E. coli was associated with a 1 MPN and 6 MPN increase in cefotaxime resistant E. coli in surface water and surface soil, respectively.
Confirmation testing and QA/QC
The fraction of confirmed ESBL-producing isolates ranged from 0–60% for water samples, 14–43% for soil samples, and 0–33% for fecal samples. All field and lab blanks were processed per the standard and antibiotic-added IDEXX, MacConkey, and TBX protocols and all were free of total coliforms and E. coli. More details can be found in the ESI.†
Discussion
The modified IDEXX assay is an effective method for detecting cefotaxime resistant E. coli in surface water, surface soil, and fecal samples
This new modified IDEXX assay was rigorously tested across numerous experimental conditions with varied sample matrix, antibiotic treatment, and control strain additions. Across all conditions, IDEXX performed highly similarly to MacConkey, TBX, or often both. MacConkey agar is commonly used to culture organisms from urine,19,20 blood21 and inoculated nutrient broth.22 These sample types are likely to have high concentrations of the target organism and less inhibitors than environmental samples, and thus more closely resemble our pure culture experiment. IDEXX performed highly similarly to the MacConkey assay both with and without antibiotic treatment and with spiked culture of resistant and non-resistant organisms (Fig. 1) in our pure culture experiment where we would expect optimal performance from the MacConkey assay. TBX agar is widely used to enumerate E. coli in recreational water23 and wastewater,24,25 and is designed to be resilient to potential growth inhibitors in environmental matrices. IDEXX performed highly similarly to the TBX assay across all environmental matrices (Fig. 2) where we would expect optimal performance from the TBX assay.
In combination, data from these experiments indicate the modified IDEXX assay accurately enumerates cefotaxime resistant E. coli in environmental samples. Further, IDEXX is the preferred enumeration method for highly turbid surface water and surface soil samples. High turbidity in surface water samples or surface soil elutions posed serious complications for membrane filtration of samples (e.g., time intensive, clogging of filter) and growth on plated media (e.g., difficulty distinguishing colonies). IDEXX appeared unaffected by these highly turbid samples and still clearly fluoresced under UV light.
Our study findings have notable implications on global monitoring efforts. Accessing laboratory equipment and skilled personnel required for plating methods can be geographically infeasible in highly rural communities. Standard practice dictates environmental samples should be transported to a lab within six hours after collection and processed within two hours of receipt at the lab.26 Particularly in low- and middle-income countries or remote areas, many communities will fall outside of this radius from a lab. These communities represent a key gap in current monitoring data and can provide important information about the extent of global environmental contamination with antimicrobial resistant organisms. The IDEXX Quanti-Tray/2000 system has a similar per-test cost ($6.00 USD) and higher specialized equipment cost ($4100 USD; one-time purchase) compared to other MPN microbial drinking water tests.27 However, the fully sealed design of IDEXX trays has important implications for personnel working with cultured antibiotic resistant organisms outside of a biosafety cabinet. Another important consideration for this modified IDEXX assay is presence of reliable refrigeration for antibiotic storage in remote settings. Adoption of this modified IDEXX assay will allow for diversified monitoring locations while utilizing existing regulatory and surveillance infrastructure.
Concentrations of total E. coli and cefotaxime resistant E. coli co-vary in surface water and surface soil
A predictor of cefotaxime resistant E. coli in our data was the corresponding concentration of total E. coli in the same sample. While this was a significant relationship, it is important to note the proportion of resistant E. coli to total E. coli was generally low, and never exceeded two percent for both surface water and surface soil samples. Limited information on the health implications of contacting various levels of AMR organisms28 makes it difficult to determine the clinical significance of detecting low levels (5–10 MPN per 100 mL) of resistant E. coli. The risk thresholds for generic E. coli may not hold for AMR E. coli. A dose–response model for estimating infection risk of antibiotic resistant bacteria was created using data from bench experiments to compute total risk and to predict if illness is treatable with antibiotics,29 however a model for human exposure to AMR bacteria in environmental reservoirs is still needed to estimate risk. Additional data from varying environmental, socioeconomic, and cultural contexts on the concentration of total and AMR E. coli in surface water and surface soil are needed to determine if monitoring total E. coli could be an effective tool for estimating AMR E. coli concentrations and health risks, or if separate AMR indicators should also be monitored.
Rainfall was also identified as a potential influence on the concentration of cefotaxime resistant E. coli in surface water. The relationship between rainfall and elevated contamination levels in surface water has been investigated in numerous studies. One study found a significant positive relationship between outflow E. coli density and rainfall intensity in urban stormwater outflows30 and another study found a positive correlation between rainfall and gene concentrations in the Lahn River in Germany.31 Further modeling of this relationship could help disentangle the roles of rainfall intensity and time since rainfall in predicting contamination spikes to inform public health policy for urban recreational waters.
Limitations
Higher frequency sampling (e.g., hourly) around the rainfall event would have been helpful in characterizing the spike, and subsequent decline, in contamination after heavy rainfall. Further, road closures at one sample site due to flooding after Hurricane Isaias limited our sample frame and prevented collection of water, soil, and feces directly after rains when we observed a spike in E. coli concentrations at other sites. Lastly, significant delays in lab material deliveries due to COVID-19 resulted in a few samples only being processed with the IDEXX assay and reduced environmental sample sizes for MacConkey and TBX.
Next steps
Use of this modified IDEXX assay with additional antibiotics could allow researchers to explore resistance to other antibiotics and test for multi-drug resistance, an important step in assessing risk. It is unlikely different antibiotics would interact with the Colilert-18 media (as is the case with other selective media32) in such a way that results would be markedly different from those of our experiments. Thus, extensive validation experiments may not be needed before using this assay with other antibiotics. The IDEXX Quanti-Tray system can also be used to enumerate enterococci, Pseudomonas aeruginosa, heterotrophic plate counts (HPC), and Legionella pneumophila.7Pseudomonas aeruginosa and Enterococcus faecium (a type of enterococci) appear on the WHO's list of critical and high priority pathogens for development of new antimicrobials and numerous studies have explored antibiotic resistance of Legionella pneumophila. These organisms require the use of different selective medias and we are uncertain how those medias may interact with added antibiotics. For this reason, we recommend additional validation studies be performed before using the IDEXX system to enumerate non-E. coli AMR organisms.
Improvements in sequencing technologies have made whole genome sequencing (WGS) a quick and valuable tool in environmental surveillance of AMR E. coli. Future research could explore how WGS workflows can pair with the IDEXX method to quickly enumerate AMR E. coli in environmental reservoirs and yield more specific information on the type of AMR E. coli detected to inform better risk assessment and local policy decisions while advancing scientific understanding of these organisms.
Identifying improved methods for extending environmental surveillance is imperative. Our study validates one such method and demonstrates its utility in characterizing environmental hazards for antimicrobial resistance in multiple environmental reservoirs. More robust, high quality monitoring data on antimicrobial resistant organisms in the environment can inform strategies to interrupt environmental transmission pathways and mitigate illness and mortality from this global, multifaceted phenomenon.
Conflicts of interest
There are no conflicts to declare.
Acknowledgements
The authors would like to thank Dr Amy Kirby at the CDC for sharing preliminary SOPs for this method. Thank you to Jeremy Lowe and Sean Daly for assisting with sample collection and processing. Thank you to Nigatu Atlaw for training students on susceptibility testing methods and being available to answer questions. Additionally, we would like to thank Lisa Castellano for maintaining safe working conditions throughout the COVID-19 pandemic and allowing this work to move forward.
References
-
CDC, Antibiotic Resistance Threats in the United States, Drug Resistance, 2019 Search PubMed.
- Antimicrobial Resistance Collaborators, Global burden of bacterial antimicrobial resistance in 2019: A systematic analysis, Lancet, 2022, 399 Search PubMed.
-
J. O'Neil, Tackling Drug Resistant Infections Globally: Final Report and Recommendations, 2016, https://apo.org.au/ Search PubMed.
- H. Grundmann, Towards a global antibiotic resistance surveillance system: A Primer for a roadmap, Upsala J. Med. Sci., 2014, 119, 87–95 CrossRef PubMed.
-
F. D. A. US, The National Antimicrobial Resistance Monitoring System Strategic Plan 2021–2025, 2020 Search PubMed.
- A. Pruden, R. E. Alcalde, P. J. J. Alvarez, N. Ashbolt, H. Bischel, N. L. Capiro, E. Crossette, D. Frigon, K. Grimes, C. N. Haas, K. Ikuma, A. Kappell, T. LaPara, L. Kimbell, M. Li, X. Li, P. McNamara, Y. Seo, M. D. Sobsey, E. Sozzi, T. Navab-Daneshmand, L. Raskin, M. V. Riquelme, P. Vikesland, K. Wigginton and Z. Zhou, An Environmental Science and Engineering Framework for combating antimicrobial resistance, Environ. Eng. Sci., 2018, 35, 1005–1011 CrossRef CAS.
- Quanti-Tray System-IDEXX U. S.
- N. Nafsin, B. Bevers, R. Schruender, Q. Liao and J. Li,
Escherichia coli and enterococci bacteria in Lake Michigan Beach sand, Environ. Eng. Sci., 2022, 39, 3–14 CrossRef CAS.
- X. Li, C. A. Kelty, M. Sivaganesan and O. C. Shanks, Variable fecal source prioritization in recreational waters routinely monitored with viral and bacterial general indicators, Water Res., 2021, 192, 116845 CrossRef CAS PubMed.
- M. De Sanctis, G. Del Moro, C. Levantesi, M. L. Luprano and C. Di Iaconi, Integration of an innovative biological treatment with physical or chemical disinfection for wastewater reuse, Sci. Total Environ., 2016, 543, 206–213 CrossRef CAS PubMed.
- M. Alameddine, Z. T. How and M. Gamal El-Din, Advancing the treatment of primary influent and effluent wastewater during wet weather flow by single versus powdered activated carbon-catalyzed ozonation for the removal of trace organic compounds, Sci. Total Environ., 2021, 770, 144679 CrossRef CAS PubMed.
- S. Maysarah, G. L. Putri, M. A. Pratama, F. Zulkarnain, J. Willetts, T. Foster, F. Genter, A. Harris and C. R. Priadi, The occurrence of Escherichia coli in groundwater of Bekasi City (case study: Jatiluhur, Sumur Batu, and Jatirangga urban villages), IOP Conf. Ser.: Earth Environ. Sci., 2020, 566, 012008 CrossRef.
- E. R. Fuhrmeister, K. L. Nelson, A. J. Pickering and A. Ercumen, Predictors of enteric pathogens in the domestic environment from human and animal sources in rural Bangladesh, Environ. Sci. Technol., 2019, 53, 10023–10033 CrossRef CAS PubMed.
- A. Ercumen, A. J. Pickering, L. H. Kwong, B. F. Arnold, S. M. Parvez, M. Alam, D. Sen, S. Islam, C. Kullmann, C. Chase, R. Ahmed, L. Unicomb, S. P. Luby and J. M. Colford, Animal feces contribute to domestic fecal contamination: evidence from E. coli measured in water, hands, food, flies, and soil in Bangladesh, Environ. Sci. Technol., 2017, 51, 8725–8734 CrossRef CAS PubMed.
- S. Galvin, F. Boyle, P. Hickey, A. Vellinga, D. Morris and M. Cormican, Enumeration and characterization of antimicrobial-resistant Escherichia coli bacteria in effluent from municipal, hospital, and Secondary Treatment Facility sources, Appl. Environ. Microbiol., 2010, 76, 4772–4779 CrossRef CAS PubMed.
- M. E. Jacob, S. Keelara, A. Aidara-Kane, J. R. Matheu Alvarez and P. J. Fedorka-Cray, Optimizing a screening protocol for potential extended-spectrum β-lactamase Escherichia coli on MacConkey Agar for use in a global surveillance program, J. Clin. Microbiol., 2020, 58, e01039-19 CrossRef PubMed.
-
WHO, WHO Integrated Global Surveillance on ESBL-Producing E. coli Using a “One Health” Approach: Implementation and Opportunities, Global Tricycle Surveillance for ESBL E. coli, 2021 Search PubMed.
-
CSLI, Performance Standards for Antimicrobial Susceptibility Testing, 2020 Search PubMed.
- Y. Kuwa, B. Regasa Dadi, M. Seid, G. Biresaw and A. Manilal, Catheter-associated urinary tract infection: Incidence, associated factors and drug resistance patterns of bacterial isolates in southern Ethiopia, Infect. Drug Resist., 2021, 14, 2883–2894 CrossRef PubMed.
- E. Ahmed, R. Barakzai, D. Muhammad, S. Rizvi and S. Akram, Urinary Tract Infections (UTI) among females caused by staphylococcus saprophyticus, Med. Forum Mon., 2012, 23, 35–37 Search PubMed.
- M. C. Lopes, B. S. Évora, T. A. Cidral, L. B. Botelho and M. C. Melo, Bloodstream Infection by Acinetobacter Radioresistens: the First Case Report in Brazil, J. Bras. Patol. Med. Lab., 2019, 55 Search PubMed.
- F. H. AlTuraifi, A. A. AlMomatin, L. Badger-Emeka, P. M. Emeka and M. M. Islam, Assessment of microbiological content of private and public recreational water facilities and their antimicrobial susceptibility pattern in al-Ahsa, Environ. Health Insights, 2019, 13, 117863021988739 CrossRef PubMed.
- S. Jozić, D. Vukić Lušić, A. Aljinović, W. Vlakančić, A. Cenov, A. Vrdoljak Tomaš, A. Rakić and M. Šolić, Is TBX agar a suitable medium for monitoring Escherichia coli in bathing water using the membrane filtration method?, Environ. Monit. Assess., 2019, 191 Search PubMed.
- V. Barbosa, M. Morais, A. Silva, C. Delerue-Matos, S. A. Figueiredo and V. F. Domingues, Comparison of antibiotic resistance in the influent and effluent of two wastewater treatment plants, AIMS Environ. Sci., 2021, 8, 101–116 CAS.
- P. Vergine, C. Salerno, E. Barca, G. Berardi and A. Pollice, Identification of the faecal indicator Escherichia coli in wastewater through the β-D-glucuronidase activity: Comparison between two enumeration methods, membrane filtration with TBX Agar, and Colilert®-18, J. Water Health, 2016, 15, 209–217 CrossRef PubMed.
- US EPA, Method 1106.1: Enterococci in Water by Membrane Filtration Using Membrane Enterococcus-Esculin Iron Agar (mE-EIA), 2009.
- R. Bain, J. Bartram, M. Elliott, R. Matthews, L. McMahan, R. Tung, P. Chuang and S. Gundry, A summary catalogue of microbial drinking water tests for low and Medium Resource Settings, Int. J. Environ. Res. Public Health, 2012, 9, 1609–1625 CrossRef PubMed.
- J. Profitós, A. Mouhaman, S. Lee, R. Garabed, M. Moritz, B. Piperata, J. Tien, M. Bisesi and J. Lee, Muddying the waters: A new area of concern for drinking water contamination in Cameroon, Int. J. Environ. Res. Public Health, 2014, 11, 12454–12472 CrossRef PubMed.
- S. Chandrasekaran and S. C. Jiang, A dose response model for quantifying the infection risk of antibiotic-resistant bacteria, Sci. Rep., 2019, 9, 17093 CrossRef PubMed.
- S. Lee, M. Suits, D. Wituszynski, R. Winston, J. Martin and J. Lee, Residential urban stormwater runoff: A comprehensive profile of microbiome and antibiotic resistance, Sci. Total Environ., 2020, 723, 138033 CrossRef CAS PubMed.
- I. Herrig, S. Fleischmann, J. Regnery, J. Wesp, G. Reifferscheid and W. Manz, Prevalence and seasonal dynamics of blactx-m antibiotic resistance genes and fecal indicator organisms in the lower Lahn River, Germany, PLOS ONE, 2020, 15 Search PubMed.
- K. L. Kasweck and C. B. Fliermans, Lactose variability of Escherichia coli in thermally stressed reactor effluent waters, Appl. Environ. Microbiol., 1978, 36, 739–746 CrossRef CAS PubMed.
Footnote |
† Electronic supplementary information (ESI) available. Additional experimental details, materials, methods and Tables S1–S5 and Figure S1. See DOI: https://doi.org/10.1039/d2em00189f |
|
This journal is © The Royal Society of Chemistry 2023 |