Organophosphate esters (OPEs) in atmospheric particulate matter in different Brazilian regions†
Received
31st May 2023
, Accepted 24th August 2023
First published on 30th August 2023
Abstract
Organophosphate esters (OPEs) have been widely used as plasticizers. Current studies have shown that these compounds may constitute atmospheric particulate matter (PM), but no research has focused on detecting these substances in Brazil. Hence, our research aims to determine OPEs concentrations in 26 PM10 samples from three Brazilian cities in different regions. The substances found were tri-n-butyl phosphate (TNBP), tris(1,3-dichloro-2-propyl)phosphate (TDCIPP), triphenyl phosphate (TPHP), tris(2-butoxyethyl)phosphate (TBOEP), 2-ethylhexyl diphenyl phosphate (EHDPHP) and tris(2-ethylhexyl)phosphate (TEHP). In Catalão, the most abundant compounds were TNBP and TPHP, in Limeira TPHP and TEHP, and in Novo Hamburgo TEHP and TBOEP. Statistical analyses were performed to correlate the concentrations with meteorological variables. Results showed that temperature had a negative correlation with TBOEP, relative humidity had a negative correlation with TPHP and TEHP, atmospheric pressure had a positive correlation with TPHP, and wind speed had a significant influence on total and TPHP. Moreover, human exposure to these substances presents a negligible risk, for both children and adults.
Environmental significance
Atmospheric particulate matter (PM) is a pollutant that can cause damage to human health. Given this scenario, it is of extreme relevance to research PM concentration and composition, especially when it includes the identification of pollutants. Organophosphate flame retardants (OPFRs) are ubiquitous contaminants frequently detected in PM. However, no study evaluated their occurrence in PM samples from Brazil. Thus, it is necessary to determine the concentration of these compounds in the atmosphere to evaluate their principal emission sources and risks. In this study, PM samples were collected in three different regions in Brazil and several OPFRs were frequently detected, but at levels that do not pose a risk to humans. Also, the influence of meteorological variables and anthropogenic activities was assessed.
|
1 Introduction
Atmospheric particulate matter (PM) can cause major damage to human health, especially particles with smaller diameters, since the damage is inversely proportional to their size.1 One fraction that deserves attention is PM10, a PM with an aerodynamic diameter less than or equal to 10 μm.2 Considered inhalable, PM10 is retained in the upper airways3 thus causing damage to human health, such as acute and chronic respiratory infections, aggravated asthma, difficulty breathing, as well as heart disease, which can lead to premature death.4
PM composition is quite varied, especially regarding its organic fraction, due to the high number and diversity of emitting sources.5–7 Organophosphate esters (OPEs), which are widely used in the industrial sector and in the manufacturing of household products to prevent the spread of fire, have been widely detected in PM.8,9
OPEs emerged as an alternative to polybrominated diphenyl ethers (PBDEs), which were characterized by persistence and bioaccumulation.10 However, knowledge advancements on the environmental behavior and toxicity of these compounds indicated hazard to humans and biota,11 thus justifying the recent significant increase in studies focusing on these substances.12
OPEs can present toxic12 and carcinogenic13 characteristics and can cause adverse effects to the immune14 and neurological15,16 systems, altered sex hormone production,17 lung epithelial cell death,18 among other damages to human and animal health. Moreover, these compounds can undergo atmospheric chemical transformations that further increase the risks they can cause to the population's health.12
In addition to the atmosphere,19,20 these substances have also been detected in other environmental matrices, such as water,21,22 sewage,23,24 sediment25,26 and soil.17,27 Such widespread presence in different matrices increases human exposure to OPEs, thus justifying the various studies being conducted on the risks posed to individuals.
In Brazil, there is still a significant shortage of studies on OPES in particulate matter. Given this situation, this study determined the OPE concentration in PM10 samples from the cities of Catalão, Limeira and Novo Hamburgo using GC-MS, identifying their possible sources, and evaluating the human exposure to these compounds. To the best of our knowledge, this is the first study on the occurrence and risk of human exposure to OPEs in PM in these 3 Brazilian cities.
2 Methods
2.1. PM sampling sites and methodology
Sampling was performed in three Brazilian cities, located in different regions: Catalão (CAT) – Goiás state, Limeira (LIM) – São Paulo state, and Novo Hamburgo (NH) – Rio Grande do Sul state; Midwest, Southeast, and South regions, respectively (Fig. 1). Catalão had an estimated population of 113
091 inhabitants in 2021 (ref. 28) and is characterized by mining, agriculture, and cultivated pastures.29 Limeira had a population of 310
783 inhabitants in 2021 (ref. 28) and its economy is based primarily on the semi-jewelry industry and sugar cane and citrus plantations.30 Novo Hamburgo had approximately 247
303 inhabitants in 2021 (ref. 28) and has a great diversity of industries, most notably the footwear sector.31 These three cities were selected because they are from different regions, have different meteorological characteristics, different possible emission sources and no previous studies on identifying OPEs in PM.
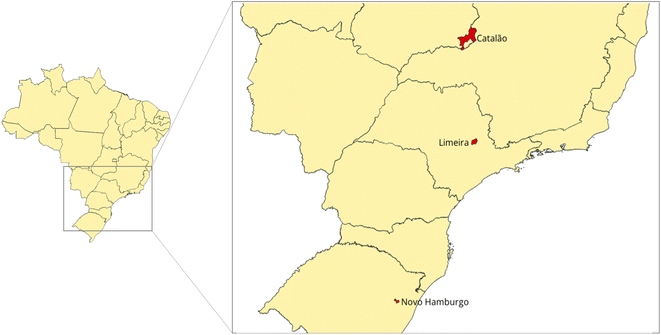 |
| Fig. 1 Location of cities where PM10 samples were collected. | |
Twenty-six PM10 samplings were conducted between June and July 2019 at the Federal University of Catalão (coordinates 18°09′17.3′′S; 47°55′38.7′′W), the School of Technology at the University of Campinas (coordinates 22°33′43.81′′S; 47°25′20.84′′W), and Feevale University (coordinates 29°40′12.4′′S; 51°07′15.7′′W), Novo Hamburgo.
All samples were collected using PM10 high volume samplers (Hi-Vol), with a constant flow of 1.13 m3 min−1 and the total sample volume of 1627.2 m3, always starting at midnight of one day and ending at midnight of the next day to complete a 24 h cycle. Fiberglass filters, porosity < 1 μm, pre-treated in a muffle furnace at 400 °C for 4 h to remove possible contaminants, were used for PM10 collection. Later, to remove moisture, they were kept in a desiccator for 24 h before sampling and only then weighed. After sampling, the filters remained for additional 24 h in the desiccator and were weighed again to obtain their mass by gravimetric analysis.
The blank filters were also kept in the muffle furnace and desiccator under the same conditions as the filters that were used for collection, but they remained 24 hours in a Hi-Vol with the device turned off.
2.2. Materials and reagents
We analyzed nine OPEs in total, including tri-n-butyl phosphate (TNBP), tris(chloroethyl)phosphate (TCEP), tris(1-chloro-2-propyl)phosphate (TCIPP), tris(1,3-dichloro-2-propyl)phosphate (TDCIPP), tris(2-butoxyethyl)phosphate (TBOEP), tris(2-ethylhexyl)phosphate (TEHP), triphenyl phosphate (TPHP), 2-ethylhexyl diphenyl phosphate (EHDPP) and trimethyl phenyl phosphate (TMPP). High purity analytical standards were acquired from Dr Ehrenstorfer (Augsburg, Germany) and the degree of purity of the standards is shown in Table S1.† Triphenyl phosphate-D15 (TPHP-D15) and tributyl phosphate-D27 (TNBP-D27) were purchased from Cambridge Isotope Laboratories (Andover, USA). HPLC grade hexane and acetone solvents were purchased from Honeywell Riedel-de-Haën (USA).
2.3. Sample preparation and extraction
For OPEs extraction, one eighth of the PM10 collected area in each filter was chopped into small pieces and transferred to a glass centrifuge tube with a lid. Each sample was spiked with 10 μL of a solution containing the deuterated surrogates TPHP-D15 and TNBP-D27 at a concentration of 5 ng μL−1. After adding 10 mL of hexane
:
acetone (1
:
1) to each tube, they were agitated in a vortex for 1 min, kept in an ultrasonic bath for 10 min, and centrifuged at 3500 rpm for 10 min. Each sample went through this complete cycle three times, but using 7 mL of hexane
:
acetone (1
:
1) instead of 10 mL in the second time and third time.
The extracts were then concentrated at a rotary evaporator until their almost complete evaporation. Clean-up used 1 g (6 mL) Florisil Cartridge (Supelco Supelclean™) conditioned with 12 mL of hexane
:
acetone (1
:
1). After removal from the rotary evaporator, the volumetric flask with the sample extracts was rinsed with two 1 mL portions of hexane
:
acetone (1
:
1), and the redissolved extract was transferred to Florisil Cartridges. Elution of the cartridges was performed using 6 mL of hexane
:
acetone (1
:
1). Subsequently, the samples were concentrated using ultrapure nitrogen gas flow (99
999%) in a water bath at 35 °C, until approximately 500 μL remained. The extracts were then transferred to 2 mL vials. For complete transfer of the analytes, the vial containing the extract was rinsed with two 400 μL portions of hexane
:
acetone (1
:
1). Finally, the extracts were completely evaporated using nitrogen gas flow, and redissolved with 500 μL of ethyl acetate.
2.4. Quality assurance (QA) and quality control (QC)
To ensure quality control, powder-free nitrile gloves were used to handle the samples throughout the process. Some glassware used, such as Pasteur pipettes, vials, and glass centrifuge tubes, were muffled for 12 h at 400 °C, and the others were washed with 4% Extran® MA02 neutral detergent (Merck), and finally rinsed with acetone (99.5%). Scissors and rulers were cleaned with acetone between cutting one filter and another. For each batch, a procedural blank was performed using a glass filter and the same extraction process, to evaluate any possible contamination.
Recovery assays were performed by preparing 3 fortified samples (spiked before extraction with 50 μL of a solution containing 9 OPEs at concentration of 4 ng μL−1 and with 10 μL of surrogate solution at concentration of 5 ng μL−1), 2 reference samples (spiked after extraction with the same amounts of standard solution and surrogate solution as described above) and 2 procedural blanks (spiked before extraction with 10 μL of surrogate solution). Since OPEs could be present in the PM samples used in the recovery assay, reference samples were used as a parameter for calculating recoveries, and the procedural blanks were used to evaluate selectivity and sample contamination during the extraction procedure. Extraction was performed as described in Section 2.2.
Analytical curve was obtained by preparing seven solutions at different concentrations, ranging from 0.005 to 0.5 mg L−1, using procedural blank filter extracts. At each calibration solution, 10 μL of a surrogate solution at a concentration of 5 ng μL−1 (TNBP-D27 and TPHP-D15) were added. Surrogate TNBP-D27 was used to quantify the compounds TNBP, TBOEP, EHDPHP and TEHP, whereas the surrogate TPHPD15 was used to quantify the compounds TCEP, TCIPP, TDCIPP, TPHP and TMPP.
Table S1† shows the method detection limits (MDL), method quantitation limits (MQL), recovery (REC), and standard deviation (SD) for each compound. MDL and MQL were calculated as 3 and 10 times the signal-to-noise ratio obtained for the lowest calibration solution, except for the compounds EHDPHP, TCIPP and TPHP, which were detected in the procedural blanks. For these compounds, MDL was calculated based on the mean blank level plus 3 times the standard deviation and the MQL was calculated as the mean blank level plus 10 times the standard deviation.
2.5. Instrumental analysis
All analyses were performed on a Trace GC Ultra Gas Chromatograph (Thermo) coupled to an ITQ900 mass spectrometer (Thermo) with ion trap mass analyzer. The injector was a programmable temperature vaporization (PTV). The glass liner used was the PTV Straight Liner (120 × 2.75 mm × 1 mm, Thermo). Solvent split injection mode was used, with a 5 μL injection volume, as reported by Cristale et al. 2020.32 The sampler used was automatic (Triplus). The column was a Zebron ZB5-MS (30 m × 0.25 mm × 0.25 μm) (Phenomenex, USA). The carrier gas was helium with a 1.5 mL min−1 flow rate. The column oven was held at 60 °C (6 min), subsequently heated at a 10 °C min−1 rate until it reached 230 °C (1 min), and finally heated at a rate of 20 °C min−1 until 280 °C (14 min). Injections were performed in SIM and MS/MS mode under the detection conditions shown in Tables S2 and S3.† Compounds were quantified using the SIM mode, as it showed better accuracy than the MS/MS. The results obtained using MS/MS mode were used as criteria for confirming the presence of OPEs in the sample extracts.32 TCEP and TCIPP were quantified by MS/MS, whereas TNBP, TDCIPP, TPHP, TBOEP, TEHP, TMPP and EHDPHP were quantified by SIM.
2.6. Analysis of OPEPs concentration in relation to meteorological variables
Pearson's correlation analysis was performed comparing the OPEs data detected with the PM10 concentration and the meteorological data (temperature, relative humidity, wind pressure, and wind speed, all variables on the logarithmic scale)33 obtained from the Meteorological and Monitoring Stations of each location. Correlation analysis was performed using the R programming language version 3.5.1.34
2.7. Health risk assessment
Risk related to human exposure to OPEs was assessed by calculating the Estimated Daily Intake (EDI) via PM inhalation using eqn (1). | 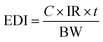 | (1) |
in which C is the concentration of each OPEs in the PM10 samples (ng m−3), IR is the inhalation rate (m3 d−1),33,35t is the outdoor exposure time (day), and BW is the average body weight (kg). IR was set as 10 m3 d−1 for children and 16 m3 d−1 for adults,36 the value of t assumed was 1 day, i.e., 100% of the time, assuming similar indoor and outdoor concentrations, and BW is considered as 70 kg for adults and 12 kg for children.37 Hazard Quotient (HQ) was calculated according to eqn (2), in which RfD is the reference dose value of each OPEs. HQ ≥ 1 indicates risk of adverse health effects.34 |  | (2) |
3 Results and discussion
3.1. OPEs concentration in the PM
We found mean PM10 concentrations of 28 μg m−3 for Catalão, 40 μg m−3 for Limeira, and 20 μg m−3 for Novo Hamburgo, ranging from 13–51 μg m−3, 6.6–70 μg m−3, and 11–33 μg m−3, respectively (Table S4†). Catalão city and the region where Novo Hamburgo is located have industrial activities and vehicular traffic as significant sources of PM emission.38,39 In Limeira, intense vehicle traffic, industrial emissions and biomass burning are important contribution sources.40 The OPEs found in the samples analyzed were TNBP, TDCIPP, TPHP, TBOEP, EHDPHP, and TEHP. While TCEP and TCIPP were probably not found in the PM as, according to the gas-particle partitioning models, these compounds should be predominantly present in the gaseous phase.41Fig. 2 shows the concentrations; those at levels >MDL and <MQL were represented by half of the MQL value.
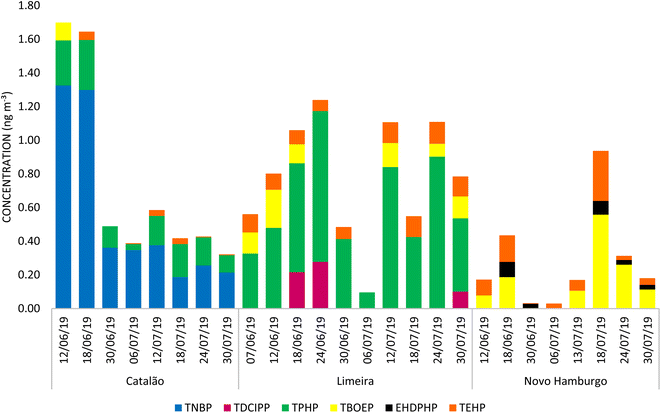 |
| Fig. 2 OPEs concentration in PM10 from Catalão, Limeira and Novo Hamburgo. | |
TNBP was detected only in samples from Catalão, compound with the highest concentrations in the locality, ranging from 0.19 to 1.3 ng m−3 (mean of 0.55 ng m−3). This compound has also been detected in PM in countries such as China, with mean concentration of 1.02 ng m−3,18 and the United States, with 0.56 ng m−3.42 TNBP can come from vehicle emissions, as it is used as a fluid in motor oil,12,43 as well as in paints.44
TPHP was also identified in all samples from the municipality, with a mean concentration of 0.17 ng m−3. Other studies have found mean PM concentrations close to this value in different places worldwide, such as Poland45 and China46 with 0.11 ng m−3, the United States with 0.2 ng m−3 (ref. 42) and Pakistan with 0.17 ng m−3.47 This compound can have, as some of its main emission sources, solid waste combustion, building materials, polymer additive and hydraulic fluids.43,45
The high concentrations of TNBP and TPHP found in Catalão are associated with the presence of a factory of precast concrete-based items, widely used in civil construction, that is located less than 1 km northwest of the sampled site. To the southeast, about 2.5 km away, a mining-chemical industrial district48 receives a daily high flow of heavy vehicles for loading and unloading inputs for manufacturing phosphate fertilizers. To the south/southeast, there is an industrial center with large-scale production of concrete, light automobiles, agricultural machinery, and production of paints from resins.
In Limeira, TPHP is the only compound to appear on all sampling days and present the highest concentrations, with a mean of 0.55 ng m−3 (range from 0.10 to 0.90 ng m−3). TEHP appears in all samples, with one exception, having a mean concentration of 0.10 ng m−3. Other research has found similar concentrations for this compound, such as in China with 0.106 ng m−3,49 Pakistan with 0.156 ng m−3 (ref. 47) and Spain with 0.12 ng m−3.50 This substance is commonly used as a plasticizer, in PVC, cellulose, lubricant, rubber and foam, and solvent.12,51
Specifically in Limeira, TEHP concentration may have tire wear as its main source, considering that the sample site is located near a roundabout with high vehicular traffic.
The two predominant compounds in Novo Hamburgo are TBOEP and TEHP, with means of 0.22 and 0.10 ng m−3, respectively. TBOEP has also been detected in PM in Spain with a 0.21 ng m−3 concentration50 and in China with 0.37 ng m−3.52 This substance is widely used in plastic and rubber products, wax, grease, paint, coating, and can have road traffic as an emission source.43,51 The sampling site is located near a federal highway and a state highway, as well as urban roads with heavy vehicular traffic.
3.2. Correlation between OPEs concentration and meteorological variables
PM10 concentration is directly correlated with the total number of OPEs and TPHP (Table 1).
Table 1 Correlation coefficient of OPEs with meteorological factors, including temperature, relative humidity, wind pressure and wind speed, and PM10 concentration
|
TNBP |
TPHP |
TBOEP |
TEHP |
ΣOPEs |
Means correlation was significant at 0.01 level.
Means correlation was significant at 0.05 level.
Means correlation was significant at 0.10 level.
|
PM10 |
0.071 |
0.765a |
−0.161 |
0.387 |
0.608a |
Temperature |
0.047 |
−0.063 |
−0.312 |
−0.025 |
0.240 |
Relative humidity |
−0.048 |
−0.739a |
−0.429 |
−0.833a |
−0.281 |
Pressure |
−0.357 |
0.483b |
0.478 |
0.136 |
−0.388c |
Wind speed |
−0.241 |
−0.709a |
−0.046 |
−0.457b |
−0.624a |
Temperature was not significantly correlated with any OPEs. The temperature variation in Catalão was 4.6 °C, in Limeira 12.1 °C and Novo Hamburgo 15 °C during the collection period. Conversely, relative humidity showed a significant negative correlation (p < 0.001) with TPHP and TEHP. Higher TEHP values occurred on days when relative humidity was below 35%, whereas higher TPHP values occurred on days when humidity was below 40%.
TPHP showed a positive correlation with atmospheric pressure. However, it was not identified in Novo Hamburgo (mean pressure of 762 mmHg). Between the cities of Limeira (mean of 708 mmHg) and Catalão (mean of 689 mmHg), the former had the highest TPHP values.
As seen in Table 1, wind speed had a significant influence on total (p < 0.001) and TPHP (p < 0.001), as observed for PM10. However, while PM10 correlation with total and TPHP was positive, with wind speed, it was negative. This is because the highest PM10 concentrations, and hence TPHP, are associated with low wind speeds, less than 0.5 m s−1. Low wind speed, low relative humidity, and low height of the mixing boundary layer hinder pollutant dispersion.40 TEHP also showed a significant negative correlation (p < 0.05) with wind speed, differently from the correlation between PM10 and TEHP.
3.3. Human exposure assessment
Table 2 presents the minimum, maximum, and mean values of the calculated EDI and HQ. Mean EDI for adults ranged from 0.009 to 0.13 ng kg per bw per day considering all municipalities. For children, mean EDI ranged from 0.033 to 0.46 ng kg per bw per day. Note that EDIs are higher for children than for adults due to lower body mass. The maximum HQs found illustrate the worst case scenario among the three cities and are well below 1, ranging from 1.5 × 10−5 to 10.7 × 10−5 for children and 0.42 × 10−5 to 3.0 × 10−5 for adults, indicating negligible health risks due to exposure to PM10-associated OPEs in Catalão, Limeira, and Novo Hamburgo. Other studies have also concluded that exposure to these compounds through atmospheric particulate matter does not represent a health risk, considering the current reference values.18,33
Table 2 Reference doses (RfD, ng kg−1 d−1), estimated daily intake (EDI, ng kg per bw per day) and hazard quotient (HQ) of OPEs for children and adults by inhalation exposurea
Compound |
RfD |
EDI Catalão |
EDI Limeira |
EDI Novo Hamburgo |
HQ (×10−5) |
Child |
Adult |
Child |
Adult |
Child |
Adult |
Child |
Adult |
Mean (range) |
Mean (range) |
Mean (range) |
Mean (range) |
Mean (range) |
Mean (range) |
Maximum |
Maximum |
Ref. 18.
|
TnBP |
24 000 |
0.46 (0.16–1.1) |
0.13 (0.043–0.30) |
— |
— |
— |
— |
4.6 |
1.3 |
TDCIPP |
15 000 |
— |
— |
0.17 (0.084–0.23) |
0.045 (0.023–0.063) |
— |
— |
1.5 |
0.42 |
TPHP |
70 000 |
0.14 (0.031–0.25) |
0.039 (0.008–0.068) |
0.46 (0.080–0.75) |
0.13 (0.022–0.21) |
— |
— |
11 |
3.0 |
TBOEP |
15 000 |
0.088 (—) |
0.024 (—) |
0.11 (0.064–0.19) |
0.031 (0.017–0.052) |
0.18 (0.066–0.47) |
0.050 (0.018–0.13) |
3.1 |
0.85 |
EHDPHP |
— |
— |
— |
— |
— |
0.072 (0.068–0.076) |
0.020 (0.019–0.021) |
— |
— |
TEHP |
— |
0.033 (0.029–0.041) |
0.009 (0.008–0.011) |
0.085 (0.055–0.11) |
0.023 (0.015–0.030) |
0.084 (0.020–0.25) |
0.023 (0.006–0.068) |
— |
— |
4 Conclusions
Our study showed the occurrence of OPEs in atmospheric PM10 from three Brazilian cities: Catalão, Limeira, and Novo Hamburgo. OPEs profile and concentration differed between the cities, indicating that the anthropic activities characteristic of each city and the meteorological variables influenced the presence of OPEs in the local atmosphere. Despite the high detection rate of various OPEs observed here, based on currently practiced reference values for non-carcinogenic risk, the levels found do not pose a threat to human health.
Author contributions
Priscila B. Gonçalves: data curation; formal analysis; investigation; methodology; validation; visualization; writing – original draft, writing – review & editing. Joyce Cristale: formal analysis; methodology; validation; writing – original draft. Amanda A. da Silva: formal analysis; methodology. Danilo C. Nogarotto: data curation; software. Daniela M. M. Osório: methodology; data curation. Lincoln L. Romualdo: methodology; data curation. Simone A. Pozza: conception; funding acquisition; investigation; methodology; project administration; resources; supervision; validation, visualization, writing – review & editing.
Conflicts of interest
The authors declare that they have no knowledge of competing financial interests or personal relationships that could have influenced the work reported in this paper.
Acknowledgements
This study was partly funded by CAPES – Finance Code No. 001. The authors thank, for financial support, CNPq (Grants: 403927-2016-1 and 428135/2018-8) and FAEPEX (Grants: 3346/2019 and 2591/2020). Joyce Cristale acknowledges the São Paulo Research Foundation (FAPESP) – Grant 2020/10918-9. The authors thank Espaço da Escrita – Pró-Reitoria de Pesquisa – UNICAMP for the language services provided.
References
-
WHO – World Health Organization, Type of pollutants, https://www.who.int/teams/environment-climate-change-and-health/air-quality-and-health/health-impacts/types-of-pollutants, 2022 Search PubMed.
-
J. H. Seinfeld and S. N. Pandis, Atmospheric Chemistry and Physics: From Air Pollution to Climate Change, Wiley, 3rd edn, 2016, pp. 1–1152 Search PubMed.
- J. Lelieveld and U. Pöschl, Chemists can help to solve the air-pollution health crisis, Nature, 2017, 551, 291–293 CrossRef PubMed.
-
USEPA – United States Environmental Protection Agency, Health and Environmental Effects of Particulate Matter (PM), 2022, cited 2022 Dec 18, available from, https://www.epa.gov/pm-pollution/health-and-environmental-effects-particulate-matter-pm Search PubMed.
- L. M. Russell, R. Bahadur and P. J. Ziemann, Identifying organic aerosol sources by comparing functional group composition in chamber and atmospheric particles, PNAS, 2011, 108, 3516–3521 CrossRef CAS PubMed.
- J. C. Ditto, T. Joo, J. H. Slade, P. B. Shepson, N. L. Ng and D. R. Gentner, Nontargeted Tandem Mass Spectrometry Analysis Reveals Diversity and Variability in Aerosol Functional Groups across Multiple Sites, Seasons, and Times of Day, Environ. Sci. Technol. Lett., 2020, 7(2), 60–69 CrossRef CAS.
- M. Ponczek, M. A. Franco, S. Carbone, L. V. Rizzo, D. Monteiro dos Santos and F. G. Morais,
et al., Linking the chemical composition and optical properties of biomass burning aerosols in Amazonia, Environ. Sci.: Atmos., 2021, 2(2), 252–269 Search PubMed.
- I. van der Veen and J. de Boer, Phosphorus flame retardants: properties, production, environmental occurrence, toxicity and analysis, Chemosphere, 2012, 88, 1119–1153 CrossRef CAS PubMed.
- A. Salamova, M. H. Hermanson and R. A. Hites, Organophosphate and halogenated flame retardants in atmospheric particles from a European Arctic site, Environ. Sci. Technol., 2014, 48(11), 6133–6140 CrossRef CAS PubMed.
- Y. Chen, Y. J. Chen, Y. Zhang, R. Li, W. Chen and S. C. Yan,
et al., Determination of HFRs and OPFRs in PM2.5 by ultrasonic-assisted extraction combined with multi-segment column purification and GC-MS/MS, Talanta, 2019, 194, 320–328 CrossRef CAS PubMed.
- I. Liagkouridis, A. P. Cousins and I. T. Cousins, Physical-chemical properties and evaluative fate modelling of “emerging” and “novel” brominated and organophosphorus flame retardants in the indoor and outdoor environment, Sci. Total Environ., 2015, 524–525, 416–426 CrossRef CAS PubMed.
- H. C. Kung, Y. K. Hsieh, B. W. Huang, N. K. Cheruiyot and G. P. Chang-Chien, An Overview: Organophosphate Flame Retardants in the Atmosphere, Aerosol Air Qual. Res., 2022, 22(7), 220148 CrossRef CAS.
- Y. Liu, Y. Li, S. Dong, L. Han, R. Guo and Y. Fu,
et al., The risk and impact of organophosphate esters on the development of female-specific cancers: comparative analysis of patients with benign and malignant tumors, J. Hazard. Mater., 2021, 404, 124020 CrossRef CAS PubMed.
- D. Canbaz, A. Logiantara, R. van Ree and L. S. van Rijt, Immunotoxicity of organophosphate flame retardants TPHP and TDCIPP on murine dendritic cells in vitro, Chemosphere, 2017, 177, 56–64 CrossRef CAS PubMed.
- T. A. Slotkin, S. Skavicus, H. M. Stapleton and F. J. Seidler, Brominated and organophosphate flame retardants target different neurodevelopmental stages, characterized with embryonic neural stem cells and neuronotypic PC12 cells, Toxicology, 2017, 390, 32–42 CrossRef CAS PubMed.
- Q. Wang, J. C. W. Lam, Y. C. Man, N. L. S. Lai, K. Y. Kwok and Y. y. Guo,
et al., Bioconcentration, metabolism and neurotoxicity of the organophorous flame retardant 1,3-dichloro 2-propyl phosphate (TDCPP) to zebrafish, Aquat. Toxicol., 2015, 158, 108–115 CrossRef CAS PubMed.
- Q. Luo, Y. Shan, A. Muhammad, S. Wang, L. Sun and H. Wang, Levels, distribution, and sources of organophosphate flame retardants and plasticizers in urban soils of Shenyang, China, Environ. Sci. Pollut. Res., 2018, 25(31), 31752–31761 CrossRef CAS PubMed.
- Y. Chen, Y. Song, Y. J. Chen, Y. Zhang, R. Li and Y. Wang,
et al., Contamination profiles and potential health risks of organophosphate flame retardants in PM2.5 from Guangzhou and Taiyuan, China, Environ. Int., 2020, 134, 105343 CrossRef CAS PubMed.
- B. L. Van Drooge, D. R. García and S. Lacorte, Analysis of organophosphorus flame retardants in submicron atmospheric particulate matter (PM1), AIMS Environ. Sci., 2018, 5(4), 294–304 CAS.
- D. Liu, T. Lin, K. Shen, J. Li, Z. Yu and G. Zhang, Occurrence and Concentrations of Halogenated Flame Retardants in the Atmospheric Fine Particles in Chinese Cities, Environ. Sci. Technol., 2016, 50(18), 9846–9854 CrossRef CAS PubMed.
- T. B. Chokwe and S. M. Mporetji, Organophosphorus flame retardants in surface and effluent water samples from the vaal river catchment, South Africa: levels and risk to aquatic life, Water SA, 2019, 45(3), 469–476 CAS.
- N. L. S. Lai, K. Y. Kwok, X. h. Wang, N. Yamashita, G. Liu and K. M. Y. Leung,
et al., Assessment of organophosphorus flame retardants and plasticizers in aquatic environments of China (Pearl River Delta, South China Sea, Yellow River Estuary) and Japan (Tokyo Bay), J. Hazard. Mater., 2019, 371, 288–294 CrossRef CAS PubMed.
- M. B. Woudneh, J. P. Benskin, G. Wang, R. Grace, M. C. Hamilton and J. R. Cosgrove, Quantitative determination of 13 organophosphorous flame retardants and plasticizers in a wastewater treatment system by high performance liquid chromatography tandem mass spectrometry, J. Chromatogr. A, 2015, 1400, 149–155 CrossRef CAS PubMed.
- L. Pang, Y. Yuan, H. He, K. Liang, H. Zhang and J. Zhao, Occurrence, distribution, and potential affecting factors of organophosphate flame retardants in sewage sludge of wastewater treatment plants in Henan Province, Central China, Chemosphere, 2016, 152, 245–251 CrossRef CAS PubMed.
- L. Hou, J. Jiang, Z. Gan, Y. Y. Dai, P. Yang and Y. Yan,
et al., Spatial Distribution of Organophosphorus and Brominated Flame Retardants in Surface Water, Sediment, Groundwater, and Wild Fish in Chengdu, China, Arch. Environ. Contam. Toxicol., 2019, 77(2), 279–290 CrossRef CAS PubMed.
- S. Lee, H. J. Cho, W. Choi and H. B. Moon, Organophosphate flame retardants (OPFRs) in water and sediment: occurrence, distribution, and hotspots of contamination of Lake Shihwa, Korea, Mar. Pollut. Bull., 2018, 130, 105–112 CrossRef CAS PubMed.
- Y. Wang, H. Sun, H. Zhu, Y. Yao, H. Chen and C. Ren,
et al., Occurrence and distribution of organophosphate flame retardants (OPFRs) in soil and outdoor settled dust from a multi-waste recycling area in China, Sci. Total Environ., 2018, 625, 1056–1064 CrossRef CAS PubMed.
-
IBGE – Instituto Brasileiro de Geografia e Estatística, Cidades, 2022, cited 2022 Aug 7, available from, https://cidades.ibge.gov.br/brasil/panorama.
- A. S. d. Silva and R. Rosa, Mapa de capacidade e potencial do uso da terra do município de Catalão (GO), Cad. Geogr., 2019, 29(59), 954–977 CrossRef.
- Limeira, Prefeitura Municipal de Limeira, Limeira: Município de Interesse Turístico, 2022, cited 2022 Aug 15, available from, https://www.limeira.sp.gov.br/sitenovo/service.php?servico=3%26categoria=null%26item=null%26conteudo=299.
-
F. K. Braun, História de Novo Hamburgo: 1824–1945, São Leopoldo, Oikos, 2016 Search PubMed.
- J. Cristale, I. Oliveira Santos and E. Fagnani, Organophosphate esters by GC–MS: an optimized method for aquatic risk assessment, J. Sep. Sci., 2020, 43(4), 748–755 CrossRef CAS PubMed.
- L. Pang, H. Yang, Y. Wang, X. Luo, S. Liu and J. Xiao, Organophosphate flame retardants in total suspended particulates from an urban area of Zhengzhou, China: temporal variations, potential affecting factors, and health risk assessment, Ecotoxicol. Environ. Saf., 2019, 176, 204–210 CrossRef CAS PubMed.
-
R Core Team, R: A language and environment for statistical computing, R Foundation for Statistical Computing, Vienna, Austria, 2021, cited 2023 Jan 18, available from, https://www.R-project.org/ Search PubMed.
- Y. Chen, Y. Song, Y. J. Chen, Y. Zhang, R. Li and Y. Wang,
et al., Contamination profiles and potential health risks of organophosphate flame retardants in PM2.5 from Guangzhou and Taiyuan, China, Environ. Int., 2020, 134, 105343 CrossRef CAS PubMed.
-
USEPA, Exposure Factors Handbook: 2011 Edition, U.S. Environmental Protection Agency, Washington, DC, EPA/600/R-09/052F, 2011 Search PubMed.
- J. Cristale, T. G. A. Belé, S. Lacorte and M. R. R. de Marchi, Occurrence and human exposure to brominated and organophosphorus flame retardants via indoor dust in a Brazilian city, Environ. Pollut., 2018, 237, 695–703 CrossRef CAS PubMed.
- D. D. Alves, R. P. Riegel, C. R. Klauck, A. M. Ceratti, J. Hansen and L. M. Cansi,
et al., Source apportionment of metallic elements in urban atmospheric particulate matter and assessment of its water-soluble fraction toxicity, Environ. Sci. Pollut. Res., 2020, 27(11), 12202–12214 CrossRef CAS PubMed.
- M. E. G. Do Carmo, F. C. D. C. Kunizaki, N. L. d. S. Sousa and L. L. Romualdo, Caracterização e Avaliação da Toxicidade de MP 10 Presentes na Área Urbana de Catalão-GO Associados a Parâmetros Climatológicos, Revista Processos Químicos, 2020, 14(27), 37–48 CrossRef.
- D. C. Nogarotto, F. L. C. de Souza, F. N. D. Ribeiro and S. A. Pozza, Use of Trajectory Regression Analysis to Understand High-PM10 Episodes: a Case Study in Limeira, Brazil, Water, Air, Soil Pollut., 2021, 232(10), 431 CrossRef CAS.
- J. O. Okeme, T. F. M. Rodgers, L. M. Jantunen and M. L. Diamond, Examining the Gas-Particle Partitioning of Organophosphate Esters: How Reliable Are Air Measurements?, Environ. Sci. Technol., 2018, 52(23), 13834–13844 CrossRef CAS PubMed.
- A. E. Clark, S. Yoon, R. J. Sheesley and S. Usenko, Spatial and Temporal Distributions of Organophosphate Ester Concentrations from Atmospheric Particulate Matter Samples Collected across Houston, TX, Environ. Sci. Technol., 2017, 51(8), 4239–4247 CrossRef CAS PubMed.
- S. Azizi, M. H. Dehghani, K. Naddafi, R. Nabizadeh and M. Yunesian, Occurrence of organophosphorus esters in outdoor air fine particulate matter and comprehensive assessment of human exposure: a global systematic review, Environ. Pollut., 2023, 318, 120895 CrossRef CAS PubMed.
- A. Zhao, C. Wei, Y. Xin, X. Wang, Q. Zhu and J. Xie,
et al., Pollution Profiles, Influencing Factors, and Source Apportionment of Target and Suspect Organophosphate Esters in Ambient Air: A Case Study in a Typical City of Northern China, J. Hazard. Mater., 2022, 130373 Search PubMed.
- M. J. Fabiańska, B. Kozielska, J. Konieczyński and P. Bielaczyc, Occurrence of organic phosphates in particulate matter of the vehicle exhausts and outdoor environment – A case study, Environ. Pollut., 2019, 244, 351–360 CrossRef PubMed.
- Q. Li, M. Guo, H. Song, J. Cui, M. Zhan and Y. Zou,
et al., Size distribution and inhalation exposure of airborne particle-bound polybrominated diphenyl ethers, new brominated flame retardants, organophosphate esters, and chlorinated paraffins at urban open consumption place, Sci. Total Environ., 2021, 794, 148695 CrossRef CAS PubMed.
- J. H. Syed, M. Iqbal, K. Breivik, M. J. I. Chaudhry, M. Shahnawaz and Z. Abbas,
et al., Legacy and emerging flame retardants (FRs) in the urban atmosphere of Pakistan: diurnal variations, gas-particle partitioning and human health exposure, Sci. Total Environ., 2020, 743, 140874 CrossRef CAS PubMed.
- L. L. Romualdo, R. S. Santos, F. C. Lima, L. S. Andrade, I. M. Ferreira and S. A. Pozza, Environmental impact monitoring of a minero-chemical complex in Catalão urban area of PTS, PM10 and PM2.5 by EDX characterization, Chem. Eng. Trans., 2015, 43, 1909–1914 Search PubMed.
- W. Zhang, P. Wang, Y. Zhu, D. Wang, R. Yang and Y. Li,
et al., Occurrence and human exposure assessment of organophosphate esters in atmospheric PM2.5 in the Beijing-Tianjin-Hebei region, China, Ecotoxicol. Environ. Saf., 2020, 206, 111399 CrossRef CAS PubMed.
- R. Olivero-Verbel, T. Moreno, J. Fernández-Arribas, C. Reche, M. C. Minguillón and V. Martins,
et al., Organophosphate esters in airborne particles from subway stations, Sci. Total Environ., 2021, 769, 145105 CrossRef CAS PubMed.
- T. Wang, N. Ding, T. Wang, S. J. Chen, X. J. Luo and B. X. Mai, Organophosphorus esters (OPEs) in PM2.5 in urban and e-waste recycling regions in southern China: concentrations, sources, and emissions, Environ. Res., 2018, 167, 437–444 CrossRef CAS PubMed.
- Y. Sun, J. Q. Guo, L. Y. Liu, E. Sverko, Z. Zhang and C. G. Tian,
et al., Seasonal variation and influence factors of organophosphate esters in air particulate matter of a northeastern Chinese test home, Sci. Total Environ., 2020, 740, 140048 CrossRef CAS PubMed.
|
This journal is © The Royal Society of Chemistry 2023 |
Click here to see how this site uses Cookies. View our privacy policy here.