Towards decarbonization of shipping: direct emissions & life cycle impacts from a biofuel trial aboard an ocean-going dry bulk vessel†
Received
22nd September 2021
, Accepted 29th December 2021
First published on 12th January 2022
Abstract
On board emission measurements from a dry bulk vessel operating on an advanced biofuel, produced from used cooking oil (UCO), are reported for the first time, in an effort to assess potential benefits and impacts compared to conventional fossil fuels. Carbon dioxide (CO2) and nitrogen oxide (NOx) emission measurements were performed on a slow-speed, two-stroke marine diesel engine of a Kamsarmax vessel, while burning a 50
:
50 biofuel blend of UCO biodiesel and marine gas oil (MGO). The same gases were monitored, under similar conditions, while the vessel was burning solely low-sulfur MGO (LSMGO) allowing for relevant comparisons. Sulfur dioxide (SO2) emissions were also calculated for the tested fuels. Apart from comparing the biofuel blend with LSMGO in terms of direct emissions from combustion, indirect emissions associated with the extraction, production and transportation of both fuels were estimated based on recent literature. Life cycle emissions were also estimated for different scenarios involving conventional marine fuels for performing the same voyage. Marginal differences were observed regarding CO2 and NOx emissions of the tested fuels, while the SO2 emissions of the biofuel blend were about 50% lower compared to LSMGO. Although the biofuel blend generates combustion CO2 emissions very similar to those of conventional marine fuels, it can achieve up to 40% emissions reduction from a life cycle analysis (LCA) perspective. These results, combined with the fact that no operational issues occurred during the biofuel trial, show that such fuels have significant potential towards the decarbonization of dry bulk shipping.
Introduction
The shipping sector currently accounts for 3% of the annual global greenhouse gas (GHG) emissions,1 while generating about 2.3 million tons of sulfur dioxide (SO2) and 3.2 million tons of nitrogen oxides (NOx) per year.2 As the sector continues to grow and stricter environmental and climate change regulations are being enforced, ship owners are under significant pressure to reduce emissions. In 2018, the International Maritime Organization (IMO) introduced its initial GHG strategy, envisaging the reduction of carbon dioxide (CO2) emissions per transport work by at least 40% by 2030, while pursuing efforts towards 70% by 2050, compared to 2008 levels.3 Moreover, IMO's regulations, enforced from January 2020 onwards, are capping the global fuel sulfur content to 0.5 mass percent (% m/m) from 3.5% m/m. This requirement is in addition to the 0.1% m/m sulfur limit in the North American, US Caribbean, North Sea and Baltic Sea Sulfur Emission Control Areas (SECAs). Furthermore, a binding international agreement (the 2008 revision to the 1997 Annex VI of the International Convention for the Prevention of Pollution from Ships – MARPOL4), apart from the sulfur oxide (SOx) emissions, limits the particulate matter (PM) and NOx emissions of ships.5–7
A plethora of technical and operational options are being explored to improve propulsion efficiency and reduce shipping emissions. Biofuels produced using organic feedstocks11 are considered among the most viable options towards early decarbonization of shipping in the short-term future. They can be blended with conventional marine fuels and used in already existing vessels without – or with minor – modifications (drop-in fuels), taking advantage of the existing bunkering infrastructure.8–10
Biofuels are classified in four main generations based on the feedstock used for their production. First generation (1G) derives from food crops and has been widely used in the automotive sector. However, 1G sustainability is strongly debated, due to competition for land with food production. Huge land areas are needed to cover shipping demand (about 300 million tons annually), while the environmental performance of 1G biofuels is doubtful if life cycle emissions from land use changes (LUC) are considered.11,12 Second generation (2G) biofuels derive from non-food biomass, including lignocellulosic feedstock, wastes and residues. 2G biofuels have significant potential for shipping, as they avoid the food-vs.-fuel debate. They still face economic and technical challenges, however, it is expected that they will achieve a significant market penetration before 2030, as production technologies are becoming more mature.7,10,11,13 Third generation (3G) biofuels derive from algae, an abundant resource, which can theoretically achieve oil yields 10–100 times greater than those of energy crops. However, scalable, commercially viable 3G systems have not yet been deployed. Several technological barriers concerning their low energy efficiency need to be overcome to enable their viability.14–21 The fourth biofuel generation (4G) concerns the use of genetically modified microorganisms and crops as feedstocks. Such biofuels are currently in a research state and their scalability has yet to be showcased.
2G biofuels are currently the most attractive and readily available biofuel option for dry bulk shipping to achieve emission reduction targets.7,9,22–24 Indeed, bulk carriers have the greatest contribution to the total shipping CO2 emissions. They represent 21% of the global merchant fleet,25 and account for approximately 47% of the total annual shipping CO2 emissions, generating 440 million tons of CO2 per year.8
In an effort to assess the contribution of 2G biofuels to the reduction of bulk carriers' emissions, we conducted a biofuel trial aboard an ocean-going bulk vessel. On board measured emission values from a bulk carrier operating on biofuel have not been reported in the literature. We have tested a 50
:
50 blend (biofuel blend) of a commercially available 2G biofuel (GoodFuels MDF1-100) with marine gas oil (MGO) in a bulk carrier bunkered in Singapore. The advanced (2G) drop-in biofuel used was derived from used cooking oil (UCO). Minor modifications were carried out to burn the biofuel blend efficiently, and monitoring instruments were installed to capture the biofuel trial data as accurately as possible. The key objective of the trial was to explore the merits and challenges of biofuels compared to conventional marine fuels. We have monitored emissions from burning low-sulfur marine gas oil (LSMGO) in the same vessel to allow for relevant comparisons. CO2 and NOx emission measurements were performed using a specifically developed protocol, and SOx emissions were calculated, following the International Organization for Standardization (ISO) 8178 guidelines.26,27 Apart from measuring direct combustion emissions, indirect CO2 emissions associated with all the production steps of both fuels were also estimated to provide comparisons from a life cycle perspective.
Experimental
Vessel & engine description
The Kira Oldendorff (IMO no. 9867566) eco-Kamsarmax bulk carrier vessel was selected for this study, sailing under the flag of Liberia and built in 2020. It is a representative ocean-going bulk vessel, with a carrying capacity of 81
290 tons deadweight (DWT), length overall (LOA) of 229 meters and width of 32.26 meters. The vessel's average and maximum speeds are 10.5 and 14.7 knots respectively.
Kira Oldendorff vessel is fitted with a MAN B&W 6S60ME-C8.5 slow-speed, two-stroke diesel main engine, whose design and performance parameters comply with the IMO Tier II emission regulations for NOx emissions.28 The main engine has a nominal maximum continuous rating (nominal MCR) of 9932 kW at 90.2 rpm (100% engine power). The vessel also has three auxiliary YANMAR 6EY18ALW diesel generators of 800 kW rated output.
Tested fuels & voyages
The tested biofuel blend consisted of the GoodFuels MDF1-100 2G biofuel derived from UCO by 50% (98.75 tons of the 197.5 tons total; sufficient amount for about six days steaming) and by 50% MGO. The biofuel blend was bunkered in Singapore in April 2021. The vessel departed from Singapore and started burning the biofuel blend having as her destination the port of Las Palmas, Spain. During this voyage biofuel blend-related emission measurements were performed. The vessel arrived in Las Palmas in May 2021 and was bunkered with LSMGO. Then, she left from Las Palmas heading to Lulea, Sweden, while burning LSMGO. For comparison purposes, emission monitoring and analysis was also conducted during this voyage, while the vessel was burning solely LSMGO.
Samples from both the biodiesel blend and LSMGO were analyzed to determine their chemical composition and physical properties. The main properties of fuels are presented in Table 1 and they are also compared with MAN B&W specifications (main engine manufacturer).28 Results from the chemical testing of the fuels including the test methods followed for their analyses are provided in detail in the ESI (ESI Note 2, Fig. S13–S16†). The conducted emission sampling and analysis concerns the main engine of the vessel, while she was operating outside of SECAs (Tier II NOx limits apply).
Table 1 Chemical composition and physical properties of tested fuels
Fuel property |
Test method |
Engine specifications |
Biofuel blend |
LSMGO |
MARPOL standard.
|
Density at 15 °C (kg m−3) |
ISO 12185 |
≤1010.00 |
856.80 |
847.80 |
Kinematic viscosity at 50 °C (cSt) |
ASTM D7042 |
≤700.00 |
3.41 |
3.36 |
Net calorific value (MJ kg−1) |
ASTM D240 for biofuel blend; ISO 8217 for LSMGO |
>35.00 |
40.20 |
42.76 |
Flash point (°C) |
LP 1503 |
≥60.00 |
>70.0 |
>70.0 |
Pour point (°C) |
LP 1305 for biofuel blend; ISO 3016 for LSMGO |
≤30.00 |
−3.00 |
0.00 |
Sulfur content (%m/m) |
ISO 8754 |
<0.50a |
0.05 |
0.10 |
Cetane index |
ISO 4264 |
— |
54.00 |
52.00 |
Carbon content (%m/m) |
ASTM D5291 |
— |
83.00 |
87.00 |
Nitrogen content (%m/m) |
ASTM D5291 |
— |
<0.10 |
<0.10 |
FAME content (%v/v) |
ASTM D7371 for biofuel blend; EN 14078 for LSMGO |
— |
45.88 |
<0.10 |
Water content (%v/v) |
ASTM D6304-C |
≤0.50 |
0.06 |
<0.01 |
Ash content (%m/m) |
LP 2605 for biofuel blend; LP 1001 for LSMGO |
≤0.15 |
<0.01 |
<0.01 |
Aluminum + silicon (mg kg−1) |
LP 1105 for biofuel blend; IP 501 for LSMGO |
≤60.00 |
<3.00 |
<2.00 |
Vanadium content (mg kg−1) |
≤450.00 |
<1.00 |
<1.00 |
Phosphorus content (mg kg−1) |
≤15 |
2.00 |
<1.00 |
Sodium content (mg kg−1) |
≤15 |
<1.00 |
<1.00 |
Potassium content (mg kg−1) |
LP1105 for biofuel blend; LP1101 for LSMGO |
≤15 |
2.00 |
<1.00 |
Engine operating modes
An emissions measurement plan was developed considering the voyage and the engine operation conditions. Efforts were made to conduct the emissions measurements at engine loads as close as possible to those specified by the ISO 8178.27 Five representative main engine operating modes have been specified based on the ahead direction positions of the engine's telegraph, i.e., dead slow ahead (mode 1), slow ahead (mode 2), half ahead (mode 3), full ahead (mode 4), full navigation ahead (mode 5). During all five main engine operating modes the two auxiliary diesel generators were working, while the third one was in stand-by mode. For each tested fuel, emission measurements were performed for all five engine operating modes in ascending order. Weighting factors for each engine operating mode were established considering the weighting factors recommended by the ISO 8178 (ref. 27) for marine applications type E2, suggesting that engine operations in loads greater than 50% have a weighting factor of 85%. This is also suggested by similar literature sources.29 Navigation data, engine information (e.g., engine power, speed, fuel consumption, etc.) and environmental conditions were also monitored during each mode by the vessel's instrumentation. The five engine operating modes, as well as their respective considered weighting factors and recorded engine conditions for the different test fuels are presented in Table 2. For modes 2 and 4, two values are given for each engine condition in case of the biofuel blend. These values represent different emission samples taken under similar engine conditions while the biofuel blend was used. A detailed presentation of the recorded engine, navigation and environmental data for each engine operating mode is provided in the ESI of this work (ESI Note 1, Fig. S1–S12†).
Table 2 Engine operating modes and relevant engine conditions per tested fuel
|
Mode 1: dead slow ahead |
Mode 2: slow ahead |
Mode 3: half ahead |
Mode 4: full ahead |
Mode 5: full navigation ahead |
Different emission samples taken under similar conditions while burning the biofuel blend.
|
Weighting factor |
0.05 |
0.05 |
0.25 |
0.50 |
0.15 |
![[thin space (1/6-em)]](https://www.rsc.org/images/entities/char_2009.gif) |
Engine conditions while using the biofuel blend
|
Engine load (%) |
20 |
32 & 37a |
41 |
61 & 63a |
90 |
Engine rpm |
37 |
55 & 66 |
63 |
79 |
90 |
Power (kW h) |
1986 |
3178 & 3675 |
4072 |
6058 & 6257 |
8939 |
Consumed fuel (kg h−1) |
280 |
561 & 825 |
736 |
1179 & 1215 |
1780 |
Specific fuel oil consumption (g kW−1 h−1) |
141 |
177 & 224 |
181 |
195 & 194 |
199 |
![[thin space (1/6-em)]](https://www.rsc.org/images/entities/char_2009.gif) |
Engine conditions while using LSMGO
|
Engine load (%) |
23 |
37 |
44 |
67 |
90 |
Engine rpm |
36 |
55 |
63 |
79 |
87 |
Power (kW h) |
2284 |
3675 |
4370 |
6654 |
8939 |
Consumed fuel (kg h−1) |
304 |
587 |
762 |
1245 |
1703 |
Specific fuel oil consumption (g kW−1 h−1) |
133 |
160 |
174 |
187 |
191 |
On board emission measurement campaign
Concentrations of CO2 and NOx were measured using two commercially available portable flue gas analyzers, the Wöhler A 550 INDUSTRIAL30 and the TESTO 350.31 Both instruments were used to measure both gases for more accurate results. Instruments were calibrated according to their manufacturers specifications and tested on board before the emission measurement campaign. The accuracy of the instruments for the measured parameters is presented in Table 3.
Table 3 Accuracy of the portable flue gas analyzers used
Measured parameter |
Wöhler A 550 INDUSTRIAL (measurement range) |
TESTO 350 (measurement range) |
CO2 |
± 0.3 vol% (0–6 vol%) |
± 0.3 vol% (0–25 vol%) |
NOx |
±5% of reading (>100 ppm) |
±5% of reading (<100–1999 ppm) |
Two sampling points inside the vessel's funnel were used to take measurements from the raw exhaust stream, one below and one above the silencer. Gas samples were taken with both devices from both sampling points for each one of the five engine operating modes, following the gas analyzer manufacturers guidelines, while conforming to the sampling requirements of ISO 8178.26,27 For sampling point 1, the gaseous emissions sampling probes were fitted sufficiently close to combustion. Sampling point 2 was added for getting additional data and increase the validity of the measurements. Steel protection flanges were fabricated for each sampling point. The schematic diagram of the sampling setup is provided in Fig. 1.
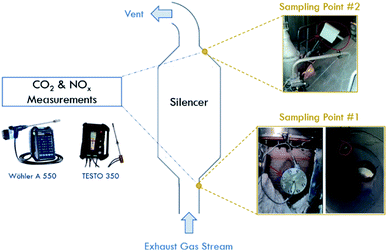 |
| Fig. 1 Schematic diagram of the onboard emission monitoring system. | |
For all engine operating modes, the main engine was first allowed to run for 30 minutes to reach steady-state conditions before taking measurements. The engine conditions were monitored during one hour of steady run for each mode, while measurements were taken. Biofuel blend measurements were taken on the 15th and the 17th of April 2021, while LSMGO measurements were taken on the 12th and the 13th of May 2021.
Calculation of emission factors (EFs)
Emissions were instantaneously measured in parts per million (ppm) for NOx and in percentage of gas volume (%v/v) for CO2. For each engine mode, measurements were taken in duplicates and the average of both measurements for both sampling points was used. After averaging, the instantaneous emissions for each monitored parameter were converted to grams of emissions per kilowatt hour (g kW−1 h−1) of the main engine to allow for comparisons. The exhaust gas flow rate was used for this conversion. Specific fuel consumption and emitted CO2 were used to calculate the exhaust gas flow rate, following the carbon balance method specified in ISO 8178 recommendations.27 This approach is commonly adopted in similar literature as well.32–35 Exhaust gas flow rate (Exh_flow in m3 h−1) was calculated based on the formed CO2, and assuming that all carbon in the fuels is converted completely into CO2 during combustion following eqn (1). | 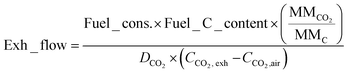 | (1) |
where Fuel_cons. is the fuel consumption (kg h−1); Fuel_C_content is the carbon content of the fuel (% m/m); MMCO2 is the molar mass of CO2 (g mol−1) and is equal to 44; MMC is the molar mass of carbon (g mol−1) and is equal to 12; DCO2 is the density of CO2 (kg m−3) and is equal to 1.96; CCO2,exh is the concentration of CO2 in the exhaust gas (%v/v); CCO2,air is the concentration of CO2 in the air (%v/v).
The emission factors of the monitored gases were then calculated in g kW−1 h−1 following eqn (2).
|  | (2) |
where EF
k are the emission factors for the
k monitored parameter (g kW
−1 h
−1); ER is the average of all the instantaneous measurements for
k monitored gas (ppm or %v/v; measured values are divided by 10
6 or 10
2 respectively);
P is the average pressure in standard conditions (101
![[thin space (1/6-em)]](https://www.rsc.org/images/entities/char_2009.gif)
325 N m
−2); Exh_flow is the volumetric flow rate of the exhaust calculated using
eqn (1) (m
3 h
−1); MM is the molar mass of the
k monitored parameter (g mol
−1);
R is the ideal gas constant (8.3145 J mol
−1 K
−1);
T is the average temperature in standard conditions (273.15 K); Engine_output is the average generated engine propulsion power (kW).
In order to calculate the weighted overall emissions, the results of eqn (2) for each monitored gas and engine type are multiplied with the respective weighting factor (Table 2) and summed together.
The SO2 emissions from each fuel were calculated based on their sulfur levels as per ISO 8178.26
Life cycle impact assessment
Based on the measured CO2 onboard emissions and on recent literature sources, the estimation of the overall CO2 emissions across the whole life cycle of the tested fuels was attempted (total GHG emissions in CO2 equivalent units would require the measurement of methane and nitrous oxide emissions as well as of particles like black carbon which has not been performed in this study). The overall weighted CO2 emissions of the tested fuels represent the direct CO2 emissions during fuel combustion, or the Tank-To-Wake (TTW) emissions of the fuels. To assess the life cycle impacts of the biofuel blend and the LSMGO, the indirect emissions of the fuels were estimated based on prior similar studies.36,37 These emissions concern all the processes involved in the extraction, production and transportation of the fuels until they were delivered into the vessel's fuel tanks and are also called Well-To-Tank (WTT) emissions.
The avoided emissions from the use of UCO in the biofuel blend were also considered, representing the emissions which would have been generated from the collection, treatment and disposal of the UCO if it has not been used as a biofuel feedstock.12 To calculate the total life cycle impacts, i.e., the Well-To-Wake (WTW) impacts, WTT and TTW were added, while subtracting any avoided emissions, as they have been reported in the literature.12,37 The description of the life cycle analysis (LCA) methods followed in the selected literature sources that were taken into account in the present study, including system boundaries, functional units, etc., are described in the ESI of this work (ESI Note 3, Fig. S17 and S18†).
Three different scenarios involving the use of different fuels for performing the same voyage were also assessed to compare conventional marine fuels to the biofuel blend from an LCA perspective. Scenario 1 is a typical or “business as usual” (BAU) scenario for bulk carriers, where only petroleum-based fuels would have been used to perform the voyage, i.e., MGO (0.5% S), LSMGO (0.1% S) and heavy fuel oil (HFO) in the following quantities: 25.7 tons, 180.4 tons and 2066.2 tons respectively. Scenario 2 represents the actual voyage as it happened and concerns the use of MGO (0.5% S), LSMGO (0.1% S), HFO, and the biofuel blend in the following quantities: 23.2 tons, 180.4 tons, 1868.4 tons and 197.5 tons respectively. Scenario 3 concerns the performance of the entire voyage using solely the biofuel blend, i.e., burning 2249.3 tons of the biofuel blend. The three scenarios are equivalent as the total energy generated for propulsion in all three cases is 92 TJ.
Results and discussion
CO2 emissions
The CO2 emissions per each engine mode for the biofuel blend and LSMGO are presented in Fig. 2a. As observed, in the first two operating modes, the CO2 emissions from the biofuel blend are slightly greater than those of LSMGO, by 0.4% and 5% respectively. In contrast, in the next three modes, where the engine operates in higher loads, the CO2 emissions of the biofuel blend are slightly lower than those of LSMGO, by 2% for modes 3 and 4 and by 1% for mode 5. An overall reduction in CO2 emissions of 1.2% was observed from the use of the biofuel blend versus LSMGO, as the overall weighted CO2 emissions of the biofuel blend were 571 g kW−1 h−1, while those of the LSMGO were 578 g kW−1 h−1 (Fig. 2f).
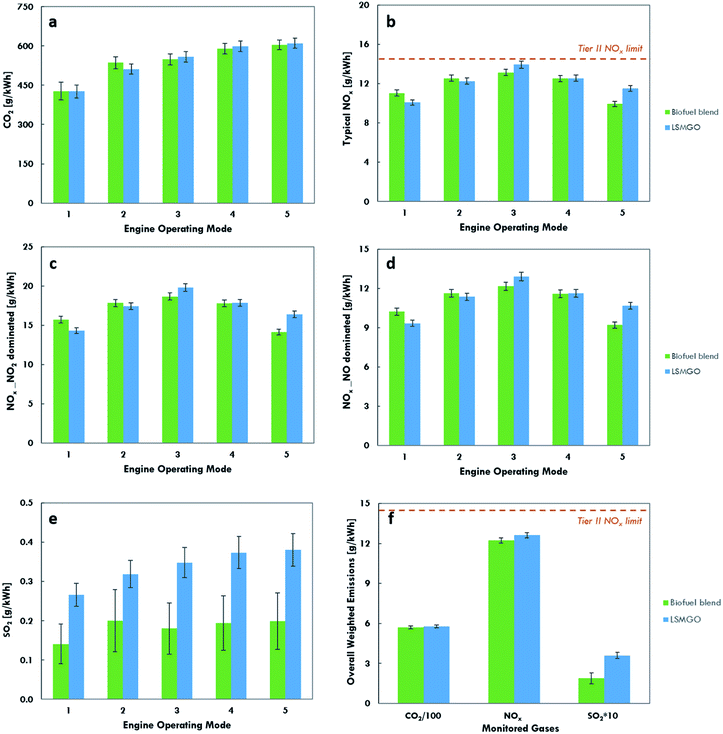 |
| Fig. 2 Emission factors of the tested fuels: (a) CO2 emissions per engine operating mode, (b) total NOx emissions per engine operating mode for a typical contribution of NO and NO2 emissions in total NOx emissions of 0.85 and 0.15 respectively; the orange dotted line represents the Tier II NOx emissions limit for bulk carriers operating with engine speed <130 rpm, i.e., 14.4 g kW−1 h−1, (c) extreme scenario of total NOx emissions being dominated by NO2 emissions per engine operating mode, (d) extreme scenario of total NOx emissions being dominated by NO emissions per engine operating mode, (e) calculated SO2 emissions per engine operating mode, and (f) overall weighted CO2, NOx and SO2 emissions; CO2 emissions are divided by 100 and SO2 emissions are multiplied by 10 to allow the presentation of the different emitted gases in the same plot; the orange dotted line represents the Tier II NOx emissions limit for bulk carriers operating with engine speed <130 rpm, i.e., 14.4 g kW−1 h−1. In all cases, green bars concern emissions related to the biofuel blend and blue bars concern emissions related to the LSMGO: Low Sulfur Marine Gas Oil. | |
CO2 emissions across all engine loads were typical of slow-speed two-stroke diesel engines.38 The slightly increased emissions of the biofuel blend compared to the LSMGO in the first two operating modes could be attributed to the fact that fuel combustion in diesel engines is inefficient in lower loads.39 In diesel engines, more than 99% of the fuel's carbon content is converted into CO2 during combustion.35 The carbon content of the biofuel blend was ∼5% lower than the carbon content of LSMGO, while the energy content of the LSMGO was 5% higher than the energy content of the biofuel blend (Table 1), leading to lower Specific Fuel Oil Consumption (SFOC) per engine mode (Table 2). Therefore, the expected theoretical reduction in CO2 emissions from the use of the biofuel blend was lower than 5%; the measured reduction of 1.2% is in the anticipated reduction range.
NOx emissions
NOx emissions are defined as the sum of nitrogen monoxide (NO) and nitrogen dioxide (NO2) emissions.39 NO and NO2 ratios in the total NOx emissions are expressed in the calculations through the relevant molar masses in eqn (2). As the exact NO and NO2 ratios in the exhaust gas emissions were not known in our case, we decided to follow a conservative approach so as to calculate the NOx emissions presented in Fig. 2b. NOx emitted from internal combustion engines are composed primarily of NO, with typical ratios of NO2 in total NOx between 0.05–0.10.39,40 The NO2 ratio in total NOx in turbocharged diesel engines (without aftertreatment) is typically higher, reaching up to 0.15.41 Hence, for the calculation of the total NOx emissions in this study, a 0.15 contribution of NO2 was considered. Two extreme scenarios of total NOx consisting completely of NO2 and of NO are presented in Fig. 2c and d respectively. The only difference between these two scenarios is the molar mass considered in the calculations, i.e., 46 for NO2 and 30 for NO. We expect the actual NOx emissions to be closer to Fig. 2d, but to be on the safer side the more conservative approach of 0.15 contribution of NO2 in total NOx was adopted (Fig. 2b).
Fig. 2b presents the NOx emissions associated with the biofuel blend and the LSMGO for the five engine operating modes. In the first and second engine operating modes the NOx emissions of the biofuel blend were 10% and 2% higher than those of LSMGO respectively. However, in the next three operating modes the NOx emissions from the biofuel blend were lower than those of LSMGO by 6%, 0.3% and 14% respectively. The overall weighted NOx emissions across all five operating modes of the biofuel blend were about 3% lower than those of the LSMGO (Fig. 2f). In both modal and overall weighted approaches, NOx emissions of the biofuel blend and the LSMGO are lower than the Tier II NOx limits for bulk carriers with maximum engine operating speed 130 rpm, i.e., 14.4 g kW−1 h−1.42
Lower engine loads can contribute to higher NOx emissions.43 This fact could explain the higher NOx emissions of the biofuel blend compared to LSMGO during the first two engine operating modes. The marginal difference observed in the overall NOx emissions of the two tested fuels could be explained if the fuels N content, density, and cetane index is considered (Table 1). Fuel N content and fuel density are corelated with NOx emissions.35,44 Both fuels have similar N content and densities which could explain the similar NOx emissions measured. The cetane index is another property that has been shown to impact NOx emissions.35 The biofuel blend has a slightly higher cetane index compared to the LSMGO, i.e., 54 versus 52 respectively. A higher cetane index correlates with a higher cetane number, which is a potential factor contributing to the slightly lower overall weighted NOx emissions of the biofuel blend. Higher cetane numbers increase the thermodynamic efficiency of the engine and minimize NOx emissions of the relevant fuels.10,35,45,46
Previous studies have also reported similar NOx emissions among biodiesels and low-sulfur distillate conventional marine fuels, however they were conducted in very different engines (marine diesel engines of ∼400 kW maximum power rating).47,48
SO2 emissions
Unlike the CO2 and NOx emissions, SO2 emissions were not measured but calculated based on the sulfur content of the considered fuels. The SO2 emissions associated with the biofuel blend and the LSMGO per engine operating mode are presented in Fig. 2e and are close to zero in all cases. The overall weighted SO2 emissions for both fuels are presented in Fig. 2f. As it is shown, both modal and overall SO2 emissions of the biofuel blend are about 50% lower than those of the LSMGO. This reduction was expected since the sulfur content of the biofuel blend is half that of LSMGO (0.05% m/m vs. 0.1% m/m respectively).
Comparison with prior emission measurement studies
To the best of our knowledge, there has been no other prior peer-reviewed study conducting emission monitoring and reporting measurements from an ocean-going vessel with a slow-speed, two-stroke main diesel engine, burning an advanced biofuel. However, numerous similar studies exist on emissions from slow speed, two-stroke engines burning distillate petroleum-based fuels29,49–52 and HFO.34,53 Emission measurements on biofuel combustion were only found in the literature for medium-speed, four-stroke diesel engines.35,54Fig. 3 shows the comparison among the overall weighted results from the current study with these literature sources and with a recent emission inventory from the port of Long Beach, CA,55 which is one of the busiest container ports in the United States. The overall weighted measured emissions of this study largely fall in the same range of values found in the literature, while any significant variations can be attributed to differences in the engine types or considered fuels.
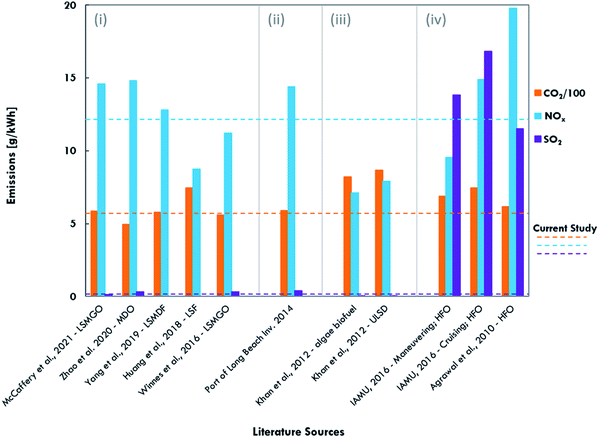 |
| Fig. 3 Comparing the emissions from the biofuel blend measured in this study (dashed lines) with prior studies and a recent emission inventory: (i) emissions from slow-speed, two-stroke diesel engines burning low-sulfur distillate petroleum-based fuels; (ii) emission inventory for vessels with slow-speed diesel engines in the port of Long Beach, CA, USA; (iii) emissions from a medium-speed, two-stroke diesel engine burning algae biofuel blend and ULSD; (iv) emissions from slow-speed, two-stroke diesel engines burning HFO. Orange color: CO2 emissions (divided by 100 for presentation purposes); light blue color: NOx emissions; dark blue color: SO2 emissions. LSMGO: Low Sulfur Marine Gas Oil; MDO: Marine Diesel Oil; LSMDF: Low Sulfur Marine Diesel Fuel; LSF: Low Sulfur Fuel; ULSD: Ultra Low Sulfur Diesel; HFO: Heavy Fuel Oil. | |
The biofuel blend generates either similar or from 7% up to 34% lower CO2 emissions compared to the considered sources. The only study that reports lower CO2 emissions than those of the biofuel blend, i.e., by ∼16%, concerns a 50% engine load.44
Regarding NOx emissions, the biofuel blend performs similarly or better (achieving up to 17% reductions) compared to studies performed in similar engine types (Fig. 3i). The two studies reporting lower NOx emissions by 40%29 and 9%,52 concern engine loads of up to 50%. A study35 on a 50
:
50 blend of ultra-low-sulfur diesel (ULSD) with algae biofuel reports 72% lower NOx emissions than the current work from the algae biofuel blend, and 55% lower NOx emissions from the ULSD alone (Fig. 3iii). This study however, concerns a medium-speed, four-stroke diesel engine of 600 kW maximum power rating at 1200 rpm. Studies on HFO emissions also report higher overall or cruising NOx values compared to the current study, while the lower NOx emissions associated with HFO concern a bulk carrier while maneuvering with engine load of around 35%34 (Fig. 3iv).
SO2 emissions are greatly dependent on the sulfur content of the tested fuels. HFO of about 3% sulfur content was used in the considered studies resulting in almost 100% higher SO2 emissions than those of the biofuel blend used in this work (Fig. 3iv). On the contrary, the algae biofuel blend and the ULSD fuels examined in a four-stroke diesel engine35 contained 0.0004% and 0.001% m/m sulfur respectively, resulting in extremely lower SO2 emissions than the current study (i.e., 0.003 and 0.007 g kW−1 h−1 respectively).
Life cycle CO2 impacts
The overall weighted CO2 emissions from the biofuel blend and the LSMGO combustion, 571 g CO2 per kW h and 578 g CO2 per kW h respectively, represent the TTW emissions of the fuels. Dividing with the SFOC for each fuel (weighted average for the five engine modes: 188 g fuel per kW h for the biofuel blend and 180 g fuel per kW h for the LSMGO), these emissions where converted to 3.037 g CO2 per g fuel for the biofuel blend, and 3.206 g CO2 per g fuel for the LSMGO. Dividing these values by the energy content of the fuels (Table 1), the TTW CO2 emissions of the fuels per MJ of energy entering the engine were calculated, i.e., 74 g CO2 per MJ for the biofuel blend and 75 g CO2 per MJ for the LSMGO, being completely aligned with existing literature.12,36,56 The estimation of the WTT CO2 emissions of the fuels, i.e., the CO2 emissions from all the steps involved in their extraction, production and transportation before their combustion, was based on relevant literature sources. According to a recent study,36 the WTT CO2 emissions of LSMGO are equal to 0.576 g CO2 per g fuel. Taking into account the amount of LSMGO used in this voyage and its energy content (Table 1), the WTT emissions of LSMGO were estimated to be around 13 g CO2 per MJ. According to an LCA37 of a second-generation biodiesel made from UCO using industrial-scale data, the WTT emissions of the UCO biodiesel are 14 g CO2 eq. per MJ. This value is in total agreement with the LCA results reported by the International Council on Clean Transportation (ICCT)12 about UCO biodiesel. Considering that the biofuel blend tested is a 50
:
50 blend of UCO biodiesel and MGO, and that CO2 emissions are at least 80% of the total GHG emissions (CO2 eq.) for these fuels, the WTT CO2 emissions of the biofuel blend were estimated to be about 12 g CO2 per MJ. The avoided emissions which would have been generated from the collection, treatment and disposal of the UCO if it had not been used as a feedstock for the biofuel blend, were also taken into account. Based on the ICCT,12 for each g CO2 per MJ emitted from the combustion of UCO biodiesel, 0.88 g CO2 per MJ are avoided. Following this approach 33 g CO2 per MJ were considered to have been avoided during the combustion of the biofuel blend in this study. Taking into account the WTT, TTW and avoided emissions, where applicable, the overall WTW CO2 emissions for the considered fuels were estimated (Fig. 4a).
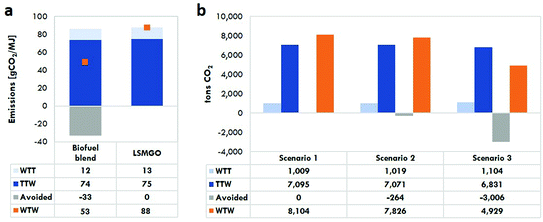 |
| Fig. 4 Results from comparing the fuels from an LCA perspective: (a) CO2 emissions across the whole life cycle of the biofuel blend (left bar) and the LSMGO (right bar) examined in this study, (b) CO2 tons emitted under the three different scenarios considered, involving the use of different fuels for performing the same voyage. WTT: Well-To-Tank; TTW: Tank-To-Wake; WTW: Well-To-Wake; Avoided: environmental benefits from avoiding the collection, treatment and disposal of the UCO waste stream, used partially as feedstock for the biofuel blend. | |
As it is shown, the WTW CO2 emissions of the biofuel blend are 40% lower than those of LSMGO. While there is no significant difference on the WTT and the TTW CO2 emissions of the two tested fuels, the avoided CO2 emissions in case of the biofuel blend, where a waste stream has been partially used as the fuel feedstock, greatly affect the overall WTW results. This can indicate that similar onboard emissions may differ substantially from an LCA perspective. However, the value reported herein on avoided emissions from UCO biodiesel cannot be generalized and is only used for discussion purposes in the current study. The environmental gains from avoiding the collection, treatment and disposal of UCO waste streams may differ significantly across various regions and techno-economic settings, affecting the final WTW results accordingly.
To further compare conventional marine fuels with the biofuel blend from an LCA standpoint, three alternative scenarios involving the use of different fuels for performing the same Kira Oldendorff's voyage were considered. As described in detail in the Experimental section, Scenario 1 is a BAU scenario for bulk carriers, concerning the operation of the vessel solely on conventional marine fuels, i.e., MGO, LSMGO and HFO. Scenario 2 represents the actual voyage as it happened combining conventional marine fuels with the biofuel blend for the purpose of the trial, and Scenario 3 concerns the performance of the entire voyage using only the biofuel blend. For the conventional marine fuels, the WTT and TTW CO2 emissions for slow-speed diesel engines reported by the ICCT36 were used. The WTT CO2 emissions of the biofuel blend were estimated on the basis of relevant literature sources.36,37 The TTW CO2 emission factors used for this comparison for the biofuel blend and LSMGO were calculated as described above and equal to 3.037 g CO2 per g fuel and 3.206 g CO2 per g fuel respectively. TTW CO2 emission factors of 3.114 g CO2 per g fuel and 3.206 g CO2 per g fuel were considered for HFO and MGO respectively.36 The avoided CO2 emissions in case of the biofuel blend were estimated based on ICCT's report,12 as explained above. The tons of CO2 emitted under each scenario are presented in Fig. 4b.
As shown, about 280 tons of CO2 were avoided from an LCA perspective by performing the biofuel trial during Kira Oldendorff's voyage (Scenario 2) compared to BAU (Scenario 1), while if the whole voyage had been performed on the biofuel blend (Scenario 3), about 3200 tons of CO2 would have been avoided. It can be observed that the transition from the complete use of fossil fuels to the use of the biofuel blend entails slightly higher WTT emissions, 1% increase compared to Scenario 2, and 9% increase compared to Scenario 3. This is due to the fact that HFO has about 25% lower WTT emissions compared to MGO,36 and in the BAU scenario, HFO is the most commonly used fuel for bulk carriers. On the other hand, there is a slight decrease in TTW CO2 emissions as we go from the complete use of conventional marine fuels to the use of the biofuel blend, i.e., 0.3% compared to Scenario 2, and 4% compared to Scenario 3. If we ignore the environmental benefits from the waste stream used to produce the biofuel blend, the WTW differences across the three scenarios are marginal. But if this amount is taken into account, the overall WTW CO2 emissions are reduced by about 3.5% in Scenario 2 and by about 40% in Scenario 3 compared to BAU.
These results suggest that a holistic approach is needed for a more complete comparison among fuels, taking into account the indirect impacts and benefits associated with a fuel, and looking beyond merely combustion emissions. Current IMO regulations focus only on TTW emissions. However, the adoption of a life cycle approach by policy makers would avoid creating perverse incentives and would encourage significantly the market penetration of environmentally sustainable marine fuels for the decarbonization of shipping. The International Civil Aviation Organization (ICAO) has already adopted such an approach, through the Sustainable Aviation Fuels framework under the Carbon Offsetting and Reduction Scheme for International Aviation,57 paving the way for adopting the LCA rationale in other transportation sectors.
Conclusions
This is the first study reporting on board emission measurements, under real conditions, on a large two-stroke marine diesel engine of a dry bulk carrier, operating on an advanced biofuel blend (50% UCO biodiesel and 50% MGO). The results of this challenging task are essential for informing outdated emission inventories and quantifying the impacts of emissions on climate change, and human health. The generated and avoided emissions from the use of the biofuel blend are demonstrated, both during combustion by performing on board emission measurements, and from an LCA perspective. The direct TTW emissions from burning the biofuel blend are similar to those of LSMGO, with only marginal reductions of 1.24% for CO2 and 3% for NOx. The only significant reductions observed (∼50%) concern the SO2 emissions, which are directly proportional to the sulfur content of the fuels. From an LCA standpoint, however, the use of the biofuel blend could result in about 40% reduction of the total WTW CO2 emissions compared to those of conventional marine petroleum-based fuels. This indicates that fuels generating similar onboard emissions may differ substantially from an LCA perspective, emphasizing the need for adopting a holistic regulatory framework for comparing marine fuels.
These results, combined with the fact that no operational issues occurred during the biofuel trial, show that such fuels can have significant potential towards the decarbonization of dry bulk shipping in the short-term future. Yet, onboard monitoring of additional pollutants, including CO and PM, and of additional engine parameters, such as intake air and fuel temperature and pressure, exhaust temperature, and instantaneous fuel flow, could provide more comprehensive future comparisons. In addition, the validation of industrial-scale data for the accurate assessment of the indirect WTT emissions and benefits of advanced biofuels is needed to further justify and eventually untap their potential. Furthermore, total GHG emissions across the fuels' life cycle, including methane, nitrous oxide, and black carbon emissions, shall be assessed to fully account for the contribution of maritime transport to climate change. Finally, long-term effects of biofuels' use and storage shall be explored to ensure proper engine operation and performance.
Author contributions
PMS, SB, CF and PJ designed the research work and the monitoring process. PMS analyzed emission data, conducted literature review and compared emissions of different fuels. CF contributed to data analysis. PJ, SB and MT provided technical inputs. NG was the overall supervisor of the project, coordinated collaboration and provided technical inputs. All authors contributed to manuscript preparation and writing.
Conflicts of interest
There are no conflicts to declare.
Acknowledgements
This study has been funded by Oldendorff Carriers GmbH & Co. KG via the proceeds of Oldendorff's multi-year research agreement with MIT CBA. The support of the global resources company BHP and the Maritime and Port Authority of Singapore for the conduction of the biofuel trial is gratefully acknowledged.
References
-
IMO: International Maritime Organization, Fourth IMO GHG Study 2020 Full Report, IMO, 4 Albert Embankment, London SE1 7SR, 2021 Search PubMed.
- P. Balcombe, J. Brierley, C. Lewis, L. Skatvedt, J. Speirs, A. Hawkes and I. Staffell, Energy Convers. Manage., 2019, 182, 72–88 CrossRef CAS.
-
IMO: International Maritime Organization, Initial IMO GHG Strategy, https://www.imo.org/en/MediaCentre/HotTopics/Pages/Reducing-greenhouse-gas-emissions-from-ships.aspx, accessed August 9, 2021 Search PubMed.
-
IMO: International Maritime Organization, Prevention of Air Pollution from Ships, https://www.imo.org/en/OurWork/Environment/Pages/Air-Pollution.aspx, accessed August 9, 2021 Search PubMed.
-
IMO: International Maritime Organization, IMO's work to cut GHG emissions from ships, https://www.imo.org/en/MediaCentre/HotTopics/Pages/Cutting-GHG-emissions.aspx, accessed August 9, 2021 Search PubMed.
-
MEPC: Marine Environment Protection Committee, Initial IMO Strategy on Reduction of GHG Emissions from Ships, IMO: International Maritime Organization, 2018 Search PubMed.
-
IRENA: International Renewable Energy Agency, Advanced Biofuels: What Holds Them Back?, IRENA, Abu Dhabi, 2019 Search PubMed.
-
Shell International B.V. and Deloitte, Decarbonising Shipping: ALL HANDS ON DECK, 2020 Search PubMed.
-
SSI: Sustainable Shipping Initiative, The Role of Sustainable Biofuels in the Decarbonisation of Shipping, SSI, 2019 Search PubMed.
- U. Kesieme, K. Pazouki, A. Murphy and A. Chrysanthou, Sustainable Energy Fuels, 2019, 3, 899–909 RSC.
-
IEA: International Energy Agency, Biofuels for the marine shipping sector: An overview and analysis of sector infrastructure, fuel technologies and regulations, IEA Bioenergy Task, 39, 2017 Search PubMed.
-
ICCT: International Council On Clean Transportation, The Potential of Liquid Biofuels in Reducing Ship Emissions, ICCT, 2020 Search PubMed.
-
ICCT: International Council On Clean Transportation, Assessing the Climate Mitigation Potential of Biofuels Derived from Residues and Wastes in the European Context, ICCT, 2014 Search PubMed.
-
IEA: International Energy Agency, State of Technology Review – Algae Bioenergy, IEA Bioenergy Task 39, 2017 Search PubMed.
- H. Chen, D. Zhou, G. Luo, S. Zhang and J. Chen, Renew. Sustain. Energy Rev., 2015, 47, 427–437 CrossRef CAS.
-
C. H. Tan, D. Nagarajan, P. L. Show and J.-S. Chang, in Biofuels: Alternative Feedstocks and Conversion Processes for the Production of Liquid and Gaseous Biofuels, Elsevier, 2019, pp. 601–628 Search PubMed.
- M. Musa, G. A. Ayoko, A. Ward, C. Rösch, R. J. Brown and T. J. Rainey, Cells, 2019, 8, 851 CrossRef CAS PubMed.
- P. Kenny and K. J. Flynn, J. Appl. Phycol., 2017, 29, 2713–2727 CrossRef CAS PubMed.
-
DOE: U.S. Department of Energy, National Algal Biofuels Technology Review, U.S. DOE, Office of Energy Efficiency and Renewable Energy, Bioenergy Technologies Office, 2016, p. 212 Search PubMed.
-
C. Lo, Algal biofuel: the long road to commercial viability, https://www.power-technology.com/features/algal-biofuels-challenges-opportunities/, accessed August 9, 2021 Search PubMed.
-
J. Winters, The Myth of Algae Biofuels, https://harvardpolitics.com/the-myth-of-algae-biofuels/, accessed August 9, 2021 Search PubMed.
-
SSI: Sustainable Shipping Initiative, Zero Emission Vessels, What Needs to Be Done?, SSI, 2018 Search PubMed.
-
SSI: Sustainable Shipping Initiative, Availability of Sustainable Biofuels: A Follow up to SSI's 2019 Inquiry into the Sustainability and Availability of Biofuels for Shipping, SSI, 2021 Search PubMed.
-
IEA: International Energy Agency, Technology Roadmap: Delivering Sustainable Bioenergy, IEA, 2017 Search PubMed.
-
Statista, Number of ships in the world merchant fleet as of January 1, 2020, by type, https://www.statista.com/statistics/264024/number-of-merchant-ships-worldwide-by-type/, accessed August 9, 2021 Search PubMed.
-
ISO: International Organization for Standardization, INTERNATIONAL STANDARD ISO 8178-2: Reciprocating Internal Combustion Engines: Exhaust Emission Measurement. Part 2: Measurement of Gaseous and Particulate Exhaust Emissions under Field Conditions, Geneva, Switzerland, 2008 Search PubMed.
-
ISO: International Organization for Standardization, INTERNATIONAL STANDARD ISO 8178-4: Reciprocating Internal Combustion Engines: Exhaust Emission Measurement. Part 4: Steady-State and Transient Test Cycles for Different Engine Applications, Geneva, Switzerland, 2020 Search PubMed.
-
MAN Diesel & Turbo, MAN B&W S60ME-C8.5-TII Project Guide: Electronically Controlled Two Stroke Engines, 2017 Search PubMed.
- C. Huang, Q. Hu, H. Wang, L. Qiao, S. Jing, H. Wang, M. Zhou, S. Zhu, Y. Ma, S. Lou, L. Li, S. Tao, Y. Li and D. Lou, Environ. Pollut., 2018, 242, 667–674 CrossRef CAS PubMed.
-
Wöhler, Wöhler A 550 INDUSTRIAL Flue Gas Analyzer, 2020 Search PubMed.
-
Testo, Testo 350 Flue Gas Analyzer: Instruction Manual, 2020 Search PubMed.
- T. Chu-Van, Z. Ristovski, A. M. Pourkhesalian, T. Rainey, V. Garaniya, R. Abbassi, R. Kimball, N. L. Cong, S. Jahangiri and R. J. Brown, Energy Rep., 2019, 5, 1390–1398 CrossRef.
- T. Chu-Van, Z. Ristovski, A. M. Pourkhesalian, T. Rainey, V. Garaniya, R. Abbassi, S. Jahangiri, H. Enshaei, U. S. Kam, R. Kimball and L. Yang, Environ. Pollut., 2018, 237, 832–841 CrossRef CAS.
-
IAMU: International Association of Maritime Universities, Development of a Methodology to Measure and Assess Ship Emissions, IAMU, Tokyo, Japan, 2016 Search PubMed.
- M. Y. Khan, R. L. Russell, W. A. Welch, D. R. Cocker and S. Ghosh, Energy Fuels, 2012, 26, 6137–6143 CrossRef CAS.
-
ICCT: International Council On Clean Transportation, Update: Accounting for Well-To-Wake Carbon Dioxide Equivalent Emissions in Maritime Transportation Climate Policies, ICCT, 2021 Search PubMed.
- S. Foteinis, E. Chatzisymeon, A. Litinas and T. Tsoutsos, Renewable Energy, 2020, 153, 588–600 CrossRef CAS.
- E. Lindstad, G. S. Eskeland, A. Rialland and A. Valland, Sustainability, 2020, 12, 8793 CrossRef CAS.
-
CIMAC, The International Council and on Combustion Engines, Guide to Diesel Exhaust Emissions Control of NOx, SOx, Particulates, Smoke and CO2: Seagoing Ships and Large Stationary Diesel Power Plants, CIMAC Working Group “Exhaust Emissions Control”, Frankfurt, Germany, 2008 Search PubMed.
- D. B. Olsen, M. Kohls and G. Arney, J. Air Waste Manage. Assoc., 2010, 60, 867–874 CrossRef CAS PubMed.
-
ECOpoint Inc., Diesel Gaseous Emissions, https://dieselnet.com/tech/emi_gas.php, accessed August 9, 2021 Search PubMed.
-
IMO: International Maritime Organization, Nitrogen Oxides (NOx) – Regulation 13, https://www.imo.org/en/OurWork/Environment/Pages/Nitrogen-oxides-(NOx)-%E2%80%93-Regulation-13.aspx, accessed August 9, 2021 Search PubMed.
- C.-W. Cheng, J. Hua and D.-S. Hwang, J. Air Waste Manage. Assoc., 2017, 67, 1146–1157 CrossRef CAS.
-
European Commission, Quantification of Emissions from Ships Associated with Ship Movements between Ports in the European Community, Final Report, Entec UK Limited, 2002 Search PubMed.
- F. Jiménez Espadafor, M. Torres García, J. Becerra Villanueva and J. Moreno Gutiérrez, Transp. Res. D, 2009, 14, 461–469 CrossRef.
- R. L. McCormick, M. S. Graboski, T. L. Alleman, A. M. Herring and K. S. Tyson, Environ. Sci. Technol., 2001, 35, 1742–1747 CrossRef CAS PubMed.
- V. Jayaram, H. Agrawal, W. A. Welch, J. W. Miller and D. R. Cocker III, Environ. Sci. Technol., 2011, 45, 2286–2292 CrossRef CAS PubMed.
- A. Petzold, P. Lauer, U. Fritsche, J. Hasselbach, M. Lichtenstern, H. Schlager and F. Fleischer, Environ. Sci. Technol., 2011, 45, 10394–10400 CrossRef CAS PubMed.
- C. McCaffery, H. Zhu, G. Karavalakis, T. D. Durbin, J. W. Miller and K. C. Johnson, Atmos. Environ., 2021, 245, 118023 CrossRef CAS.
- J. Zhao, Y. Zhang, Z. Yang, Y. Liu, S. Peng, N. Hong, J. Hu, T. Wang and H. Mao, Atmos. Environ., 2020, 223, 117286 CrossRef CAS.
- Z. Yang, Q. Tan and P. Geng, Pol. Marit. Res., 2019, 26, 153–161 CrossRef.
- H. Winnes, J. Moldanová, M. Anderson and E. Fridell, Proc. Inst. Mech. Eng. M, 2016, 230, 45–54 Search PubMed.
- H. Agrawal, W. A. Welch, S. Henningsen, J. W. Miller and D. R. Cocker, J. Geophys. Res., 2010, 115, D23205 CrossRef.
- S. J. Eaton, T. T. Wallace, B. G. Sarnacki, T. L. Adams, R. W. Kimball, J. A. Henry and G. N. Harakas, J. Mar. Eng. Technol., 2019, 18, 102–111 CrossRef.
-
ICF, Port of San Diego 2016 Maritime Air Emissions Inventory, Port of San Diego, San Diego, California, USA, 2018 Search PubMed.
- E. C. D. Tan, T. R. Hawkins, U. Lee, L. Tao, P. A. Meyer, M. Wang and T. Thompson, Environ. Sci. Technol., 2021, 55, 7561–7570 CrossRef CAS PubMed.
-
N. Rehmatulla, P. Piris-Cabezas, D. Baresic, M. Fricaudet, C. Raucci, M. Cabbia Hubatova, A. O'Leary, N. Stamatiou and A. Stratton, Exploring the Relevance of ICAO's Sustainable Aviation Fuels Framework for the IMO, Environmental Defense Fund, London, UK, 2020 Search PubMed.
Footnote |
† Electronic supplementary information (ESI) available. See DOI: 10.1039/d1se01495a |
|
This journal is © The Royal Society of Chemistry 2022 |