DOI:
10.1039/D2SC02654F
(Edge Article)
Chem. Sci., 2022,
13, 12818-12830
Amphiphilic stilbene derivatives attenuate the neurotoxicity of soluble Aβ42 oligomers by controlling their interactions with cell membranes†
Received
12th May 2022
, Accepted 19th October 2022
First published on 19th October 2022
Abstract
The misfolded proteins or polypeptides commonly observed in neurodegenerative diseases, including Alzheimer's disease (AD), are promising drug targets for developing therapeutic agents. To target the amyloid-β (Aβ) peptide plaques and oligomers, the hallmarks of AD, we have developed twelve amphiphilic small molecules with different hydrophobic and hydrophilic fragments. In vitro fluorescence binding assays demonstrate that these amphiphilic compounds show high binding affinity to both Aβ plaques and oligomers, and six of them exhibit selective binding toward Aβ oligomers. These amphiphilic compounds can also label the Aβ species in the brain sections of transgenic AD mice, as shown by immunostaining with an Aβ antibody. Molecular docking studies were performed to obtain structure–affinity relationships. To our delight, four amphiphilic compounds can alleviate the Cu2+–Aβ induced toxicity in cell viability assays. In addition, confocal fluorescence imaging studies provide evidence that two compounds, ZY-15-MT and ZY-15-OMe, can disrupt the interactions between Aβ oligomers and human neuroblastoma SH-SY5Y cell membranes. Overall, these studies strongly suggest that developing compounds with amphiphilic properties that target Aβ oligomers and modulate the Aβ oligomer–cell membrane interactions can be an effective strategy for the development of small molecule AD therapeutics.
Introduction
The aggregation of misfolded proteins is commonly observed in different neurodegenerative diseases, including the amyloid-β (Aβ) peptides and tau proteins in Alzheimer's disease (AD), or α-synuclein in Parkinson's disease.1–3 AD is the most prevalent neurodegenerative disease and affects more than 44 million people worldwide, yet there is still a lack of effective treatments.4,5 Although the insoluble amyloid plaques have been considered the main hallmark of AD over the past century, recently the soluble Aβ oligomers were found to be the most neurotoxic species that directly affect synapse loss and neuronal injury.6–9 Since Aβ oligomer species appear in the early stages of the disease and are the cause of continuous synaptic damage, they have become attractive targets for AD drug development.10,11 Therefore, numerous strategies have been developed to target soluble Aβ oligomers by using various antibodies,12,13 polypeptides,14,15 natural products,16–20 as well as small molecules. Small molecules utilized as therapeutic agents for central nervous system (CNS) disorders have advantages over biologics, including low molecular weight for higher blood–brain barrier (BBB) permeability and simpler structures for easy access at low cost.21 However, due to the lack of a molecular-level understanding of Aβ oligomers' structures, there is no effective way to design small molecules with high and selective Aβ oligomers binding affinity and to modulate their neurotoxicity.
With the aim of developing therapeutic agents to alleviate the neurotoxicity of Aβ oligomers, we report herein an effective strategy to develop twelve amphiphilic compounds with different amphiphilicity by linking various hydrophobic stilbene derivatives to the hydrophilic triazamacrocycle (Me2TACN, Fig. 1). The binding affinity of the amphiphilic compounds was measured by in vitro fluorescence saturation assays. These compounds show high binding affinities to both Aβ fibrils and oligomers in the low micromolar to high nanomolar range. More importantly, six of the compounds exhibit selective binding affinity toward Aβ oligomers. Molecular docking studies were performed to extract structure–affinity relationships need for the design of second-generation compounds. Immunostaining assays with an Aβ antibody HJ 3.4 confirms that our compounds are able to label ex vivo Aβ species in the brain sections of transgenic AD mice. Cell toxicity studies demonstrate that four compounds could rescue mouse neuroblastoma N2a cells from Cu2+–Aβ induced toxicity. Finally, the ZY-15-MT and ZY-15-OMe compounds were found to decrease the interaction between Aβ oligomers and human neuroblastoma SH-SY5Y cells as shown via confocal microscopy studies. Overall, these findings provide lead compounds for future optimization and demonstrate an effective strategy for small molecule development.
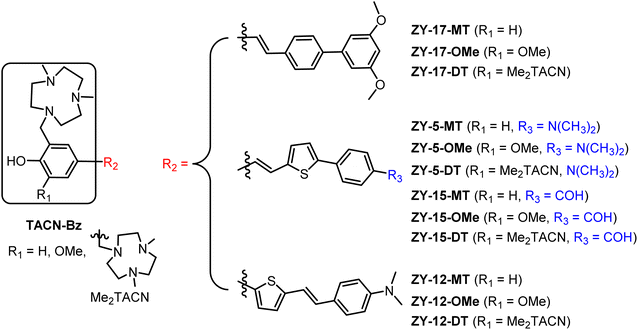 |
| Fig. 1 Molecular structures of the twelve amphiphilic compounds. | |
Results and discussion
Design and synthesis of the amphiphilic compounds
Inspired by the amphiphilic nature of the Aβ peptide, we proposed that developing compounds with amphiphilic properties targeting Aβ species could effectively treat AD. Recently, peptidomimetic-based amphiphilic compounds were shown to inhibit Aβ fibrillation process and attenuate Aβ cytotoxicity in both neuroblastoma N2a and human neuroblastoma SH-SY5Y cells.22 Moreover, our group successfully developed an amphiphilic small molecule, LS-4, that can serve as a therapeutic and imaging agent for Aβ oligomers in AD.23 LS-4 was synthesized by attaching a hydrophilic azamacrocycle, 2,4-dimethyl-1,4,7-triazacyclononane (Me2HTACN), to a hydrophobic distyryl stilbene derivative. Interestingly, because of the incorporation of the hydrophilic TACN fragment, the binding affinity of LS-4 toward both Aβ fibrils and oligomers increased dramatically vs. the Pre-LS-4 precursor, which does not contain the Me2TACN group. Although LS-4 showed high binding affinity to Aβ fibrils (Kd = 58 ± 15 nM) and Aβ oligomers (Kd = 50 ± 9 nM), there is no selectivity of LS-4 toward Aβ oligomers. Considering the higher neurotoxicity of Aβ oligomers and their appearance in the early stages of AD, it is beneficial to develop compounds with high affinity and selectivity toward Aβ oligomers. For this purpose, we designed a series of compounds with different amphiphilicity by adding different hydrophobic aromatic ring systems and changing the number of hydrophilic Me2TACN groups attached to the (hetero)aromatic conjugated fragments. More specifically, we designed the TACN-Bz component of the compounds with (1) a hydroxyl group with one Me2TACN (where R1 = H, yielding the ZY-#-MT series), (2) a methoxy group with one Me2TACN (where R1 = OMe, yielding the ZY-#-OMe series), and (3) two Me2TACN groups (where R1 = Me2TACN, giving ZY-#-DT series). In the ZY-#-OMe series, the methoxy group ortho to the hydroxyl group was introduced as its interaction with Aβ oligomers was reported previously.24 For the R2 component of the molecules, we incorporated different aromatic ring systems (thiophene–benzene or benzene–benzene) with different substituents for their potential hydrophobic π–π interactions with the Aβ species. Based on this design approach, twelve compounds were designed for structure–activity relationships (SAR) studies and further analysis.
These 12 compounds were synthesized following a streamlined synthetic process (Scheme 1).25 For ZY-5-OMe/MT/DT and ZY-17-OMe/MT/DT compounds (Scheme 1A and B), the Horner–Wadsworth–Emmons (HWE) olefination reaction was used to form (E)-selective olefins between the MOM-protected benzyl diethyl phosphonate and different aldehyde groups under basic conditions. The free hydroxyl group was obtained after MOM deprotection using HCl, followed by a Mannich reaction with paraformaldehyde and Me2HTACN to generate the corresponding final “OMe” or “MT” compounds.26,27 Interestingly, an additional Mannich reaction on the “MT” compounds can be performed to add a second Me2TACN group on the unsubstituted ortho position, using paraformaldehyde and Me2HTACN to obtain the “DT” compounds. For ZY-15-OMe/MT/DT (Scheme 1C), due to the presence of an aldehyde group on the R2 component of the compound, we modified the synthesis by first forming the double bond via the HWE olefination reaction, followed by the addition of the benzaldehyde by using a Suzuki coupling reaction. In order to study the effect of the position of the double bond relative to the heterocycles, we also synthesized ZY-12-OMe/MT/DT (Scheme 1D) with similar structures to ZY-5-OME/MT/DT except for the position of the double bonds. Due to the structural differences, the synthetic steps for ZY-12-OMe/MT/DT were modified accordingly. First, a Suzuki coupling was performed between MOM-protected boron pinacol esters and thiophene bromide, followed by the HWE olefination reaction to synthesize the second intermediate containing the double bond. The MOM deprotection and the Mannich reaction were performed to obtain the final “OMe” or “MT” compounds. Similar to the synthesis of other “DT” compounds, ZY-12-DT was synthesized through an additional Mannich reaction.
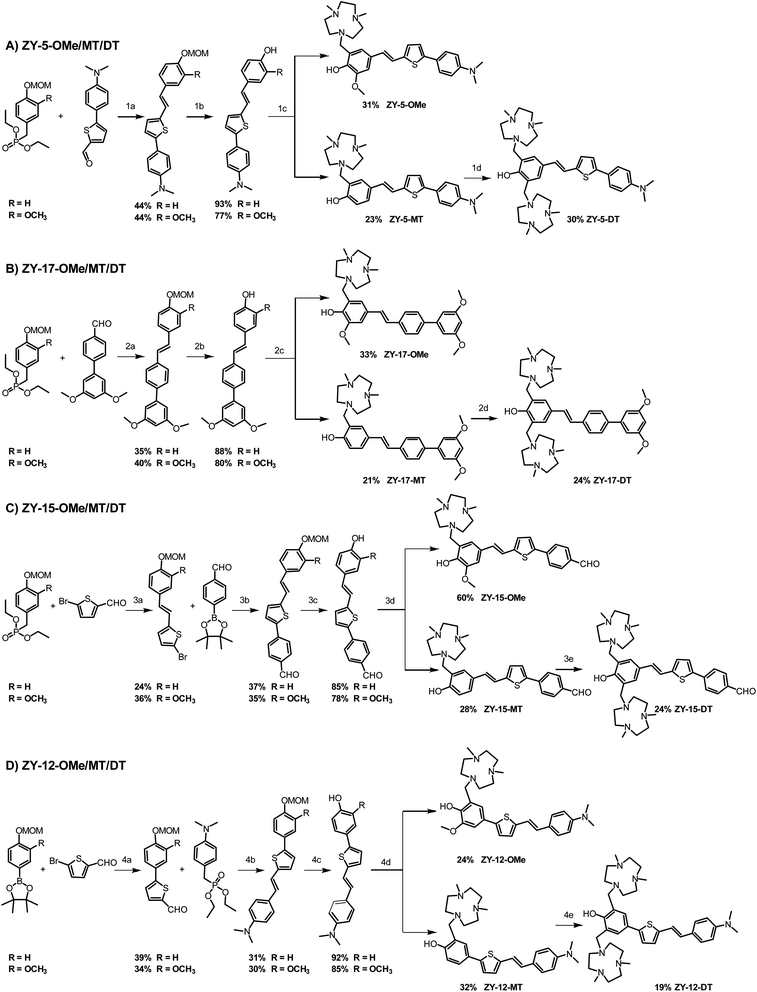 |
| Scheme 1 Synthetic route for the amphiphilic compounds. (A) Synthesis for ZY-5-OMe/MT/DT. (B) Synthesis for ZY-17-OMe/MT/DT. (C) Synthesis for ZY-15-OMe/MT/DT. (D) Synthesis for ZY-12-OMe/MT/DT. Reagents and conditions: (1a) KOtBu, DMF, rt, overnight; (1b) HCl, CH2Cl2, MeOH, rt, 12 h; (1c) (CH2O)n, Me2HTACN, MeCN, reflux, 16 h; (1d) (CH2O)n, Me2HTACN, MeCN, reflux, 24 h; (2a) NaOMe, DMF, rt; (2b) HCl, CH2Cl2, MeOH, rt, overnight; (2c) (CH2O)n, Me2HTACN, MeCN, reflux; (2d) (CH2O)n, Me2HTACN, MeCN, reflux, 24 h; (3a) NaOMe, DMF, r, 24 h; (3b) Pd(PPh3)4, K2CO3, toluene, ethanol, reflux; (3c) HCl, CH2Cl2, MeOH, rt, 12 h; (3d) (CH2O)n, Me2HTACN, MeCN, reflux, overnight; (3e) (CH2O)n, Me2HTACN, MeCN, reflux 24 h; (4a) Pd(PPh3)4, K2CO3, toluene, ethanol, reflux, 6 h; (4b) HCl, CH2Cl2, MeOH, rt, overnight; (4c) (CH2O)n, Me2HTACN, MeCN, reflux, 16 h; (4d) (CH2O)n, Me2HTACN, MeCN, reflux, 24 h. | |
Fluorescence binding assays
To investigate whether these amphiphilic compounds can interact with different Aβ species, we recorded the fluorescence intensity changes in the absence and presence of Aβ42 fibrils and oligomers, respectively, which were prepared as previously reported.28 These compounds, such as the ZY-5 series exhibit a fluorescence turn-on effect when binding to Aβ species, along with a dramatic blue shift of the emission wavelength (Fig. 2 and S3†). The enhanced fluorescence intensity and blue shift are possibly due to the restriction in a rotation of the aromatic rings and the changes of hydrophobicity at the Aβ binding sites. In addition, the compounds also showed excellent selectivity over human serum albumin (HSA), and most compounds showed minimal enhanced fluorescence intensity in the presence of HSA (Fig. S4†). Surprisingly, the ZY-17 series of compounds had a significant turn-on effect towards HSA. The compounds ZY-17-OMe and ZY-17-DT showed an even higher fluorescence intensity increase in the presence of HSA than for Aβ42 fibrils (Fig. S4†). Comparing the ZY-17 series with the other series of compounds, we believe that the thiophene ring in the stilbene-like binding scaffold is essential for the selectively toward Aβ species, while the more hydrophobic tri-benzene rings might lead to unspecific binding to other proteins; such a design principle will be key for the development of second-generation compounds with increased Aβ-binding selectivity.
 |
| Fig. 2 Fluorescence turn-on effects with Aβ42 oligomers and fibrils. Left ZY-5-OMe, middle ZY-5-MT right ZY-5-DT. Black: compound only; Blue: compound + Aβ42 oligomers; Red: compound + Aβ42 fibrils; [compound] = 5 μM; [Aβ42 oligomers] = 25 μM; [Aβ42 fibrils] = 25 μM. | |
Inspired by the observed fluorescence turn-on effect, we then measured the binding constants (Kd values) toward Aβ42 fibrils and oligomers using fluorescence saturation assays. One site-specific binding model was used for all the compounds to rank the binding affinities, while there is the potential that some compounds might have multiple binding sites. Representative Kd curves and Kd values obtained for the ZY-5 series are shown in Fig. 3 (for other compounds, see Fig. S5 and S6†). Kd values for all twelve compounds provide a direct comparison of their interaction with fibrils and oligomers (Table 1). Even though the Kd values are tentative, by performing the same type of fluorescence titration assays, the affinity ranking orders can be used to select oligomer-specific compounds for further studies. Thioflavin T (ThT) was shown to have a binding ratio of 30 to 40 (i.e. 1 ligand per 35 Aβ42 monomers), while the binding ratios for our amphiphilic compounds are mostly between 2 and 10 (i.e. 1 ligand per 5 Aβ42 monomers, Table S2†), which is similar to some halogenated benzothiazole or benzofuran derivatives, such as TZDM, TZPI, and BF1.29 The different binding ratios suggest that these compounds would bind to a different but a higher density binding site vs. ThT. For diagnostic purposes, targeting the higher density binding site may help to generate enhanced in vivo signals from imaging agents bound to Aβ42 aggregates.29 Considering the difference between the soluble Aβ42 oligomers and the insoluble Aβ42 fibrils, we consider the extra hydrophilic interactions between the amphiphilic compounds and Aβ aggregates, such as hydrogen bonding and cation–π interactions, would contribute to targeting the higher density binding site, which would be even more beneficial for oligomer binding and in vivo targeting.29 It is worth noting that some compounds have high binding affinity to Aβ42 oligomers evidenced by the low Kd values (e.g., ZY-17-OMe: Kd = 0.12 μM, ZY-12-OMe: Kd = 0.32 μM). These values are comparable to the Kd values of other reported oligomer-selective probes: CRANAD-102 (7.5 ± 10 nM),30 BD-Oligo (Kd = 0.48 μM),31 and F-SLOH (Kd = 0.66 μM).32 More interestingly, six of the compounds (ZY-5-MT, ZY-12-OMe, ZY-12-DT, ZY-15-OMe, ZY-17-OMe, and ZY-17-DT) showed relatively lower Kd values for Aβ oligomers than Aβ fibrils, indicating their higher binding affinity to oligomers over fibrils. Except for ZY-5-OMe, the other compounds bearing methoxy groups ZY-12-OMe, ZY-15-OMe, and ZY-17-OMe showed higher affinity toward Aβ oligomer suggesting that the methoxy group might increase the compounds' interactions with Aβ oligomers. Moreover, the ZY-5 and ZY-12 series bind to Aβ species differently despite their similar chemical structures. More specifically, ZY-5-MT showed a high affinity to both fibrils and oligomers, with an increased selectivity to Aβ oligomers (of 3.9 times higher than that for Aβ fibrils). In contrast, ZY-12-MT exhibited low affinity to both fibrils and oligomers. Taken together, we consider the different structures developed along with the Kd values will be beneficial for developing more oligomer-specific compounds.
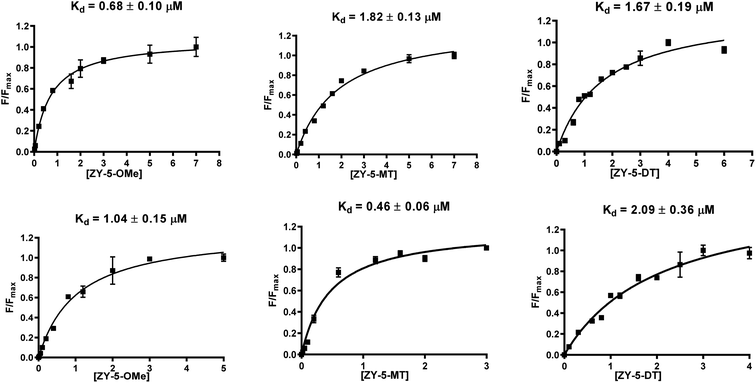 |
| Fig. 3 Binding constant measurements of ZY-5-OMe (left), ZY-5-MT (middle), ZY-5-DT (right) with Aβ42 fibrils (top), and oligomers (bottom). The measurements were performed in 10 mM phosphate buffered saline (PBS), pH 7.4. The Kd curves were fitted in GraphPad Prism with one site-specific binding model. Equation: Y = Bmax × X/(Kd + X). [Aβ42 fibrils] = [Aβ42 oligomers] = 5 μM. | |
Table 1 Summary of Kd values of amphiphilic compounds binding to Aβ42 fibrils and oligomers
Compounds |
Fibrils (μM) |
Oligomers (μM) |
ZY-5-OMe |
0.68 |
1.04 |
ZY-5-MT |
1.82 |
0.46 |
ZY-5-DT |
1.67 |
2.09 |
ZY-12-OMe |
0.39 |
0.32 |
ZY-12-MT |
4.19 |
6.27 |
ZY-12-DT |
1.38 |
0.67 |
ZY-15-OMe |
1.24 |
0.47 |
ZY-15-MT |
0.53 |
2.03 |
ZY-15-DT |
1.27 |
4.55 |
ZY-17-OMe |
0.18 |
0.12 |
ZY-17-MT |
0.94 |
2.08 |
ZY-17-DT |
2.25 |
1.03 |
Fluorescence imaging of 5xFAD mouse brain sections
In order to confirm that the compounds can also bind to native Aβ species, brain sections collected from 9-month-old 5xFAD transgenic mice have been employed in the fluorescence imaging studies. 5xFAD transgenic mice were shown to develop AD pathologies at a young age, and aggregated Aβ species were commonly observed in brain sections from 5xFAD transgenic mice.33 The brain sections were first incubated with our compounds, followed by Congo Red (CR), which is a well-established fluorescent probe that can bind to Aβ plaques.34 The treated brain sections were then imaged using fluorescence microscope. The six compounds with high binding affinities for Aβ42 oligomers based on Kd measurements showed well-defined fluorescence staining signals and good colocalization with Congo Red (CR), as indicated by the Pearson's correlation coefficients (Fig. 4 and S7†). Interestingly, these compounds tend to bind preferentially to the periphery of the amyloid plagues, while CR binds to the dense core region of the plaques. Immunostaining with the CF594-conjugated HJ 3.4 antibody (CF594-HJ3.4) was also performed to confirm that these six compounds can bind to Aβ species specifically in the brain sections (Fig. 5). Moreover, the log
D values of the compounds were also measured by octanol-PBS partition assays.35 These twelve compounds exhibit similar log
D values ranging from 0.8 to 1.2 (Table S3†), indicating their ability to cross the blood–brain barrier (BBB) for potential in vivo applications.36,37
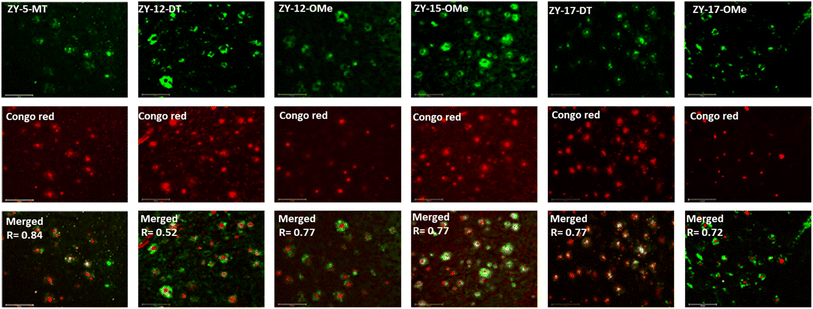 |
| Fig. 4 Fluorescence microscopy images of 5xFAD mice brain sections co-incubated with amphiphilic compounds (top), Congo red (middle) and merged images (bottom, along with the Parson's correlation coefficients R). Concentrations: [amphiphilic compounds] = 5 μM, [Congo red] = 2.5 μM; scale bar: 125 μm. | |
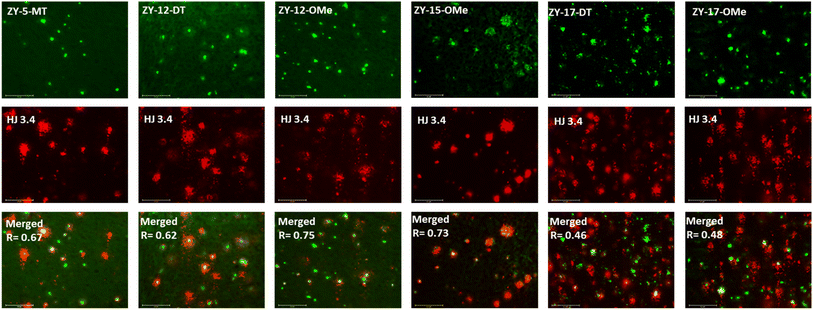 |
| Fig. 5 Fluorescence microscopy images of 5xFAD mice brain sections co-incubated with amphiphilic compounds (top), HJ 3.4 (middle) and merged images (bottom, along with the Pearson's correlation coefficients R). Concentrations: [amphiphilic compounds] = 5 μM, [CF594-HJ 3.4]: 1 μg mL−1; scale bar: 125 μm. | |
Molecular docking studies
To better understand at a molecular level why some compounds showed a higher binding affinity to Aβ42 oligomers than fibrils, a series of docking studies were performed using the Schrödinger program Glide.38 Schröder and coworkers reported a Aβ42 fibrils structure (PDB ID: 5OQV) obtained by cryo-electron microscopy in 2017, and the structure had been widely used as a molecular docking model to study the binding of small molecules to Aβ42 fibrils.39–41 The structures of Aβ42 oligomers are less defined due to their heterogeneous and aggregation-prone nature. Recently, Aβ42 tetramers (PDB ID: 6RHY) in membrane-mimicking conditions were prepared and reported based on NMR spectroscopy and mass spectrometry by Carulla and coworkers.42 According to their molecular dynamic studies, the tetramer dimerizes and forms pore-like structures within the membranes. This structure could be a critical model for understanding how the compounds disrupt the interactions between the toxic oligomers and cell membranes. In our docking studies, compounds ZY-5-MT, ZY-12-DT, ZY-15-OMe were investigated due to their higher binding affinity towards Aβ oligomers vs. Aβ fibrils. Even though ZY-17-DT and ZY-17-OMe also showed higher affinities towards Aβ oligomers, they were not chosen due to their decreased selectivity towards HSA. The docking scores and glide e-model energies were summarized in Tables S4 and S5.† When compounds ZY-5-MT, ZY-15-OMe, and ZY-12-DT were docked onto the structure of Aβ42 fibrils' structure, neither hydrogen bond interactions nor π–π interactions were observed. Instead, these compounds bind to the Aβ42 fibrils by inserting into the pocket formed by the residues KLVFF and residues NKGAI (Fig. 6a–c), similar to ThT – a well-known compound used for Aβ fibril binding.43,44 Even though the binding ratio determination indicates that our compounds and ThT would bind to different sites, it was demonstrated that the two binding sites are spatially close to each other via fluorescent resonance energy transfer (FRET) measurements.29 Therefore, we consider that the stilbene-like fragments of the compounds bind similar to ThT, and mainly interacting with the β-sheet structures. In addition, the TACN azamacrocycle would reach out and interact with the polar side chains, and the combination of both hydrophobic and hydrophilic fragments should lead to slightly different binding pockets and different binding ratios.
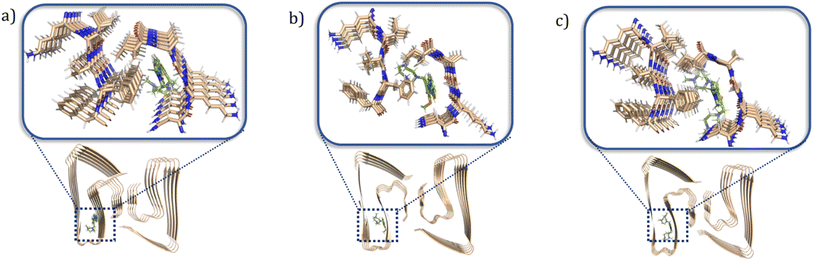 |
| Fig. 6 Calculated binding modes of amphiphilic compounds to Aβ42 fibrillar structure (5OQV). (a) ZY-5-MT (b) ZY-15-OMe (c) ZY-12-DT. | |
When considering the docking results with the Aβ42 tetramers, the compounds exhibit more interactions with these oligomeric structures. ZY-5-MT interacts with His6 and Asp7 via hydrogen bonds and salt bridges (Fig. 7a). On the other hand, ZY-12-DT interacts with Phe4 and Asp7 instead of His6 and Asp7 (Fig. 7b). ZY-15-OMe binds to another amino acid – Tyr10 – through hydrogen bonds and π–π interactions (Fig. 7c). Since the compound ZY-5-MT (with the highest selectivity for Aβ oligomers) shows interactions with amino acids His6 and Asp7, we posited that targeting these two residues would increase the binding affinity and selectivity to Aβ oligomers.
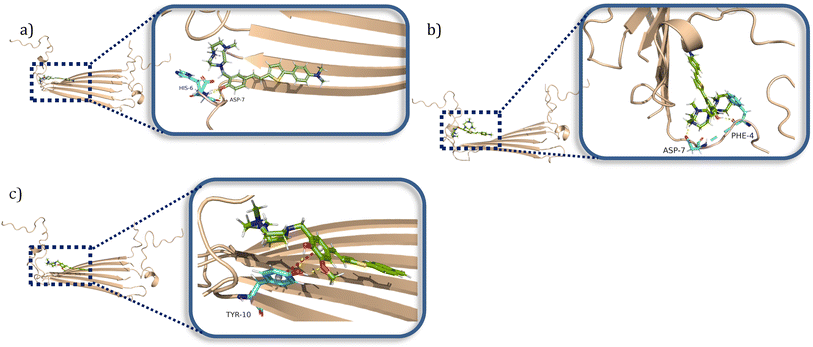 |
| Fig. 7 Calculated binding modes of amphiphilic compounds to Aβ42 tetramer structure (6RHY). (a) ZY-5-MT (b) ZY-12-DT (c) ZY-15-OMe. | |
Modulation of Cu2+–Aβ42 neurotoxicity
After confirming these amphiphilic compounds can bind to Aβ species both in vitro and ex vivo, we next investigated whether these compounds could attenuate the toxicity of the Cu–Aβ species, as the Cu2+ ions were reported to promote the formation of neurotoxic soluble Aβ42 oligomers.45,46 Firstly, the Alamar Blue cell viability assay was used to measure the cytotoxicity of the compounds at different concentrations ranging from 20 μM to 2 μM in mouse neuroblastoma N2a cells (Fig. 8). Some compounds (ZY-12-MT, ZY-15-MT, ZY-15-OMe, ZY-17-MT, ZY-5-MT, ZY-5-DT, and ZY-5-OMe) exhibited no significant cytotoxicity (indicated by >80% cell viability) up to 10 μM. Hence, these compounds are good candidates for the Cu2+–Aβ42-induced cytotoxicity studies (see below). For ZY-12-OMe, the compound was quite toxic even at 10 μM (cell viability less than 50%), yet at 5 μM it exhibited less cytotoxicity (more than 75% cell viability). Considering its high binding affinity to both Aβ fibrils and oligomers, we also further tested its ability to alleviate Cu2+–Aβ42-induced toxicity. Unfortunately, some of the compounds showed a high binding affinity to oligomers, such as ZY-12-OMe, ZY-12-DT, ZY-17-DT, and ZY-17-OMe, exhibited higher cytotoxicity than others. Additionally, ZY-17-OMe, which has the highest binding affinity to oligomers, showed the highest toxicity. The underlying mechanism is unclear, but we propose that these compounds might perform similarly to the toxic oligomers that bind to some cell membrane receptors or insert into the membrane lipids to form porous channels.
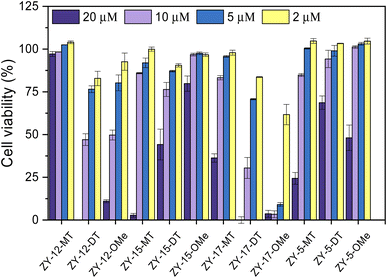 |
| Fig. 8 Toxicity of the amphiphilic compounds at different concentrations in mouse neuroblastoma Neuro2A (N2a) cells. | |
Since our compounds exhibit high binding affinity to both Aβ fibrils and oligomers and contain TACN group(s) that can potentially bind to Cu and disrupt the Cu–Aβ42 interaction,47 it is essential to study their roles in alleviating Cu2+–Aβ42-induced toxicity. As mentioned above, compounds that are not cytotoxic at 10 μM, including ZY-12-MT, ZY-15-MT, ZY-15-OMe, ZY-17-MT, ZY-5-MT, ZY-5-DT, ZY-5-OMe, and ZY-12-OMe, were chosen for this study. Firstly, during the control studies, we observed that monomeric Aβ42 led to negligible neurotoxicity. Nevertheless, in the presence of both Cu2+ and monomeric Aβ42, there was a significant cell death, which is likely due to the Cu2+ associated neurotoxic Aβ42 oligomers formation.46 We observed compounds ZY-12-MT, ZY-15-OMe, ZY-15-MT, and ZY-5-OMe could significantly increase cell viability, while the other ones could not reduce the neurotoxicity of the Cu2+–Aβ42 species (Fig. 9). Furthermore, the Cu-chelating fragments Me2HTACN or Me3TACN were not able to alleviate the neurotoxicity of the Cu2+–Aβ42 species (Fig. S8†), confirming the neuroprotective effect of the developed amphiphilic compounds is not solely due to the copper chelation ability and that the hydrophobic fragment also plays an essential role. Interestingly, when compared to ZY-5-MT, even though ZY-15-OMe is less selective toward Aβ oligomers, it can alleviate Cu2+–Aβ42-induced toxicity, likely due to its interaction with Tyr10 via hydrogen bond and π–π interactions. According to the docking results, ZY-12-MT, ZY-15-OMe, ZY-15-MT, and ZY-5-OMe interact with Aβ42 tetramers mainly through residues His6, Asp7, Tyr10 (via hydrogen bonds), and Tyr10 (via π–π interactions). Some reports have also shown that His6, Asp7, and Tyr10 are potentially involved in the Cu–Aβ interactions, which explains why the compounds are able to attenuate the Cu–Aβ toxicity.48
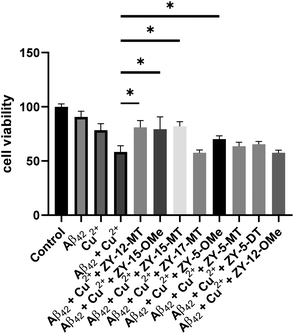 |
| Fig. 9 Cell viability results upon incubation of Neuro2A cells with monomeric Aβ42 in the presence or absence of metal ions and amphiphilic compounds. Concentrations: [Aβ42] = 40 μM, [Cu2+] = 40 μM, [ZY-12-MT] = [ZY-15-OMe] = [ZY-15-MT] = [ZY-17-MT] = [ZY-5-OMe] = [ZY-5-MT] = [ZY-5-DT] = 10 μM, [ZY-12-OMe] = 5 μM. The error bars represent the standard deviation from five independent experiments, and the statistical analysis was evaluated according to one-way ANOVA (*p < 0.05). | |
Modulating the Aβ–cell membrane interactions
Encouraged by the ability of the developed amphiphilic compounds to attenuate the neurotoxicity of Cu–Aβ species, we sought out to study the possible molecular mechanisms for this beneficial effect. The Aβ oligomers were reported to interact with cell membranes in various ways, such as binding to receptors on cell membranes,49,50 inserting into membranes,51 or even showing cellular uptake via endocytosis.52 The abnormal interactions between Aβ oligomers and neuron cells, which could disrupt the neuronal ion homeostasis and neuron cell membrane integrity, might be why Aβ oligomers are highly neurotoxic.53–55 Moreover, molecules that can disrupt interactions between oligomers and cell membranes are promising candidates for drug development.56,57 Thus, we proceeded to probe the interactions of the Aβ42 oligomers with SH-SY5Y cellular membranes, in the absence and presence of the compounds, via confocal microscopy. While all four compounds, ZY-12-MT, ZY-15-OMe, ZY-15-MT, and ZY-5-OMe, are not toxic towards SH-SY5Y cells (Fig. S9a†), ZY-15-MT and ZY-15-OMe were chosen for the cell imaging experiments, since they can rescue cell viability to a higher extent, and also exhibit a higher affinity for Aβ42 oligomers. Moreover, a lower concentration of Aβ42 (5 μM) or Aβ42–Cu (5 μM) were chosen to avoid significant cell death (Fig. S9b†). Before investigating the neuroprotective effect of our compounds, we have also confirmed that Aβ aggregates interact tightly with the cell membranes, as shown via co-staining with a membrane specific dye (Fig. S10†). Subsequently, SH-SY5Y cells were treated with Aβ oligomers or a combination of Aβ oligomers and compounds for 24 h, followed by the immunofluorescence staining with the CF594-labeled anti-Aβ antibody HJ 3.4 (Fig. 10) and nuclei staining, shown in the red and blue channels, respectively. Compared to the untreated group, the Aβ oligomers were found mainly bound to cell membranes. Moreover, in the presence of both ZY-15-MT and ZY-15-OMe, there were fewer numbers of Aβ oligomers bound to the cell membranes, as shown in the red channel (Fig. 10a). Interesting, ZY-15-MT is able to decrease the numbers of the Aβ oligomers binding to the cell membranes to a larger extent (about 50%, Fig. 10b), even though ZY-15-MT shows a lower affinity to oligomers than ZY-15-OMe. To understand this unusual behavior, we posited that the Aβ oligomers would start the fibrilization process when incubated in the cell media. ZY-15-MT, which exhibits a higher affinity to Aβ fibrils than ZY-15-OMe, might be able to bind to the more aggregated Aβ species and can also decrease their interactions with cell membranes. Therefore, we proceeded to probe if our compounds could prevent the binding of Aβ fibrils to cell membranes. When SH-SY5Y cells were treated with Aβ fibrils or a combination of fibrils and compounds for 24 h, both ZY-15-MT and ZY-15-OMe were not able to significantly decrease the numbers of the Aβ fibrils binding to cell membranes, while ZY-15-MT seems to decrease the interactions between Aβ fibrils and SH-SY5Y more than ZY-15-OMe (Fig. S11†). These results further suggest that both ZY-15-MT and ZY-15-OMe are more potent to alleviate the neurotoxicity of the Aβ oligomeric species. Given these encouraging results, we moved to study if the amphiphilic compounds can further control the interactions between Cu–Aβ species and cell membranes. Firstly, when SH-SY5Y cells were treated with Aβ monomers for 48 h, the aggregated Aβ species interacting with cell membranes were observed (Fig. 11a). Moreover, in the presence of Cu2+, even though there were only slightly increased interactions with membranes, larger aggregated Aβ species were observed compared to the group only with Aβ monomers treated (Fig. 11a and b). However, these aggregates are still smaller than the insoluble fibrils (Fig. S11†), indicating they are still soluble oligomeric Aβ species. Excitingly, in the presence of either compound, the Cu–Aβ species showed significantly decreased interactions with cell membranes (Fig. 11a and b), which might explain why the compounds can attenuate the toxicity of the Cu–Aβ aggregates. Taken together, we believe these findings suggest the developed amphiphilic compounds are neuroprotective by controlling the interactions between the Aβ oligomers and cell membranes, which we consider is a novel approach for the development of AD therapeutics.
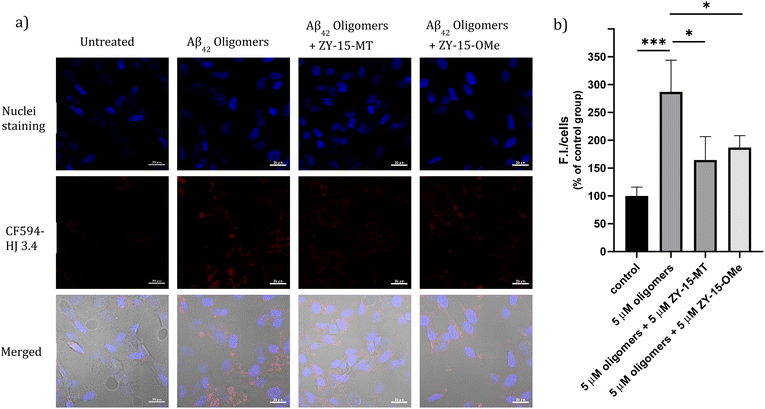 |
| Fig. 10 (a) SH-SY5Y cells were treated with 5 μM Aβ oligomers in the presence or absence of 5 μM ZY-15-MT or 5 μM ZY-15-OMe for 24 h before imaging. Red and blue fluorescence indicate the Aβ oligomers and nuclei, respectively. Scar bar, 20 μm. (b) Three independent experiments were subjected for the statistical analysis and analyzed by one-way ANOVA (*P < 0.05, ***P < 0.001). | |
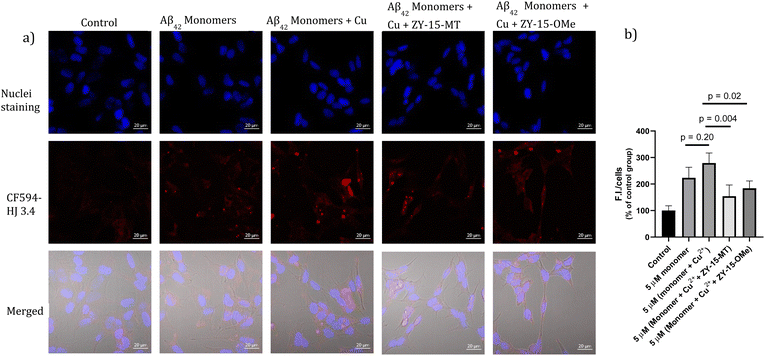 |
| Fig. 11 (a) SH-SY5Y cells were treated with 5 μM Aβ monomers in the presence or absence of 5 μM Cu2+, 5 μM (Cu2+ + ZY-15-MT) or 5 μM (Cu2+ + ZY-15-OMe) for 48 h before imaging. Red and blue fluorescence indicate the Aβ species and nuclei, respectively. Scar bar, 20 μm. (b) Three independent experiments were subjected for the statistical analysis and analyzed by one-way ANOVA (*P < 0.05, **P < 0.01). | |
Conclusions
In conclusion, we have designed and synthesized twelve amphiphilic compounds and studied their binding affinity toward Aβ42 species in vitro and ex vivo. Six of the compounds showed higher binding affinity towards Aβ42 oligomers, with compound ZY-5-MT showing the highest selectivity toward Aβ42 oligomers over Aβ42 fibrils. Interestingly, the less sterically hindered and less hydrophilic compounds are more selective towards Aβ42 oligomers in the ZY-5 and ZY-15 series, as evidenced by the DT compounds showing lower binding affinity towards both Aβ42 fibrils and Aβ42 oligomers compared to their ZY-MT or ZY-OMe analogs. However, when the stilbene backbone was changed, by replacing the thiophene with phenyl or switching the double bond position, both DT compounds were more selective towards Aβ42 oligomers in the ZY-12 and ZY-17 series. These structural studies indicate the hindrance of the molecules may not be the major factor, and we believe that the combination of hydrophobic fragments and hydrophilic groups is critical for the development of Aβ42 oligomer-selective compounds. More importantly, according to molecular docking studies, we found that targeting amino acids His6 and Asp7 might increase the binding affinity and selectivity toward Aβ42 oligomers. Based on cellular studies, we found that compounds with a higher binding affinity toward Aβ42 oligomers exhibit higher neurotoxicity, and most of them interact mainly with the amino acids His6 and Asp7. The only exception is compound ZY-15-OMe, which maintains its selectivity to Aβ42 oligomers via hydrogen bonds and π–π interactions with Tyr10, and, while exhibiting lower inherent cell toxicity, it was able to alleviate the Cu–Aβ42 neurotoxicity. Finally, confocal microscopy imaging studies show that both ZY-15-MT and ZY-15-OMe were able to decrease the interactions between Aβ42 oligomers and SH-SY5Y cell membranes, demonstrating their ability to target Aβ42 oligomers. These studies strongly suggest that developing such amphiphilic compounds could be an effective strategy to differentiate between Aβ42 oligomers and Aβ42 fibrils. We believe these encouraging results will help design Aβ42 oligomer-selective lead compounds to be used for AD therapeutic agent development.
Data availability
General methods, synthetic details, fluorescence imaging studies, molecular docking studies, and any additional experimental details are available in the ESI.† Any additional data that support the findings of this study are available from the corresponding author upon request.
Author contributions
L. M. M. directed the overall project. Z. Y. and L. M. M. conceived and designed the experiments. Z. Y. performed the chemical synthesis and in vitro characterization. Z. Y. and W. G. performed the cell imaging studies and analyzed data. Z. Y., S. P. and H.-J. C. performed the brain section imaging staining studies. Z. Y. and L. S. performed molecular docking studies. Z. Y. and L. M. M. wrote the paper with input from all authors.
Conflicts of interest
The authors declare no competing financial interest.
Acknowledgements
This work was supported by research funding from the NIH (R01GM114588 to L. M. M.). We thank the School of Chemical Sciences NMR lab and MS lab for their help. We thank the core facilities at the Carl R. Woese Institute for Genomic Biology (IGB) for microscope training and support. We also thank the small animal facilities at IGB for their excellent support.
References
- J. Hardy and D. J. Selkoe, The amyloid hypothesis of Alzheimer's disease: progress and problems on the road to therapeutics, Science, 2002, 297(5580), 353–356 CrossRef CAS PubMed.
- D. J. Selkoe and J. Hardy, The amyloid hypothesis of Alzheimer's disease at 25 years, EMBO Mol. Med., 2016, 8(6), 595–608 CrossRef CAS PubMed.
- M. G. Spillantini, M. L. Schmidt, V. M. Y. Lee, J. Q. Trojanowski, R. Jakes and M. Goedert, α-Synuclein in Lewy bodies, Nature, 1997, 388(6645), 839–840 CrossRef CAS PubMed.
-
2020 Alzheimer's disease facts and figures, Alzheimer's Dementia, 2020, 16(3), 391–460 Search PubMed.
- D. J. Selkoe, Resolving controversies on the path to Alzheimer's therapeutics, Nat. Med., 2011, 17(9), 1060–1065 CrossRef CAS PubMed.
- C. Haass and D. J. Selkoe, Soluble Protein Oligomers in Neurodegeneration: Lessons from the Alzheimer's Amyloid b-Peptide, Nat. Rev. Mol. Cell Biol., 2007, 8(2), 101–112 CrossRef CAS PubMed.
- I. Benilova, E. Karran and B. De Strooper, The Toxic Aβ Oligomer and Alzheimer's Disease: An Emperor in Need of Clothes, Nat. Neurosci., 2012, 15, 349–357 CrossRef CAS PubMed.
- S. J. C. Lee, E. Nam, H. J. Lee, M. G. Savelieff and M. H. Lim, Towards an understanding of amyloid-beta oligomers: characterization, toxicity mechanisms, and inhibitors, Chem. Soc. Rev., 2017, 46(2), 310–323 RSC.
- E. N. Cline, M. A. Bicca, K. L. Viola and W. L. Klein, The Amyloid-β Oligomer Hypothesis: Beginning of the Third Decade, J. Alzheimer's Dis., 2018, 64, S567–S610 CAS.
- E. Karran, M. Mercken and B. D. Strooper, The Amyloid Cascade Hypothesis for Alzheimer's Disease: An Appraisal for the Development of Therapeutics, Nat. Rev. Drug Discovery, 2011, 10(9), 698–712 CrossRef CAS PubMed.
- F. Hefti, W. F. Goure, J. Jerecic, K. S. Iverson, P. A. Walicke and G. A. Krafft, The case for soluble Aβ oligomers as a drug target in Alzheimer's disease, Trends Pharmacol. Sci., 2013, 34(5), 261–266 CrossRef CAS PubMed.
- A. R. A. Ladiwala, M. Bhattacharya, J. M. Perchiacca, P. Cao, D. P. Raleigh, A. Abedini, A. M. Schmidt, J. Varkey, R. Langen and P. M. Tessier, Rational design of potent domain antibody inhibitors of amyloid fibril assembly, Proc. Natl. Acad. Sci. U. S. A., 2012, 109(49), 19965 CrossRef CAS.
- C. Xiao, F. J. Davis, B. C. Chauhan, K. L. Viola, P. N. Lacor, P. T. Velasco, W. L. Klein and N. B. Chauhan, Brain transit and ameliorative effects of intranasally delivered anti-amyloid-β oligomer antibody in 5XFAD mice, J. Alzheimer's Dis., 2013, 35(4), 777–788 Search PubMed.
- A. Frydman-Marom, M. Rechter, I. Shefler, Y. Bram, D. E. Shalev and E. Gazit, Cognitive-Performance Recovery of Alzheimer's Disease Model Mice by Modulation of Early Soluble Amyloidal Assemblies, Angew. Chem., Int. Ed., 2009, 48(11), 1981–1986 CrossRef CAS PubMed.
- P. J. Salveson, S. Haerianardakani, A. Thuy-Boun, A. G. Kreutzer and J. S. Nowick, Controlling the Oligomerization State of Aβ-Derived Peptides with Light, J. Am. Chem. Soc., 2018, 140(17), 5842–5852 CrossRef CAS PubMed.
- D. E. Ehrnhoefer, J. Bieschke, A. Boeddrich, M. Herbst, L. Masino, R. Lurz, S. Engemann, A. Pastore and E. E. Wanker, EGCG redirects amyloidogenic polypeptides into unstructured, off-pathway oligomers, Nat. Struct. Mol. Biol., 2008, 15(6), 558–566 CrossRef CAS PubMed.
- A. R. A. Ladiwala, J. S. Dordick and P. M. Tessier, Aromatic small molecules remodel toxic soluble oligomers of amyloid beta through three independent pathways, J. Biol. Chem., 2011, 286(5), 3209–3218 CrossRef CAS.
- J. A. Lenhart, X. Ling, R. Gandhi, T. L. Guo, P. M. Gerk, D. H. Brunzell and S. Zhang, “Clicked” Bivalent Ligands Containing Curcumin and Cholesterol As Multifunctional Aβ Oligomerization Inhibitors: Design, Synthesis, and Biological Characterization, J. Med. Chem., 2010, 53(16), 6198–6209 CrossRef CAS.
- L. Sun, A. K. Sharma, B.-H. Han and L. M. Mirica, Amentoflavone: A Bifunctional Metal Chelator that Controls the Formation of Neurotoxic Soluble Aβ42 Oligomers, ACS Chem. Neurosci., 2020, 11(17), 2741–2752 CrossRef CAS PubMed.
- J. Bieschke, M. Herbst, T. Wiglenda, R. P. Friedrich, A. Boeddrich, F. Schiele, D. Kleckers, J. M. Lopez del Amo, B. A. Grüning, Q. Wang, M. R. Schmidt, R. Lurz, R. Anwyl, S. Schnoegl, M. Fändrich, R. F. Frank, B. Reif, S. Günther, D. M. Walsh and E. E. Wanker, Small-molecule conversion of toxic oligomers to nontoxic β-sheet–rich amyloid fibrils, Nat. Chem. Biol., 2012, 8(1), 93–101 CrossRef CAS.
- P.-O. Freskgård and E. Urich, Antibody therapies in CNS diseases, Neuropharm, 2017, 120, 38–55 CrossRef.
- D. Maity, M. Howarth, M. C. Vogel, M. Magzoub and A. D. Hamilton, Peptidomimetic-Based Vesicles Inhibit Amyloid-β Fibrillation and Attenuate Cytotoxicity, J. Am. Chem. Soc., 2021, 143(8), 3086–3093 CrossRef CAS.
- L. Sun, H.-J. Chi, S. Sen, A. S. Arango, T. T. Huynh, Y. Huang, N. Bandara, B. E. Rogers, E. Tajkhorshid and L. M. Mirica, Amphiphilic Distyrylbenzene Derivatives as Potential Therapeutic and Imaging Agents for the Soluble Amyloid-β Oligomers in Alzheimer's Disease, J. Am. Chem. Soc., 2021, 143, 10462–10476 CrossRef CAS PubMed.
- M. Necula, R. Kayed, S. Milton and C. G. Glabe, Small molecule inhibitors of aggregation indicate that amyloid β oligomerization and fibrillization pathways are independent and distinct, J. Biol. Chem., 2007, 282(14), 10311–10324 CrossRef CAS PubMed.
- See ESI.†.
- N. Bandara, A. K. Sharma, S. Krieger, J. W. Schultz, B. H. Han, B. E. Rogers and L. M. Mirica, Evaluation of 64Cu-based Radiopharmaceuticals That Target Aβ Peptide Aggregates as Diagnostic Tools for Alzheimer's Disease, J. Am. Chem. Soc., 2017, 139(36), 12550–12558 CrossRef CAS PubMed.
- A. K. Sharma, J. W. Schultz, J. T. Prior, N. P. Rath and L. M. Mirica, Coordination Chemistry of Bifunctional Chemical Agents Designed for Applications in (64)Cu PET Imaging for Alzheimer's Disease, Inorg. Chem., 2017, 56(22), 13801–13814 CrossRef CAS PubMed.
- W. L. Klein, G. A. Krafft and C. E. Finch, Targeting small A beta oligomers: the solution to an Alzheimer's disease conundrum?, Trends Neurosci., 2001, 24(4), 219–224 CrossRef CAS.
- A. Lockhart, L. Ye, D. B. Judd, A. T. Merritt, P. N. Lowe, J. L. Morgenstern, G. Z. Hong, A. D. Gee and J. Brown, Evidence for the presence of three distinct binding sites for the thioflavin T class of Alzheimer's disease PET imaging agents on beta-amyloid peptide fibrils, J. Biol. Chem., 2005, 280(9), 7677–7684 CrossRef CAS PubMed.
- Y. Li, J. Yang, H. Liu, J. Yang, L. Du, H. Feng, Y. Tian, J. Cao and C. Ran, Tuning the stereo-hindrance of a curcumin scaffold for the selective imaging of the soluble forms of amyloid beta species, Chem. Sci., 2017, 8(11), 7710–7717 RSC.
- C. L. Teoh, D. Su, S. Sahu, S. W. Yun, E. Drummond, F. Prelli, S. Lim, S. Cho, S. Ham, T. Wisniewski and Y. T. Chang, Chemical Fluorescent Probe for Detection of Abeta Oligomers, J. Am. Chem. Soc., 2015, 137(42), 13503–13509 CrossRef CAS PubMed.
- Y. Li, D. Xu, A. Sun, S. L. Ho, C. Y. Poon, H. N. Chan, O. T. W. Ng, K. K. L. Yung, H. Yan, H. W. Li and M. S. Wong, Fluoro-substituted cyanine for reliable in vivo labelling of amyloid-beta oligomers and neuroprotection against amyloid-beta induced toxicity, Chem. Sci., 2017, 8(12), 8279–8284 RSC.
- H. Oakley, S. L. Cole, S. Logan, E. Maus, P. Shao, J. Craft, A. Guillozet-Bongaarts, M. Ohno, J. Disterhoft, L. Van Eldik, R. Berry and R. Vassar, Intraneuronal beta-amyloid aggregates, neurodegeneration, and neuron loss in transgenic mice with five familial Alzheimer's disease mutations: potential factors in amyloid plaque formation, J. Neurosci., 2006, 26(40), 10129–10140 CrossRef CAS PubMed.
- D. M. Wilcock, M. N. Gordon and D. Morgan, Quantification of cerebral amyloid angiopathy and parenchymal amyloid plaques with Congo red histochemical stain, Nat. Protoc., 2006, 1, 1591–1595 CrossRef CAS PubMed.
- C. Z. Ran, X. Y. Xu, S. B. Raymond, B. J. Ferrara, K. Neal, B. J. Bacskai, Z. Medarova and A. Moore, Design, Synthesis, and Testing of Difluoroboron-Derivatized Curcumins as Near-Infrared Probes for in Vivo Detection of Amyloid-beta Deposits, J. Am. Chem. Soc., 2009, 131(42), 15257–15261 CrossRef CAS PubMed.
- T. T. Wager, R. Y. Chandrasekaran, X. Hou, M. D. Troutman, P. R. Verhoest, A. Villalobos and Y. Will, Defining Desirable Central Nervous System Drug Space through the Alignment of Molecular Properties, in Vitro ADME, and Safety Attributes, ACS Chem. Neurosci., 2010, 1(6), 420–434 CrossRef CAS PubMed.
- T. T. Wager, X. Hou, P. R. Verhoest and A. Villalobos, Moving beyond Rules: The Development of a Central Nervous System Multiparameter Optimization (CNS MPO) Approach to Enable Alignment of Druglike Properties, ACS Chem. Neurosci., 2010, 1(6), 435–449 CrossRef CAS PubMed.
- R. A. Friesner, J. L. Banks, R. B. Murphy, T. A. Halgren, J. J. Klicic, D. T. Mainz, M. P. Repasky, E. H. Knoll, M. Shelley, J. K. Perry, D. E. Shaw, P. Francis and P. S. Shenkin, Glide: a new approach for rapid, accurate docking and scoring. 1. Method and assessment of docking accuracy, J. Med. Chem., 2004, 47(7), 1739–1749 CrossRef CAS.
- L. Gremer, D. Scholzel, C. Schenk, E. Reinartz, J. Labahn, R. B. G. Ravelli, M. Tusche, C. Lopez-Iglesias, W. Hoyer, H. Heise, D. Willbold and G. F. Schroder, Fibril structure of amyloid-beta(1-42) by cryo-electron microscopy, Science, 2017, 358(6359), 116–119 CrossRef CAS PubMed.
- Y. Zou, Z. Qian, Y. Chen, H. Qian, G. Wei and Q. Zhang, Norepinephrine Inhibits Alzheimer's Amyloid-β Peptide Aggregation and Destabilizes Amyloid-β Protofibrils: A Molecular Dynamics Simulation Study, ACS Chem. Neurosci., 2019, 10(3), 1585–1594 CrossRef CAS PubMed.
- A. Gautieri, M. Beeg, M. Gobbi, F. Rigoldi, L. Colombo and M. Salmona, The Anti-Amyloidogenic Action of Doxycycline: A Molecular Dynamics Study on the Interaction with Aβ42, Int. J. Mol. Sci., 2019, 20(18), 4641 CrossRef CAS PubMed.
- S. Ciudad, E. Puig, T. Botzanowski, M. Meigooni, A. S. Arango, J. Do, M. Mayzel, M. Bayoumi, S. Chaignepain, G. Maglia, S. Cianferani, V. Orekhov, E. Tajkhorshid, B. Bardiaux and N. Carulla, A beta(1-42) tetramer and octamer structures reveal edge conductivity pores as a mechanism for membrane damage, Nat. Commun., 2020, 11(1), 3014 CrossRef CAS PubMed.
- M. Biancalana and S. Koide, Molecular mechanism of Thioflavin-T binding to amyloid fibrils, Biochim. Biophys. Acta, 2010, 1804(7), 1405–1412 CrossRef CAS PubMed.
- J. Yang, B. Zhu, W. Yin, Z. Han, C. Zheng, P. Wang and C. Ran, Differentiating Aβ40 and Aβ42 in amyloid plaques with a small molecule fluorescence probe, Chem. Sci., 2020, 11(20), 5238–5245 RSC.
- S. S. Hindo, A. M. Mancino, J. J. Braymer, Y. H. Liu, S. Vivekanandan, A. Ramamoorthy and M. H. Lim, Small Molecule Modulators of Copper-Induced A beta Aggregation, J. Am. Chem. Soc., 2009, 131(46), 16663–16664 CrossRef CAS PubMed.
- A. K. Sharma, S. T. Pavlova, J. Kim, J. Kim and L. M. Mirica, The effect of Cu2+ and Zn2+ on the Aβ42 peptide aggregation and cellular toxicity, Metallomics, 2013, 5(11), 1529–1536 CrossRef CAS PubMed.
- Y. Huang, H.-J. Cho, N. Bandara, L. Sun, D. Tran, B. E. Rogers and L. M. Mirica, Metal-chelating benzothiazole multifunctional compounds for the modulation and 64Cu PET imaging of Aβ aggregation, Chem. Sci., 2020, 11(30), 7789–7799 RSC.
- E. Atrian-Blasco, P. Gonzalez, A. Santoro, B. Alies, P. Faller and C. Hureau, Cu and Zn coordination to amyloid peptides: From fascinating chemistry to debated pathological relevance, Coord. Chem. Rev., 2018, 375, 38–55 CrossRef PubMed.
- M. Talantova, S. Sanz-Blasco, X. Zhang, P. Xia, M. W. Akhtar, S.-i. Okamoto, G. Dziewczapolski, T. Nakamura, G. Cao, A. E. Pratt, Y.-J. Kang, S. Tu, E. Molokanova, S. R. McKercher, S. A. Hires, H. Sason, D. G. Stouffer, M. W. Buczynski, J. P. Solomon, S. Michael, E. T. Powers, J. W. Kelly, A. Roberts, G. Tong, T. Fang-Newmeyer, J. Parker, E. A. Holland, D. Zhang, N. Nakanishi, H. S. V. Chen, H. Wolosker, Y. Wang, L. H. Parsons, R. Ambasudhan, E. Masliah, S. F. Heinemann, J. C. Piña-Crespo and S. A. Lipton, Aβ induces astrocytic glutamate release, extrasynaptic NMDA receptor activation, and synaptic loss, Proc. Natl. Acad. Sci. U. S. A., 2013, 110(27), E2518 CrossRef CAS PubMed.
- J. W. Um, H. B. Nygaard, J. K. Heiss, M. A. Kostylev, M. Stagi, A. Vortmeyer, T. Wisniewski, E. C. Gunther and S. M. Strittmatter, Alzheimer amyloid-beta oligomer bound to postsynaptic prion protein activates Fyn to impair neurons, Nat. Neurosci., 2012, 15(9), 1227–1235 CrossRef CAS PubMed.
- E. Cerf, R. Sarroukh, S. Tamamizu-Kato, L. Breydo, S. Derclaye, Y. F. Dufrêne, V. Narayanaswami, E. Goormaghtigh, J.-M. Ruysschaert and V. Raussens, Antiparallel β-sheet: a signature structure of the oligomeric amyloid β-peptide, Biochem. J., 2009, 421(3), 415–423 CrossRef CAS PubMed.
- A. Y. Lai and J. McLaurin, Mechanisms of amyloid-Beta Peptide uptake by neurons: the role of lipid rafts and lipid raft-associated proteins, Int. J. Alzheimer's Dis., 2010, 2011, 548380 Search PubMed.
- N. Arispe, E. Rojas and H. B. Pollard, Alzheimer-Disease Amyloid Beta-Protein Forms Calcium Channels in Bilayer-Membranes - Blockade by Tromethamine and Aluminum, Proc. Natl. Acad. Sci. U. S. A., 1993, 90(2), 567–571 CrossRef CAS PubMed.
- N. Arispe, H. B. Pollard and E. Rojas, Zn2+ interaction with Alzheimer amyloid beta protein calcium channels, Proc. Natl. Acad. Sci. U. S. A., 1996, 93(4), 1710–1715 CrossRef CAS PubMed.
- H. Lin, R. Bhatia and R. Lal, Amyloid beta protein forms ion channels: implications for Alzheimer's disease pathophysiology, FASEB J., 2001, 15(13), 2433–2444 CrossRef CAS PubMed.
- R. Limbocker, S. Chia, F. S. Ruggeri, M. Perni, R. Cascella, G. T. Heller, G. Meisl, B. Mannini, J. Habchi, T. C. T. Michaels, P. K. Challa, M. Ahn, S. T. Casford, N. Fernando, C. K. Xu, N. D. Kloss, S. I. A. Cohen, J. R. Kumita, C. Cecchi, M. Zasloff, S. Linse, T. P. J. Knowles, F. Chiti, M. Vendruscolo and C. M. Dobson, Trodusquemine enhances Aβ42 aggregation but suppresses its toxicity by displacing oligomers from cell membranes, Nat. Commun., 2019, 10(1), 225 CrossRef CAS PubMed.
- J. D. Grayson, M. P. Baumgartner, C. D. Santos Souza, S. J. Dawes, I. G. El Idrissi, J. C. Louth, S. Stimpson, E. Mead, C. Dunbar, J. Wolak, G. Sharman, D. Evans, A. Zhuravleva, M. S. Roldan, N. A. Colabufo, K. Ning, C. Garwood, J. A. Thomas, B. M. Partridge, A. de la Vega de Leon, V. J. Gillet, A. P. Rauter and B. Chen, Amyloid binding and beyond: a new approach for Alzheimer's disease drug discovery targeting Aβo–PrPC binding and downstream pathways, Chem. Sci., 2021, 12(10), 3768–3785 RSC.
|
This journal is © The Royal Society of Chemistry 2022 |
Click here to see how this site uses Cookies. View our privacy policy here.