DOI:
10.1039/D2SC00982J
(Edge Article)
Chem. Sci., 2022,
13, 5616-5621
Directing group assisted rhodium catalyzed meta-C–H alkynylation of arenes†
Received
15th February 2022
, Accepted 8th April 2022
First published on 20th April 2022
Abstract
Site-selective C–H alkynylation of arenes to produce aryl alkynes is a highly desirable transformation due to the prevalence of aryl alkynes in various natural products, drug molecules and in materials. To ensure site-selective C–H functionalization, directing group (DG) assisted C–H activation has been evolved as a useful synthetic tool. In contrast to DG-assisted ortho-C–H activation, distal meta-C–H activation is highly challenging and has attracted significant attention in recent years. However, developments are majorly focused on Pd-based catalytic systems. In order to diversify the scope of distal meta-C–H functionalization, herein we disclosed the first Rh(I) catalyzed meta-C–H alkynylation protocol through the inverse Sonogashira coupling reaction. The protocol is compatible with various substrate classes which include phenylacetic acids, hydrocinnamic acids, 2-phenyl benzoic acids, 2-phenyl phenols, benzyl sulfonates and ether-based scaffolds. The post-synthetic modification of meta-alkynylated arenes is also demonstrated through DG-removal as well as functional group interconversion.
Transition metal catalyzed C–H activation has evolved as a powerful synthetic tool as it offers a simplified route to incorporate several functional groups by converting the inert C–H bond into various carbon–carbon or carbon–heteroatom bonds.1 The key to success in molecular diversification through C–H bond activation require recognition of a selective C–H bond amongst multiple C–H bonds.2 Directed C–H activation in this regard provides a unique solution to ensure site-selective C–H activation in a predictable manner.3 Directing ability of an attached functional group to accommodate transition metal catalysts to the closest proximity of the desired C–H bond ensured site-selective C–H functionalization. However, the progress of directed C–H activation is majorly centered around ortho-C–H activation, which typically proceeds via five- to seven-membered metallacyclic intermediates.4 Nevertheless, distal meta-C–H functionalization5 aided by directing group assistance has recently attracted significant attention.6 The formation of a large macrocyclic pre-transition state (usually greater than 11-membered) is the prerequisite criterion to be successful in site-selective distal C–H activation.7
As far as meta-C–H activation is concerned, a “U-shaped” template was elegantly designed by the group of Yu to achieve selective meta-C–H activation relying on the linear, end-on, weak coordinating ability of nitrile-based directing groups.8 Based on this seminal report, various nitrile-based templates were developed by Tan,9a Li9b and us9c to accomplish majorly meta-C–H olefination reaction. Later, our group developed a strong σ-coordinating pyrimidine-based directing group which allowed several functional groups to be incorporated selectively at the meta-position with different classes of substrates.10 Despite the success in achieving various meta-selective functionalizations utilizing both weak and strong coordinating directing templates, developments were majorly focused on systems formed by the combination of the Pd-catalyst and MPAA-ligands (MPAA: mono-protected-amino acid).11 Considering the rapid resurgence in template assisted meta-C–H functionalization under transition metal catalyzed conditions other than the Pd–MPAA catalytic system, we are intrigued to develop Rh-catalyzed methods for various meta-C–H functionalizations. In this context, Rh-catalyzed meta-C–H alkenylation using activated alkenes was achieved by the group of Yu12 and us13 in 2017 (Scheme 1a). In 2019, the Yu group also demonstrated meta-C–H alkenylation of hydrocinnamic acids using internal alkynes (Scheme 1b).14 However, the wide applicability of Rh-catalysis for regioselective distal C–H functionalization15 is yet to be explored. We, thus, became interested in examining the feasibility of meta-selective alkynylation reaction with the Rh-catalyst (Scheme 1c).
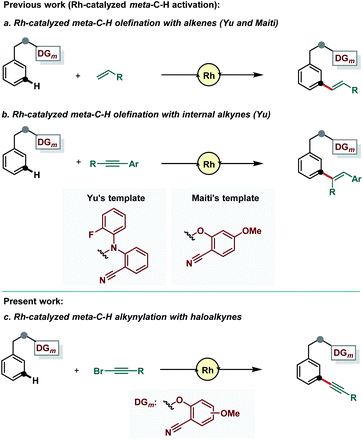 |
| Scheme 1 Rh-catalyzed meta-C–H functionalization. | |
Alkynylation of arenes has been a reaction of great interest due to the ubiquity of aryl alkynes in various natural products, agrochemicals, pharmaceuticals, and in materials.16 In addition to that, alkynes are considered as one of the most versatile synthons as they can serve as a transformative handle for further functionalization (through cycloaddition reaction, cross coupling reaction, metathesis reaction and many more).17 Importantly, alkynes provide a linear and rigid spacer in molecular arrangement. Therefore, regioselective alkynylation of arenes would play a beneficial role in developing new materials, pharmaceuticals and other valuable compounds, where two presently known components can be attached through the C–C triple bond and a new drug or materials could be synthesized with improved activity. Despite the enormous success of the traditional Sonogashira coupling reaction in generating aryl alkynes,18 direct C(sp2)–H alkynylation is an extremely useful method as it precludes the use of pre-functionalized arenes.19,20 Encouraged by the prospect of direct C(sp2)–H alkynylation, we have developed a Pd-catalyzed meta-C–H alkynylation protocol using a pyrimidine-based directing group.11g In order to diversify the scope of template assisted meta-C–H functionalization involving transition metal catalysts other than palladium, herein we report meta-C–H alkynylation of structurally different classes of arenes via the inverse Sonogashira coupling reaction utilizing the Rh(I)-catalyst.
Benzyl sulfonate ester (1) embedded with 2-hydroxy-4-methoxybenzonitrile (DG1), and (bromoethynyl)triisopropylsilane (2a) was chosen as the model substrate and alkynylating reagent, respectively, to test the feasibility of the meta-selective inverse Sonogashira coupling reaction.11g Initial attempts of meta-C–H alkynylation using [Rh(cod)Cl]2 as the catalyst, Cu(TFA)2 as the oxidant and XPhos as the ligand in DCE solvent remained unsuccessful.13 Subsequently, different copper-based and silver-based oxidants were examined and gratifyingly in the presence of silver sulfate the desired meta-alkynylated compound was formed in 40% yield with moderate selectivity.21 Further optimization of reaction parameters revealed that a combination of Ag2SO4 and Cu2Cr2O5 as the oxidant was effective in delivering the desired product in 55% yield. The yield and selectivity were significantly improved while 1-adamantanecarboxylic acid was used as an additive. Careful optimization of other reaction parameters led us to produce the desired meta-alkynylated compounds in 70% yield and 10
:
1 meta-selectivity.21 Notably, RhCp*Cl2 and Rh2(OAc)4 were ineffective in producing the expected compound in synthetically acceptable yield and selectivity. Thereafter, we examined the efficacy of previously developed meta-DGs. While strongly coordinating pyridine, pyrimidine and quinoline-based DGs (DG4 to DG6) were ineffective for the present transformation, the electronically modified cyano-based DG3 was found to be superior in comparison to DG1 and DG2 to deliver the desired product in 72% isolated yield with improved meta-selectivity (Table 1).
Table 1 Directing group (DG) optimization
Isolated yield.
|
|
After having the optimized reaction conditions and the suitable directing group, we explored the scope of the reaction with respect to various benzyl sulfonate esters (1) (Scheme 2). Arenes bearing electron donating as well as electron withdrawing substituents at ortho- and meta-positions were well tolerated to deliver the desired meta-alkynylated products in synthetically useful yields and selectivity. Notably, ortho-chloro and meta-bromo substrates (1d and 1k, respectively) were also compatible under the reaction conditions to produce meta-C–H alkynylated products without any interference, caused by the probable cross coupling reaction.
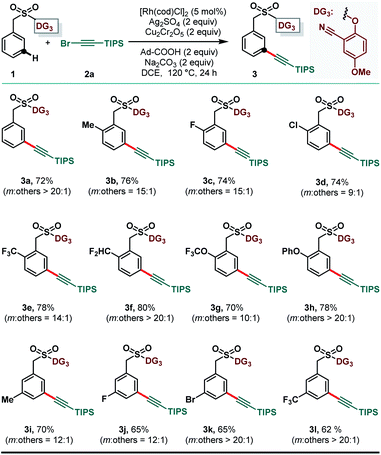 |
| Scheme 2 Rh-catalyzed meta-C–H alkynylation of benzyl sulfonate esters. | |
The versatility of the developed protocol was further demonstrated with phenylacetic acid, hydrocinnamic acid and benzoic acid derivatives (4) (Scheme 3). Considering the prevalence of phenylacetic acid derivatives in pharmaceuticals, meta-selective alkynylation would render a unique opportunity to study the impact of structurally modified pharmaceutical cores. However, DG1 was found to be more efficacious for meta-C–H alkynylation of carboxylic acid derivatives. While ortho- and meta-methyl substituted phenylacetic acid derivatives (4a and 4c, respectively) provided the desired meta-alkynylated compounds in good yields, pharmaceutically relevant ketoprofen derived 4d delivered the expected product (5d) in 75% yield. The phenylacetic acid derivative possessing the methoxy group also provided the useful yield of the meta-alkynylated product (5b) without compromising the meta-selectivity. The Rh-catalyzed method for meta-selective alkynylation was also successful with derivatized hydrocinnamic acids (4e–4h) and 2-phenylbenzoic acids (4i–4n). Notably, ortho-chloro and meta-chloro hydrocinnamic acid derivatives delivered the desired meta-alkynylated products (5g and 5h, respectively) in good yield and selectivity.
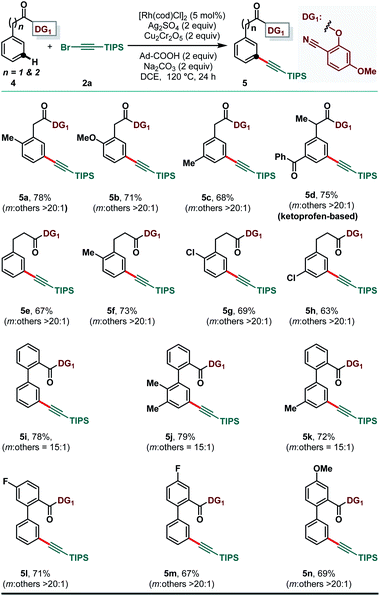 |
| Scheme 3 Rh-catalyzed meta-C–H alkynylation of phenylacetic acids, hydrocinnamic acids and biphenyls. | |
While the distance and geometric relationship between the appended directing group and the desired site of C–H activation is the key parameter in accomplishing regioselective distal C–H activation, our developed method was efficacious in delivering the desired meta-selective alkynylation even when DG3 was tethered to the targeted arenes through flexible ether linkages. A number of substituted arenes bearing variable linker length were compatible under the reaction conditions to produce meta-alkynylated arenes (7) in excellent yield and uncompromised selectivity (Scheme 4).
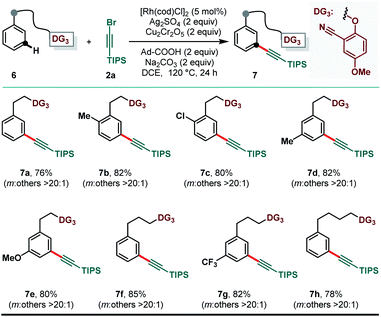 |
| Scheme 4 Rh-catalyzed meta-C–H alkynylation of ethers with variable linker lengths. | |
The compatibility of various classes of substrates was, thus far, examined with (bromoethynyl)triisopropylsilane (2a) as the alkynylating reagent. We further investigated the scope of the reaction with respect to other alkynyl bromides, derived from propargyl silyl ethers and with these derivatized alkynyl bromides the meta-alkynylation reaction proceeded smoothly (Scheme 5). Alkynyl bromide, derived from menthone, was easily coupled at the meta-position of the benzyl sulfonate scaffold (8b), ester derivative of phenylacetic acid (8c), hydrocinnamic acid (8f), and 2-phenethyl ether (8i) furnishing good yields and selectivity. Importantly, meta-selective alkynylation of the appended arene in 2-phenyl phenol (8g) was also achieved in excellent yield and selectivity by embedding the 2-cyano benzoic acid (DG7) as the meta-directing group. It is worth noting that the reaction outcome in terms of yield and selectivity was not significantly impacted by the electronic nature of the substituents, establishing the fact that the directing ability of the cyano group could override the electronically controlled C–H activation.
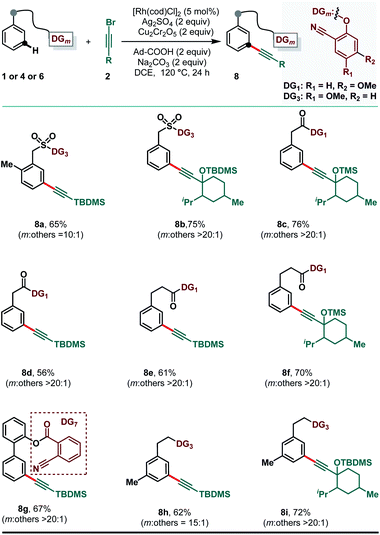 |
| Scheme 5
meta-C–H alkynylation with alkyne variants. | |
Post functionalization DG removal is crucial in DG-assisted C–H functionalization methods (Scheme 6). The appended meta-directing group was successfully cleaved producing the benzyl sulfonic acid derivative (9) when the corresponding meta-alkynylated benzyl sulfonate (3a) was treated with TBAF. Additionally, the sulfonate linker was modified to form a styrene derivative via modified Julia reaction conditions and a meta-alkynylated styrene (10) was prepared in 70% yield. In both the cases, the directing group was recovered in quantitative amount, highlighting the practicality of the developed method. As alluded earlier, alkyne is one of the versatile synthons and an acyl group was readily incorporated at the meta-position (11) via functional group interconversion. We majorly used TIPS-acetylene bromide as a coupling partner to introduce TIPS-acetylene, a masked ethynyl motif, at the meta-position. Easy removal of the triisopropyl silyl (TIPS) group resulted in the meta-ethynyl arene (12), which can be further used in the Sonogashira cross coupling reaction or in the cross dehydrogenative coupling reaction. Notably, these cross-coupling reactions would allow various structural units to be assembled, which are important pharmacophores or prevalent in materials, through a carbon–carbon triple bond.
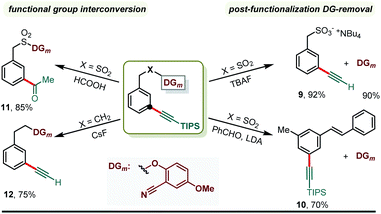 |
| Scheme 6 Post-synthetic modification of meta-functionalized arenes and DG-removal. | |
A plausible mechanism is outlined in Scheme 7, in which in situ oxidation of Rh(I) to Rh(III) takes place prior to the participation of the Rh-catalyst in the catalytic process. The linear coordination of the nitrile group directs the Rh(III)-catalyst to the meta-C–H bond of the targeted arenes.12,13 The meta-C–H activation resulted in a cyclophane type intermediate I, which is coordinated with bromoalkynes to produce II. Subsequent syn-insertion of alkyne to the rhodium–carbon bond would generate intermediate III. Further, an Ag-assisted β-bromide elimination reaction would lead to the generation of IV.11g,19p Finally, ligand exchange released the expected alkynylated product and restarted the catalytic cycle by regenerating the active Rh-catalyst.
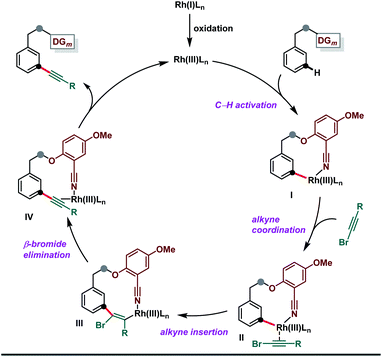 |
| Scheme 7 Plausible mechanism of Rh-catalyzed meta-C–H alkynylation. | |
Conclusions
In summary, an unprecedented report on Rh-catalyzed meta-C–H alkynylation of arenes, aided by a weakly coordinating cyano-based directing template, was developed using bromoalkynes as the coupling partner. The generality of the developed protocol was demonstrated by transforming various classes of substrates including benzyl sulfonate esters, phenyl acetic acids, elongated alkyl ethers, 2-phenyl benzoic acids and 2-phenyl phenols to the corresponding meta-C–H alkynylated compounds. The post-synthetic modification of the synthesized meta-alkynylated arenes was carried out either through the removal of the directing group or via functional group interconversion of the alkyne functionality. Considering the recent upsurge in site-selective distal C–H functionalization through template assistance, the present Rh-catalyzed method is expected to have a significant impact on future development.
Data availability
All experimental data, and detailed experimental procedures are available in the ESI.†
Author contributions
U. D. and D. M. conceived the project. S. S., G. P., U. D. and R. L. completed the experimental work. D. M. and G. K. L. supervised the work. All authors contributed to writing the manuscript.
Conflicts of interest
The authors declare no conflict of interest.
Acknowledgements
This activity is funded by SERB-India (CRG/2018/003951 for D. M.), and the J. C. Bose Fellowship (SERB, G. K. L.). Financial support received as a fellowship from IIT Bombay (to S. S.), CSIR-India (to G. P.) is gratefully acknowledged.
Notes and references
-
(a) L. McMurray, F. O. Hara and M. J. Gaunt, Chem. Soc. Rev., 2011, 40, 1885–1898 RSC;
(b) J. Yamaguchi, A. D. Yamaguchi and K. Itami, Angew. Chem., Int. Ed., 2012, 51, 8960–9009 CrossRef CAS PubMed;
(c)
X. Ribas, C–H and C–X Bond Function: Transition Metal Mediation, RSC Publishing, London, 2013 RSC;
(d) J. Wencel-Delord and F. Glorius, Nat. Chem., 2013, 5, 369–375 CrossRef CAS PubMed;
(e) D. J. Abrams, P. A. Provencher and E. J. Sorensen, Chem. Soc. Rev., 2018, 47, 8925–8967 RSC;
(f) A. Tortajada, F. Juli-Hernandez, M. Borjesson, T. Moragas and R. Martin, Angew. Chem., Int. Ed., 2018, 57, 15948–15982 (
Angew. Chem.
, 2018
, 130
, 16178–16214
) CrossRef CAS PubMed.
- Z. Huang and G. Dong, Acc. Chem. Res., 2017, 50, 465–471 CrossRef CAS PubMed.
- For selected references on C–H functionalizations, see:
(a)
J.-Q. Yu and Z. Shi, C–H Activation; Topics in Current Chemistry, Springer, New York, 2010, vol. 26 CrossRef;
(b)
A. Kapdi and D. Maiti, Strategies for Palladium-Catalyzed Non-directed and Directed C–H Bond Functionalization, Elsevier, Amsterdam, 2017 Search PubMed.
- Selected examples on DG assisted ortho-C–H functionalization:
(a) S. Murai, F. Kakiuchi, S. Sekine, Y. Tanaka, A. Kamatani, M. Sonoda and N. Chatani, Nature, 1993, 366, 529–531 CrossRef CAS;
(b) T. W. Lyons and M. S. Sanford, Chem. Rev., 2010, 110, 1147–1169 CrossRef CAS PubMed;
(c) N. Kuhl, M. N. Hopkinson, J. Wencel-Delord and F. Glorius, Angew. Chem., Int. Ed., 2012, 51, 10236–10254 (
Angew. Chem.
, 2012
, 124
, 10382–10401
) CrossRef CAS PubMed;
(d) G. Rouquet and N. Chatani, Angew. Chem., Int. Ed., 2013, 52, 11726–11743 (
Angew. Chem.
, 2013
, 125
, 11942–11959
) CrossRef CAS PubMed;
(e) M. Zhang, Y. Zhang, X. Jie, H. Zhao, G. Li and W. Su, Org. Chem. Front., 2014, 1, 843–895 RSC;
(f) L. Ackermann, Acc. Chem. Res., 2014, 47, 281–295 CrossRef CAS PubMed;
(g) Z. Chen, B. Wang, J. Zhang, W. Yu, Z. Liu and Y. Zhang, Org. Chem. Front., 2015, 2, 1107–1295 RSC;
(h) Z. Huang, H. N. Lim, F. Mo, M. C. Young and G. Dong, Chem. Soc. Rev., 2015, 44, 7764–7786 RSC;
(i) T. Gensch, M. N. Hopkinson, F. Glorius and J. Wencel-Delord, Chem. Soc. Rev., 2016, 45, 2900–2936 RSC;
(j) B. Shrestha, P. Basnet, R. K. Dhungana, S. KC, S. Thapa, J. M. Sears and R. Giri, J. Am. Chem. Soc., 2017, 139, 10653–10656 CrossRef CAS PubMed;
(k) J. R. Hummel, J. A. Boerth and J. A. Ellman, Chem. Rev., 2017, 117, 9163–9227 CrossRef CAS PubMed;
(l) B. Li, K. Seth, B. Niu, L. Pan, H. Yang and H. Ge, Angew. Chem., Int. Ed., 2018, 57, 3401–3405 (
Angew. Chem.
, 2018
, 130
, 3459–3463
) CrossRef CAS PubMed;
(m) C. Sambiagio, D. Schonbauer, R. Blieck, T. Dao-Huy, G. Pototschnig, P. Schaaf, T. Wiesinger, M. F. Zia, J. Wencel-Delord, T. Besset, B. U. W. Maes and M. Schnurch, Chem. Soc. Rev., 2018, 47, 6603–6743 RSC;
(n) B. Niu, K. Yang, B. Lawrence and H. Ge, ChemSusChem, 2019, 12, 2955–2969 CrossRef CAS PubMed;
(o) D. Niroula, R. Sapkota, R. K. Dhungana, B. Shrestha and R. Giri, Isr. J. Chem., 2020, 60, 424–428 CrossRef CAS PubMed.
-
(a) M. Tobisu and N. Chatani, Science, 2014, 343, 850–851 CrossRef CAS PubMed;
(b) J. Wang and G. Dong, Chem. Rev., 2019, 119, 7478–7528 CrossRef CAS PubMed;
(c) J. A. Leitch and C. G. Frost, Chem. Soc. Rev., 2017, 46, 7145–7153 RSC;
(d) M. T. Mihai, G. R. Genov and R. J. Phipps, Chem. Soc. Rev., 2018, 47, 149–171 RSC;
(e) U. Dutta, S. Maiti, T. Bhattacharya and D. Maiti, Science, 2021, 372, eabd5992 CrossRef CAS PubMed;
(f) S. K. Sinha, S. Guin, S. Maiti, J. P. Biswas, S. Porey and D. Maiti, Chem. Rev., 2022, 122, 5682–5841 CrossRef CAS PubMed.
- For selected reviews and publications on distal C(sp2)–H functionalizations, see:
(a) A. Dey, S. K. Sinha, T. K. Achar and D. Maiti, Angew. Chem., Int. Ed., 2019, 58, 10820–10843 CrossRef CAS PubMed;
(b) S. Sasmal, U. Dutta, G. K. Lahiri and D. Maiti, Chem. Lett., 2020, 49, 1406–1420 CrossRef CAS.
- G. Meng, N. Y. S. Lam, E. L. Lucas, T. G. Saint-Denis, P. Verma, N. Chekshin and J.-Q. Yu, J. Am. Chem. Soc., 2020, 142, 10571–10591 CrossRef CAS PubMed.
- D. Leow, G. Li, T.-S. Mei and J.-Q. Yu, Nature, 2012, 486, 518–522 CrossRef CAS PubMed.
-
(a) S. Lee, H. Lee and K. L. Tan, J. Am. Chem. Soc., 2013, 135, 18778–18781 CrossRef CAS PubMed;
(b) S. Li, H. Ji, L. Cai and G. Li, Chem. Sci., 2015, 6, 5595–5600 RSC;
(c) M. Bera, A. Maji, S. K. Sahoo and D. Maiti, Angew. Chem., Int. Ed., 2015, 54, 8515–8519 (
Angew. Chem.
, 2015
, 127
, 8635–8639
) CrossRef CAS PubMed.
- S. Bag, R. Jayarajan, R. Mondal and D. Maiti, Angew. Chem., Int. Ed., 2017, 56, 3182–3186 CrossRef CAS PubMed.
-
(a) L. Chu, M. Shang, K. Tanaka, Q. Chen, N. Pissarnitski, E. Streckfuss and J.-Q. Yu, ACS Cent. Sci., 2015, 1, 394–399 CrossRef CAS PubMed;
(b) S. Li, L. Cai, H. Ji, L. Yang and G. Li, Nat. Commun., 2016, 7, 10443–10450 CrossRef PubMed;
(c) U. Dutta, A. Modak, B. Bhaskararao, M. Bera, S. Bag, A. Mondal, D. W. Lupton, R. B. Sunoj and D. Maiti, ACS Catal., 2017, 7, 3162–3168 CrossRef CAS;
(d) L. Fang, T. G. Saint-Denis, B. L. H. Taylor, S. Ahlquist, K. Hong, S. Liu, L. Han, K. N. Houk and J.-Q. Yu, J. Am. Chem. Soc., 2017, 139, 10702–10714 CrossRef CAS PubMed;
(e) L. Zhang, C. Zhao, Y. Liu, J. Xu, X. Xu and Z. Jin, Angew. Chem., Int. Ed., 2017, 56, 12245–12249 CrossRef CAS PubMed;
(f) S. Li, H. Wang, Y. Weng and G. Li, Angew. Chem., Int. Ed., 2019, 58, 18502–18507 (
Angew. Chem.
, 2019
, 131
, 18673–18678
) CrossRef CAS PubMed;
(g) S. Porey, X. Zhang, S. Bhowmick, V. Singh, S. Guin, R. S. Paton and D. Maiti, J. Am. Chem. Soc., 2020, 142, 3762–3774 CrossRef CAS PubMed;
(h) S. Bag, S. Jana, S. Pradhan, S. Bhowmick, N. Goswami, S. K. Sinha and D. Maiti, Nat. Commun., 2021, 12, 1393 CrossRef CAS PubMed;
(i) D. Srinivas and G. Satyanarayana, Org. Lett., 2021, 23, 7353–7358 CrossRef CAS PubMed;
(j) S. Li, C. Zhang, L. Fu, H. Wang, L. Cai, X. Chen, X. Wang and G. Li, CCS Chem., 2021, 3, 2360–2371 CrossRef.
- H.-J. Xu, Y. Lu, M. E. Farmer, H.-W. Wang, D. Zhao, Y.-S. Kang, W.-Y. Sun and J.-Q. Yu, J. Am. Chem. Soc., 2017, 139, 2200–2203 CrossRef CAS PubMed.
- M. Bera, S. Agasti, R. Chowdhury, R. Mondal, D. Pal and D. Maiti, Angew. Chem., Int. Ed., 2017, 56, 5272–5276 CrossRef CAS PubMed.
- H.-J. Xu, Y.-S. Kang, H. Shi, P. Zhang, Y.-K. Chen, B. Zhang, Z.-Q. Liu, J. Zhao, W.-Y. Sun, J.-Q. Yu and Y. Lu, J. Am. Chem. Soc., 2019, 141, 76–79 CrossRef CAS PubMed.
-
(a) U. Dutta, S. Maiti, S. Pimparkar, S. Maiti, L. R. Gahan, E. H. Krenske, D. W. Lupton and D. Maiti, Chem. Sci., 2019, 10, 7426–7432 RSC;
(b) R.-J. Mi, Y.-Z. Sun, J.-Y. Wang, J. Sun, Z. Xu and M.-D. Zhou, Org. Lett., 2018, 20, 5126–5129 CrossRef CAS PubMed.
-
(a)
Acetylene Chemistry, ed. F. Diederich, P. J. Stang and R. R. Tykwinski, Wiley-VCH, Weinheim, Germany, 2005 Search PubMed;
(b)
Triple Bonded Functional Groups, ed. G. V. Boyd and S. Patai, Wiley, Hoboken, NJ, 1994, ch. 5 Search PubMed.
-
(a) H. C. Kolb, M. G. Finn and K. B. Sharpless, Angew. Chem., Int. Ed., 2001, 40, 2004–2021 CrossRef CAS;
(b) A. Fürstner and P. W. Davies, Chem. Commun., 2005, 2307 (
Chem. Soc. Rev.
, 2009
, 38
, 3208–3221
) RSC;
(c) M. G. Finn and V. V. Fokin, Chem. Soc. Rev., 2010, 39, 1231 RSC;
(d) J. P. Brand and J. Waser, Chem. Soc. Rev., 2012, 41, 4165–4179 RSC;
(e) C. Obradors and A. M. Echavarren, Acc. Chem. Res., 2014, 47, 902–912 CrossRef CAS PubMed;
(f) L. Fensterbank and M. Malacria, Acc. Chem. Res., 2014, 47, 953–965 CrossRef CAS PubMed;
(g) R. Dorel and A. M. Echavarren, Chem. Rev., 2015, 115, 9028–9072 CrossRef CAS PubMed;
(h) D. Hashmi and A. S. K. Hashmi, Chem. Soc. Rev., 2016, 45, 1331–1367 RSC.
- Selected examples of Sonogashira coupling reaction:
(a) K. Sonogashira, J. Organomet. Chem., 2002, 653, 46–49 CrossRef CAS;
(b) E. Negishi and L. Anastasia, Chem. Rev., 2003, 103, 1979–2018 CrossRef CAS PubMed;
(c) A. O. King and N. Yasuda, Top. Organomet. Chem., 2004, 6, 205 CrossRef CAS;
(d) H. Doucet and J.-C. Hierso, Angew. Chem., Int. Ed., 2007, 46, 834–871 CrossRef CAS PubMed;
(e) H. Plenio, Angew. Chem., Int. Ed., 2008, 47, 6954–6956 CrossRef CAS PubMed;
(f) R. Chinchilla and C. Najera, Chem. Soc. Rev., 2011, 40, 5084–5121 RSC;
(g) R. Chinchilla and C. Nájera, Chem. Soc. Rev., 2011, 40, 5084–5121 RSC;
(h) R. Chinchilla and C. Nájera, Chem. Rev., 2014, 114, 1783–1826 CrossRef CAS PubMed;
(i) D. Wang and S. Gao, Org. Chem. Front., 2014, 1, 556–566 RSC.
- Selected examples on DG-assisted C(sp2)–H alkynylation with alkynyl halide:
(a) M. Tobisu, Y. Ano and N. Chatani, Org. Lett., 2009, 11, 3250–3252 CrossRef CAS PubMed;
(b) Y. Ano, M. Tobisu and N. Chatani, Org. Lett., 2012, 14, 354–357 CrossRef CAS PubMed;
(c) M. Shang, H.-L. Wang, S.-Z. Sun, H.-X. Dai and J.-Q. Yu, J. Am. Chem. Soc., 2014, 136, 11590–11593 CrossRef CAS PubMed;
(d) F. Xie, Z. Qi, S. Yu and X. Li, J. Am. Chem. Soc., 2014, 136, 4780–4787 CrossRef CAS PubMed;
(e) H. M.-F. Viart, A. Bachmann, W. Kayitare and R. Sarpong, J. Am. Chem. Soc., 2017, 139, 1325–1329 CrossRef CAS PubMed;
(f) C. Feng and T.-P. Loh, Angew. Chem., Int. Ed., 2014, 53, 2722–2726 (
Angew. Chem.
, 2014
, 126
, 2760–2764
) CrossRef CAS PubMed;
(g) Y.-H. Liu, Y.-J. Liu, S.-Y. Yan and B.-F. Shi, Chem. Commun., 2015, 51, 11650–11653 RSC;
(h) N. Sauermann, M. J. Gonzalez and L. Ackermann, Org. Lett., 2015, 17, 5316–5319 CrossRef CAS PubMed;
(i) Z.-Z. Zhang, B. Liu, C.-Y. Wang and B.-F. Shi, Org. Lett., 2015, 17, 4094–4097 CrossRef CAS PubMed;
(j) Y.-J. Liu, Y.-H. Liu, X.-S. Yin, W.-J. Gu and B.-F. Shi, Chem.–Eur. J., 2015, 21, 205–209 CrossRef CAS PubMed;
(k) P. Wang, G.-C. Li, P. Jain, M. E. Farmer, J. He, P.-X. Shen and J.-Q. Yu, J. Am. Chem. Soc., 2016, 138, 14092–14099 CrossRef CAS PubMed;
(l) R. Boobalan, P. Gandeepan and C.-H. Chieng, Org. Lett., 2016, 18, 3314–3317 CrossRef CAS PubMed;
(m) V. G. Landge, G. Jaiswal and E. Balaraman, Org. Lett., 2016, 18, 812–815 CrossRef CAS PubMed;
(n) Z. Ruan, S. Lackner and L. Ackermann, ACS Catal., 2016, 6, 4690–4693 CrossRef CAS;
(o) Z. Ruan, N. Sauermann, E. Manoni and L. Ackermann, Angew. Chem., Int. Ed., 2017, 56, 3172–3176 CrossRef CAS PubMed;
(p) E. Tan, O. Quinonero, M. Elena de Orbe and A. M. Echavarren, ACS Catal., 2018, 8, 2166–2172 CrossRef CAS PubMed , and the references therein..
- Selected examples on C(sp2)–H alkynylation other than DG-assistance:
(a) I. V. Seregin, V. Ryabova and V. Gevorgyan, J. Am. Chem. Soc., 2007, 129, 7742–7743 CrossRef CAS PubMed;
(b) J. P. Brand, J. Charpentier and J. Waser, Angew. Chem., Int. Ed., 2009, 48, 9346–9349 (
Angew. Chem.
, 2009
, 121
, 9510–9513
) CrossRef CAS PubMed;
(c) N. Matsuyama, K. Hirano, T. Satoh and M. Miura, Org. Lett., 2009, 11, 4156–4159 CrossRef CAS PubMed;
(d) T. de Haro and C. Nevado, J. Am. Chem. Soc., 2010, 132, 1512–1513 CrossRef CAS PubMed;
(e) A. S. Dudnik and V. Gevorgyan, Angew. Chem., Int. Ed., 2010, 49, 2096–2098 CrossRef CAS PubMed;
(f) L. Ackermann, C. Kornhaass and Y. Zhu, Org. Lett., 2012, 14, 1824–1826 CrossRef CAS PubMed , and the references therein..
- For detailed information, see the ESI.†.
|
This journal is © The Royal Society of Chemistry 2022 |