DOI:
10.1039/D2SC00758D
(Edge Article)
Chem. Sci., 2022,
13, 4821-4827
Site-selective amination and/or nitrilation via metal-free C(sp2)–C(sp3) cleavage of benzylic and allylic alcohols†
Received
7th February 2022
, Accepted 4th April 2022
First published on 5th April 2022
Abstract
Benzylic/allylic alcohols are converted via site-selective C(sp2)–C(sp3) cleavage to value-added nitrogenous motifs, viz., anilines and/or nitriles as well as N-heterocycles, utilizing commercial hydroxylamine-O-sulfonic acid (HOSA) and Et3N in an operationally simple, one-pot process. Notably, cyclic benzylic/allylic alcohols undergo bis-functionalization with attendant increases in architectural complexity and step-economy.
Introduction
In recognition of increasingly strict conservational and regulatory imperatives,1 much attention is now focused upon innovative functionalization methodologies2 that are applicable to more readily available and economic feedstocks,3 while simultaneously emphasizing environmentally friendly, especially metal-free, reaction conditions.4 To these ends, there has been encouraging progress recently in the application of site-selective C(sp2)–C(sp3) and C(sp2)–C(sp2) cleavage strategies,5 despite the comparatively high bond dissociation energy of most unstrained C–C bonds and the formidable challenges posed by discriminating amongst otherwise chemically equivalent C–C bonds6 (Fig. 1).
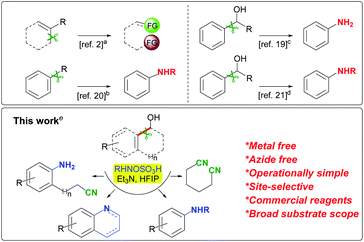 |
| Fig. 1 Representative site-selective functionalizations via C(sp2)–C(sp3) and C(sp2)–C(sp2) cleavage. aSelect examples of olefin α-cleavage. bAlkyl- and alkene-aryl cleavages. cC(sp2)–C(sp3) cleavages of acyclic benzyl alcohols to 1° anilines. dC(sp2)–C(sp3) cleavages of acyclic benzyl alcohols leading to 1° & 2° anilines. eThis work: C(sp2)–C(sp3) cleavage of benzylic and allylic alcohols delivering anilines and/or nitriles or N-heterocycles. FG = functional group. | |
Canonical syntheses of anilines7 and other nitrogen containing functional groups are often trammeled by multi-step sequences,8 harsh reaction conditions,9 limited scope,10 and/or lack of regioselectivity. Given the continuing interest in nitrogenous compounds,11 especially anilines12 and nitriles, by the pharmaceutical, dye, agricultural, and specialty materials markets, several novel, site-selective procedures have been introduced, intra alia, decarboxylative aminations,13 transition metal cross-couplings (e.g., Buchwald–Hartwig,14 Chan–Lam15), electrophilic amination reagents,16 ligand-directed aminations,17 and boronate rearrangement.18 More recently, influential examples from the Jiao19,20 and Hashmi21 laboratories pioneered efficient, site-specific C–C cleavages of benzylic alcohols, alkylarenes and styrenes to anilines. Herein, we describe the operationally simple, one-pot, site-selective C(sp2)–C(sp3) cleavage of benzylic/allylic alcohols using commercial hydroxylamine-O-sulfonic acid (HOSA)22 and Et3N to prepare value-added nitrogenous motifs, viz., anilines and/or nitriles in addition to N-heterocycles. Of particular note are applications to cyclic alcohols that result in either symmetrical or unsymmetrical bis-functionalization with their attendant increases in architectural complexity and step-economy.
Results and discussion
Prompted by the above C–C cleavage reports and our prior aryl and alkene amination studies,17a,18,23 we foresaw (i) the untapped potential to develop novel bis-functionalization applications with attendant improvements in architectural complexity and step-economy, (ii) extension to an additional substrate class, i.e., allylic alcohols, in addition to alkylarenes/styrenes, and (iii) utilization of a more acceptable aminating reagent. To these ends, a mixture of model substrate 1-tetrahydronaphthol (1a, α-tetralol) and aminating reagent hydroxylamine-O-sulfonic acid (HOSA) was screened with a variety of organic/inorganic bases in several common solvents (see ESI†) and discovered to give anilino-nitrile 2a, whose differentiated nitrogen functionality was deemed to hold considerable synthetic potential. Following optimization of reagent ratios (see ESI†), the isolated yield of 2a was raised to 92% using equimolar HOSA and Et3N in 1,1,1,3,3,3-hexafluoroisopropanol (HFIP) at rt (Table 1); while CsOH as base was comparable to Et3N, its deliquescence precluded routine use. These studies also revealed that a base for deprotonation of zwitterionic HOSA is crucial for reaction (entry 6). No or little reaction occurred in MeOH, CH2Cl2 or THF. For α-tetralol (1a, Table 1), the observed migratory aptitude (aryl ≫ alkyl) correlated with well established migratory aptitudes. Application of these conditions to seven-membered 2-methyl-α-tetralol (1c), and 4-(3,4-dichlorophenyl)-α-tetralol (1d) likewise gave good yields of the corresponding anilino-nitriles 2b–d; of interest, 2d's benzhydryl hydrogen proved stable. Substituents on the aromatic ring also were well tolerated ranging from electron donating groups, viz., free phenol (1e), methoxy (1f), silyl ether (1g), allyl ether (1h) and benzyl ether (1i) to halogens (1j,k) and even the more powerful electron withdrawing groups nitro (1l) and methoxycarbonyl (1m), although the latter two alcohols needed gentle warming to achieve acceptable reaction rates. As an indication of the mild reaction conditions, the labile cis-stilbene 2n was obtained from dibenzosuberenol (1n) without isomerization to the thermodynamically more stable trans-isomer. Other sensitive and/or polyfunctional molecules that proved compatible with the bis-functionalization protocol are evident in 9H-fluoren-9-amide 2o, seco-estradiol dibenzoate 2p, Cbz-protected dipeptide 2q, thiazole 2r, and pyrazine 2s, all of which were realized in good yields. The scope of the site-selective C–C cleavage was further explored using readily available acyclic benzyl alcohols leading to the corresponding anilines (Table 2). Access to aniline itself (4a) and N-methylaniline (4a′) from 1-phenylethanol 3a proceeded smoothly (71% and 72% overall yield, respectively) using HOSA and N-methylhydroxylamine-O-sulphonic acid,24 respectively. Additional substitution at the benzylic position capable of supporting a carbocation, e.g., benzhydrol (3a′) and 2-phenylisopropanol (3a′′), accelerated the reaction rate; the non-migrating phenyl of 3a′ was obtained as a 2
:
1 mixture of benzaldehyde and benzonitrile (54%). Also, moderate to strong electron donating aryl substituents were also more reactive as illustrated in 4b–f, 4s while simultaneously offering comparatively better yields vis-à-vis the hydrazoic acid based procedure;19,20 even the easily oxidized p-phenylenediamines 4g,h were well behaved. Additionally, examples containing 2-naphthyl 4i, biphenyl 4j, and quinoline 4k as well as typical electron withdrawing substituents such as acetamide 4l, bromide 4m, and nitro 4n delivered the corresponding anilines without incident. For validation within the context of polyfunctional bioactive scaffolds, benzyl alcohols derived from acebutolol, iloperidone, and adapalene were converted to anilines 4o–q. To better understand the reaction course, 3r was treated with 1 equivalent each of HOSA and Et3N in HFIP as solvent resulting in 4r (45%) and 4-phenylcyclohexanone (38%), most likely arising from hydrolysis of the intermediate imine. As might be expected, acid sensitive functionality such as acetonides and epoxides are not stable under the moderately acidic reaction conditions.
Table 1 Optimization table of cyclic benzyl alcohols via C–C cleavagea
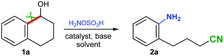
|
Entry |
Photocatalyst (mol%) blue LED (470 nm) |
Solvent |
Base |
Temp. (°C) |
Time (h) |
Yield (%) |
See ESI for additional details.
|
1 |
[Ir{dF(CF3)ppy}2(dtbpy)PF6 (2) |
HFIP |
Et3N |
— |
1 |
72 |
2 |
Eosin-Y |
HFIP |
Et3N |
— |
1 |
70 |
3 |
— |
HFIP |
Et3N |
60 |
1 |
66 |
4 |
[Ir{dF(CF3)ppy}2(dtbpy)]PF6 (2) |
CH2Cl2 |
Et3N |
— |
24 |
0 |
5
|
—
|
HFIP
|
Et
3
N
|
23
|
14
|
92
|
6 |
— |
HFIP |
— |
23 |
14 |
0 |
7 |
— |
HFIP |
Pyridine |
23 |
14 |
79 |
8 |
— |
HFIP |
DMAP |
23 |
14 |
85 |
9 |
— |
TFE |
Et3N |
60 |
24 |
42 |
10 |
— |
THE |
Et3N |
60 |
24 |
0 |
11 |
— |
MeOH |
Et3N |
60 |
24 |
<5 |
12 |
— |
CH2Cl2 |
Et3N |
60 |
24 |
<5 |
Table 2 Bis-functionalization of cyclic benzyl alcohols via C–C cleavagea,b
Reactions conditions: benzyl alcohol (0.5 mmol), H2NOSO3H (2.2 equiv.), Et3N (2.2 equiv.) at 0.15 M in hexafluoroisopropanol (HFIP) under argon.
Isolated yields.
5 mmol scale. H2NOSO3H: hydroxylamine-O-sulfonic acid; HFIP: 1,1,1,3,3,3-hexafluoroisopropanol.
|
|
Extension of the bis-functionalization repertoire to cyclic allylic alcohol 5a gave rise to adiponitrile (6a), an industrially important commodity, whereas acyclic 5b led to 2-phenylacetonitrile (6b) (Table 3). On the other hand, a 1
:
2 mixture of 3-phenylpropnitrile (6c) and 4-phenylbutyronitrile (6c′) was obtained from 5c, although in good combined yield. Similarly, a 1
:
1.3 mixture of undecanenitrile (6d) and dodecanenitrile (6d′) was observed coming from 5d reflecting the competing influences of charge distribution in the allylic carbocation vs. steric approach of HOSA. For trisubstituted allylic alcohol 5e, nitrile 6e was the minor product and Beckmann lactam 6e′, resulting from initial addition of HOSA to the tertiary center, was favored. For some cyclic benzylic alcohols (Table 4), arene migration to the HOSA nitrogen (Fig. 2) directly resulted in a stable aromatic system, e.g., phenanthridene (7a) from 9-hydroxyfluorene and dibenzoxazepine (7b) dibenzoxazepine (7b) from xanthydrol. In other systems, aromatization occurred following in situ oxidation of the intermediate imine, e.g., quinoline (7c) from 1-indanol and substituted quinoline 7d; the former via exposure to air during the course of the reaction and the latter induced by HOSA, itself a mild oxidant. Alternatively, the process can be paused after the first rearrangement step by restricting the amount of HOSA/Et3N and the newly generated imine reduced in situ. For instance, the one-pot, sequential treatment of 1a with just 1.2 equivalents each of HOSA and Et3N, followed by sodium cyanoborohydride after 1a was consumed, produced 1H-tetrahydrobenzazepine (7e) in 71% yield accompanied by 4a (4%); 2-methyl-1H-tetrahydrobenzazepine (7f; 91%) was secured analogously from 1-methyl-1-tetrahydronaphthol (Table 5).
Table 3 Synthesis of anilines via C–C cleavage of acyclic benzyl alcoholsa,b
Reactions conditions: benzyl alcohol (0.5 mmol), H2NOSO3H (1.5 equiv.), Et3N (1.5 equiv.) at 0.15 M in hexafluoroisopropanol (HFIP) under argon.
Isolated yields.
For convenience, isolated as the N-acetamide; overall yield for C–C cleavage and N-acylation.
MeHNOSO3H (3 equiv.) and Et3N (1.5 equiv.).
3 equiv. each of H2NOSO3H and Et3N.
1.5 equiv. each of H2NOSO3H and Et3N gave 4m (33%) and unreacted starting material (51%).
1.5 equiv. each of H2NOSO3H and Et3N gave 4n (21%) and unreacted starting material (68%).
Using 1 equiv. each of H2NOSO3H and Et3N, 4-phenylcyclohexanone was also isolated in 38% yield.
Using 1.5 equiv. each of H2NOSO3H and Et3N, 4-phenylcyclohexanone was also isolated in 45% yield.
|
|
Table 4 Mono-/bis-nitriles via C–C cleavage of allylic alcoholsa,b
Reactions conditions: allylic alcohol (0.5 mmol), HOSA (4.0 equiv.), Et3N (4.0 equiv.) at 0.15 M in hexafluoroisopropanol (HFIP) under argon.
Isolated yields.
Ratio determined via1H NMR.
Ratio determined via LC/HRMS.
|
|
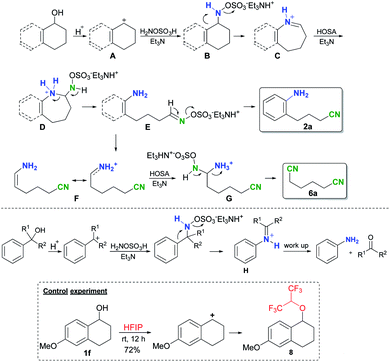 |
| Fig. 2 Plausible bis-functionalization mechanism. Illustrated for (i) α-tetralol (1 → 2a) and 1-cyclohexenol (5a → 6a) (ii) plausible mechanism for acyclic benzyl alcohols and (iii) control experiment consistent with carbocation intermediacy. | |
Table 5 N-Heterocycles from cyclic benzyl alcohols via C–C cleavagea,b
Reactions conditions: isolated yields.
Benzyl alcohol (0.5 mmol), HOSA (2.2 equiv.), Et3N (2.2 equiv.) at 0.15 M in HFIP under argon.
Conducted open to atmosphere.
Used (i) HOSA (1.2 equiv.) and Et3N (1.2 equiv.), 0 °C; (ii) NaBH3CN (2 equiv.), rt.
|
|
Control experiments (see ESI†) proved instructive in understanding the plausible mechanism. For example, 2f in HFIP (0.15 M) at rt, but in the absence of HOSA and TEA, formed the corresponding HFIP ether 8 overnight, consistent with the formation of a carbocation intermediate. Treatment of 1a with butylated hydroxytoluene (BHT) under our standard reaction condition resulted in no change in the yield of 2a which doesn't support a long lived radical mechanism. On the other hand, the yield of 2a was significantly decreased in the presence of 2 equiv. of TEMPO; however, control experiments suggested that the HOSA is decomposed by TEMPO under our reaction conditions (see ESI†).
Fig. 2 presents a generalized, mechanistic scenario by which benzylic and allylic alcohols undergo bis-functionalization either type of alcohol is readily converted to the corresponding hydroxylamine-O-sulfonate trimethylamine salt Bvia stabilized carbocation A. Subsequent rearrangement of B forms iminium C that in turn forms D upon addition of a second equivalent of triethylammonium HOSA. For benzyl alcohols, a quick succession of eliminations, firstly to aldoxime E by collapse of the aminal in D, and finally yields anilino-nitrile (illustrated by 2a) via loss of sulfate and a proton. If an allylic alcohol is the substrate, E leads to imine-enamine F that eventually terminates at bis-nitrile (illustrated by 6a) following an addition/elimination sequence similar to the one that led to 2a.
For acyclic benzyl alcohols, iminium intermediate H provided the corresponding anilines and aldehydes/ketones upon aqueous isolation.
Conclusions
The foregoing one-pot methodology exploits the site-selectivity of C(sp2)–C(sp3) cleavage of benzylic and allylic alcohols for non-hazardous, metal-free access to anilines and/or nitriles or, most notably for cyclic systems, to anilino-nitriles and bis-nitriles, respectively, with highly advantageous step and atom efficacy. In preliminary studies, we also sought to extend our methodology to substrates that are otherwise refractory and for this the reaction conditions were conflated with prior methodology.19,25 Proof of principle was gained using the otherwise unreactive benzyl alcohol (3t) that was converted with commercial N-tBoc-O-tosylhydroxylamine under Mitsunobu conditions to O-tosyloxime 9 that subsequently underwent rearrangement to aniline (4a) under the influence of trifluoroacetic acid in methanol (eqn (1a), isolated as the N-acetamide) (Table 6).
Table 6 Synthesis of anilines via C–C cleavage of alkylarenes and primary benzyl alcohols
Unexpectedly, N-heterocycle (10) was observed (see ESI†) when 9 was stirred with excess TFA in HFIP as solvent (eqn (1b)), presumably involving three successive transformations (C–C cleavage, decarboxylation and rearrangement). α-Unfunctionalized alkylarenes, e.g., 11, are also inert to the standard reaction conditions, but could be coaxed to rearrange using a mixture of DDQ and HOSA/Et3N (eqn (2)). Detailed studies will appear elsewhere.
Data availability
Data for this work, including optimization tables, general experimental procedures and characterization data for all new compounds are provided in the ESI.†
Author contributions
R. R. A. conceptualized the project, conducted the experiments, analyzed the data and wrote the original draft. J. R. F. provide project supervision and manuscript review.
Conflicts of interest
The authors declare no competing financial interests.
Acknowledgements
Financial support provided by the Robert A. Welch Foundation (I-0011) and USPHS NIH (RO1 HL139793, DK126452). Dedicated to Prof. Dong-Soo Shin, Changwon National University, a much admired educator, innovative researcher and collaborator. Dr Adeniyi Michael Adebesin and Sailu Munnuri are thanked for advice and critical commentary.
Notes and references
- A. Ivankovic, A. Dronjic, A. M. Bevanda and S. Talic, Int. J. Sustainable Green Energy, 2017, 6, 39–48 CrossRef CAS.
-
(a) A. J. Smalingo, M. Swain, J. C. Quintana, M. F. Tan, D. A. Kim and O. Kwon, Science, 2019, 364, 681–685 CrossRef PubMed;
(b) A. J. Smaligo and O. Kwon, Org. Lett., 2019, 21, 8592–8597 CrossRef CAS PubMed;
(c) A. J. Smaligo, J. Wu, N. R. Burton, A. S. Hacker, A. C. Shaikh, J. C. Quintana, R. Wang, C. Xie and O. Kwon, Angew. Chem., Int. Ed., 2020, 59, 1211–1215 CrossRef CAS PubMed;
(d) T.-L. Liu, J. E. Wu and Y. Zhao, Chem. Sci., 2017, 8, 3885–3890 RSC;
(e) P. R. Leger, Y. Kuroda, S. Chang, J. Jurczyk and R. Sarpong, J. Am. Chem. Soc., 2020, 142, 15536–15547 CrossRef CAS PubMed.
-
(a) A. Rahimi, A. Ulbrich, J. J. Coon and S. S. Stahl, Nature, 2014, 515, 249–252 CrossRef CAS PubMed;
(b) E. Subbotina, T. Rukkijakan, M. D. Marquez-Medina, X. Yu, M. Johnsson and J. S. M. Samec, Nat. Chem., 2021, 13, 1118–1125 CrossRef CAS PubMed;
(c) A. Rahimi, A. Azarpira, H. Kim, J. Ralph and S. S. Stahl, J. Am. Chem. Soc., 2013, 135, 6415–6418 CrossRef CAS PubMed;
(d) X. Wu and C. Zhu, Chem. Rec., 2018, 18, 587–598 CrossRef CAS PubMed;
(e) R. Maa, M. Guoa and X. Zhang, Catal. Today, 2018, 302, 50–60 CrossRef.
-
(a) W. Lossen, Ann. Chem. Pharm., 1872, 161, 347–362 CrossRef;
(b) H. L. Yale, Chem. Rev., 1943, 33, 209–256 CrossRef CAS;
(c) A. W. Hofmann, Ber. Dtsch. Chem. Ges., 1881, 14, 2725–2736 CrossRef;
(d) X.-W. Lan, N.-X. Wang and Y. Xing, Eur. J. Org. Chem., 2017, 5821–5851 CrossRef CAS;
(e) S. Donikela, P. S. Mainkar, K. Nayani and S. Chandrasekhar, J. Org. Chem., 2019, 84, 10546–10553 CrossRef CAS PubMed;
(f) R. Kranthikumar, R. Chegondi and S. Chandrasekhar, J. Org. Chem., 2016, 81, 2451–2459 CrossRef CAS PubMed.
-
(a) Z. Q. Lei, F. Pan, H. Li, Y. Li, X. S. Zhang, K. Chen, X. Wang, Y. X. Li, J. Sun and Z. J. Shi, J. Am. Chem. Soc., 2015, 137, 5012–5020 CrossRef CAS PubMed;
(b) X. Huang, X. Li, M. Zou, S. Song, C. Tang, Y. Yuan and N. Jiao, J. Am. Chem. Soc., 2014, 136, 14858–14865 CrossRef CAS PubMed;
(c) C. Tang and N. Jiao, Angew. Chem., Int. Ed., 2014, 53, 6528–6532 CrossRef CAS PubMed;
(d) C. Zhang, P. Feng and N. Jiao, J. Am. Chem. Soc., 2013, 135, 15257–15262 CrossRef CAS PubMed;
(e) L. Souillart, E. Parker and N. Cramer, Angew. Chem., Int. Ed., 2014, 53, 3001–3005 CrossRef CAS PubMed;
(f) X.-Y. Yu, J.-R. Chen and W.-J. Xiao, Chem. Rev., 2021, 121, 506–561 CrossRef CAS PubMed;
(g) K. Nogi and H. Yorimitsu, Chem. Rev., 2021, 121, 345–364 CrossRef CAS PubMed;
(h) J. Y. Becker, L. R. Byrd, L. L. Miller and Y.-H. So, J. Am. Chem. Soc., 1975, 97, 849–853 CrossRef CAS;
(i) X. Zhou, Y. Xu and G. Dong, J. Am. Chem. Soc., 2021, 143, 20042–20048 CrossRef CAS PubMed.
-
(a) D. F. McMillen and D. M. Golden, Ann. Rev. Phys. Chem., 1982, 33, 493–532 CrossRef CAS;
(b) J. J. Brocks, H. D. Beckhaus, A. L. Beckwith and C. Rüchardt, J. Org. Chem., 1998, 63, 1935–1943 CrossRef CAS.
-
(a) T. Curtius, Ber. Dtsch. Chem. Ges., 1890, 23, 3023–3033 CrossRef;
(b) H. Wolff, Organic Reactions, 2011, 307–336 Search PubMed;
(c) R. G. Wallace, J. M. Barker and M. L. Wood, Synthesis, 1990, 1143–1144 CrossRef CAS;
(d) E. Beckmann, Ber. Dtsch. Chem. Ges., 1886, 19, 988–993 CrossRef;
(e)
R. E. Gawley, Organic Reactions, 1988, pp. 1–247 Search PubMed.
- K. Hyodo, G. Hasegawa, H. Maki and K. Uchida, Org. Lett., 2019, 21, 2818–2822 CrossRef CAS PubMed.
-
F. Chen, CN108047059A, 2018.
- E. H. Huntress and H. C. Walter, J. Am. Chem. Soc., 1948, 70, 3702–3707 CrossRef CAS PubMed.
-
(a) Y. Park, Y. Kim and S. Chang, Chem. Rev., 2017, 117, 9247–9301 CrossRef CAS PubMed;
(b) J. Jiao, K. Murakami and K. Itami, ACS Catal., 2016, 6, 610–633 CrossRef CAS.
-
(a) B. J. Wood, M. J. Walter and J. Wade, Nature, 2006, 441, 825–833 CrossRef CAS PubMed;
(b) U. Mann, D. J. Frost, D. C. Rubie, H. Becker and A. Audetat, Geochim. Cosmochim. Acta, 2012, 84, 593–613 CrossRef CAS;
(c) R. J. Walker, K. Bermingham, J. Liu, I. S. Puchtel, M. Touboul and E. A. Worsham, Chem. Geol., 2015, 411, 125–142 CrossRef CAS.
- S. Arshadi, S. Ebrahimiasl, A. Hosseinian, A. Monfared and E. Vessally, RSC Adv., 2019, 9, 8964–8976 RSC.
- M. M. Heravi, Z. Kheilkordi, V. Zadsirjan, M. Heydari and M. Malmir, J. Organomet. Chem., 2018, 861, 17–104 CrossRef CAS.
- J. C. Vantourout, H. N. Miras, A. Isidro-Llobet, S. Sproules and A. J. B. Watson, J. Am. Chem. Soc., 2017, 139, 4769–4779 CrossRef CAS PubMed.
-
(a) H. Gao, Z. Zhou, D.-H. Kwon, J. Coombs, S. Jones, N. E. Behnke, D. H. Ess and L. Kürti, Nat. Chem., 2017, 9, 681–688 CrossRef CAS PubMed;
(b) Z. Zhou, Z. Ma, N. E. Behnke, H. Gao and L. Kürti, J. Am. Chem. Soc., 2017, 139, 115–118 CrossRef CAS PubMed.
-
(a) R. R. Anugu, S. Munnuri and J. R. Falck, J. Am. Chem. Soc., 2020, 142, 5266–5271 CrossRef CAS PubMed;
(b) P. Wang, G.-C. Li, P. Jain, M. E. Farmer, J. He, P.-X. Shen and J.-Q. Yu, J. Am. Chem. Soc., 2016, 138, 14092–14099 CrossRef CAS PubMed.
- C. Zhu, G. Li, D. H. Ess, J. R. Falck and L. Kürti, J. Am. Chem. Soc., 2012, 134, 18253–18256 CrossRef CAS PubMed.
- J. Liu, X. Qiu, X. Huang, X. Luo, C. Zhang, J. Wei, J. Pan, Y. Liang, Y. Zhu, Q. Qin, S. Song and N. Jiao, Nat. Chem., 2019, 11, 71–77 CrossRef CAS PubMed.
-
(a) Y. Adeli, K. Huang, Y. Liang, Y. Jiang, J. Liu, S. Song, C. C. Zeng and N. Jiao, ACS Catal., 2019, 9, 2063–2067 CrossRef CAS;
(b) J. Liu, J. Pan, X. Luo, X. Qiu, C. Zhang and N. Jiao, Research, 2020, 2020, 7947029 CAS.
- T. Wang, P. M. Stein, H. Shi, C. Hu, M. Rudolph and A. S. K. Hashmi, Nat. Commun., 2021, 12, 7029 CrossRef CAS PubMed.
-
(a) Z. Ma, Z. Zhou and L. Kürti, Angew. Chem., Int. Ed., 2017, 56, 9886–9890 CrossRef CAS PubMed;
(b) H. C. Brown, W. R. Heydkamp, E. Breuer and W. S. Murphy, J. Am. Chem. Soc., 1964, 86, 3565–3566 CrossRef CAS;
(c) Q. Q. Cheng, Z. Zhou, H. Jiang, J. H. Siitonen, D. H. Ess, X. Zhang and L. Kürti, Nature Catalysis, 2020, 3, 386–392 CrossRef CAS;
(d) S. Voth, J. W. Hollett and J. A. McCubbin, J. Org. Chem., 2015, 80, 2545–2553 CrossRef CAS PubMed;
(e) C. Zhu, G. Li, D. H. Ess, J. R. Falck and L. Kürti, J. Am. Chem. Soc., 2012, 134, 18253–18256 CrossRef CAS PubMed.
- S. Munnuri, R. R. Anugu and J. R. Falck, Org. Lett., 2019, 21, 1926–1929 CrossRef CAS PubMed.
- A. E. Strom and J. F. Hartwig, J. Org. Chem., 2013, 78, 8909–8914 CrossRef CAS PubMed.
- M. P. Paudyal, A. M. Adebesin, S. R. Burt, D. H. Ess, Z. Ma, L. Kürti and J. R. Falck, Science, 2016, 353, 1144–1147 CrossRef CAS PubMed.
|
This journal is © The Royal Society of Chemistry 2022 |