DOI:
10.1039/D2SC00036A
(Edge Article)
Chem. Sci., 2022,
13, 6519-6524
Direct visible-light-induced synthesis of P-stereogenic phosphine oxides under air conditions†
Received
5th January 2022
, Accepted 11th April 2022
First published on 25th April 2022
Abstract
Over the past two decades, visible-light-induced transformations have been regarded as being among the most environmentally benign and powerful strategies for constructing complex molecules and diverse synthetic building blocks in organic synthesis. However, the development of efficient photochemical processes for assembling enantiomerically pure molecules remains a significant challenge. Herein, we describe a simple and efficient visible-light-induced C–P bond forming reaction for the synthesis of P-chiral heteroaryl phosphine oxides in moderate to high yields with excellent ee values (97–99% ee). Even in the absence of transition metal or photoredox catalysts, a variety of P-chiral heteroaryl phosphine oxides, including chiral diphosphine oxide 41, have been directly obtained under air conditions. Density functional theory (DFT) calculations have shown that the reaction involves intersystem crossing and single electron transfer to give a diradical intermediate under visible light irradiation.
Introduction
P-stereogenic phosphorus compounds are valuable ligands for enantioselective metal-catalysed reactions, that are usually used in the synthesis of pharmaceuticals and agrochemicals.1 In general, the synthesis of P-stereogenic phosphorus compounds frequently relies on auxiliary-based or resolution processes.2 These methods usually require the use of a stoichiometric amount of a chiral reagent under harsh reaction conditions, and often suffer from limited substrate scope. As more efficient alternatives, several metal-catalysed asymmetric reactions of secondary phosphines or phosphine oxides have been developed for the construction of P-chiral compounds in recent years (Scheme 1).3–5 However, such metal-catalysed reactions still suffer from drawbacks, such as high loadings of expensive metal catalysts and chiral ligands, and reactions need to be carried out under an atmosphere of argon or nitrogen. With these limitations in mind, it is still important to develop distinct and robust strategies that provide convenient access to a wide range of P-chiral compounds.
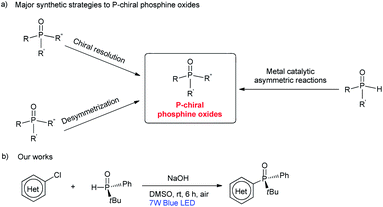 |
| Scheme 1 Synthesis of P-chiral phosphine oxides. | |
Visible-light-driven organic reactions have emerged as a powerful strategy for constructing C–E bonds (E = C, N, P, etc.) under mild and ecologically benign conditions.6 However, the high energy intermediates generated in photo-induced transformations often have very short lifetimes and can be difficult to stereo-control with exogenous catalysts. The application of visible-light-driven transformations to access chiral compounds is a real challenge. Over the past decade, many light-induced asymmetric transformations have been developed that operate in the presence of dual catalysis systems or a single bifunctional catalyst.7 Despite these significant achievements, one might concede that efficient methods for the visible-light-induced synthesis of P-chiral compounds have yet to be described. Herein, we disclose our results on the visible-light-promoted C–P cross-coupling of heteroaryl chlorides with (R)-tert-butyl(phenyl)phosphine oxide through a diradical pathway. This reaction can be performed in the absence of a transition metal or photo-redox catalyst under air conditions. In this way, we have succeeded in preparing a series of P-chiral heteroaryl phosphine oxides with high ee values (ee ≥ 97%).
Our group has long sought to develop efficient strategies for producing phosphine compounds.4h,8 In 2018, we found that unsymmetric tert-butyl(phenyl)phosphine oxide (rac-1) reacted with 2-chloropyridine to afford rac-2 in 54% yield in the presence of tBuOK under visible-light irradiation (Table 1, entry 1).8b Subsequently, we screened the reaction conditions by employing other solvents. When conducted in DMSO, toluene, DMF, and THF, the reaction gave rac-2 in yields of 59%, 28%, 27%, and 40%, respectively (Table 1, entries 2–5). No product was observed using CH2Cl2 as solvent (Table 1, entry 6). Next, screening of bases revealed that NaOH was optimal in DMSO.
Table 1 Optimization of the reaction conditionsa,b
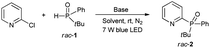
|
Entry |
Base |
Solvent |
Time (h) |
Yieldb (%) |
Reaction conditions: 2-chloropyridine (0.24 mmol), tert-butyl(phenyl)phosphine oxide (0.20 mmol) and base (0.3 mmol) in solvent (1 mL) at room temperature under N2 and blue LED irradiation (7 W).
Based on 31P NMR.
Isolated yield.
Under air.
Reaction performed in the absence of light.
|
1 |
tBuOK |
CH3CN |
18 |
54 |
2 |
tBuOK |
DMSO |
18 |
59 |
3 |
tBuOK |
Toluene |
18 |
28 |
4 |
tBuOK |
DMF |
18 |
27 |
5 |
tBuOK |
THF |
18 |
40 |
6 |
tBuOK |
DCM |
18 |
<1 |
7 |
tBuONa |
DMSO |
18 |
80 |
8 |
tBuOLi |
DMSO |
18 |
<1 |
9 |
NaOH |
DMSO |
18 |
87 |
10 |
Na2CO3 |
DMSO |
18 |
<1 |
11 |
NaOH |
DMSO |
6 |
87 (84c,d) |
12 |
NaOH |
DMSO |
18 |
<1e |
No reaction occurred when using tBuOLi or Na2CO3 as bases (Table 1, entries 7–10). The reaction time could be reduced to 6 h under air, with rac-2 being isolated in 84% yield (Table 1, entry 11). Additionally, the expected product rac-2 was not obtained in the absence of light (Table 1, entry 12). Based on these results, we surmised that chiral heteroaryl phosphine oxides could probably be constructed by this method. We proceeded to examine the applicability of the standard conditions for the reaction of (R)-tert-butyl(phenyl)phosphine oxide (3) with 2-chloropyridine. The desired chiral product 4 was obtained in 84% yield with 98% ee. The use of other solvents, such as DMF, toluene, and THF, resulted in 8–44% yields with 98% ee, revealing that solvent had no influence on the ee value (Table 2, entries 1–5). The reaction also gave product 4 in 52% yield with 96% ee at 120 °C in the absence of light (Table 2, entry 6). Reactions with 2-bromopyridine and 2-iodopyridine afforded 4 in yields of 75% and 27%, respectively, both with 98% ee (Table 3).
Table 2 The reaction of 2-chloropyridine with 3 in different solventsa,b
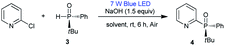
|
Entry |
Solvent |
Yieldb (%) |
ee (%) |
Reaction conditions: 2-chloropyridine (0.24 mmol), 3 (0.20 mmol) and NaOH (0.3 mmol) in solvent (1 mL) at room temperature under blue LED irradiation (7 W).
Based on 31P NMR.
Isolated yield.
Reaction performed at 120 °C in the absence of light.
|
1 |
DMSO |
87 (84c) |
98 |
2 |
Toluene |
8 |
98 |
3 |
DMF |
44 |
98 |
4 |
THF |
29 |
98 |
5 |
CH3CN |
<1 |
— |
6d |
DMSO |
52 |
96 |
Table 3 Visible-light-induced phosphinylation of pyridinyl halides with compound 3a,b
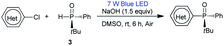
|
Reaction conditions: pyridinyl chlorides (0.6 mmol), 3 (0.50 mmol) and NaOH (0.75 mmol) in DMSO (2 mL) under blue LED irradiation (7 W) for 6 h at room temperature.
Isolated yield.
2-Bromopyridine as a substrate.
2-Iodopyridine as a substrate.
|
|
Results and discussion
Having determined the optimal conditions, the substrate scope of the reaction was explored. As shown in Table 3, 2-chloropyridines bearing methyl, methoxy, phenyl, fluoro, chloro, and trifluoromethyl groups were phosphinylated to give the desired chiral products 5–9 and 12–15 in moderate to good yields (32–86%) with excellent ee values (97–99%). 2-Chloro-6-methylpyridine, 2-chloro-5-methylpyridine, and 2-chloro-3-methylpyridine gave 5, 6, and 8 in yields of 86%, 79%, and 32%, respectively, revealing that steric effects hinder the transformation. The expected products 10 and 11 were scarcely observed following the reactions of substrates bearing amino and hydroxymethyl groups. The partial deprotonation of –NH2 and –OH groups probably deactivate the substrates in the presence of NaOH. On the other hand, 3-chloropyridine, 5-chloro-2-methylpyridine, and 4-chloro-2,6-dimethylpyridine were well tolerated, and the expected products 16–18 were obtained in yields of 50–73% with ee values of 98–99%.
Next, the visible-light-induced C–P bond forming reaction was extended to other heteroaryl chlorides. Chloroquinolines, 1-chloroisoquinoline, 2-chloropyrazines, and 2-chloropyrimidines were also successfully phosphinylated to afford the expected products 19–30 in yields of 50–88% with 97–99% ee. Remarkably, heteroaryl chlorides based on bipyridyl, terpyridyl, and 1,10-phenanthrolinyl cores also proved to be compatible with the standard conditions, giving the expected products 31–35 in 55–90% yields with 97–99% ee (Table 4). Meanwhile, diphosphinylated product was not found in the reaction of 2,6-dichloropyrazine with 1 equivalent of 3, but diphosphinylated product 41 was obtained in 17% yield with 99% ee in the reaction of 2,9-dichloro-1,10-phenanthroline with 1 equivalent of 3. However, the expected products 36 and 37 were not found in the reactions of 2-bromothiophene and 2-bromofuran. When methyl(phenyl)phosphine oxide was used, 38 was scarcely observed under the optimized conditions. 2-Chloroquinoxaline and 2-chloropyrimidine reacted readily with (R)-cyclohexyl(phenyl)phosphine oxide gave products 39 and 40 in 60% and 50% yields with ee values of 98% and 97%, respectively. The reaction of 2,9-dichloro-1,10-phenanthroline with 2 equivalents of diphenylphosphine oxide 3 gave the chiral diphosphinylated product 41 selectively in 82% yield with 99% ee, and no meso product was formed. Among the various products, the absolute configuration of compound 23 was determined by X-ray crystallographic analysis.
Table 4 Visible-light-induced phosphinylation of heteroaryl chlorides with chiral secondary phosphine oxidesa,b

|
Reaction conditions: heteroaryl chlorides (0.6 mmol), chiral secondary phosphine oxides (0.50 mmol) and NaOH (0.75 mmol) in DMSO (2 mL) under blue LED irradiation (7 W) for 6 h at room temperature.
Isolated yield.
The substrate is 2-bromothiophene.
The substrate is 2-bromofuran.
2,9-dichloro-1,10-phenanthroline (0.5 mmol), 3 (1.0 mmol) and NaOH (1.5 mmol) in DMSO (2 mL) under blue LED irradiation (7 W) for 6 h at room temperature.
|
|
For the photo-induced phosphinylation of aryl/heteroaryl halides, general mechanistic studies have suggested the generation of a reactive phosphorus-centered monoradical and its subsequent coupling with a carbon-centered radical species to construct the C–P bond.6 The ee value of 3 is maintained in the presence of NaOH at room temperature or 80 °C, but the ee value is decreased to 62% in the presence of DBU at 80 °C for 4 h (Scheme 3).9 By DFT calculations, Minnaard and co-workers suggested that phosphorus-centered monoradical species is easily racemized.10 In order to probe the mechanism, the reaction of 3 with 2-chloropyridine in the presence of 2,2,6,6-tetramethylpiperdine-1-oxyl (TEMPO) was examined. Even in the presence of 4 equivalents of TEMPO, approximately 58% of the desired product 4 with 98% ee was obtained (Scheme 2). On the other hand, following the addition of NaOH (1 equiv.) to a 0.05 M solution of 2-chloropyridine in DMSO, we observed an absorption band with a peak at 480 nm and a relatively weak tail spanning the range from 500 to 650 nm (Fig. 1). It is possible that a complex was formed between the pyridine substrate and NaOH in DMSO.8b
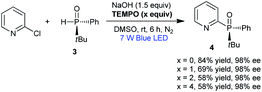 |
| Scheme 2 Phosphinylation of 2-chloropyridine in the presence of TEMPO. | |
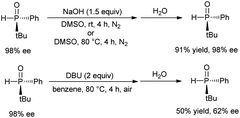 |
| Scheme 3 Control experiments. | |
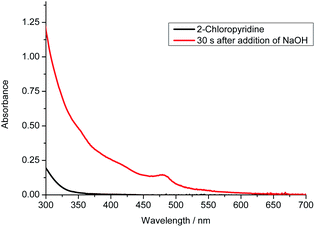 |
| Fig. 1 UV-vis absorption of the addition of NaOH (1 equiv.) into a DMSO solution of 2-chloropyridine (0.05 M). | |
Based on these results, we further performed density functional theory calculations (for computational details, see the ESI†) on the reaction of 3 with 2-chloropyridine under the standard conditions. The most favorable pathway for generating the product while maintaining the phosphine center is shown in Fig. 2. Initially, binding interaction between 2-chloropyridine, 3 and NaOH in DMSO would afford intermediate INT-A, which easily undergoes a deprotonation reaction to give intermediate INT-C. Subsequently, INT-C can be converted to 3[INT-C] (ΔG≠ = 50.5 kcal mol−1) via intersystem crossing (ISC) under blue light irradiation. 3[INT-C] undergoes a single electron transfer to give a diradical intermediate 3[INT-D]. The energy barrier for the generation of 3[TS3] from 3[INT-D] is 17.6 kcal mol−1. Then, product 4 is formed via an intramolecular radical–radical coupling reaction, which is compatible with the experimental conditions described in this work.11
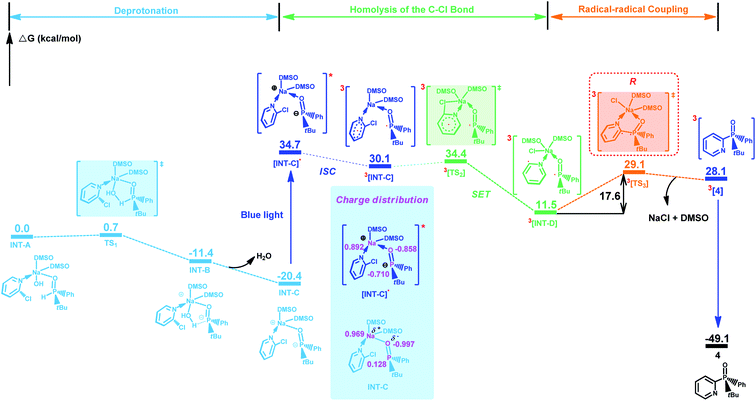 |
| Fig. 2 Gibbs free energy profiles for the reaction of 2-chloropyridine, 3 and NaOH in DMSO. The energies are obtained by SMD (solvent = dimethylsulfoxide) solvent model corrected free energy based on optimized geometry from ωB97XD/6-31+G(d) calculations. | |
Conclusions
In summary, we have developed a highly enantioretentive visible-light-induced C–P coupling of (R)-tert-butyl(phenyl)phosphine oxide with heteroaryl chlorides, which represents a powerful strategy for the synthesis of P-stereogenic heteroaryl phosphine oxides under air conditions without the use of an external photosensitizer. Various heteroaryl chlorides, including challenging polypyridyl and 1,10-phenanthrolinyl chlorides, were also successfully phosphinylated to give the chiral products in moderate to high yields with excellent ee values. Mechanistic studies and DFT calculations have revealed that the key intermediate 3[INT-C] was generated under blue LED irradiation, and subsequently undergoes single electron transfer and intramolecular radical–radical coupling to afford product with retention of the chiral phosphine center. These findings will probably lead to expedient and green approaches for the synthesis of valuable chiral molecules.
Data availability
All experimental, computational and crystallographic data associated with this study can be found in the article or in the ESI.†
Author contributions
C.-M. Che, G.-A. Yu and J. Yuan conceived and designed the experiments. Y. Zhang and J. Liu performed the experiments. G. Huang, J.-J. Zhong and L. Dang performed the DFT calculations. H. Yu, J. Chen and S. Meng analyzed the experiments. C.-M. Che, G.-A. Yu, and J. Yuan revised writing of the manuscript. All authors have given approval to the final version of the manuscript.
Conflicts of interest
There are no conflicts to declare.
Acknowledgements
The authors are grateful to the National Natural Science Foundation of China (No. 21472060, 21772058 and 21072071), the 111 Project B17019, self-determined research funds of CCNU from the colleges' basic research and operation of MOE (No. CCNU17CG0011, CCNU17KYZHSY07, and CCNU18KYZHSY06), the Chemistry and Chemical Engineering Guangdong Laboratory (1922003) and Guangdong Major Project of Basic and Applied Basic Research (2019B030302009) for financial support.
Notes and references
- Selected reviews on P-stereogenic phosphorus compounds:
(a)
M. Sasaki, in Chirality in Agrochemicals, ed. N. Kurihara and J. Miyamoto, Wiley & Sons, Chichester, 1998, p. 85 Search PubMed;
(b)
L. D. Quin, A Guide to Organophosphorus Chemistry, Wiley-Interscience, New York, 2000 Search PubMed;
(c) W. Tang and X. Zhang, Chem. Rev., 2003, 103, 3029 CrossRef CAS PubMed;
(d)
C. Darcel, J. Uziel and S. Jugé, in Phosphorous Ligands in Asymmetric Catalysis, ed. A. Börner, Wiley-VCH, Weinheim, 2008, vol. 3, p. 1211 Search PubMed;
(e) W. Fu and W. Tang, ACS Catal., 2016, 6, 4814 CrossRef CAS;
(f) G. Xu, C. H. Senanayake and W. Tang, Acc. Chem. Res., 2019, 52, 1101 CrossRef CAS PubMed;
(g) X. Ye, L. Peng, X. Bao, C.-H. Tan and H. Wang, Green Synth. Catal., 2021, 2, 6 CrossRef;
(h) X. Kang, C. Qian, H. Yang, J. Shi, J. Claverie and W. Tang, Green Synth. Catal., 2022 DOI:10.1016/j.gresc.2022.01.002.
- Selected works on synthesis of P-stereogenic phosphorus compounds:
(a) F. A. Kortmann, M.-C. Chang, E. Otten, E. P. A. Couzijn, M. Lutz and A. J. Minnaard, Chem. Sci., 2014, 5, 1322 RSC;
(b) O. I. Kolodiazhnyi, Top. Curr. Chem., 2015, 360, 161 CrossRef CAS PubMed;
(c) Z. S. Han, L. Zhang, Y. Xu, J. D. Sieber, M. A. Marsini, Z. Li, J. T. Reeves, K. R. Fandrick, N. D. Patel, J.-N. Desrosiers, B. Qu, A. Chen, D. M. Rudzinski, L. P. Samankumara, S. Ma, N. Grinberg, F. Roschangar, N. K. Yee, G. Wang, J. J. Song and C. H. Senanayake, Angew. Chem., Int. Ed., 2015, 54, 5474 CrossRef CAS PubMed;
(d) B. J. Anderson, S. C. Reynolds, M. A. Guino-o, Z. Xu and D. S. Glueck, ACS Catal., 2016, 6, 8106 CrossRef CAS;
(e) Z. S. Han, H. Wu, Y. Xu, Y. Zhang, B. Qu, Z. Li, D. R. Caldwell, K. R. Fandrick, L. Zhang, F. Roschangar, J. J. Song and C. H. Senanayake, Org. Lett., 2017, 19, 1796 CrossRef CAS PubMed;
(f) J. D. Sieber, S. Rodriguez, R. Frutos, F. Buono, Y. Zhang, N. Li, B. Qu, A. Premasiri, Z. Li, Z. S. Han, Y. Xu, D. Byrne, N. Haddad, J. Lorenz, N. Grinberg, D. Kurouski, H. Lee, B. Narayanan, L. Nummy, J. Mulder, J. D. Brown, A. Granger, J. Gao, M. Krawiec, Z. Williams, S. Pennino, J. J. Song, A. Hossain, N. K. Yee, C. Busacca, F. Roschangar, Y. Xin, Z. Mao, X. Zhang, Y. Hong and C. H. Senanayake, J. Org. Chem., 2018, 83, 1448 CrossRef CAS PubMed;
(g) R.-Y. Zhu, L. Chen, X.-S. Hu, F. Zhou and J. Zhou, Chem. Sci., 2020, 11, 97 RSC.
- Selected works on synthesis of P-stereogenic phosphines via transition-metal-catalyzed alkylation:
(a) V. S. Chan, I. C. Stewart, R. G. Bergman and F. D. Toste, J. Am. Chem. Soc., 2006, 128, 2786 CrossRef CAS PubMed;
(b) C. Scriban and D. S. Glueck, J. Am. Chem. Soc., 2006, 128, 2788 CrossRef CAS PubMed;
(c) B. J. Anderson, D. S. Glueck, A. G. DiPasquale and A. L. Rheingold, Organometallics, 2008, 27, 4992 CrossRef CAS;
(d) B. J. Anderson, M. A. Guino-o, D. S. Glueck, J. A. Golen, A. G. DiPasquale, L. M. Liable-Sands and A. L. Rheingold, Org. Lett., 2008, 10, 4425 CrossRef CAS PubMed;
(e) V. S. Chan, M. Chiu, R. G. Bergman and F. D. Toste, J. Am. Chem. Soc., 2009, 131, 6021 CrossRef CAS PubMed;
(f) B. J. Anderson, S. C. Reynolds, M. A. Guino-o, Z. Xu and D. S. Glueck, ACS Catal., 2016, 6, 8106 CrossRef CAS;
(g) X.-T. Liu, Y.-Q. Zhang, X.-Y. Han, S.-P. Sun and Q.-W. Zhang, J. Am. Chem. Soc., 2019, 141, 16584 CrossRef CAS PubMed;
(h) Q. Dai, L. Liu and J. Zhang, Angew. Chem., Int. Ed., 2021, 60, 27247 CrossRef CAS PubMed.
- Selected works on synthesis of P-stereogenic phosphines via transition-metal-catalyzed arylation:
(a) J. R. Moncarz, N. F. Laritcheva and D. S. Glueck, J. Am. Chem. Soc., 2002, 124, 13356 CrossRef CAS PubMed;
(b) T. J. Brunker, B. J. Anderson, N. F. Blank, D. S. Glueck and A. L. Rheingold, Org. Lett., 2007, 9, 1109 CrossRef CAS PubMed;
(c) V. S. Chan, R. G. Bergman and F. D. Toste, J. Am. Chem. Soc., 2007, 129, 15122 CrossRef CAS PubMed;
(d) N. F. Blank, J. R. Moncarz, T. J. Brunker, C. Scriban, B. J. Anderson, O. Amir, D. S. Glueck, L. N. Zakharov, J. A. Golen, C. D. Incarvito and A. L. Rheingold, J. Am. Chem. Soc., 2007, 129, 6847 CrossRef CAS PubMed;
(e) Z.-Q. Lin, W.-Z. Wang, S.-B. Yan and W.-L. Duan, Angew. Chem., Int. Ed., 2015, 54, 6265 CrossRef CAS PubMed;
(f) R. Beaud, R. J. Phipps and M. J. Gaunt, J. Am. Chem. Soc., 2016, 138, 13183 CrossRef CAS PubMed;
(g) Q. Dai, W. Li, Z. Li and J. Zhang, J. Am. Chem. Soc., 2019, 141, 20556 CrossRef CAS PubMed;
(h) C. Wang, C.-D. Yue, J. Yuan, J.-L. Zheng, Y. Zhang, H. Yu, J. Chen, S. Meng, Y. Yu, G.-A. Yu and C.-M. Che, Chem. Commun., 2020, 56, 11775 RSC;
(i) S. Zhang, J.-Z. Xiao, Y.-B. Li, C.-Y. Shi and L. Yin, J. Am. Chem. Soc., 2021, 143, 9912 CrossRef CAS PubMed.
- Selected works on synthesis of P-stereogenic phosphines via transition-metal-catalyzed hydrophosphination reactions of unsaturated bonds:
(a) A. D. Sadow, I. Haller, L. Fadini and A. Togni, J. Am. Chem. Soc., 2004, 126, 14704 CrossRef CAS PubMed;
(b) A. D. Sadow and A. Togni, J. Am. Chem. Soc., 2005, 127, 17012 CrossRef CAS PubMed;
(c) J.-J. Feng, X.-F. Chen, M. Shi and W.-L. Duan, J. Am. Chem. Soc., 2010, 132, 5562 CrossRef CAS PubMed;
(d) V. Koshti, S. Gaikwad and S. H. Chikkali, Coord. Chem. Rev., 2014, 265, 52 CrossRef CAS;
(e) S. A. Pullarkat, Synthesis, 2016, 48, 493 CrossRef CAS;
(f) S.-Z. Nie, R. T. Davison and V. M. Dong, J. Am. Chem. Soc., 2018, 140, 16450 CrossRef CAS PubMed;
(g) Z. Lu, H. Zhang, Z. Yang, N. Ding, L. Meng and J. Wang, ACS Catal., 2019, 9, 1457 CrossRef CAS;
(h) Q. Dai, L. Liu, Y. Qian, W. Li and J. Zhang, Angew. Chem., Int. Ed., 2020, 59, 20645 CrossRef CAS PubMed;
(i) X.-T. Liu, X.-Y. Han, Y. Wu, Y.-Y. Sun, L. Gao, Z. Huang and Q.-W. Zhang, J. Am. Chem. Soc., 2021, 143, 11309 CrossRef CAS PubMed.
- Selected reviews on visible-light-driven organic reactions:
(a) C. K. Prier, D. A. Rankic and D. W. C. MacMillan, Chem. Rev., 2013, 113, 5322 CrossRef CAS PubMed;
(b) J.-R. Chen, X.-Q. Hu, L.-Q. Lu and W.-J. Xiao, Acc. Chem. Res., 2016, 49, 1911 CrossRef CAS PubMed;
(c) S. A. Morris, J. Wang and N. Zheng, Acc. Chem. Res., 2016, 49, 1957 CrossRef CAS PubMed;
(d) T. Chatterjee, N. Iqbal, Y. You and E. J. Cho, Acc. Chem. Res., 2016, 49, 2284 CrossRef CAS PubMed;
(e) Y.-Y. Gui, L. Sun, Z.-P. Lu and D.-G. Yu, Org. Chem. Front., 2016, 3, 522 RSC;
(f) K. Luo, W.-C. Yang and L. Wu, Asian J. Org. Chem., 2017, 6, 350 CrossRef CAS;
(g) B.-G. Cai, J. Xuan and W.-J. Xiao, Sci. Bull., 2019, 64, 337 CrossRef CAS;
(h) S. P.-M. Ung, V. A. Mechrouk and C.-J. Li, Synthesis, 2021, 53, 1003 CrossRef CAS.
- Selected works on asymmetric photoredox reactions:
(a) A. Bauer, F. Westkämper, S. Grimme and T. Bach, Nature, 2005, 436, 1139 CrossRef CAS PubMed;
(b) Y. Inoue, Nature, 2005, 436, 1099 CrossRef CAS PubMed;
(c) H. Huo, X. Shen, C. Wang, L. Zhang, P. Röse, L.-A. Chen, K. Harms, M. Marsch, G. Hilt and E. Meggers, Nature, 2014, 515, 100 CrossRef CAS PubMed;
(d) E. Kumarasamy, R. Raghunathan, S. Jockusch, A. Ugrinov and J. Sivaguru, J. Am. Chem. Soc., 2014, 136, 8729 CrossRef CAS PubMed;
(e) A. G. Amador and T. P. Yoon, Angew. Chem., Int. Ed., 2016, 55, 2304 CrossRef CAS PubMed;
(f) T. P. Yoon, Acc. Chem. Res., 2016, 49, 2307 CrossRef CAS PubMed;
(g) E. Kumarasamy, A. J.-L. Ayitou, N. Vallavoju, R. Raghunathan, A. Iyer, A. Clay, S. K. Kandappa and J. Sivaguru, Acc. Chem. Res., 2016, 49, 2713 CrossRef CAS PubMed;
(h) M. Silvi, C. Verrier, Y. P. Rey, L. Buzzetti and P. Melchiorre, Nat. Chem., 2017, 9, 868 CrossRef CAS PubMed;
(i) F. Li, D. Tian, Y. Fan, R. Lee, G. Lu, Y. Yin, B. Qiao, X. Zhao, Z. Xiao and Z. Jiang, Nat. Commun., 2019, 10, 1774 CrossRef PubMed;
(j) C. Jiang, W. Chen, W.-H. Zheng and H. Lu, Org. Biomol. Chem., 2019, 17, 8673 RSC;
(k) G. Song, L. Yang, J.-S. Li, W.-J. Tang, W. Zhang, R. Cao, C. Wang, J. Xiao and D. Xue, Angew. Chem., Int. Ed., 2021, 60, 21536 CrossRef CAS PubMed.
-
(a) X. Luo, J. Yuan, C.-D. Yue, Z.-Y. Zhang, J. Chen, G.-A. Yu and C.-M. Che, Org. Lett., 2018, 20, 1810 CrossRef CAS PubMed;
(b) J. Yuan, W.-P. To, Z.-Y. Zhang, C.-D. Yue, S. Meng, J. Chen, Y. Liu, G.-A. Yu and C.-M. Che, Org. Lett., 2018, 20, 7816 CrossRef CAS PubMed;
(c) Z.-Y. Zhang, X. Zhang, J. Yuan, C.-D. Yue, S. Meng, J. Chen, G.-A. Yu and C.-M. Che, Chem.–Eur. J., 2020, 26, 5037 CrossRef CAS PubMed.
- Y. Ou, Y. Huang, Z. He, G. Yu, Y. Huo, X. Li, Y. Gao and Q. Chen, Chem. Commun., 2020, 56, 1357 RSC.
- F. A. Kortmann, M.-C. Chang, E. Otten, E. P. A. Couzijn, M. Lutz and A. J. Minnaard, Chem. Sci., 2014, 5, 1322 RSC.
-
(a) R. K. Mohamed, P. W. Peterson and I. V. Alabugin, Chem. Rev., 2013, 113, 7089 CrossRef CAS PubMed;
(b) G. Nocera, A. Young, F. Palumbo, K. J. Emery, G. Coulthard, T. McGuire, T. Tuttle and J. A. Murphy, J. Am. Chem. Soc., 2018, 140, 9751 CrossRef CAS PubMed;
(c) T. Stuyver, B. Chen, T. Zeng, P. Geerlings, F. D. Proft and R. Hoffmann, Chem. Rev., 2019, 119, 11291 CrossRef CAS PubMed;
(d) Y. Yin, X. Zhao, B. Qiao and Z. Jiang, Org. Chem. Front., 2020, 7, 1283 RSC;
(e) S. Mondal, F. Dumur, D. Gigmes, M. P. Sibi, M. P. Bertrand and M. Nechab, Chem. Rev., 2022, 122, 5842 CrossRef CAS PubMed.
Footnotes |
† Electronic supplementary information (ESI) available. CCDC 2121510. For ESI and crystallographic data in CIF or other electronic format see https://doi.org/10.1039/d2sc00036a |
‡ These authors contributed equally. |
|
This journal is © The Royal Society of Chemistry 2022 |