DOI:
10.1039/D1RE00471A
(Communication)
React. Chem. Eng., 2022,
7, 269-274
NMR relaxation time measurements of solvent effects in an organocatalysed asymmetric aldol reaction over silica SBA-15 supported proline†
Received
23rd October 2021
, Accepted 6th January 2022
First published on 6th January 2022
Abstract
Immobilisation of organocatalysts onto solid supports represents a very promising solution to tackle their low productivity by enabling their reuse. Herein, the use of NMR relaxation measurements, coupled with reaction screening, was used to investigate the effect of solvent interactions with the immobilised catalyst matrix on reactivity in an asymmetric organocatalysed aldol reaction. Important insights for the further development of such complex, yet promising catalytic systems are provided.
Introduction
Organocatalysis has nowadays consolidated its role as an advanced approach to access complex molecules, in a sustainable manner.1 The field grew at a dramatic pace with a plethora of new reactions and activation modes being developed.2 Unfortunately, albeit sometimes counterbalanced by economic and environmental factors, the low turnover number (TON) is often a drawback for industrial applications. Researchers have tried to overcome this setback by immobilising catalysts,3 or recycling them, by engineering highly active catalysts, and combining different types of catalysts synergistically.4 Among the different approaches, the most promising way seems to be the heterogenisation of the organocatalyst itself. By anchoring the organocatalyst onto an inert, mechanically-stable, insoluble support,5 it can be reused and implemented in a flow reactor.6 In the last two decades a large number of organocatalysts have been heterogenised on both organic and inorganic supports.7–17 Reactions and conditions cannot be simply translated from homogeneous to heterogeneous catalysis; adsorption effects due to the presence of a solid surface have to be taken into account.18 In fact, in the transition state (TS), the environment around the reacting partners experiences a drastic change with new interactions taking place.19 For example, SBA-15 and silica-based materials that exhibit a strong external acidity can be involved in the mechanism of enamine catalysis; Katz et al. reported that the silanol groups on the silica surface act as hydrogen bond donor towards some substrates.20 This dual activation mechanism was studied also relatively to silica functionalised with stronger acidic groups to investigate how variation in pKa affects the kinetics of aldol reactions.21 This suggests that a deeper understanding of the factors involved would aid the design of catalysts and supports. Characterising interactions within the pore space of such catalysts is challenging. In this context, NMR relaxation has recently emerged as a rapid and non-invasive tool to explore the chemical and morphological effects of solid surfaces in catalysis22–25 and to investigate the solvent effect in metal-catalysed homogeneous reactions.26 In particular, the ratio of the NMR relaxation times, T1/T2, has been proven to be a robust parameter to characterize surface interactions; strong molecule-surface interactions are characterised by a high T1/T2 ratio. In bulk liquids, spin–lattice T1 and spin–spin T2 relaxation times are similar and the T1/T2 ∼ 1. Within porous materials, they both decrease due to a more limited mobility of the molecules confined in the pore space. However, T2 experiences translational and rotational limitations associated with the surface diffusion to a higher degree than T1 and T1/T2 becomes greater than 1; thus, the T1/T2 ratio can be correlated with the molecule-surface affinity.27 For example, T1/T2 measurements have been successfully used to rationalize solvent effects in Au/TiO2 catalysts.28,29 It was shown that surface morphology may affect affinity of reaction species with the catalyst and influence catalytic performances. Recently, we used this approach to investigate the solvent effect in oxidative coupling of aldehydes mediated by polystyrene-supported NHCs.30
There is a number of reports where organocatalysts are supported on silica; however, the effect of the support on the reactivity remains largely unexplored.31 Herein, we combine reaction studies with NMR relaxation measurements to investigate the behaviour of a heterogenised proline organocatalyst over a structured silica support, SBA-15, widely used for its tuneable properties and high surface area, in a selection of the solvents, catalysing an aldol reaction between benzaldehyde and hydroxyacetone.
Results and discussion
The aldol reaction between benzaldehyde 1 and hydroxyacetone 2 was chosen as a benchmark reaction. Hydroxyacetone is an α-ketoalcohol, available in large quantities from the biofuel industry, produced by partial hydrogenolysis of glycerol.32 The aldol product 3 is a key diol intermediate for the synthesis of polyol species.33 A proline supported organocatalyst on SBA-15 mesoporous silica was selected to investigate the solvent effect in the proline-organocatalysed aldol reaction. Catalyst 4 was prepared according to previously reported procedures34 (Scheme 1, see ESI† for further information).
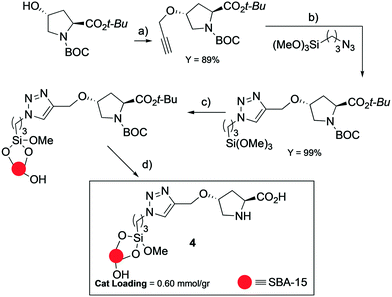 |
| Scheme 1 Synthesis of the immobilised proline catalyst 4. Reagents and conditions: a) NaH, propargyl bromide, −25 °C then RT, DMF, 16 h; b) (3-azidopropyl)trimethoxysilane, triethyl amine, CuI, RT, THF, 21 h; c) SBA-15, reflux, toluene, 48 h; d) TFA, 0 °C to RT, DCM, 32 h. | |
The benchmark reaction, taking the conditions from the literature report,1e was then run in different solvents comparing the behaviour of catalyst 4 and L-proline (Table 1).
Table 1 Aldol reaction between benzaldehyde 1 and hydroxyacetone 2 promoted by either L-proline or 4 in different solventsa
In agreement with previous reports, the reaction was slower over the heterogeneous catalyst with respect to the homogeneous counterpart.7a The heterogenised proline catalyst 4 performed best in the absence of solvent (neat, TOF = 0.51 × 10−5 s−1, entry 2), whereas L-proline worked better in solution (DMSO, TOF = 2.91 × 10−5 s−1vs. neat, TOF = 2.0 × 10−5 s−1, entries 3 and 1). In the accepted mechanism of proline-catalysed aldol reaction, a key step is the addition of the catalyst-substrate enamine adduct to the electrophilic aldehyde.35 The solvent may play an important role in this transition state through weak non-covalent interactions.19–21 Reactions performed in water and ethanol (entries 5–8) show that the solvent effect is dramatically different in homogeneous and heterogeneous catalysis. In fact, homogeneous L-proline is not able to catalyze the reaction in these two solvents, whereas it works when supported (H2O, TOF = 0.37 × 10−5 s−1; EtOH, TOF = 0.45 × 10−5 s−1).36 Thus the local combined environment of the organocatalysts and support imparts a great influence, potentially through control over the arrangement of the solvent molecules in the active site. The transition state of the enamine-aldehyde pair may therefore be different and affected by a distinct network established between the solvent and the silanol groups of the silica.20
Although an effect of water in the aldol reaction promoted by proline has been previously reported, this effect has not yet been studied when hydroxyacetone is used as a pro-nucleophile. Previous works suggest that traces of water in organic solvents may have a beneficial effect by suppressing substrate inhibition.20c,37 However, this aspect has not yet been elucidated when water is the main solvent and our results here suggest that the use of water as a solvent for such reactions may also be beneficial. The solvent significantly affects the enantioselectivity, not only using proline, but also using the supported catalyst 4. The best results are obtained with polar aprotic solvents (DMSO and DMF); these findings may suggest that more polar aprotic solvents are able to establish similar interactions regardless of the presence of the silica-based support. Leaching of the organocatalyst into solution was investigated by running the reaction in deuterated solvents and looking for leached moieties, and ruled out.
NMR relaxation measurements were used to investigate the solvent-matrix surface interactions. A high T1/T2 ratio represents high affinity and strong interactions between the solvent and the solid support. Relaxation measurements of the solvents and reactants, over 4 and the unfunctionalised matrix SBA-15 were performed (Fig. 1 and Table 2, see ESI† for further details). It can be clearly seen that functionalisation of silica with proline may have a marked effect on surface interactions with different solvents. Affinity of polar solvents decreases when silica is functionalised with the catalyst (Table 2, T1/T2 ratio for SBA-15 vs.4, entries 3–8), which can be rationalised by considering the replacement of silanol groups by the proline group. The loss of silanol groups decreases the interaction of the surface of silica and the H-bond donors and acceptors of the solvent. Indeed, a minimal change in T1/T2 was observed in the case of cyclohexane, which is incapable of forming H-bond interactions (entry 9). Conversely, water exhibits the most prominent decrease in T1/T2, as it is known to form strong and extensive hydrogen bonding (entry 4). Ethanol, cyclohexane and hydroxyacetone 2, showed the lowest values of T1/T2 ratios in functionalised silica 4 (entries 5, 9, and 1).
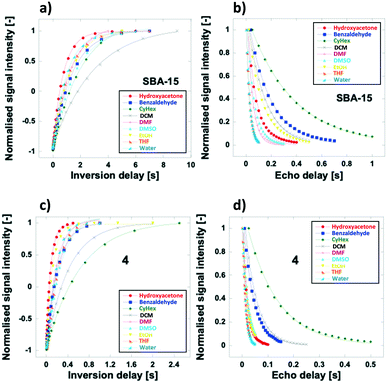 |
| Fig. 1 a) T1 inversion recovery plots for solvents adsorbed within SBA-15; b) T2 CPMG decay plots for solvents adsorbed within SBA-15; c) T1 inversion recovery plots for solvents adsorbed within 4; d) T2 CPMG decay plots for solvents adsorbed within 4. | |
Table 2
T
1/T2 relaxation measurements for different solvents in 4 and SBA-15
Entry |
Solvent |
4
|
SBA-15 |
T
1/T2 |
T
1/T2 |
1 |
Hydroxyacetone |
5.6 ± 0.2 |
10.2 ± 0.3 |
2 |
Benzaldehyde |
5.3 ± 0.2 |
7.8 ± 0.2 |
3 |
DMSO |
7.5 ± 0.2 |
24.4 ± 0.7 |
4 |
H2O |
10.0 ± 0.3 |
38.4 ± 1.2 |
5 |
EtOH |
6.5 ± 0.2 |
11.0 ± 0.3 |
6 |
DMF |
9.4 ± 0.3 |
30.3 ± 0.9 |
7 |
DCM |
9.1 ± 0.3 |
20.8 ± 0.6 |
8 |
THF |
12.6 ± 0.4 |
44.6 ± 1.4 |
9 |
CyHex |
5.2 ± 0.2 |
5.7 ± 0.2 |
It is intriguing to note the lower interaction with 4 showed by ethanol compared to DCM or THF. Whilst a more detailed study on the surface science, possibly with the aid of DFT calculations, is out of the scope of the current work, we may suggest some insights. Molecules such as THF are known to often act as catalyst poisons and this is attributed to their ability to adsorb strongly onto the surface;38 we believe this might be associated to the ability of the unpaired electrons on the oxygen atom of THF to act as a Lewis base and bind to positive charges on the catalyst surface. Kamlet–Taft solvatochromic parameters may also play a role in determining the T1/T2 values; for example, the solvent polarizability of DCM and THF is greater than that of ethanol and cyclohexane. Indeed, the highest reactivity occurs in ethanol and cyclohexane, which are also the solvents with the lowest polarizability values among those studied. This is in agreement with the low T1/T2 values reported for such solvents; hence, this parameter, whilst not being the only one responsible for determining the T1/T2 values, certainly plays a significant role in determining this trend.39
By combining the observations from Tables 1 and 2, the highest reactivity is measured in solvents with low affinity with the solid catalyst (cyclohexane, ethanol and hydroxyacetone 2, the latter roughly comparable to a solvent when used in large excess in neat conditions). Relaxation measurements show that such solvents interact less strongly to the surface and make the catalytic site more accessible to substrates. Surprisingly, no stereoselectivity is observed in these solvents. As mentioned previously, the reaction proceeds only in heterogeneous conditions when water and ethanol were used as the solvent (Table 1, entries 5–8); this corroborates the assumption that the presence of solid surface changes the environment around catalytic sites, as evidenced by the change in T1/T2.
A plot of the T1/T2 ratio against the TOF of the examined reaction is reported in Fig. 2. The results in Fig. 2 show that in general solvents with a higher T1/T2, hence a higher surface affinity, lead to a lower reactivity, as indicated by the lower TOF. This trend is in agreement with previous findings reported for more traditional metal catalysts.40 The results in Fig. 2 suggest that the T1/T2 ratio is a useful indicator to differentiate solvents based on strength of surface interactions and that solvents with a preferential interaction with the solid matrix inhibit access of reactants to the active sites. The solvent affinity for the surface of the solid matrix, therefore, is a key parameter to consider when designing catalytic materials, while the T1/T2 ratio is a useful and easily accessible indicator for the affinity.
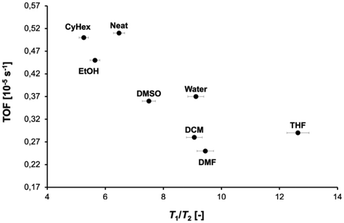 |
| Fig. 2
T
1/T2 of solvents (adsorbed within catalyst 4) used in the aldol reaction between benzaldehyde 1 and hydroxyacetone 2versus TOF. | |
We note that the solvent is likely to impact not only the TOF but also the enantioselectivity. However, such effects would be much more complex to unravel purely based on competitive adsorption effects as they would involve other aspects, such as adsorption geometry and changes in transition states. Other approaches, such as computational DFT, could be useful in this regard, which are however out of the scope of this work.
Conclusions
Silica-supported organocatalysts are a potential solution to increase productivity by enabling easy separation and reuse of such catalysts. However, the effect of the solid matrix on the reactivity has largely remained unexplored. We have assessed reactivity and adsorption phenomena, via NMR relaxation measurements, in an aldol reaction promoted by SBA-15 immobilised proline organocatalyst. The results show that solvents with a high surface affinity lead to a lower reactivity and this may be ascribed to the inability of reactant molecules to access active sites. This study suggests that the solvent interaction with the solid surface is an important parameter to consider when screening heterogenised organocatalysts and NMR relaxation can be used as a fast screening method for solvent evaluation (typical data acquisition time is of the order of tens of minutes). A further significant finding was that in water and ethanol the reaction is catalysed by the immobilised organocatalyst, in sharp contrast with the behaviour of the homogeneous organocatalyst. This observation opens up the potential for the use of more environmentally benign, less hazardous solvents for these transformations, while simultaneous employing an easy-to-separate immobilised catalyst, with critical implications for industrial applications. More work is underway to study this phenomenon and will be the subject of further studies.
Author contributions
Graziano Di Carmine: Conceptualisation, data curation, investigation, methodology, writing-original draft, writing-review & editing. Luke Forster: Data curation, formal analysis, writing-review & editing. Simeng Wang: Data curation, investigation, methodology. Christopher Parlett: Methodology, formal analysis, writing-review & editing. Armando Carlone: Conceptualisation, supervision, writing-review & editing. Carmine D'Agostino: Conceptualisation, funding acquisition, project administration, resources, supervision, writing-review & editing.
Conflicts of interest
There are no conflicts to declare.
Acknowledgements
Carmine D'Agostino, Simeng Wang and Graziano Di Carmine would like to acknowledge the EPSRC, grant no. EP/S019138/1, for funding the research activities carried out for this work. We also acknowledge Dr Andy York from Johnson Matthey for fruitful discussions. Christopher Parlett would like to thank the Research Complex and the UK Catalysis Hub for access and support to these facilities and equipment.
Notes and references
-
(a) B. List, R. A. Lerner and C. F. Barbas, J. Am. Chem. Soc., 2000, 122, 2395 CrossRef CAS;
(b) K. A. Ahrendt, C. J. Borths and D. W. C. MacMillan, J. Am. Chem. Soc., 2000, 122, 4243 CrossRef CAS;
(c) S. Mitsumori, H. Zhang, P. H.-Y. Cheong, K. N. Houk, F. Tanaka and C. F. Barbas, J. Am. Chem. Soc., 2006, 128, 1040 CrossRef CAS PubMed;
(d) A. Dondoni and A. Massi, Angew. Chem., Int. Ed., 2008, 47, 4638 CrossRef CAS PubMed;
(e) K. Sakthivel, W. Notz, T. Bui and C. F. Barbas, J. Am. Chem. Soc., 2001, 123, 5260 CrossRef CAS PubMed.
-
(a) P. Melchiorre, M. Marigo, A. Carlone and G. Bartoli, Angew. Chem., Int. Ed., 2008, 47, 6138 CrossRef CAS PubMed;
(b) A. Carlone and L. Bernardi, Phys. Sci. Rev., 2019, 4, 20180097 Search PubMed.
-
(a) T. Fulgheri, F. Della Penna, A. Baschieri and A. Carlone, Curr. Opin. Green Sustainable Chem., 2020, 25, 100387 CrossRef;
(b) L. Zhang, S. Luo and J.-P. Cheng, Catal. Sci. Technol., 2011, 1, 507 RSC;
(c) M. Gruttadauria, F. Giacalone and R. Noto, Chem. Soc. Rev., 2008, 37, 1666 RSC.
-
(a) R. A. Angnes, Z. Li, C. R. D. Correisa and G. B. Hammond, Org. Biomol. Chem., 2015, 13, 9152 RSC;
(b) A. Sinibaldi, V. Nori, A. Baschieri, F. Fini, A. Arcadi and A. Carlone, Catalysts, 2019, 9, 928 CrossRef CAS;
(c) H. Ohmiya, ACS Catal., 2020, 10, 6862 CrossRef CAS;
(d) P. G. Cozzi, A. Gualandi, S. Potenti, F. Calogero and G. Rodeghiero, Top. Curr. Chem., 2020, 378, 1 CrossRef CAS PubMed.
-
(a) M. Benaglia, A. Puglisi and F. Cozzi, Chem. Rev., 2003, 103, 3401 CrossRef CAS PubMed;
(b) L. Xu, J. Huang, M. Zhang, L. Yu and Y. Fan, ChemistrySelect, 2016, 1, 1933 CrossRef CAS;
(c) P. Kasaplar, C. Rodriguez-Escrich and M. A. Pericàs, Org. Lett., 2013, 15, 3498 CrossRef CAS PubMed;
(d) J. Lai, M. Fianchini and M. A. Pericàs, ACS Catal., 2020, 10, 14971 CrossRef CAS;
(e) D. Ragno, G. Di Carmine, A. Brandolese, O. Bortolini, P. P. Giovannini and A. Massi, ACS Catal., 2017, 7, 6365 CrossRef CAS;
(f) A. M. Goldys, M. G. Nunez and D. J. Dixon, Org. Lett., 2014, 16, 6294 CrossRef CAS PubMed;
(g) A. Corma and H. Garcia, Adv. Synth. Catal., 2006, 348, 1391 CrossRef CAS.
-
(a) M. B. Plutschack, B. Pieber, K. Gilmore and P. H. Seeberger, Chem. Rev., 2017, 117, 11796 CrossRef CAS PubMed;
(b) D. Cantillo, O. de Frutos, J. A. Rincoń, C. Mateos and C. O. Kappe, Org. Lett., 2014, 16, 896 CrossRef CAS PubMed;
(c) T. Tsubogo, T. Ishiwata and S. Kobayashi, Angew. Chem., Int. Ed., 2013, 52, 6590 CrossRef CAS PubMed;
(d) I. Atodiresei, C. Vila and M. Rueping, ACS Catal., 2015, 5, 1972 CrossRef CAS;
(e) C. De Risi, O. Bortolini, A. Brandolese, G. Di Carmine, D. Ragno and A. Massi, React. Chem. Eng., 2020, 5, 1017 RSC;
(f) D. Ragno, A. Brandolese, D. Urbani, G. Di Carmine, C. De Risi, O. Bortolini, P. P. Giovannini and A. Massi, React. Chem. Eng., 2018, 3, 816 RSC;
(g) A. Brandolese, M. D. Greenhalgh, T. Desrues, X. Liu, S. Qu, C. Bressy and A. D. Smith, Org. Biomol. Chem., 2021, 19, 3620 RSC;
(h) A. Brandolese, D. Ragno, G. Di Carmine, T. Bernardi, O. Bortolini, P. P. Giovannini, O. G. Pandoli, A. Altomare and A. Massi, Org. Biomol. Chem., 2018, 16, 8955 RSC;
(i) D. Ragno, C. Leonardi, G. Di Carmine, O. Bortolini, A. Brandolese, C. De Risi and A. Massi, ACS Sustainable Chem. Eng., 2021, 9, 8295 CrossRef CAS.
-
(a) F. Calderón, R. Fernández, F. Sánchez and A. Fernández-Mayoralas, Adv. Synth. Catal., 2005, 347, 1395 CrossRef;
(b) C. Zhi, J. Wang, B. Luo, X. Li, X. Cao, Y. Pana and H. Gu, RSC Adv., 2014, 4, 15036 RSC;
(c) A. Khalafi-Nezhad, E. S. Shahidzadeh, S. Sarikhani and F. Panahi, J. Mol. Catal. A: Chem., 2013, 379, 1 CrossRef CAS;
(d) Z. An, Y. Guo, L. Zhao, Z. Li and J. He, ACS Catal., 2014, 4, 2566 CrossRef CAS;
(e) E. Montroni, M. Lombardo, A. Quintavalla, C. Trombini, M. Gruttadauria and F. Giacalone, ChemCatChem, 2012, 4, 1000 CrossRef CAS.
-
(a) Y. Tang, Q. Wang, L. Wu, K. Liu, W. Wang, Y. Shen, Y. Xue and S. Dai, React. Funct. Polym., 2020, 150, 104544 CrossRef CAS;
(b) M. Benaglia, M. Cinquini, F. Cozzi, A. Puglisi and G. Celentano, Adv. Synth. Catal., 2002, 344, 533 CrossRef CAS;
(c) K. Ponnuru, J. C. Manayil, H. J. Cho, A. Osatiashtiani, W. Fan, K. Wilson and F. C. Jentoft, Mol. Catal., 2018, 458, 247 CrossRef CAS;
(d) C. Leonardi, A. Brandolese, L. Preti, O. Bortolini, E. Polo, P. Dambruoso, D. Ragno, G. Di Carmine and A. Massi, Adv. Synth. Catal., 2021, 363, 5473 CrossRef CAS.
- A. R. Hajipour and Z. Khorsandi, ChemistrySelect, 2017, 2, 8976 CrossRef CAS.
- M. Azlouka, M. Durmazb, E. Zorc and H. Bingol, Mater. Chem. Phys., 2020, 239, 122298 CrossRef.
-
(a) R. Firouzi-Haji and A. Maleki, ChemistrySelect, 2019, 4, 853 CrossRef CAS;
(b) F. Saberi, D. Rodriguez-Padrón, A. Garcia, H. R. Shaterian and R. Luque, Catalysts, 2018, 8, 167 CrossRef CAS;
(c) N. Esfandiary and A. Heydari, Appl. Organomet. Chem., 2020, 34, e5760 CAS.
-
(a) Z. Wang, J. Yan, X. Zhang and L. Wan, Synthesis, 2009, 22, 3744 Search PubMed;
(b) F. Giacalone, M. Gruttadauria, A. Mossuto Marculescu and R. Noto, Tetrahedron Lett., 2007, 48, 255 CrossRef CAS;
(c) Y.-X. Liu, Y.-N. Sun, H.-H. Tan, W. Liu and J.-C. Tao, Tetrahedron: Asymmetry, 2007, 18, 2649 CrossRef CAS.
- N. C. Ellebracht and C. W. Jones, Cellulose, 2018, 25, 6495 CrossRef CAS.
- N. C. Ellebracht and C. W. Jones, ACS Catal., 2019, 9, 3266 CrossRef CAS.
- N. C. Ellebracht and C. W. Jones, Carbohydr. Polym., 2020, 233, 115825 CrossRef CAS PubMed.
- A. De Vylder, J. Lauwaert, J. De Clercq, P. van der Voort, C. W. Jones and J. W. Thybaut, J. Catal., 2020, 381, 540 CrossRef CAS.
- A. Erigoni and U. Diaz, Catalysts, 2021, 11, 79 CrossRef CAS.
-
(a) P. de Anna, J. Jimenez-Martinez, H. Tabuteau, R. Turuban, T. Le Borgne, M. Derrien and Y. Méheust, Environ. Sci. Technol., 2014, 48, 508 CrossRef PubMed;
(b) J. Lauwaert, J. Ouwehand, J. De Clercq, P. Cool, P. van der Voort and J. W. Thybaut, Catal. Commun., 2017, 88, 85 CrossRef CAS.
- D. Gärtner, S. Sandl and A. J. von Wangelin, Catal. Sci. Technol., 2020, 10, 3502 RSC.
-
(a) J. D. Bass, S. L. Anderson and A. Katz, Angew. Chem., Int. Ed., 2003, 42, 5219 CrossRef CAS PubMed;
(b) J. D. Bass, A. Solovyov, A. J. Pascall and A. Katz, J. Am. Chem. Soc., 2006, 128, 3737 CrossRef CAS PubMed;
(c) K. Kandel, S. M. Althaus, C. Peeraphatdit, T. Kobayashi, B. G. Trewyn, M. Pruski and I. I. Slowing, ACS Catal., 2013, 3, 265 CrossRef CAS.
-
(a) R. K. Zeidan, S.-J. Hwang and M. E. Davis, Angew. Chem., Int. Ed., 2006, 45, 6332 CrossRef CAS PubMed;
(b) R. K. Zeidan and M. E. Davis, J. Catal., 2007, 247, 379 CrossRef CAS;
(c) N. A. Brunelli, K. Venkatasubbaiah and C. W. Jones, Chem. Mater., 2012, 24, 2433 CrossRef CAS;
(d) J. Lauwaert, E. G. Moschetta, P. van der Voort, J. W. Thybaut, C. W. Jones and G. B. Marin, J. Catal., 2015, 325, 19 CrossRef CAS;
(e) P. M. Heynderickx, Int. J. Mol. Sci., 2019, 20, 3819 CrossRef CAS PubMed.
-
(a) A. T. Krzyzak and I. Habina, Microporous Mesoporous Mater., 2016, 231, 230 CrossRef CAS;
(b) D. Weber, J. Mitchell, J. McGregor and L. F. Gladden, J. Phys. Chem. C, 2009, 113, 6610 CrossRef CAS;
(c) D. W. Aksnes, K. Førland and L. Kimtys, J. Mol. Struct., 2004, 708, 23 CrossRef CAS;
(d) I. Habina, N. Radzik, T. Topoŕ and A. T. Krzyzǎk, Microporous Mesoporous Mater., 2017, 252, 37 CrossRef CAS.
-
(a) C. D'Agostino, J. Mitchell, M. D. Mantle and L. F. Gladden, Chem. – Eur. J., 2014, 20, 13009 CrossRef PubMed;
(b) G. Filippini, F. Longobardo, L. Forster, A. Criado, G. Di Carmine, L. Nasi, C. D'Agostino, M. Melchionna, P. Fornasiero and M. Prato, Sci. Adv., 2020, 6, eabc9923 CrossRef CAS PubMed.
- M. H. Haider, C. D'Agostino, N. F. Dummer, M. D. Mantle, L. F. Gladden, D. W. Knight, D. J. Willock, D. J. Morgan, S. H. Taylor and G. J. Hutchings, Chem. – Eur. J., 2014, 20, 1743 CrossRef CAS PubMed.
- C. D'Agostino, Y. Ryabenkova, P. J. Miedziak, S. H. Taylor, G. J. Hutchings, L. F. Gladden and M. D. Mantle, Catal. Sci. Technol., 2014, 4, 1313 RSC.
- A. Muhammad, G. Di Carmine, L. Forster and C. D'Agostino, ChemPhysChem, 2020, 21, 1101 CrossRef CAS PubMed.
- S. Godefroy, J.-P. Korb, M. Fleury and R. G. Bryant, Phys. Rev. E: Stat., Nonlinear, Soft Matter Phys., 2001, 64, 021605 CrossRef CAS PubMed.
- C. D'Agostino, G. Brett, G. Divitini, C. Ducati, G. J. Hutchings, M. D. Mantle and L. F. Gladden, ACS Catal., 2017, 7, 4235 CrossRef.
- C. D'Agostino, R. D. Armstrong, G. J. Hutchings and L. F. Gladden, ACS Catal., 2018, 8, 7334 CrossRef.
- G. Di Carmine, D. Ragno, A. Massi and C. D'Agostino, Org. Lett., 2020, 22, 4927 CrossRef CAS PubMed.
-
(a) N. A. Brunelli and C. W. Jones, J. Catal., 2013, 308, 60 CrossRef CAS;
(b) A. Monge-Marcet, X. Cattoën, D. A. Alonso, C. Nájera, M. W. C. Man and R. Pleixats, Green Chem., 2012, 14, 1601 RSC;
(c) S. Rostamnia and E. Doustkhah, RSC Adv., 2014, 4, 28238 RSC;
(d) A. Erigoni, M. C. Hernández-Soto, F. Rey, C. Segarra and U. Díaz, Catal. Today, 2020, 345, 227 CrossRef CAS;
(e) O. Bortolini, L. Caciolli, A. Cavazzini, V. Costa, R. Greco, A. Massi and L. Pasti, Green Chem., 2012, 14, 992 RSC.
- J. González-Cobos, S. Baranton and C. Coutanceau, ChemElectroChem, 2016, 3, 1694 CrossRef.
- S. E. Bode, M. Wolberg and M. Müller, Synthesis, 2006, 4, 557 Search PubMed.
-
(a) C. Ayats, A. H. Henseler and M. A. Pericàs, ChemSusChem, 2012, 5, 320 CrossRef CAS PubMed;
(b) A. Massi, A. Cavazzini, L. Del Zoppo, O. Pandoli, V. Costa, L. Pasti and P. P. Giovannini, Tetrahedron Lett., 2011, 52, 619 CrossRef CAS;
(c) C.-J. Yoo, D. Rackl, W. Liu, C. B. Hoyt, B. Pimentel, R. P. Lively, H. M. L. Davies and C. W. Jones, Angew. Chem., Int. Ed., 2018, 57, 10923 CrossRef CAS PubMed.
- N. Zotova, A. Franzke, A. Armstrong and D. G. Blackmond, J. Am. Chem. Soc., 2007, 129, 15100 CrossRef CAS PubMed.
- Running the reaction with 1 and 2 in presence of SBA-15, either in water or EtOH, showed no formation of product.
-
(a) E. G. Doyagüez, F. Calderón, F. Sánchez and A. Fernández-Mayoralas, J. Org. Chem., 2007, 72, 9353 CrossRef PubMed;
(b) K. Kandel, S. M. Althaus, C. Peeraphatdit, T. Kobayashi, B. G. Trewyn, M. Pruski and I. I. Slowing, J. Catal., 2012, 291, 63 CrossRef CAS;
(c) A. De Vylder, J. Lauwaert, D. Esquivel, D. Poelman, J. De Clercq, P. van der Voort and J. W. Thybaut, J. Catal., 2018, 361, 51 CrossRef CAS;
(d) A. De Vylder, J. Lauwaert, M. K. Sabbe, M.-F. Reyniers, J. De Clercq, P. van der Voort and J. W. Thybaut, Chem. Eng. J., 2021, 404, 127070 CrossRef CAS;
(e) D. Singappuli-Arachchige, T. Kobayashi, Z. Wang, S. J. Burkhow, E. A. Smith, M. Pruski and I. I. Slowing, ACS Catal., 2019, 9, 5574 CrossRef CAS.
- See for example a very recent work, S. Zhao, J. Cai, H. Chen and J. Shen, Catal. Commun., 2021, 157, 106330 CrossRef CAS.
-
(a) L. Crowhurst, R. Falcone, N. L. Lancaster, V. Llopis-Mestre and T. Welton, J. Org. Chem., 2006, 71, 8847 CrossRef CAS PubMed;
(b) M. Roses, J. Ortega and E. Bosch, J. Solution Chem., 1995, 24, 51 CrossRef CAS;
(c) B. U. Emenike, S. N. Bey, B. C. Bigelow and S. V. S. Chakravartula, Chem. Sci., 2016, 7, 1401 RSC.
-
(a) C. D'Agostino, M. R. Feaviour, G. L. Brett, J. Mitchell, A. P. E. York, G. J. Hutchings, M. D. Mantle and L. F. Gladden, Catal. Sci. Technol., 2016, 6, 7896 RSC;
(b) C. D'Agostino, M. D. Mantle and L. F. Gladden, React. Chem. Eng., 2020, 5, 1053 RSC.
Footnote |
† Electronic supplementary information (ESI) available. See DOI: 10.1039/d1re00471a |
|
This journal is © The Royal Society of Chemistry 2022 |
Click here to see how this site uses Cookies. View our privacy policy here.