DOI:
10.1039/D2RA06357C
(Paper)
RSC Adv., 2022,
12, 34404-34413
Luminescence and energy transfer of single-phase white-emitting phosphor Ba2Mg(PO4)2:Ce3+, Eu2+ for white LEDs†
Received
9th October 2022
, Accepted 25th November 2022
First published on 30th November 2022
Abstract
A series of Ba2Mg(PO4)2:Ce3+, Eu2+ phosphors were synthesized by the traditional high temperature solid-state method, and the crystal structures and luminescence properties of the samples were discussed systematically. The energy transfer from Ce3+ to Eu2+ in Ba2Mg(PO4)2 was proved to be of resonant type via a dipole–dipole interaction mechanism. With a precisely controlled relative proportion of Ce3+/Eu2+, the emission color of the samples can vary from blue (0.157, 0.071) to white (0.352, 0.332) and ultimately to yellow (0.452, 0.466) under the 323 nm ultraviolet light radiation excitation. The result reveals that the Ba2Mg(PO4)2:Ce3+, Eu2+ phosphor may have potential application as a single-phased white-emitting phosphor for light emitting diodes.
1. Introduction
In recent years, white light emitting diodes (LEDs) have attracted the attention of domestic and foreign researchers due to their energy saving, high efficiency, long life, and other advantages.1 At present, white LEDs can be achieved by combining a blue InGaN LED chip with a yellow phosphor, YAG:Ce, which shows a poor color rendering index (Ra ≈ 70–80) and high CCT (CCT ≈ 7750 K) due to a lack of red component.1 To solve these problems, white LEDs can also be fabricated by near-ultraviolet (UV) LED or UV-LED pumping red/green/blue multi-phased phosphors or a single-phase white emitting phosphor.2–4 Generally, a white emitting phosphor can be obtained by controlling the concentration of several rare earths, however, due to the lack of certain light components, the obtained white phosphor shows a low color rendering index and cannot be applied in practice.6–11 Therefore, the discovery of new phosphors, the design of crystal structure and the optimization of luminescence performance are still the hot spots of current research. Actually, the method of obtaining white light phosphor by using two kinds of rare earth ions doped together to generate energy transfer is the most popular among researchers. The phosphor obtained by this method presents a wide spectrum coverage. In addition, since there are only two types of activated ions, there are fewer energy transfer processes and relatively less energy loss. Therefore, this is an ideal method at present. In the process of obtaining single-phase white emitting phosphor, the choice of two kinds of activated particles is also crucial. Generally, the selected activated ions are two rare earth ions or rare earth/transition metal ions. Under the condition of energy level matching, energy transfer can occur between the doped activation ions, such as Ce3+/Eu2+, Ce3+/Mn2+ and Eu2+/Mn2+.2–6 Considering that the luminescence properties of Ce3+ and Eu2+ ions are relatively good, and the spectral coverage is wide, especially for Eu2+ ions, their spectral coverage can be from ultraviolet to near infrared, hence the researchers are more inclined to use Ce3+/Eu2+ co-doping to obtain white emission, this work also uses this method to obtain white emitting phosphor.7–26
To the best our knowledge, in addition to the selection of activated ions, the matrix materials is also very important. For the host materials of phosphors, phosphate compounds are widely used currently owing to the facile synthesis, environmental-friendly characteristics and high stabilities, such as (Ca, Sr)9Sc(PO4)7:Eu2+, Mn2+,27 Ca9−x−yCe(PO4)7:xEu2+, yMn2+28 and Ca9La(PO4)7:Eu2+.29 BaMg2(PO4)2 is an alkaline earth phosphate compound with a rigid tetrahedral three-dimensional structure. Its physical and chemical properties are stable. Although the luminescence performance of Ba2Mg(PO4)2:Eu2+ has been widely investigated,30–36 however, a systematic study on the energy transfer between Eu2+ and Ce3+ in Ba2Mg(PO4)2 was not reported. In this work, the crystal structure of Ba2Mg(PO4)2 and preferred crystallographic sites for activators were reported. The structure of Ba2Mg(PO4)2 is monoclinic with space group P21/n (Z = 4). There are two Ba2+ sites in this crystal lattice, one is 7 coordination, the other is 8 coordination, which can be replaced by rare earth dopants. The luminescence properties of Eu2+ and Ce3+ in Ba2Mg(PO4)2 is demonstrated. After co-doping with Ce3+ ions, the emission intensity of Eu2+ ions was enhanced and a novel blue-yellow tunable phosphor Ba2Mg(PO4)2:Ce3+, Eu2+ can be obtained by varying the relative ratio of Ce3+/Eu2+ under the irradiation of 323 nm light. Moreover, the energy transfer process and mechanism between Ce3+ and Eu2+ has been discussed systematically.
2. Experimental section
2.1 Synthesis
A series of Ba2Mg(PO4)2:xCe3+, yEu2+ phosphors were prepared by a traditional high-temperature solid-state method. The raw materials are BaCO3 (99.99%), MgO (99.99%), NH4H2PO4 (99.99%), CeO2 (99.99%) and Eu2O3 (99.99%), which thoroughly mix and grind in an agate mortar for 20 min. Then powder mixtures were pre-heated in furnace at 500 °C for 2 h, and subsequently sintered at 1100 °C for 5 h in CO reducing atmosphere to get the final samples. Finally, the furnace temperature was lowered to room temperature, and the phosphor was ground before subsequent characterization.
2.2 Material characterization
The structure of samples is identified by powder X-ray diffraction (Bruker D8 X-ray diffractometer), with Ni-filtered Cu Kα radiation (λ = 0.15405 nm), operating at 40 mA and 40 kV and recorded the patterns in the range of 2θ from 10° to 80°. The structural refinements were analyzed by General Structural Analysis System (GSAS) program. The steady time photoluminescence spectra including excitation (PLE) and emission (PL) spectra are measured in the range of 240 nm to 800 nm with scanning speed 1200 nm min−1 by a HITACHI F-4600 fluorescence spectrometer. The temperature dependent luminescence properties were measured on the same spectrophotometer, which was assembled with a TAP-02 high temperature fluorescence controller. The decay curves of the photoluminescence are acquired by FLS920 fluorescence spectrometer.
3. Results and discussion
3.1 Phase formation and structure of Ba2Mg(PO4)2
It was reported that Ba2Mg(PO4)2 (BMPO) is member of apatite-type family, which has a monoclinic structure with space group P121/n1.37 The crystal structure of BMPO is shown in Fig. 1a. There are two types of Ba ions polyhedrons in this host, and Ba ions polyhedrons are accommodated in the tunnels that come out of three-dimensional framework of MgO6 and PO4 tetrahedrons. The Ba1 is surrounded by eight O atoms and Ba2 coordinate with seven O atoms. The Rietveld refinement with the results of BMPO presented in Fig. 2b, and the Rietveld refinement method has been conducted with GSAS. More XRD patterns and refined parameters are in Fig. S1 and Table S1,† and the diffraction peaks of the samples are well matched with the standard card BMPO (ICSD#59253), and no other impurity peaks are found, indicating the samples are all single crystal pure phase samples.
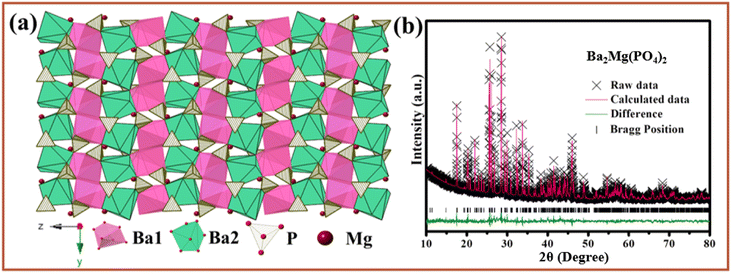 |
| Fig. 1 (a) Crystal structure of Ba2Mg(PO4)2. (b) Powder XRD patterns for Rietveld structure analysis of Ba2Mg(PO4)2 based on the Ba2Mg(PO4)2 ICSD#59253 phase model. | |
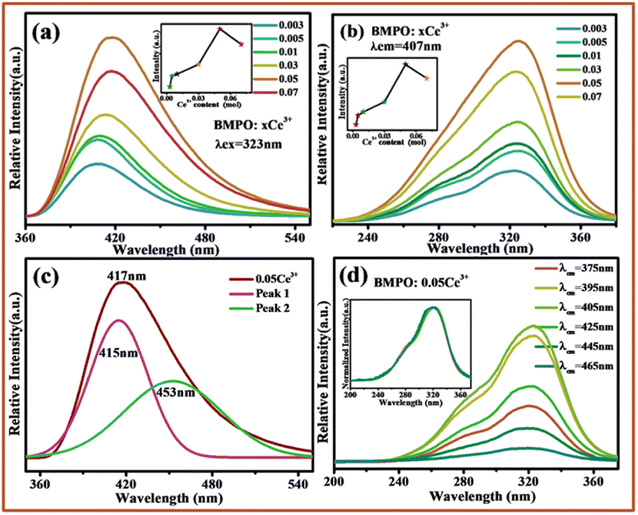 |
| Fig. 2 (a) Excitation spectra of Ba2Mg(PO4)2:xCe3+ (λem = 407 nm). (b) Emission spectra of Ba2Mg(PO4)2:xCe3+ (λex = 323 nm). (c) Emission spectra and the two sub-peaks of Ba2Mg(PO4)2:0.05Ce3+ (λex = 323 nm). (d) Excitation spectra of Ba2Mg(PO4)2:0.05Ce3+ for the different emission wavelengths, the insert describes the normalized excitation spectra. | |
3.2 Luminescence properties of Ba2Mg(PO4)2:Ce3+
Fig. 2a and b show the emission spectra and excitation spectra of BMPO:xCe3+(x = 0.003–0.07). It can be seen that under the excitation of 323 nm, the emission spectra of BMPO:xCe3+ show an asymmetric emission band with a peak around 407 nm, which originates from the transition from 5d to 4f ground state. The excitation spectrum recorded by monitoring the emission of 407 nm consists of a stronger excitation band centered at 323 nm, and the spectrum goes from 220 nm to 380 nm. The insets of Fig. 2a and b present the emission intensity of Ce3+ ions with its concentration, respectively. The intensities both increase with the increase of Ce3+ ion content. The intensity reaches the strongest at x = 0.05, and then the intensity gradually decreases due to the quenching effect of the activated ions. It is well known that Ce3+ emission should be composed of a double band due to the splitting of its 4f ground state, and the energy difference of this splitting between 2F7/2 and 2F5/2 of Ce3+ is about 2000 cm−1. In this study, the asymmetric emission band can be decomposed into two well-separated Gaussian components with maxima at 415 nm and 453 nm, as shown in Fig. 2c, and the energy difference between the two sub peaks is about 2021 cm−1, which is close to the theoretical energy difference between the 2F7/2 and 2F5/2 levels. In order to further determine the source of the two sub peaks, the excitation spectra of the sample BMPO:0.05Ce3+ at 375 nm, 395 nm, 405 nm, 425 nm, 445 nm and 465 nm were monitored, as shown in Fig. 2d. The inset shows the normalized excitation spectrum. It can be seen from the figure that, except for the difference in luminous intensity, the spectral types of all excitation spectra are completely the same, which indicates that the Ce3+ ion is very likely to have only one luminescence center. This proves the asymmetry emission peak of BMPO:0.05Ce3+ is caused by the spin–orbit coupling effect of Ce3+.
3.3 Luminescence properties of Ba2Mg(PO4)2:Eu2+
Fig. 3a shows the excitation and emission spectra of BMPO:0.07Eu2+. Upon excitation at 350 nm, BMPO:0.07Eu2+ exhibits a broad emission band extending from 450 nm to 750 nm, and the emission peak is located at about 580 nm due to the 4f0 5d1 → 4f1 transition of Eu2+ ion. Monitored at 580 nm, the excitation spectra give a band with peak center at about 340 nm in the wavelength range of 250–450 nm. Fig. 3b shows the emission spectra of BMPO:yEu2+ with different Eu2+ concentration. It can be seen that the emission spectra consist of a relatively symmetric broad band center at 580 nm. In order to clarify the influence of Eu2+ concentration on its luminescence performance, the normalized emission spectra of BMPO:yEu2+ are shown in Fig. 3c, when changed the concentrations of Eu2+ ions, the emission spectrum has not changed significantly, and there is no obvious red-blue shift. The emission intensities of BMPO:Eu2+ with different Eu2+ concentrations are shown in Fig. 3d. The emission intensities firstly increase with increasing the content of Eu2+, the emission intensity is maximized at x = 0.07, and then decrease due to the concentration quenching effect.
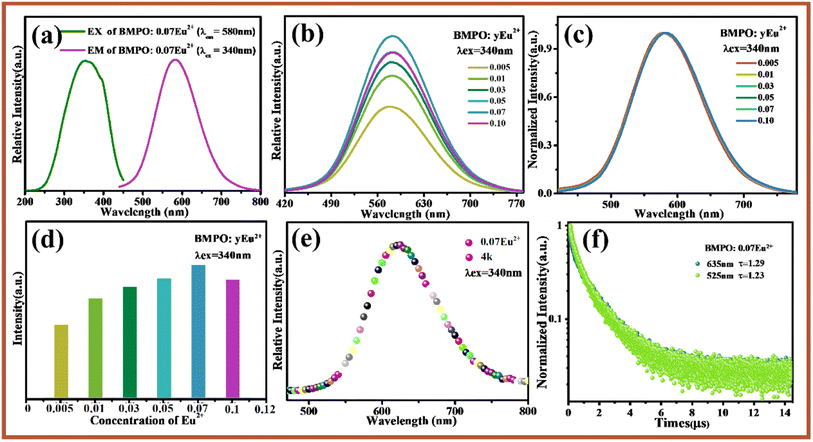 |
| Fig. 3 (a) Excitation spectra and emission spectra of Ba2Mg(PO4)2:0.07Eu2+. (b) Emission spectra of Ba2Mg(PO4)2:yEu2+ (λex = 340 nm). (c) The normalized emission spectra of Ba2Mg(PO4)2:yEu2+. (d) The emission intensity of Ba2Mg(PO4)2:yEu2+ with the different concentrations of Eu2+ ions. (e) The emission spectrum of Ba2Mg(PO4)2:0.07Eu2+ at 4 K. (f) The lifetime decay curve at 525 nm and 635 nm emission peaks of Ba2Mg(PO4)2:0.07Eu2+. | |
For BMPO, there are three cation sites, namely Ba1, Ba2 and Mg3. Among them, Ba1 is surrounded by eight O atoms, Ba2 is surrounded by seven O atoms, and Mg3 is surrounded by six oxygen atoms. Taking into account the degree of suitability of the doped ion and the substitute ion in radius and valence, there are only two lattice sites suitable for Eu2+ ions to enter, namely Ba1 and Ba2 sites. It can be found that the emission spectrum of Eu2+ ions is basically symmetrical, which indicates that Eu2+ ions are likely to occupy only one of the lattice sites and have only one luminescence center, which results in a relatively symmetrical emission spectrum. In order to clarify the number of luminescence centers, BMPO:0.07Eu2+ was subjected to a low-temperature spectrum test at 4 K and a fluorescence lifetime test at room temperature. Fig. 3e shows that the emission spectrum of BMPO:0.07Eu2+ has only one relatively symmetrical emission peak under the excitation of 340 nm, which proves that there is only one luminescence center. In order to further verify the number of luminescence centers, the decay curves of BMPO:0.07Eu2+ monitored at 525 and 635 nm were tested and are presented in Fig. 3f, and the lifetimes of them are 1.23 and 1.29 μs. From the results, it can be seen that the lifetimes of the two emission peaks are almost the same, which further verifies that there is only one luminescence center of Eu2+ ions in the BMPO.
Due to the special electronic configuration, Eu2+ ion is particularly sensitive to the crystal field environment surrounding the central ion. In order to obtain the detailed structural information of BMPO:yEu2+ and find out which lattice site Eu2+ ions enter, the structure of BMPO:yEu2+ (y = 0–0.1) by GSAS software based on the standard card of BMPO (ICSD#59253) were refined, and shown in Fig. S2 and Table S2.† It can be seen that all parameters meet the experimental requirements, which prove that the refinement results are true and reliable. The evolution of lattice parameters a, b, c and the unit cell volume V of BMPO:yEu2+(y = 0–0.1) is shown in Fig. S3.† It is found that parameters a, b, c and the unit cell volume decrease gradually with the increase of Eu2+ content, which is caused by lattice shrinkage caused by Eu2+ with smaller radius entering the lattice instead of Ba2+ with larger radius. At the same time, it also shows that Eu2+ ions are successfully doped in.
As shown in Fig. 4a, the refined crystal structure of BMPO:yEu2+(y = 0–0.1) was analyzed in detail and the volume changes of the lattice sites Ba1 and Ba2 were summarized. According to the previous analysis, the radius of Eu2+ is smaller than that of Ba2+. If Eu2+ successfully occupies a certain lattice site after entering the host, it will definitely cause a change in the volume of this site is shown in Fig. 4b. It can be seen from the Fig. 4a that the volume of Ba1 gradually decreases with the increase of Eu2+ concentration, which is caused by the entry of Eu2+ ions with a smaller radius into the site Ba1, while the volume of Ba2 shows a slight increase first and then a slight decrease. Through analysis, it is found that the lattice sites Ba1 and Ba2 are connected to each other through the sides and apex angles. When Eu2+ ions enter the Ba1 lattice site, the unit cell volume of Ba1 decreases, and the decrease of Ba1 also has a pulling effect on Ba2, so that the volume of Ba2 increases slightly. When the doping concentration of Eu2+ ions is large, it cannot completely enter the Ba1 site, and part of it will enter the gap in the lattice site, and the Eu2+ ions that enter the gap will Ba1 has a certain degree of squeezing effect, hence its unit cell volume is slightly reduced. In summary, Eu2+ ions do occupy the Ba1 site after entering the BMPO.
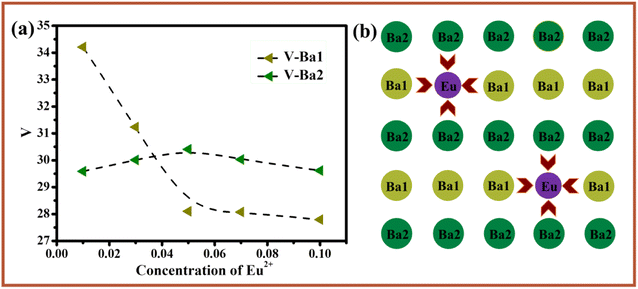 |
| Fig. 4 (a) The lattice volume Ba1 and Ba2 with Eu2+ doping concentration in Ba2Mg(PO4)2:yEu2+. (b) Schematic diagram of the lattice change after Eu2+ enters the host. | |
3.4 Luminescence properties of BMPO:0.05Ce3+, yEu2+
Fig. 5a shows the emission spectra of BMPO:0.05Ce3+, yEu2+ under excitation at 323 nm. Due to the 5d → 4f transition of Ce3+ and Eu2+, there are two emission bands from 370 nm to 520 nm with a peak at 407 nm, and from 540 nm to 720 nm with a peak at 580 nm. With increasing the concentration of Eu2+, the emission intensity of Ce3+ decreases monotonically. Meanwhile, the emission intensity of Eu2+ increases gradually until the Eu2+ content is x = 0.07 and the concentration quenching occurs is shown in Fig. 5b. In addition, the emission spectra of Ce3+ and excitation spectra of Eu2+ are also shown in Fig. 5c. A significant spectral overlap between the emission spectrum of BMPO:0.05Ce3+ and the excitation spectrum of BMPO:0.07Eu2+ is observed, which indicates that the energy transfer from the Ce3+ to Eu2+ ions can be expected in BMPO.
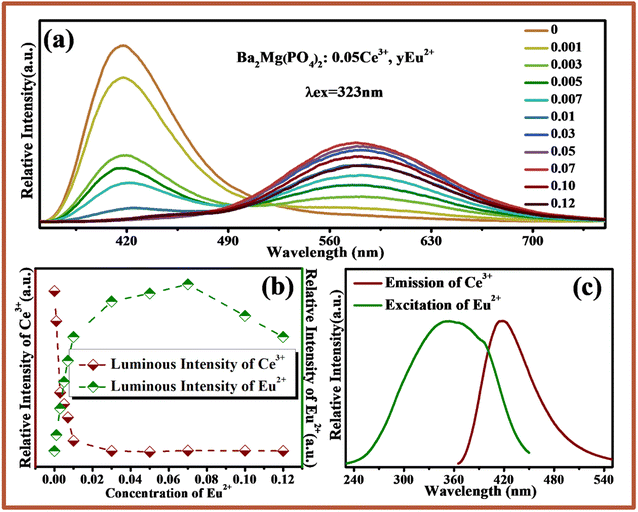 |
| Fig. 5 (a) The emission spectra of Ba2Mg(PO4)2:0.05Ce3+, yEu2+ (λex = 323 nm). (b) The luminous intensity of Eu2+ and Ce3+ varies with the concentration of Eu2+ ions. (c) The emission spectra of Ba2Mg(PO4)2:0.05Ce3+ and the excitation spectra of Ba2Mg(PO4)2:0.07Eu2+. | |
To further validate the energy transfer process between Ce3+ and Eu2+, the decay curves of Ce3+ fluorescence lifetime for BMPO:0.05Ce3+, yEu2+ were measured by monitoring at 407 nm with the excitation at 323 nm, and the measured decay curves are depicted in Fig. 6a. It is found that all the decay curves can be well fitted with a second-order exponential decay, which can be fitted using the eqn (1):38–40
|
I(t) = I0 + A1 exp(−t/τ1) + A2 exp(−t/τ2)
| (1) |
where
I is luminescence intensity,
A1 and
A2 are constants,
τ is average lifetime, and
τ1 and
τ2 are lifetime for rapid and slow decays, respectively. The average lifetime
τ* can be obtained using the
formula (2):
38–40 |
τ* = (A1τ12 + A2τ22)/(A1τ1 + A2τ2)
| (2) |
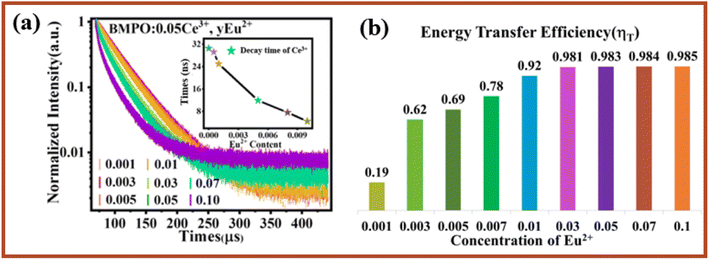 |
| Fig. 6 (a) Decay curves of Ce3+ ions in Ba2Mg(PO4)2:0.05Ce3+, yEu2+ (λex = 320 nm). (b) The energy transfer efficiency between Ce3+–Eu2+ in Ba2Mg(PO4)2:0.05Ce3+, yEu2+. | |
The effective decay time (τ*) of Ce3+ ions were calculated to be 32.0170, 30.5329, 29.2241, 25.0169, 11.9310, 8.3924, 7.5280 and 4.4988 ns, respectively. Obviously, the lifetime values decreased monotonically as the Eu2+ concentration increased, which strongly demonstrates energy transfer from Ce3+ to Eu2+. In addition, the energy transfer efficiency (ηT) can be calculated using the following eqn (3).41–43
where
Is and
Is0 are luminescence intensity of sensitizer Ce
3+ in the presence and absence of activator Eu
2+. As shown in
Fig. 6b, the energy-transfer efficiency increased sharply with the increasing of the Eu
2+ content. The
ηT value reached 98.5% at
y = 0.1, which also indicated that the energy transfer efficiency of Ce
3+–Eu
2+ can increase with increasing Eu
2+ content.
Based on the above argument, there are the energy transfer from Ce3+ to Eu2+ ions, hence the determination of their energy transfer mechanism is also an important factor to study their energy transfer process. In general, the exchange interaction and multipolar interaction are the main types of interactions between sensitizer and activator.44 With the increase of activator concentration, the distance between the sensitizer and the activator begins to approach, and there is an obvious energy transfer between the sensitizer and the activator. When the distance reaches the critical value, the energy transfer will be hindered. The critical distance RCe–Eu between Ce3+ and Eu2+ can be estimated by45,46
|
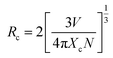 | (4) |
where
V is the volume in the unit cell,
N is the number of cations in the unit cell,
Xc is the total concentration of Ce
3+ ions and the critical concentration of Eu
2+. For BMPO host, the value of
V is 758.43 Å
3 and
N =
Z = 4, the critical concentration of Eu
2+ is 0.07. The critical distance of energy transfer is calculated to be about 14.3 Å for BMPO:0.05Ce
3+,
yEu
2+, which is larger than the critical distance for exchange interaction (5 Å). Therefore, the interaction between Ce
3+ and Eu
2+ ions in BMPO might take place
via multipolar interaction.
On the basis of Dexter's the formula of multipolar interaction and Reisfeld's approximation, the following relationship (5) can be obtained:47
|
 | (5) |
where
ISO and
IS are the luminescence intensity of Ce
3+ in the absence and presence of Eu
2+,
C is the total doping content of sensitizer (Ce
3+) and activator (Eu
2+), and
n = 6, 8, 10 corresponds to the dipole–dipole, dipole–quadrupole, and quadrupole–quadrupole interactions, respectively. The relationship of

is shown in
Fig. 7a–c. Considering the fitting factor
R2, the relation

has the best fitting, implying that the energy transfer between Ce
3+ and Eu
2+ in BMPO occurs
via a dipole–dipole interaction mechanism.
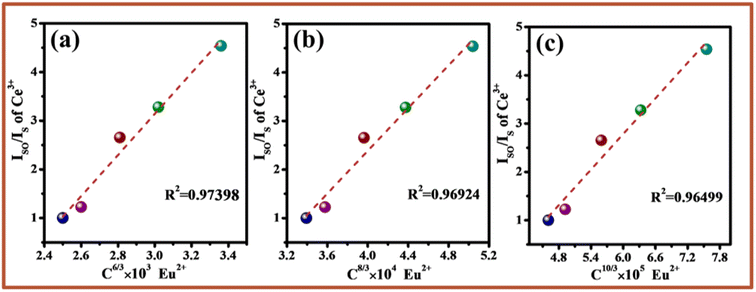 |
| Fig. 7 Relationships between IS0/IS and Cn/3: (a) n = 6, (b) n = 8, (c) n = 10. | |
Fig. 8 describes the energy level transitions of Ce3+ and Eu2+ ions and the energy transfer mechanism of Ce3+–Eu2+ in BMPO. Under the 323 nm excitation, the electrons of Ce3+ ions transition from the 4f energy level to the 5d energy level. Because the exposed 5d orbital of Ce3+ is susceptible to splitting by the crystal field, and it has two ground states the electron configurations are 2F7/2 and 2F5/2, respectively, hence they show a relatively wide emission range. At the same time, because the upper energy level of Eu2+ ions is lower than that of Ce3+ ions, they are excited to the 5d energy level. A part of the electrons will jump to the upper energy level of Eu2+ ion, and then return to the lower energy level and emit photon. Where εcfs denotes the 5d energy level cleavage of Eu2+ and ΔS is the Stokes shift, which is the energy transfer process of Ce3+–Eu2+.
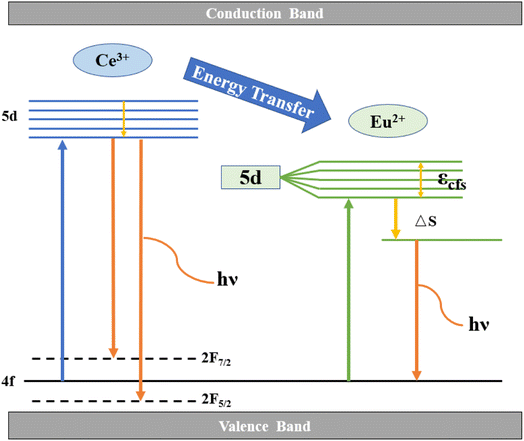 |
| Fig. 8 Energy level scheme for ET in Ba2Mg(PO4)2:0.05Ce3+, yEu2+. | |
The chromaticity calculation was performed based on the luminescence spectra of BMPO:0.05Ce3+, yEu2+ under the 323 nm excitation, and the calculation results of the CIE coordinates and a series of digital photographs upon the 365 nm UV lamp are shown in Fig. 9. As the doping concentrations of Eu2+ ions are from 0 to 0.1, the color coordinates (x, y) of BMPO:0.05Ce3+, yEu2+ shift from blue (0.157, 0.071) to yellow (0.452, 0.466). It is worth noting that the CIE chromaticity coordinates of BMPO:0.05Ce3+, 0.005Eu2+, BMPO:0.05Ce3+, 0.007Eu2+ and BMPO:0.05Ce3+, 0.01Eu2+ are (0.294, 0.257), (0.319, 0.279) and (0.352, 0.332), which are all located in the white light region. The results indicate that BMPO:0.05Ce3+, yEu2+ can be achieved by appropriately adjusting the ratio of Ce/Eu ions.
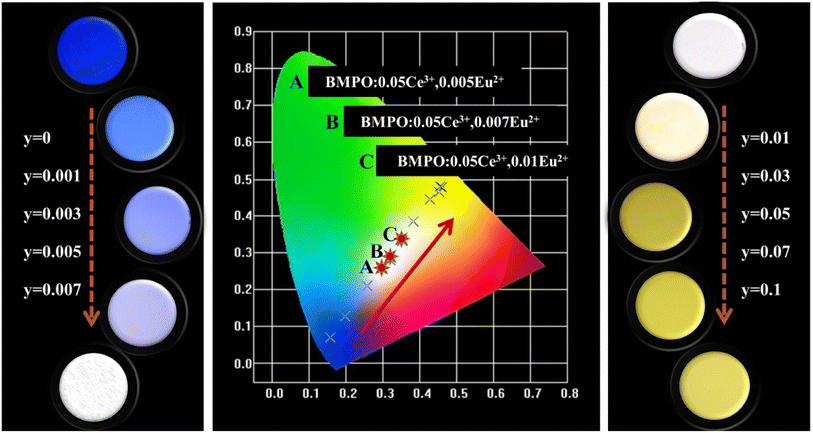 |
| Fig. 9 The color coordinates and photographs of Ba2Mg(PO4)2:0.05Ce3+, yEu2+ (λex = 365 nm). | |
4. Conclusions
In summary, series color-tunable and single-composition white emitting phosphors BMPO:0.05Ce3+, yEu2+ were successfully synthesized. The samples could get varied color emission from blue (0.157, 0.071) towards white (0.352, 0.332) and ultimately to yellow (0.452, 0.466) under the excitation of 323 nm light by precisely controlling the relative proportion of Ce3+/Eu2+. The energy transfer from Ce3+ to Eu2+ in BMPO has been validated and the energy transfer mechanism is the dipole–dipole interaction. The results proved that the single-phased white emitting phosphor BMPO:xCe3+, yEu2+ can be excited by UV-LED chips and has the potential application in white LEDs.
Conflicts of interest
The authors declare no competing financial interest.
Acknowledgements
The work is supported by the National Natural Science Foundation of China (No. 61805285).
References
- G. Li, Y. Tian, Y. Zhao and J. Lin, Recent Progress in Luminescence tuning of Ce3+ and Eu2+-activated phosphors for PC W-LEDs, Chem. Soc. Rev., 2015, 44, 8688–8713 RSC.
- J. Zhao, X. Sun and Z. Wang, Ce3+/Eu2+ doped SrSc2O4 phosphors: synthesis, luminescence and energy transfer from Ce3+ to Eu2+, Chem. Phys. Lett., 2018, 691, 68–72 CrossRef CAS.
- W. Lv, J. Huo, Y. Feng, S. Zhao and H. You, Photoluminescence, energy transfer and tunable color of Ce3+, Tb3+ and Eu2+ activated oxynitrides phosphor with high brightness, Dalton Trans., 2016, 45(23), 9676–9683 RSC.
- J. Ha, Z. Wang, E. Novitskaya, G. A. Hirata, O. A. Graeve, S. P. Ong and J. McKittrick, An integrated first principles and experimental investigation of the relationship between structural rigidity and quantum efficiency in phosphors for solid state lighting, J. Lumin., 2016, 179, 297–305 CrossRef CAS.
- C. Guo, Y. Xu, F. Lv and X. Ding, Luminescent properties of Sr2SiO4:Eu2+ nanorods for near-UV white LED, J. Alloys Compd., 2010, 497(1–2), L21–L24 CAS.
- G. Li, Y. Fan, H. Guo and Y. Wang, Synthesis, structure and photoluminescence properties of Ce3+-doped SrSc2O4: a new scandate green-emitting phosphor with blue excitation, New J. Chem., 2017, 41, 5565–5571 RSC.
- C. Guo, M. Li, Y. Xu, T. Li, Z. Ren and J. Bai, A potential green-emitting phosphor Ca8Mg(SiO4)4Cl2:Eu2+ for white light emitting diodes prepared by sol-gel method, Appl. Surf. Sci., 2011, 257(21), 8836–8839 CrossRef CAS.
- G. Li, Y. Zhao, Y. Wei, Y. Tian, Z. Quan and J. Lin, Novel yellowish-green light-emitting Ca10(PO4)6O: Ce3+ phosphor: structural refinement, preferential site occupancy and color tuning, Chem. Commun., 2016, 52, 3376–3379 RSC.
- J. Xiang, J. Zheng, Z. Zhou, H. Suo, X. Zhao, X. Zhou, N. Zhang, M. S. Molokeev and C. Guo, Enhancement of red emission and site analysis in Eu2+ doped new-type structure Ba3CaK(PO4)3 for plant growth white LEDs, Chem. Eng. J., 2019, 356, 236–244 CrossRef CAS.
- K. Uheda, N. Hirosaki, Y. Yamamoto, A. Naito, T. Nakajima and H. Yamamoto, Luminescence properties of a red phosphor, CaAlSiN3:Eu2+, for white light-emitting diodes, Electrochem. Solid-State Lett., 2006, 9, H22–H25 CrossRef CAS.
- P. Li, Z. Wang, Z. Yang and Q. Guo, Ba2B2O5:Ce3+: a novel blue emitting phosphor for white LEDs, Mater. Res. Bull., 2014, 60, 679–681 CrossRef CAS.
- Q. Wu, Z. Yang, Z. Zhao, M. Que, X. Wang and Y. Wang, Synthesis, crystal structure and luminescence properties of a Y4Si2O7N2: Ce3+ phosphor for near-UV white LEDs, J. Mater. Chem. C, 2014, 2, 4967–4973 RSC.
- R. Yu, C. Guo, T. Li and Y. Xu, Preparation and luminescence of blue-emitting phosphor Ca2PO4Cl: Eu2+ for n-UV white LEDs, Curr. Appl. Phys., 2013, 13(5), 880–884 CrossRef.
- W. Geng, X. Zhou, J. Ding, G. Li and Y. Wang, K7Ca9[Si2O7]4F:Ce3+: a novel blue-emitting phosphor with good thermal stability for ultraviolet-excited light emitting diodes, J. Mater. Chem. C, 2017, 5, 11605–11613 RSC.
- X. Ding and Y. Wang, Structure and photoluminescence properties of a novel apatite green phosphor Ba5(PO4)2SiO4: Eu2+ excited by NUV light, Phys. Chem. Chem. Phys., 2017, 19, 2449–2458 RSC.
- X. Wang, Z. Zhao, Q. Wu, C. Wang, Q. Wang, Y. Li and Y. Wang, Structure, photoluminescence and abnormal thermal quenching behavior of Eu2+-doped Na3Sc2(PO4)3: a novel blue-emitting phosphor for n-UV LEDs, J. Mater. Chem. C, 2016, 4, 8795–8801 RSC.
- X. Wang, Z. Zhao, Q. Wu, Y. Li and Y. Wang, Synthesis, structure and photoluminescence properties of Ca2LuHf2(AlO4)3: Ce3+, a novel garnet-based cyan lightemitting phosphor, J. Mater. Chem. C, 2016, 4, 11396–11403 RSC.
- Y. Liu, J. Zhang, C. Zhang, J. Xu, G. Liu, J. Jiang and H. Jiang, Ba9Lu2Si6O24: Ce3+: An Efficient Green Phosphor with High Thermal and Radiation Stability for Solid-State Lighting, Adv. Opt. Mater., 2015, 3(8), 1096–1101 CrossRef CAS.
- Z. Wang, B. Yang, P. Li, Z. Yang and Q. Guo, Energy transfer between activators at different crystallographic sites in Sr3SiO5: Eu2+, Physica B, 2012, 407(8), 1282–1286 CrossRef CAS.
- M. Zhang, J. Wang, Q. Zhang, W. Ding and Q. Su, Optical properties of Ba2SiO4:Eu2+ phosphor for green light emitting diode (LED), Mater. Res. Bull., 2007, 42, 33–39 CrossRef CAS.
- W. Lv, Y. Jia, Q. Zhao, M. Jiao, B. Shao, W. Lu and H. You, Crystal Structure and Luminescence Properties of Ca8Mg3Al2Si7O28: Eu2+ for WLEDs, Adv. Opt. Mater., 2014, 2, 183–188 CrossRef.
- M. Zhao, Z. Xia, M. S. Molokeev, L. Ning and Q. Liu, Temperature and Eu2+ doping induced phase selection in NaAlSiO4 polymorphs and the controlled yellow/blue emission, Chem. Mater., 2017, 29, 6552–6559 CrossRef CAS.
- Z. Xia, R. Liu, K. Huang and V. Drozd, Ca2Al3O6F: Eu2+: a green-emitting oxyfluoride phosphor for white lightemitting diodes, J. Mater. Chem., 2012, 22, 15183–15189 RSC.
- J. Qiao, L. Ning, M. S. Molokeev, Y. Chuang, Q. Liu and Z. Xia, Eu2+ site preferences in the mixed cation K2BaCa(PO4)2 and thermally stable luminescence, J. Am. Chem. Soc., 2018, 140(30), 9730–9736 CrossRef CAS PubMed.
- W. Sun, Y. Jia, R. Pang, H. Li, T. Ma, D. Li, J. Fu, S. Zhang, L. Jiang and C. Li, Sr9Mg1.5(PO4)7: Eu2+: a novel broadband orange-yellow-emitting phosphor for blue light-excited warm white LEDs, ACS Appl. Mater. Interfaces, 2015, 7(45), 25219–25226 CrossRef CAS PubMed.
- D. Zhao, S.-R. Zhang, Y.-P. Fan, B.-Z. Liu and R.-J. Zhang, Thermally stable phosphor KBa2(PO3)5: Eu2+ with broadband cyan emission caused by multisite occupancy of Eu2+, Inorg. Chem., 2020, 59, 8789–8799 CrossRef CAS PubMed.
- S. Liang, P. Dang, G. Li, M. S. Molokeev, Y. Wei, Y. Wei, H. Lian, M. Shang, A. A. Al Kheraiff and J. Lin, Controllable two-dimensional luminescence tuning in Eu2+, Mn2+ doped (Ca,Sr)9Sc(PO4)7 based on crystal field regulation and energy transfer, J. Mater. Chem. C, 2018, 6, 6714–6725 RSC.
- C. Wang, P. Li, Z. Wang, Y. Sun, J. Cheng, Z. Li, M. Tian and Z. Yang, Crystal structure, luminescence properties, energy transfer and thermal properties of a novel color-tunable, white light-emitting phosphor Ca9−x−yCe(PO4)7:xEu2+, yMn2+, Phys. Chem. Chem. Phys., 2016, 18, 28661–28673 RSC.
- X. Li, P. Li, C. Liu, L. Zhang, Z. Xing, Z. Yang and Z. Wang, Tuning the luminescence of Ca9La(PO4)7: Eu2+ via artificially inducing potential luminescence centers, J. Mater. Chem. C, 2019, 7, 14601–14611 RSC.
- X. Fu, S. Zheng, Y. Liu and H. Zhang, Yellow phosphors Ba2Mg(Po4)2: Eu2+ fit for white light-emitting diodes prepared with sol-gel precursor route, J. Lumin., 2018, 206, 120–124 CrossRef.
- S. K. Ramteke, N. S. Kokode, A. N. Yerpude, G. N. Nikhare and S. J. Dhoble, Synthesis and photoluminescence properties of Eu3+ activated Ba2Mg(Po4)2 phosphor, J. Lumin., 2020, 35(4), 618–621 CrossRef CAS PubMed.
- Q. Hu, Z. Pan, Y. Xu, L. Wang and L. Ning, Dopant site environment and spectrum blue shift of yellow-emitting solid solution phosphor Ba2xSrxMg(Po4)2:Eu2+, J. Am. Ceram. Soc., 2016, 99(2), 645–650 CrossRef CAS.
- H. Song, C. Lee, S. Hwang and I. Cho, Wet-chemical preparation of barium magnesium orthophosphate, Ba2Mg(Po4)2:Eu2+, nanorod phosphor with enhanced optical and photoluminescence properties, RSC Adv., 2016, 6, 61378–61385 RSC.
- S. Xu, K. Tang, D. Zhu and T. Han, Luminescence properties and energy transfer of Ba2Mg(PO4)2:Eu2+, Mn2+ phosphor synthesized by co-precipitation method, Opt. Mater., 2015, 42, 106–110 CrossRef CAS.
- Z. Yang, S. Ma, H. Yu, F. Wang, X. Ma, Y. Liu and P. Li, Luminescence studies of Ba1-xMg2-y(Po4)2:xEu2+, yMn2+ phosphor, J. Alloys Compd., 2011, 509, 76–79 CrossRef CAS.
- J. Zhang, B. Ji and H. Zheng, Investigations on the luminescence of Ba2Mg(PO4)2:Eu2+, Mn2+ phosphors for LEDs, Opt. Mater. Express, 2016, 6(11), 3470–3475 CrossRef CAS.
- Q. S. Hu, Z. F. Pan, Y. Xu., L. L. Wang and L. X. Ning, Dopant Site Environment and Spectrum Blue Shift of Yellow-Emitting Solid Solution Phosphor Ba2−xSrxMg(PO4)2:Eu2+, J. Am. Ceram. Soc., 2016, 99(2), 645–650 CrossRef CAS.
- X. Zhang, J. Xu, Z. Guo and M. Gong, Luminescence and energy transfer of dual-emitting solid solution phosphors (Ca, Sr)10Li(PO4)7: Ce3+, Mn2+ for ratiometric temperature sensing, Ind. Eng. Chem. Res., 2017, 56, 890–898 CrossRef CAS.
- M. Li, J. Zhang, J. Han, Z. Qiu, W. Zhou, L. Yu, S. Lian and Z. Li, Changing Ce3+ content and codoping Mn2+ induced tunable emission and energy transfer in Ca2.5Sr0.5Al2O6: Ce3+, Mn2+, . Inorg. Chem., 2017, 56, 241–251 CrossRef CAS PubMed.
- K. Li, H. Lian, M. Shang and J. Lin, A novel greenish yellow-orange red Ba3Y4O9: Bi3+, Eu3+ phosphor with efficient energy transfer for UV-LEDs, Dalton Trans., 2015, 44, 2054–20550 Search PubMed.
- W. Yang and T. Chen, White-light generation and energy transfer in SrZn2(PO4)2: Eu, Mn phosphor for ultraviolet light-emitting diodes, Appl. Phys. Lett., 2006, 88, 101903 CrossRef.
- K. H. Kwon, W. B. Im, H. S. Jang, H. S. Yoo and D. Y. Jeon, Luminescence properties and energy transfer of site-sensitive Ca6−x−yMgx−z(PO4)4: yEu2+, zMn2+ phosphors and their application to near-UV LED based white LEDSs, Inorg. Chem., 2009, 48, 11525–11532 CrossRef CAS PubMed.
- G. Li, Y. Zhang, D. Geng, M. Shang, C. Peng, Z. Cheng and J. Lin, Single-composition trichromatic white-emitting Ca4Y6(SiO4)6O:Ce3+/Mn2+/Tb3+ phosphor: luminescence and energy transfer, ACS Appl. Mater. Interfaces, 2012, 4, 296–305 CrossRef CAS PubMed.
- D. L. Dexter and J. H. Schulman, Theory of concentration quenching in inorganic phosphors, J. Chem. Phys., 1954, 22(6), 1063–1070 CrossRef CAS.
- D. Geng, G. Li, M. Shang, D. Yang, Y. Zhang, Z. Cheng and J. Lin, Color tuning via energy transfer in Sr3In(PO4)3:Ce3+/Tb3+/Mn2+ phosphors, J. Mater. Chem., 2012, 22(28), 14262–14271 RSC.
- M. Cui, J. Wang, J. Li, S. Huang and M. Shang, An abnormal yellow emission and temperature-sensitive properties for perovskite-type Ca2MgWO6 phosphor via cation substitution and energy transfer, J. Lumin., 2019, 214, 116588 CrossRef CAS.
- M. Shang, G. Li, D. Geng and D. Yang, J. Lin. Blue emitting Ca8La2(PO4)6O2:Ce3+/Eu2+ phosphors with high color purity and brightness for White LED: Soft-chemical synthesis, luminescence, and energy transfer properties, J. Phys. Chem. C, 2012, 116(18), 10222–10231 CrossRef CAS.
|
This journal is © The Royal Society of Chemistry 2022 |