DOI:
10.1039/D2RA06070A
(Paper)
RSC Adv., 2022,
12, 34496-34502
Decarboxylative ring-opening of 2-oxazolidinones: a facile and modular synthesis of β-chalcogen amines†
Received
26th September 2022
, Accepted 18th November 2022
First published on 30th November 2022
Abstract
We report herein the synthesis of primary and secondary β-chalcogen amines through the regioselective ring-opening reaction of non-activated 2-oxazolidinones promoted by in situ generated chalcogenolate anions. The developed one-step protocol enabled the preparation of β-selenoamines, β-telluroamines and β-thioamines with appreciable structural diversity and in yields of up to 95%.
Introduction
Organochalcogen compounds (OCs) are known for their notable applications in the fields of organic synthesis,1 catalysis,2 and materials science.3 In addition, a variety of biological activities are associated with OCs,4 making them promising targets in the search for new therapeutic agents.5 Notably, many of these valuable OCs contain a nitrogen atom close to the chalcogen in their structures, including β-chalcogen amines (β-CAs, shown in Fig. 1A). Examples of naturally occurring β-CAs include the proteinogenic amino acids L-cysteine and L-selenocysteine, which play crucial roles in the catalytic activity of enzymes such as cruzain6 and glutathione peroxidase (GPx),7 respectively (Fig. 1B). At the same time, synthetic β-CAs are known as a class of bioactive compounds,8 and have been used as chiral ligands in asymmetric catalysis9 (Fig. 1C).
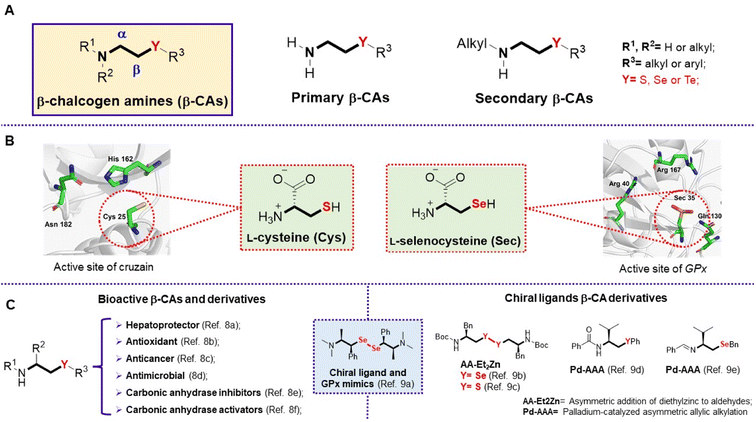 |
| Fig. 1 (A) Structure of β-chalcogen amines; (B) structures of L-cysteine and L-selenocysteine; (C) examples of relevant synthetic β-Cas and their application fields. | |
Although the β-CA backbone is apparently simple, its preparation poses significant synthetic challenges, especially when the goal is to obtain primary amines (i.e. free amino group). In this sense, the substitution of 2-haloethylamines by chalcogenolate anions offers a direct route.10 However, the structural variety commercially available for 2-haloethylamines is relatively limited, particularly regarding optically active substrates. β-Amino alcohols can also be used as starting materials for the synthesis of β-CAs,11 but they require the use of protecting groups which is a disadvantage. While regioselective aminochalcogenation of alkenes has been shown to be a promising alternative for the synthesis of β-CAs, most reports are still limited to the use of soft-conjugated nitrogenous nucleophiles.12 Ring-opening of N-H-aziridines, in contrast, is a well-established strategy for the synthesis of primary β-CAs,8f,13 but the toxic and carcinogenic properties associated with these starting materials are a major concern.14
In view of these limitations, 2-oxazolidinones offer a safer option acting as latent aziridine equivalents, since they can undergo decarboxylative ring-opening reaction to provide aminoethylated products.15 Although there are some reports on the synthesis of primary β-thioamines using this strategy (Scheme 1A),16 there is a remarkable lack of synthetic studies for β-selenoamines and β-telluroamines. To the best of our knowledge, there is only one prior report on the preparation of selenium and tellurium analogues via the ring-opening reaction of 2-oxazolidinones (Scheme 1B).17 And even in this report, the protocol was restricted to the use of N-activated 2-oxazolidinones, which does not allow for the production of primary and secondary amines. To address this clear gap and the need for more versatile synthetic routes, we focused our efforts on establishing a new methodology to allow a simple, rapid and modular synthesis of β-CAs from non-activated 2-oxazolidinone rings (Scheme 1C).
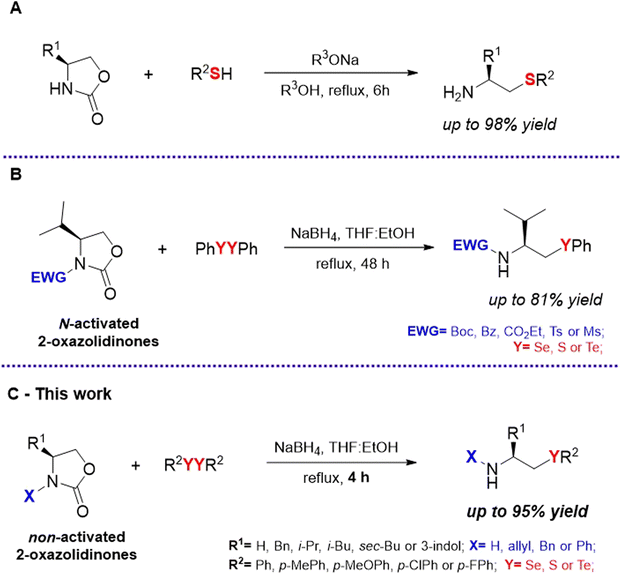 |
| Scheme 1 Preparation of β-CAs and derivatives via 2-oxazolidinone decarboxylative ring-opening strategy: (A) synthesis of β-thioamines; (B) synthesis of β-CA derivatives from N-activated 2-oxazolidinones; (C) synthesis of β-CAs from non-activated 2-oxazolidinones (this work). | |
Results and discussion
To find suitable conditions to carry out the reaction, we selected (S)-4-benzyl-2-oxazolidinone 1a as the standard substrate and diphenyl diselenide as the source of the selenolate anion (Table 1). We also chose NaBH4 in THF
:
EtOH as it is an established system for in situ generation of chalcogenolate anions (Table 1, entries 1–8). First, we sought to determine an appropriate amount of NaBH4 to promote the opening reaction (entries 1–4), and we observed that the use of 3.0 equiv. produced compound 2a in 85% yield (entry 4). Next, we focused on determining the time required for the reaction completion (entries 5–7) with the best yield for 2a being obtained after 4 h of reaction (entry 6). Notably, the use of absolute ethanol instead of 95% ethanol led to a drastic decrease in the product yield (entry 8). This result suggests that water is crucial for this reaction, probably acting as the main source of protons to promote the decarboxylation step. To verify this hypothesis, we performed a new test with absolute ethanol, but this time by adding together a small volume of distilled water, which resulted in a significant increase in the yield of the desired product (entry 9). We also evaluated the possibility of replacing ethanol with water, but substituting the cosolvent was found to be detrimental due to the lower miscibility of water in THF (entries 10 and 11). Finally, our attempts to use methanol instead of ethanol (entry 12) as well as to conduct the reaction in ethanol alone (entry 13) did not improve the yield. As determined by chiral HPLC analysis, the ring-opening reaction proceeds with full preservation of the stereochemical integrity of the initial 2-oxazolidinone 1a (see ESI†).
Table 1 Optimization of the reaction conditionsa
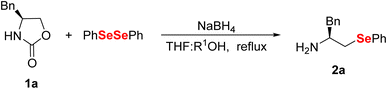
|
Entry |
NaBH4c (equiv.) |
Time (h) |
R1 |
Yieldb (%) |
Standard reaction conditions: 2-oxazolidinone 1a (1.0 mmol), diphenyl diselenide (1.2 equiv., 0.60 mmol), NaBH4, THF (7.0 mL) and R1OH (0.6 mL) under reflux and nitrogen atmosphere. Isolated yields. Regarding the limiting agent, 1a. 95% ethanol. Absolute ethanol was used instead of 95% ethanol. 0.55 mL of absolute ethanol + 0.05 mL of distilled water. 0.6 mL of distilled water was used. 0.1 mL of distilled water was used. 95% methanol. THF was omitted and 7.6 mL of 95% EtOH was employed. n.d. = not determined. |
1 |
0.6 |
12 |
Etd |
n.d. |
2 |
1.2 |
12 |
Etd |
n.d. |
3 |
2.0 |
12 |
Etd |
48 |
4 |
3.0 |
12 |
Etd |
85 |
5 |
3.0 |
6 |
Etd |
89 |
6 |
3.0 |
4 |
Etd |
87 |
7 |
3.0 |
3 |
Etd |
73 |
8 |
3.0 |
4 |
Ete |
11 |
9 |
3.0 |
4 |
Etf |
83 |
10 |
3.0 |
4 |
Hg |
n.d. |
11 |
3.0 |
4 |
Hh |
73 |
12 |
3.0 |
4 |
Mei |
75 |
13 |
3.0 |
4 |
Etj |
n.d. |
After determining suitable reaction conditions (Table 1, entry 6), the method was extended to the synthesis of a library of β-selenoamines 2 (Table 2). Initially, a few N-H-2-oxazolidinones derived from L-amino acids were screened to exemplify the possibilities of structural diversification in the R1 moiety (2a–2f). Then, different diaryl diselenides were used to examine the influence of electronic effects on the selenolate anion (2g–2j). Remarkably, halogenated derivatives 2i and 2j were prepared in 77% and 67% of yield respectively, which is very interesting given the versatility of these blocks. In view of the challenge involved in the monoalkylation of amines, we also attempted to the use of N-alkylated-2-oxazolidinones, aiming to obtain secondary β-selenoamines. Surprisingly, reactions with N-allyl-2-oxazolidinones selectively furnished the corresponding ring-opening products 2k and 2n in 91% and 86% of yield, respectively. Comparable yields were obtained in the cases of N-benzyl-β-selenoamines 2l and 2o, while the L-valine derivative 2q was obtained in moderate yield. Finally, we evaluated the possibility of preparing selenium-containing anilines from N-phenyl-2-oxazolidinones, and the corresponding products 2m and 2p were obtained in 89% and 95% of yield, respectively.
Table 2 Synthesis of β-selenoamines 2a,b
Reaction scale: 2-oxazolidinone 1 (1.0 mmol), diorganoyl diselenide (1.2 equiv., 0.60 mmol), NaBH4 (3.0 equiv., 3.0 mmol, 0.114 g), THF (7.0 mL) and EtOH (95.0%, 0.6 mL). Isolated yields. |
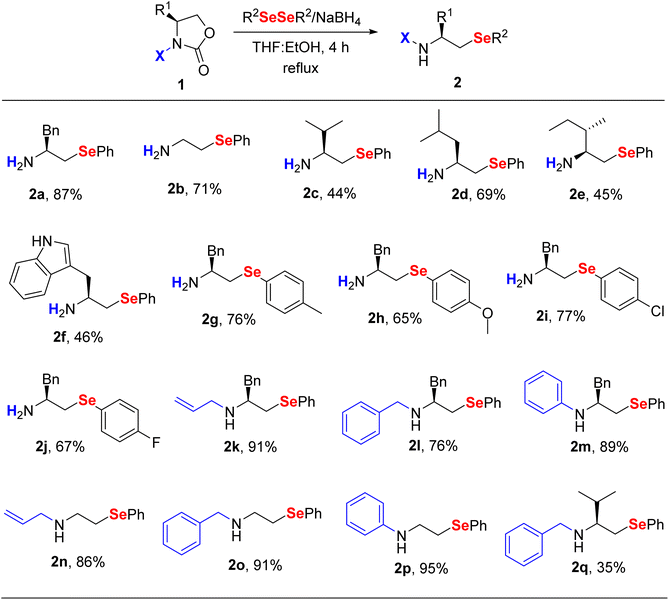 |
To further evaluate the scope and limitations, the established protocol was extended to the synthesis of β-telluroamines 3 and β-thioamines 4 (Table 3). In particular, primary β-telluroamines were obtained in good to excellent yields in just one step (15–89%, compounds 3a–3e), with the L-valine derivative 3b being an exception. The generality of the developed method could be demonstrated through the preparation of 25 examples of primary and secondary β-thioamines (4a–4y). It can be noted that the use of disulfides as source of thiolate anions instead of thiols contributes to the establishment of a safer protocol, avoiding the handling of volatile, corrosive and malodorous reagents.18 In general, we observed that the ring-opening reaction proceeded better with tellurium and selenium than with sulfur anions, which is consistent with the relative nucleophilicity of these species. Moreover, the steric hindrance caused by the neighboring R1 group proved to be significant, given that L-valine and L-isoleucine derivatives were normally obtained in lower yields. We also observed that secondary β-CAs were generally obtained in higher yields than their primary analogues, suggesting that electron-donating groups attached to nitrogen may also improve the reaction.
Table 3 Synthesis of β-telluroamines 3 and β-thioamines 4a,b
Reaction scale: 2-oxazolidinone 1 (1.0 mmol), diorganoyl dichalcogenide (1.2 equiv., 0.60 mmol), NaBH4 (3.0 equiv., 3.0 mmol, 0.114 g), THF (7.0 mL) and EtOH (95.0%, 0.6 mL). Isolated yields. |
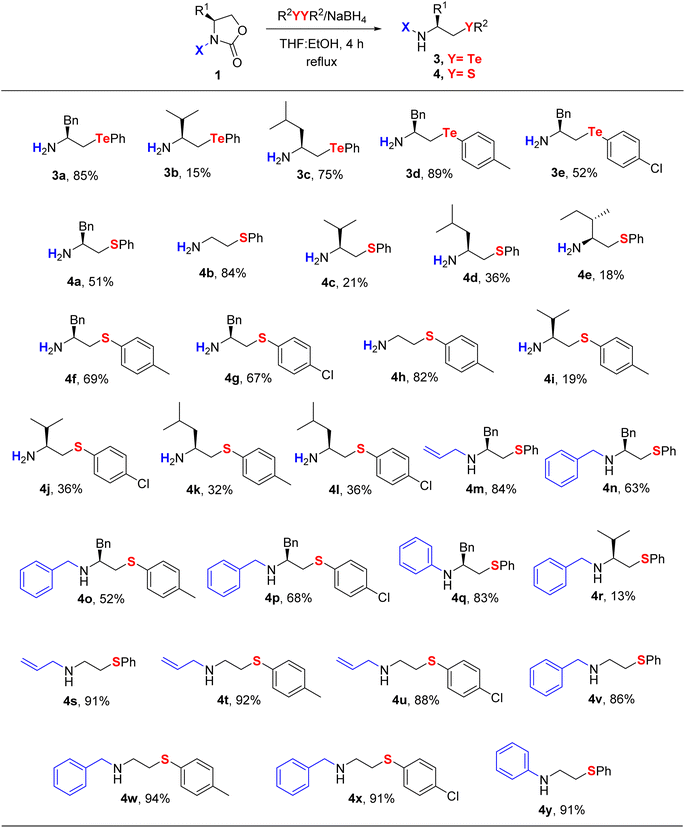 |
In view of the potential applications of β-CAs and derivatives,8,9 we also carried out a scale-up study for this reaction, and the results are compiled in Table 4. Seeking to develop a more economical and environmentally sustainable process, we focused our efforts on reducing the amount of solvent employed in these experiments. In this sense, we observed that the use of 2 mL of solvent for 1 mmol of oxazolidinone was sufficient to provide the desired products in good yields. Furthermore, the β-CAs obtained from these studies were purified by acid-base extractions, showing that the chromatographic purification can be bypassed for ethanolamine derivatives (see procedure in ESI†). Fortunately, we found that reactions carried out at 30 mmol or 60 mmol of 1b provided the products 2b, 4b, and 4h in yields comparable to those obtained in milligram-scale reactions.
Table 4 Scale-up study of the 2-oxazolidinone ring opening-reactiona
Isolated yields. Reaction scale: 2-oxazolidinone 1b (30 mmol, 2.612 g), diorganoyl dichalcogenide (18 mmol), NaBH4 (90 mmol, 3.420 g), THF (210 mL) and EtOH (95.0%, 18 mL). Reaction scale: 2-oxazolidinone 1b (60 mmol, 5.224 g), diorganoyl dichalcogenide (36 mmol), NaBH4 (180 mmol, 6.840 g), THF (420 mL) and EtOH (95.0%, 36 mL). Reaction scale: 2-oxazolidinone 1b (30 mmol, 2.612 g), diorganoyl dichalcogenide (18 mmol), NaBH4 (90 mmol, 3.420 g), THF (56 mL) and EtOH (95.0%, 4.8 mL). Reaction scale: 2-oxazolidinone 1b (60 mmol, 5.224 g), diorganoyl dichalcogenide (36 mmol), NaBH4 (180 mmol, 6.840 g), THF (112 mL) and EtOH (95.0%, 9.6 mL). |
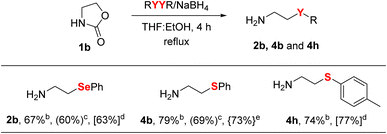 |
Conclusions
In summary, we have developed a simple and efficient methodology for the synthesis of primary and secondary β-CAs through the decarboxylative ring-opening reaction of non-activated 2-oxazolidinones. This protocol proved to be applicable to a wide range of 2-oxazolidinone rings and diorganoyl dichalcogenides, providing quick access to structurally diverse β-CAs with good yields in most cases. Studies related to the determination of the precise reaction mechanism and on new biological applications for β-CAs are ongoing in our laboratories, as well as the possible application of these β-CAS as building blocks for functional materials.
Conflicts of interest
There are no conflicts to declare.
Acknowledgements
The authors gratefully acknowledge FAPESC, CAPES and CNPq (grant 457479/2014-0) for financial support. X. Su acknowledges support by the National Science Foundation (NSF) under CBET Grant # 1942971. We also express our gratitude to Ajinomoto do Brasil for the kind donation of amino acids. Finally, we thank CEBIME-UFSC for the HRMS analyzes.
References
-
(a) Z. Rappoport, J. F. Liebman, I. Marek and S. Patai, The Chemistry of Organic Selenium and Tellurium Compounds, 2014, https://www.wiley.com/en-us/The+Chemistry+of+Organic+Selenium+and+Tellurium+Compounds%2C+Volume+4%2C+Parts+1+and+2+Set-p-9781118336939 Search PubMed;
(b) T. Wirth, Organoselenium Chemistry: Synthesis and Reactions, 2012, https://www.wiley.com/enus/Organoselenium+Chemistry%3A+Synthesis+and+Reactions-p-9783527641956 Search PubMed;
(c) T. Wirth, Angew. Chem., Int. Ed., 2000, 39, 3740–3749 CrossRef CAS.
-
(a) D. M. Freudendahl, S. Santoro, S. A. Shahzad, C. Santi and T. Wirth, Angew. Chem., Int. Ed., 2009, 48, 8409–8411 CrossRef CAS PubMed;
(b) M. Godoi, M. W. Paixão and A. L. Braga, Dalton Trans., 2011, 40, 11347–11355 RSC;
(c) J. Trenner, C. Depken, T. Weber and A. Breder, Angew. Chem., Int. Ed., 2013, 52, 8952–8956 CrossRef CAS PubMed.
-
(a) G. C. Hoover and D. S. Seferos, Chem. Sci., 2019, 10, 9182–9188 RSC;
(b) M. Jeffries-El, B. M. Kobilka and B. J. Hale, Macromolecules, 2014, 47, 7253–7271 CrossRef CAS;
(c) Z. Lou, P. Li and K. Han, Acc. Chem. Res., 2015, 48, 1358–1368 CrossRef CAS PubMed;
(d) S. A. Gregory, A. K. Menon, S. Ye, D. S. Seferos, J. R. Reynolds and S. K. Yee, Adv. Energy Mater., 2018, 8, 1802419 CrossRef.
-
(a) G. Mugesh and H. B. Singh, Chem. Soc. Rev., 2000, 29, 347–357 RSC;
(b) G. Mugesh, W. W. Du Mont and H. Sies, Chem. Rev., 2001, 101, 2125–2180 CrossRef CAS PubMed;
(c) C. W. Nogueira, G. Zeni and J. B. T. Rocha, Chem. Rev., 2004, 104, 6255–6286 CrossRef CAS PubMed.
-
(a) C. Santi, C. Tidei, C. Scalera, M. Piroddi and F. Galli, Curr. Chem. Biol., 2013, 7, 25–36 CrossRef CAS;
(b) D. Manna, G. Roy and G. Mugesh, Acc. Chem. Res., 2013, 46, 2706–2715 CrossRef CAS PubMed;
(c) S. Kumar, J. Yan, J. Poon, V. P. Singh, X. Lu, M. K. Ott, L. Engman and S. Kumar, Angew. Chem., Int. Ed., 2016, 55, 3729–3733 CrossRef CAS PubMed;
(d) H. J. Reich and R. J. Hondal, ACS Chem. Biol., 2016, 11, 821–841 CrossRef CAS PubMed;
(e) Z. Jin, X. Du, Y. Xu, Y. Deng, M. Liu, Y. Zhao, B. Zhang, X. Li, L. Zhang, C. Peng, Y. Duan, J. Yu, L. Wang, K. Yang, F. Liu, R. Jiang, X. Yang, T. You, X. Liu, X. Yang, F. Bai, H. Liu, X. Liu, L. W. Guddat, W. Xu, G. Xiao, C. Qin, Z. Shi, H. Jiang, Z. Rao and H. Yang, Nature, 2020, 582, 289–293 CrossRef CAS PubMed.
-
(a) M. E. McGrath, A. E. Eakin, J. C. Engel, J. H. McKerrow, C. S. Craik and R. J. Fletterick, J. Mol. Biol., 1995, 247, 251–259 CrossRef CAS PubMed;
(b) J. J. Cazzulo, V. Stoka and V. Turk, Curr. Pharm. Des., 2001, 7, 1143–1156 CrossRef CAS PubMed;
(c) M. Sajid and J. H. McKerrow, Mol. Biochem. Parasitol., 2002, 120, 1–21 CrossRef CAS PubMed.
-
(a) L. Flohe, W. A. Günzler and H. H. Schock, FEBS Lett., 1973, 32, 132–134 CrossRef CAS PubMed;
(b) J. T. Rotruck, A. L. Pope and H. E. Ganther, Nutr. Rev., 1980, 38, 280–283 CrossRef PubMed;
(c) G. V. Kryukov, S. Castellano, S. V. Novoselov, A. V. Lobanov, O. Zehtab, R. Guigó and V. N. Gladyshev, Science, 2003, 300, 1439–1443 CrossRef CAS;
(d) V. M. Labunskyy, D. L. Hatfield and V. N. Gladyshev, Physiol. Rev., 2014, 94, 739–777 CrossRef CAS PubMed.
-
(a) S. T. Stefanello, D. D. Hartmann, G. P. Amaral, A. A. Courtes, M. T. B. Leite, T. C. da Silva, D. F. Gonçalves, M. B. Souza, P. C. da Rosa, L. Dornelles and F. A. A. Soares, J. Biochem. Mol. Toxicol., 2017, 31, e21974 CrossRef PubMed;
(b) A. S. Prestes, S. T. Stefanello, S. M. Salman, A. M. Pazini, R. S. Schwab, A. L. Braga, N. B. V. Barbosa and J. B. T. Rocha, Mol. Cell. Biochem., 2012, 365, 85–92 CrossRef PubMed;
(c) D. Plano, D. N. Karelia, M. K. Pandey, J. E. Spallholz, S. Amin and A. K. Sharma, J. Med. Chem., 2016, 59, 1946–1959 CrossRef CAS PubMed;
(d) J. Vargas, S. Narayanaperumal, K. Gul, B. B. Ravanello, L. Dornelles, L. C. Soares, C. F. S. Alves, T. Schneider, R. A. Vaucher, R. C. V. Santos and O. E. D. Rodrigues, Tetrahedron, 2012, 68, 10444–10448 CrossRef CAS;
(e) A. Angeli, D. Tanini, A. Capperucci and C. T. Supuran, ACS Med. Chem. Lett., 2017, 8, 1213–1217 CrossRef CAS PubMed;
(f) D. Tanini, A. Capperucci, C. T. Supuran and A. Angeli, Bioorg. Chem., 2019, 87, 516–522 CrossRef CAS PubMed.
-
(a) L. C. Soares, E. E. Alberto, R. S. Schwab, P. S. Taube, V. Nascimento, O. E. D. Rodrigues and A. L. Braga, Org. Biomol. Chem., 2012, 10, 6595–6599 RSC;
(b) A. L. Braga, M. W. Paixão, D. S. Lüdtke, C. C. Silveira and O. E. D. Rodrigues, Org. Lett., 2003, 5, 2635–2638 CrossRef CAS PubMed;
(c) A. L. Braga, F. Z. Galetto, O. E. D. Rodrigues, C. C. Silveira and M. W. Paixão, Chirality, 2008, 20, 839–845 CrossRef CAS PubMed;
(d) F. Vargas, J. A. Sehnem, F. Z. Galetto and A. L. Braga, Tetrahedron, 2008, 64, 392–398 CrossRef CAS;
(e) A. L. Braga, M. W. Paixão and G. Marin, Synlett, 2005, 11, 1675–1678 CrossRef.
-
(a) P. Chocat, N. Easaki, H. Tanaka and K. Soda, Anal. Biochem., 1985, 148, 485–489 CrossRef CAS PubMed;
(b) J. Roy, W. Gordon, I. L. Schwartz and R. Walter, J. Org. Chem., 1970, 35, 510–513 CrossRef CAS PubMed;
(c) E. M. Stocking, J. N. Schwarz, H. Senn, M. Salzmann and L. A. Silks, J. Chem. Soc., Perkin Trans. 1, 1997, 1, 2443–2448 RSC;
(d) P. P. Phadnis and G. Mugesh, Org. Biomol. Chem., 2005, 3, 2476–2481 RSC;
(e) K. S. S. Raju, H. B. Singh and R. J. Butcher, Chem.–Eur. J., 2018, 24, 17513–17522 CrossRef PubMed.
-
(a) A. L. Braga, L. A. Wessjohann, P. S. Taube, F. Z. Galetto and F. M. de Andrade, Synthesis, 2010, 18, 3131–3137 CrossRef;
(b) R. H. Revanna, R. P. Kumar, A. Hosamani and P. B. Siddagangaiah, J. Organomet. Chem., 2015, 9, 61–69 CrossRef;
(c) S. Shimodaira and M. Iwaoka, Arkivoc, 2017, 2, 260–271 Search PubMed;
(d) Z. Han, T. Dong, X. Ming, F. Kuang and C. Zhang, ChemMedChem, 2021, 16, 3177–3180 CrossRef CAS PubMed.
-
(a) M. Tiecco, L. Testaferri, C. Santi, C. Tomassini, F. Marini, L. Bagnoli and A. Temperini, Angew. Chem., Int. Ed., 2003, 42, 3131–3133 CrossRef CAS;
(b) Y. Zheng, Y. He, G. Rong, X. Zhang, Y. Weng, K. Dong, X. Xu and J. Mao, Org. Lett., 2015, 17, 5444–5447 CrossRef CAS;
(c) H. Cui, X. Liu, W. Wei, D. Yang, C. He, T. Zhang and H. Wang, J. Org. Chem., 2016, 81, 2252–2260 CrossRef CAS PubMed;
(d) M. Iwasaki, K. Nonaka, S. Zou, Y. Sawanaka, T. Shinozaki, T. Fujii, K. Nakajima and Y. Nishihara, J. Org. Chem., 2019, 84, 15373–15379 CrossRef CAS PubMed;
(e) L. Sun, Y. Yuan, M. Yao, H. Wang, D. Wang, M. Gao, Y. H. Chen and A. Lei, Org. Lett., 2019, 21, 1297–1300 CrossRef CAS;
(f) B. Huang, Y. Li, C. Yang and W. Xia, Green Chem., 2020, 22, 2804–2809 RSC.
-
(a) A. L. Braga, R. S. Schwab, E. E. Alberto, S. M. Salman, J. Vargas and J. B. Azeredo, Tetrahedron Lett., 2009, 50, 2309–2311 CrossRef CAS;
(b) S. M. Salman, R. S. Schwab, E. E. Alberto, J. Vargas, L. Dornelles, O. E. D. Rodrigues and A. L. Braga, Synlett, 2011, 1, 69–72 Search PubMed;
(c) D. Tanini, C. Borgogni and A. Capperucci, New J. Chem., 2019, 43, 6388–6393 RSC;
(d) D. Tanini and A. Capperucci, New J. Chem., 2019, 43, 11451–11468 RSC.
-
(a) Y. Hata, M. Watanabe, O. Shiratori and S. Takase, Biochem. Biophys. Res. Commun., 1978, 80, 911–916 CrossRef CAS PubMed;
(b) Y. Hata and M. Watanabe, Drug Metab. Rev., 1994, 26, 575–604 CrossRef CAS PubMed.
-
(a) G. S. Poindexter, D. A. Owens, P. L. Dolan and E. Woo, J. Org. Chem., 1992, 57, 6257–6265 CrossRef CAS;
(b) A. J. Mukherjee, S. S. Zade, H. B. Singh and R. B. Sunoj, Chem. Rev., 2010, 110, 4357–4416 CrossRef CAS PubMed;
(c) P. Oroz, C. D. Navo, A. Avenoza, J. H. Busto, F. Corzana, G. Jimenez-Oses and J. M. Peregrina, Org. Lett., 2021, 23, 1955–1959 CrossRef CAS PubMed.
-
(a) H. Ishibashi, M. Uegaki and M. Sakai, Synlett, 1997, 8, 915–916 CrossRef;
(b) H. Ishibashi, M. Uegaki, M. Sakai and Y. Takeda, Tetrahedron, 2001, 57, 2115–2120 CrossRef CAS.
- J. A. Sehnem, F. Vargas, P. Milani, V. Nascimento and A. L. Braga, Synthesis, 2008, 8, 1262–1268 Search PubMed.
-
(a) M. Node, K. Kumar, K. Nishide, S. I. Ohsugi and T. Miyamoto, Tetrahedron Lett., 2001, 42, 9207–9210 CrossRef CAS;
(b) K. Nishide, T. Miyamoto, K. Kumar, S. I. Ohsugi and M. Node, Tetrahedron Lett., 2002, 43, 8569–8573 CrossRef CAS;
(c) P. K. Patra, K. Shanmugasundaram, M. Matoba, K. Nishide, T. Kajimoto and M. Node, Synthesis, 2005, 3, 447–457 Search PubMed.
|
This journal is © The Royal Society of Chemistry 2022 |
Click here to see how this site uses Cookies. View our privacy policy here.