DOI:
10.1039/D2RA05933A
(Paper)
RSC Adv., 2022,
12, 28902-28909
Association thermodynamic parameters for nano Cu(NO3)2·2.5H2O with ligands at different temperatures
Received
20th September 2022
, Accepted 3rd October 2022
First published on 12th October 2022
Abstract
Association thermodynamic parameters are important because they give information about the nature of ion–ion interaction in solution, the dielectric constant of the medium and the intermolecular hydrogen bonding between the solvent molecules. The different association thermodynamic parameters for nano copper(II) nitrate hemi pentahydrate in the presence of 4,6-diacetylresorcinol and 4,6-bis(1-hydrazonoethyl)benzene-1,3-diol as ligands were calculated. Conductance measurements were used in different concentrations of binary mixed solvents (DMF and water) at different temperatures, 293.15, 303.15, 313.15 and 323.15 K. A comparison between association thermodynamic parameter data such as association constants (KA), degree of dissociation (α), free energies of association (ΔGA), enthalpies of association (ΔHA) and entropies of association (ΔSA) in the case of using the two ligands was done. Different calculated thermodynamic parameters indicate that the association is more favorable with 4,6-diacetylresorcinol as a ligand than 4,6-bis(1-hydrazonoethyl)benzene-1,3-diol due to the large size of 6-bis(1-hydrazonoethyl)benzene-1,3-diol.
1. Introduction
The study of thermodynamic parameters is very important to know information about the intermolecular interactions and geometrical effects in the systems, thermo-physical and bulk properties of solutions. Also, study of thermodynamic parameters is necessary in theoretical and applied areas of research and very useful in many other fields of industry.1 In addition, thermodynamics parameters have been utilized to get vital information about spontaneity of a given process at a particular temperature.2,3
Studying information about transport properties such as association, conductance and ionic mobility of electrolytes in aqueous and partially aqueous media tells us all about ion–ion and ion–solvent interactions in these solutions.4–9 Fuoss–Shedlovsky equation is one of the mathematic equations of conductivity theories, which have been used successfully to investigate many electrolytes in solutions. The physical properties of the binary mixed solvents can be varied over a wide range making them a favorite solvent system for the study of ion association and ion mobility.10–13
Research on ions and ionic interactions in solution has become a leading scientific direction. Fundamental studies on ionic species in the liquid phase promote new conceptual development of ionic drugs. Application of ionic liquids in pharmacy and biochemistry is a rapidly developing multidisciplinary area.14
Copper(II) salts have many uses in organic synthesis as catalysts, mediators or oxidants. Compared with some noble metals such as rhodium, gold or palladium, copper(II) reagents have emerged as efficient and competitive promoters.15–19
Copper(II) nitrate hemi pentahydrate is one of the most common copper(II) salts. It presents as a blue crystalline solid, with features such as low toxicity, inexpensiveness, commercial availability and operational easiness. Copper(II) nitrate hemi pentahydrate has five different hydrates structures and the most common one is trihydrate form.20–22
Copper(II) nitrate hemi pentahydrate has a variety of important applications, the main one being its conversion to copper(II) oxide, which is used as catalyst in many reactions in organic chemistry. Copper(II) nitrate hemi pentahydrate solution is used in textiles and polishing agents for other metals. It is also found in some pyrotechnics. It is often used in school laboratories to demonstrate chemical voltaic cell reactions. Finally, it is a component in some ceramic glazes and metal patinas.23–26
4,6-Diacetylresorcinol and 4,6-bis(1-hydrazonoethyl)benzene-1,3-diol (Schemes 1 and 2) have been used as bis(bidentate) ligands towards transition metal ions (VO(IV),27 Co(II),28 Ni(II),29 Cu(II)28,29 and Ru(II)30), alkaline earth metal ions (Mg(II), Ca(II), Sr(II) and Ba(II)31) in addition to Ce(III) and UO2(VI) ions.27 In addition, these organic compounds have been used to synthesize different polydentate ligands including ON, ONN and ONS-donor ligands, which used to obtain solid polynuclear complexes.32–47 These complexes showed structure versatility with various modes of bonding and potential biological applications.32–34,36,38,39,46 However, literature survey showed that no previous papers studied the interactions (association) of nano copper(II) nitrate hemi pentahydrate with 4,6-diacetylresorcinol and 4,6-bis(1-hydrazonoethyl)benzene-1,3-diol as ligands using the temperatures and ratios of DMF, which used in the current study.
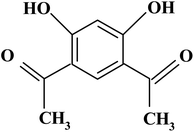 |
| Scheme 1 Molecular structure of the ligand; 4,6-diacetylresorcinol. | |
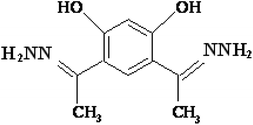 |
| Scheme 2 Molecular structure of the ligand; 4,6-bis(1-hydrazonoethyl)benzene-1,3-diol. | |
In previous work, authors have studied the complexation thermodynamic parameters of nano Cu(NO3)2·2.5H2O with 4,6-diacetylresorcinol in mixed DMF-water solvents. To provide a complete study of all thermodynamic parameters, the current study was presented on the association parameters of nano copper nitrate hemi pentahydrate with different ligands in the same mixed solvents and temperature of the pervious published paper.48
The aim of the present work is to investigate the effect of association thermodynamic parameters on the interactions of nano copper(II) nitrate hemi pentahydrate with different ligands; 4,6-diacetylresorcinol and 4,6-bis(1-hydrazonoethyl)benzene-1,3-diol in a binary mixed solvents using conductometric measurements. Also, study the effect of concentration of organic solvent in mixture and the effect of temperature on the interaction of nano salt with ligands in solutions.
2. Experimental
2.1. Materials
The salt used is copper(II) nitrate hemi pentahydrate (Cu(NO3)2·2.5H2O) which obtained from Merck company (with purity 99.5%). The ligands; 4,6-diacetylresorcinol and 4,6-bis(1-hydrazonoethyl)benzene-1,3-diol were synthesized according to the literature methods48 and,29 respectively as follows:
2.1.1 4,6-Diacetylresorcinol. In a round-bottom flask 5 g; 36.7 mmol of anhydrous zinc(II) chloride and 2.5 g resorcinol; 22.75 mmol, 4.64 g of acetic anhydride; 45.5 mmol was added drop by drop with continuous stirring. The mixture was heated to reflux at ∼140–150 °C in a paraffin oil bath for ∼1 h. After cooling, the mixture was slowly poured onto 50% dil. HCl (∼75 mL) where an orange-red precipitate was obtained, which was then filtered, washed with bidistilled water till the color of the filtrate is approximately colorless. Ethanol is used in crystallization and the obtained crystals were kept in a desiccator until used. % yield = 78% and m.p. 179 °C.
2.1.2 4,6-Bis(1-hydrazonoethyl)benzene-1,3-diol. To a hot solution of 4,6-diacetylresorcinol (4.85 g, 25 mmol) in methanol, a solution of hydrazine hydrate (2.5 mL, 50 mmol) in methanol (∼30 mL) was added dropwise. The mixture was heated to reflux on a water bath for ∼4 h where a bright-yellow crystalline precipitate was formed, which was washed with methanol and dried in a desiccator. % yield = 80% and m.p. >300 °C.
2.2. Preparation of nano copper(II) nitrate hemi pentahydrate
The bulk salt of copper(II) nitrate hemi pentahydrate (Cu(NO3)2·2.5H2O) was milled using ball mill instrument of type Retsch MM 2000 swing mill with two balls of stainless steel with diameter of 12 mm to obtain copper(II) nitrate hemi pentahydrate in nano form. Ball milling was performed at 20
225 Hz and room temperature. The temperature was kept lower than 293 K.
2.3. Preparation of solutions and conductance measurements
Four solutions of (1 × 10−4 M) nano copper(II) nitrate hemi pentahydrate were prepared in different molar ratios of DMF and water (70%, 80%, 90% and 100% DMF). Four solutions of (1 × 10−3 M) of each ligand were prepared also in the same previous molar ratios of mixed solvents DMF-water. Each solution of nano copper(II) nitrate hemi pentahydrate was placed in a titration cell at a certain temperature. Then the solution of the ligand; 4,6-diacetylresorcinol was added to the copper(II) nitrate hemi pentahydrate solution step by step and the conductivity was measured after each addition of the ligand.49–55 The pervious steps are repeated for each molar ratio of DMF-water mixed solvents at 293.15, 303.15, 313.15 and 323.15 K. The same method was used in case of another ligand; 4,6-bis(1-hydrazonoethyl)benzene-1,3-diol. The specific conductance (Ks) of solutions was measured by using a conductivity bridge of type (JENCO – 3173 COND), which has cell constant equal to one.
3. Results and discussion
3.1. Calculations of molar and limiting molar conductance
The molar and limiting molar conductance of nano copper(II) nitrate hemi pentahydrate in presence of 4,6-diacetylresorcinol and 4,6-bis(1-hydrazonoethyl)benzene-1,3-diol as ligands were calculated in different concentrations of binary mixed solvents (DMF and water) at different temperatures, 293.15, 303.15, 313.15 and 323.15 K.
The molar conductance Λm values were calculated using eqn (1):56,57
|
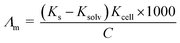 | (1) |
where
Ks and
Ksolv are the specific conductance of the solution and the solvent, respectively;
Kcell is the cell constant and
C is the molar concentration of the solution. The limiting molar conductances (
Λ0) at infinite dilutions were estimated at different temperatures by extrapolating the relation between
Λm and
C1/2 to zero.
58–60 The values of molar and limiting molar conductance of nano copper(
II) nitrate hemi pentahydrate in presence of ligands are listed in
Tables 1 and
2.
Table 1 Molar conductance (Λm), limiting molar conductance (Λ0) and degree of dissociation (α) of nano copper(II) nitrate hemi pentahydrate in presence of 4,6-diacetylresorcinol at different temperatures
T (K) |
Mole fraction of DMF |
Λm (Scm2 mol−1) |
Λ0 (Scm2 mol−1) |
α |
293.15 |
0.3528 |
246.42 |
521.71 |
0.4723 |
0.4831 |
281.17 |
619.85 |
0.4536 |
0.6774 |
313.82 |
711.85 |
0.4409 |
1.0000 |
359.11 |
839.37 |
0.4278 |
303.15 |
0.3528 |
278.01 |
610.92 |
0.4551 |
0.4831 |
313.82 |
711.85 |
0.4409 |
0.6774 |
333.82 |
768.39 |
0.4344 |
1.0000 |
398.06 |
949.75 |
0.4191 |
313.15 |
0.3528 |
334.88 |
771.53 |
0.4340 |
0.4831 |
382.27 |
905.16 |
0.4223 |
0.6774 |
422.28 |
1018.15 |
0.4148 |
1.0000 |
462.30 |
1131.11 |
0.4087 |
323.15 |
0.3528 |
419.12 |
1009.22 |
0.4153 |
0.4831 |
455.98 |
1113.27 |
0.4096 |
0.6774 |
491.79 |
1214.11 |
0.4051 |
1.0000 |
531.80 |
1327.06 |
0.4007 |
Table 2 Molar conductance (Λm), limiting molar conductance (Λ0) and degree of dissociation (α) of nano copper(II) nitrate hemi pentahydrate in presence of 4,6-bis(1-hydrazonoethyl)benzene-1,3-diol at different temperatures
T (K) |
Mole fraction of DMF |
Λm (Scm2 mol−1) |
Λ0 (Scm2 mol−1) |
α |
293.15 |
0.3528 |
273.80 |
598.99 |
0.4571 |
0.4831 |
213.77 |
429.46 |
0.4978 |
0.6774 |
148.48 |
245.12 |
0.6057 |
1.0000 |
207.46 |
411.63 |
0.5040 |
303.15 |
0.3528 |
310.66 |
702.93 |
0.4420 |
0.4831 |
251.68 |
536.52 |
0.4691 |
0.6774 |
184.29 |
346.30 |
0.5322 |
1.0000 |
244.31 |
515.70 |
0.4737 |
313.15 |
0.3528 |
379.11 |
896.23 |
0.4230 |
0.4831 |
341.20 |
789.37 |
0.4322 |
0.6774 |
264.32 |
572.21 |
0.4619 |
1.0000 |
307.50 |
694.01 |
0.4431 |
323.15 |
0.3528 |
461.25 |
1128.10 |
0.4089 |
0.4831 |
350.67 |
816.12 |
0.4297 |
0.6774 |
281.17 |
619.85 |
0.4536 |
1.0000 |
336.98 |
777.31 |
0.4335 |
The molar conductances and limiting molar conductances for nano copper(II) nitrate hemi pentahydrate in presence of 4,6-diacetylresorcinol have higher values than those in presence of 4,6-bis(1-hydrazonoethyl)benzene-1,3-diol due to the large size of 4,6-bis(1-hydrazonoethyl)benzene-1,3-diol as a ligand, which decrease the conductance.61 The degree of dissociation (α) of nano copper(II) nitrate hemi pentahydrate in presence of 4,6-diacetylresorcinol and in presence of 4,6-bis(1-hydrazonoethyl)benzene-1,3-diol decreased with the increase of the temperature and this support that molar conductances and limiting molar conductances were increased with the increase of temperature.62
3.2. Relation between Λm and C1/2 in presence of ligands
The relations between Λm and C1/2 of nano copper(II) nitrate hemi pentahydrate in presence of 4,6-diacetylresorcinol and 4,6-bis(1-hydrazonoethyl)benzene-1,3-diol at different temperatures are shown in Fig. 1–4 and 5–8, respectively. Series 1: 70% DMF – 30% water, series 2: 80% DMF – 20% water, series 3: 90% DMF – 10% water and series 4: 100% DMF – 0% water. The correlation coefficients for the lines in Fig. 1–4 and 5–8 are in the range 0.992 to 0.999, which means very strong reversible relation between Λm and C1/2.
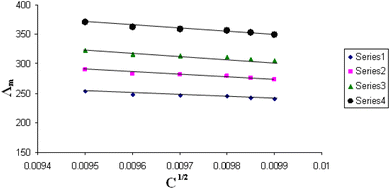 |
| Fig. 1 Relation between Λm and C1/2 for nano copper(II) nitrate hemi pentahydrate in presence of 4,6-diacetylresorcinol at 293.15 K. | |
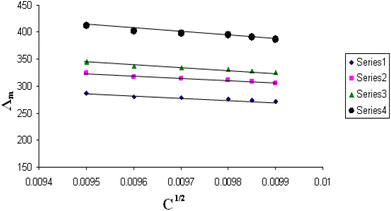 |
| Fig. 2 Relation between Λm and C1/2 for nano copper(II) nitrate hemi pentahydrate in presence of 4,6-diacetylresorcinol at 303.15 K. | |
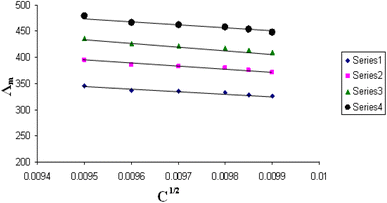 |
| Fig. 3 Relation between Λm and C1/2 for nano copper(II) nitrate hemi pentahydrate in presence of 4,6-diacetylresorcinol at 313.15 K. | |
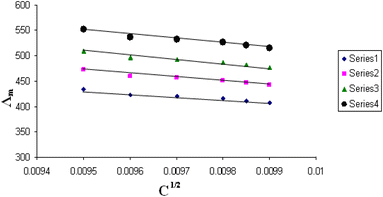 |
| Fig. 4 Relation between Λm and C1/2 for nano copper(II) nitrate hemi pentahydrate in presence of 4,6-diacetylresorcinol at 323.15 K. | |
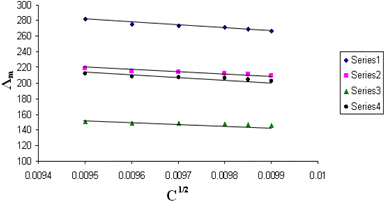 |
| Fig. 5 Relation between Λm and C1/2 for nano copper(II) nitrate hemi pentahydrate in presence of 4,6-bis(1-hydrazonoethyl)benzene-1,3-diol at 293.15 K. | |
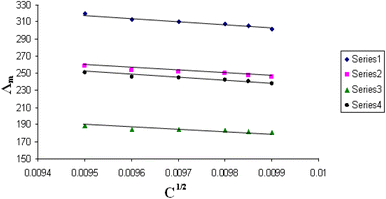 |
| Fig. 6 Relation between Λm and C1/2 for nano copper(II) nitrate hemi pentahydrate in presence of 4,6-bis(1-hydrazonoethyl)benzene-1,3-diol at 303.15 K. | |
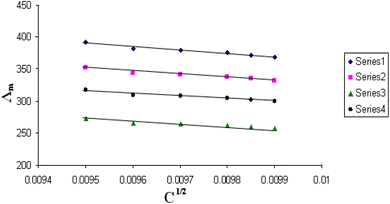 |
| Fig. 7 Relation between Λm and C1/2 for nano copper(II) nitrate hemi pentahydrate in presence of 4,6-bis(1-hydrazonoethyl)benzene-1,3-diol at 313.15 K. | |
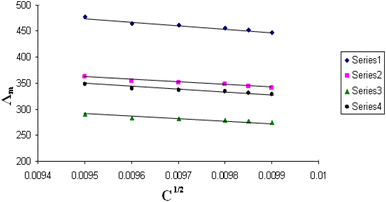 |
| Fig. 8 Relation between Λm and C1/2 for nano copper(II) nitrate hemi pentahydrate in presence of 4,6-bis(1-hydrazonoethyl)benzene-1,3-diol at 323.15 K. | |
All figures show straight lines in relation between molar conductance and square root of concentration due to strong electrolytes.
3.3. Association thermodynamic parameters
The association thermodynamic parameters; association constants (KA), free energies of association (ΔGA), enthalpies of association (ΔHA) and entropies of association (ΔSA) of nano copper(II) nitrate hemi pentahydrate in presence of 4,6-diacetylresorcinol and 4,6-bis(1-hydrazonoethyl)benzene-1,3-diol are listed Tables 3 and 4, respectively. The data are obtained in different concentrations of binary mixed solvents (DMF-water) at different temperatures; 293.15, 303.15, 313.15 and 323.15 K.
Table 3 Association constants (KA), free energies of association (ΔGA), enthalpies of association (ΔHA) and entropies of association (ΔSA) of nano copper(II) nitrate hemi pentahydrate in presence of 4,6-diacetylresorcinol at different temperatures
T (K) |
Xs of DMF |
KA |
ΔG (kJ mol−1) |
ΔH (kJ mol−1) |
TΔS |
ΔS (kJ mol−1 K) |
293.15 |
0.3528 |
2.5481 × 104 |
−24.7276 |
11.0288 |
35.7564 |
0.1220 |
0.4831 |
2.8608 × 104 |
−25.0096 |
9.8416 |
34.8512 |
0.1189 |
0.6774 |
3.0995 × 104 |
−25.2051 |
10.5501 |
35.7552 |
0.1220 |
1.0000 |
3.3678 × 104 |
−25.4073 |
5.6676 |
31.0749 |
0.1060 |
303.15 |
0.3528 |
2.8349 × 104 |
−25.8398 |
11.0288 |
36.8686 |
0.1216 |
0.4831 |
3.0995 × 104 |
−26.0649 |
9.8416 |
35.9065 |
0.1184 |
0.6774 |
3.2282 × 104 |
−26.1674 |
10.5501 |
36.7175 |
0.1211 |
1.0000 |
3.5625 × 104 |
−26.4157 |
5.6676 |
32.0833 |
0.1058 |
313.15 |
0.3528 |
3.2363 × 104 |
−27.0371 |
11.0288 |
38.0659 |
0.1216 |
0.4831 |
3.4893 × 104 |
−27.2329 |
9.8416 |
37.0745 |
0.1184 |
0.6774 |
3.6653 × 104 |
−27.3610 |
10.5501 |
37.9111 |
0.1211 |
1.0000 |
3.8133 × 104 |
−27.4641 |
5.6676 |
33.1317 |
0.1058 |
323.15 |
0.3528 |
3.6524 × 104 |
−28.2253 |
11.0288 |
39.2541 |
0.1215 |
0.4831 |
3.7915 × 104 |
−28.3258 |
9.8416 |
38.1674 |
0.1181 |
0.6774 |
3.9063 × 104 |
−28.4059 |
10.5501 |
38.9560 |
0.1206 |
1.0000 |
4.0202 × 104 |
−28.4833 |
5.6676 |
34.1509 |
0.1057 |
Table 4 Association constants (KA), free energies of association (ΔGA), enthalpies of association (ΔHA) and entropies of association (ΔSA) of nano copper(II) nitrate hemi pentahydrate in presence of 4,6-bis(1-hydrazonoethyl)benzene-1,3-diol at different temperatures
T (K) |
Xs of DMF |
KA |
ΔG (kJ mol−1) |
ΔH (kJ mol−1) |
TΔS |
ΔS (kJ mol−1 K) |
293.15 |
0.3528 |
2.7992 × 104 |
−24.9566 |
10.0625 |
35.0191 |
0.1195 |
0.4831 |
2.1837 × 104 |
−24.3515 |
11.5296 |
35.8811 |
0.1224 |
0.6774 |
1.1576 × 104 |
−22.8045 |
23.6852 |
46.4897 |
0.1586 |
1.0000 |
2.1036 × 104 |
−24.2604 |
21.5841 |
45.8445 |
0.1564 |
303.15 |
0.3528 |
3.0780 × 104 |
−26.0473 |
10.0625 |
36.1098 |
0.1191 |
0.4831 |
2.5992 × 104 |
−25.6211 |
11.5296 |
37.1507 |
0.1225 |
0.6774 |
1.7796 × 104 |
−24.6664 |
23.6852 |
48.3516 |
0.1595 |
1.0000 |
2.5261 × 104 |
−25.5492 |
21.5841 |
47.1333 |
0.1555 |
313.15 |
0.3528 |
3.4739 × 104 |
−27.2215 |
10.0625 |
37.2840 |
0.1191 |
0.4831 |
3.2738 × 104 |
−27.0670 |
11.5296 |
38.5966 |
0.1233 |
0.6774 |
2.7166 × 104 |
−26.5814 |
23.6852 |
50.2666 |
0.1605 |
1.0000 |
3.0562 × 104 |
−26.8880 |
21.5841 |
48.4721 |
0.1548 |
323.15 |
0.3528 |
3.8093 × 104 |
−28.3384 |
10.0625 |
38.4009 |
0.1188 |
0.4831 |
3.3279 × 104 |
−27.9755 |
11.5296 |
39.5051 |
0.1223 |
0.6774 |
2.8608 × 104 |
−27.5691 |
23.6852 |
51.2543 |
0.1586 |
1.0000 |
3.2472 × 104 |
−27.9095 |
21.5841 |
49.4936 |
0.1532 |
The association constants were calculated by using eqn (2):63–66
|
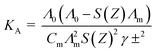 | (2) |
where (
Λm,
Λ0) are the molar and limiting molar conductance of nano copper(
II) nitrate hemi pentahydrate in presence of ligands, respectively,
Cm is the molar concentration of copper(
II) nitrate hemi pentahydrate,
S(
Z) is Fouss–Shedlovsky factor, equal with unity for strong electrolytes and
γ± is the mean activity coefficient.
Gibbs free energies of association ΔGA of nano copper(II) nitrate hemi pentahydrate in presence of ligands were calculated by using eqn (3).67–72
From the linear plots of log
Ksp vs. 1/T, the enthalpies were calculated from the slopes (slopes = −ΔH/2.303R)73 and their values are given in Tables 3 and 4. The entropies of solvation were calculated by use of Gibbs–Helmholtz eqn (4):74–80
Association constants of nano copper(II) nitrate hemi pentahydrate in presence of 4,6-diacetylresorcinol have higher values than those in presence of 4,6-bis(1-hydrazonoethyl)benzene-1,3-diol. Negative free energies mean that all interaction processes are spontaneous at all temperatures and mole fractions of DMF used for two ligands.
3.4. Relations between log
KA and 1/T
The relations between log
KA and 1/T for different concentrations of DMF and water (mixed solvents) by volumes for nano copper(II) nitrate hemi pentahydrate in presence of 4,6-diacetylresorcinol and 4,6-bis(1-hydrazonoethyl)benzene-1,3-diol are shown in Fig. 9 and 10, respectively. Straight lines relations at all of the used DMF percentages are obtained. The correlation coefficients for the lines in Fig. 9 and 10 are in the range 0.989 to 0.997, which means very strong reversible relation between log
KA and 1/T.
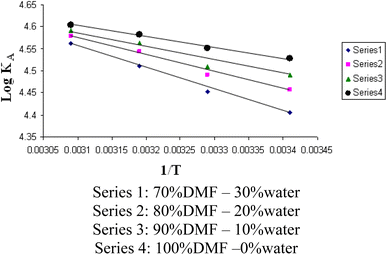 |
| Fig. 9 Relation between log KA and 1/T for nano copper(II) nitrate hemi pentahydrate in presence of 4,6-diacetylresorcinol. | |
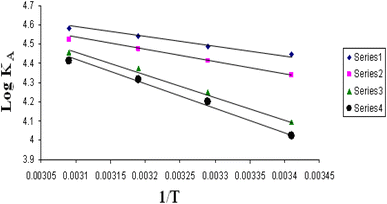 |
| Fig. 10 Relation between log KA and 1/T for nano copper(II) nitrate hemi pentahydrate in presence of 4,6-bis(1-hydrazonoethyl)benzene-1,3-diol. | |
The relation between log
KA and 1/T is inversely proportional which means endothermic processes so, enthalpy is positive.
4. Conclusion
The most values of molar conductance and limiting molar conductance for nano copper(II) nitrate hemi pentahydrate in presence of 4,6-diacetylresorcinol have higher values than those in presence of 4,6-bis(1-hydrazonoethyl)benzene-1,3-diol due to the large size of 4,6-bis(1-hydrazonoethyl)benzene-1,3-diol as a ligand, which decrease the conductance. The molar and limiting molar conductance in presence of each ligand increase with increasing temperature. The effect of organic solvent in the mixture on conductivity differs from ligand to another where the molar and limiting molar conductance in presence of 4,6-diacetylresorcinol increases with increasing the concentration of DMF while in presence of 4,6-bis(1-hydrazonoethyl)benzene-1,3-diol, the molar and limiting molar conductance decrease with increasing the concentration of DMF. The association constants in case of 4,6-diacetylresorcinol have higher values than those in case of 4,6-bis(1-hydrazonoethyl)benzene-1,3-diol at all temperatures, which indicates that the interactions of nano copper(II) nitrate hemi pentahydrate is more favourable with 4,6-diacetylresorcinol. The free energies of association of nano copper(II) nitrate hemi pentahydrate with 4,6-diacetylresorcinol and 4,6-bis(1-hydrazonoethyl)benzene-1,3-diol are of negative values, which means that the association process is spontaneously at all temperature and ratios of DMF used. The free energies in case of 4,6-diacetylresorcinol are more negative and this is the second parameter which indicates that the association is more favorable with 4,6-diacetylresorcinol as a ligand. The interaction process of nano copper(II) nitrate hemi pentahydrate with the two ligands is endothermic due to positive enthalpies but small positive values of enthalpies in case of 4,6-diacetylresorcinol is the third parameters which indicates that the interaction with 4,6-diacetylresorcinol is more favourable. Positive values of entropies in case of two ligands mean that the interactions are spontaneously. These data provide an opportunity for researchers to study the interactions in binary solvents solutions at different temperatures. In future work, authors will study different thermodynamic parameters for other heavy metals.
Conflicts of interest
There are no conflicts to declare.
References
- S.-D. Zhang, P.-H. Ma, Y.-C. Zhai and W.-M. Chen, Rare Metals, 2015, 34(12), 873–876 CrossRef CAS.
- M. N. Abd El-Hady, R. R. Zaky, K. M. Ibrahim and E. A. Gomaa, J. Mol. Struct., 2012, 1016, 169–180 CrossRef CAS.
- E. M. AbouElleef and S. D. Mekky, J. Biochem. Technol., 2019, 10(1), 57–66 CAS.
- P. Haldar and B. Das, J. Mol. Liq., 2007, 130, 29–33 CrossRef CAS.
- N. G. Tsierkezos and I. E. Molinou, J. Solution Chem., 2007, 36, 153–170 CrossRef CAS.
- F. I. El-Dossoki, J. Mol. Liq., 2010, 151(1), 1–8 CrossRef CAS.
- U. N. Dash, J. R. Mahapatra and B. Lal, J. Mol. Liq., 2006, 124(1–3), 13–18 CrossRef CAS.
- M. N. Roy, B. B. Gurung and V. K. Dakua, Int. J. Thermophys., 2006, 27, 1539–1550 CrossRef CAS.
- N. G. Tsierkezos and I. E. Molinou, J. Chem. Thermodyn., 2006, 38, 1422–1431 CrossRef CAS.
- M. Bešter-Rogač, N. Hauptman and J. Barthel, J. Mol. Liq., 2007, 131–132, 29–35 CrossRef.
- C. Klofutar and N. Šegatin, J. Solution Chem., 2007, 36, 879–889 CrossRef CAS.
- F. I. El-Dossoki, J. Mol. Liq., 2011, 158(1), 18–22 CrossRef CAS.
- F. I. El-Dossoki, J. Mol. Liq., 2011, 160(2), 119–123 CrossRef CAS.
- K. S. Egorova and V. P. Ananikov, J. Mol. Liq., 2018, 272, 271–300 CrossRef CAS.
- S. D. McCann and S. S. Stahl, Acc. Chem. Res., 2015, 48, 1756–1766 CrossRef CAS PubMed.
- X.-X. Guo, D.-W. Gu, Z. Wu and W. Zhang, Chem. Rev., 2015, 115(3), 1622–1651 CrossRef CAS PubMed.
- S. Thapa, B. Shrestha, S. K. Gurung and R. Giri, Org. Biomol. Chem., 2015, 13(17), 4816–4827 RSC.
- J. Liu, G. Chen and Z. Tan, Adv. Synth. Catal., 2016, 358, 1174–1194 CrossRef CAS.
- A. P. Jadhav, D. Ray, V. U. B. Rao and R. P. Singh, Eur. J. Org. Chem., 2016,(14), 2369–2382 CrossRef CAS.
- X. Zhu and S. Chiba, Chem. Soc. Rev., 2016, 45, 4504–4523 RSC.
- S. Buffagni, L. M. Vallarino and J. V. Quagliano, Inorg. Chem., 1964, 3, 671–678 CrossRef CAS.
- R. L. Carlin and M. J. Baker, J. Chem. Soc., 1964, 5008–5014 RSC.
- H. W. Richardson, Ullmann’s Encyclopedia of Industrial Chemistry, Electronic release, Wiley-VCH, Weinheim, 2000 Search PubMed.
- S. C. Wallwork and W. E. Addison, J. Chem. Soc., 1965, 2925–2933 RSC.
- S. I. Troyanov, I. V. Morozov, K. O. Znamenkov and M. Korenev, Z. Anorg. Allg. Chem., 1995, 621(7), 1261–1265 CrossRef CAS.
- R. E. LaVilla and S. H. Bauer, J. Am. Chem. Soc., 1963, 85(22), 3597–3600 CrossRef CAS.
- H. F. El-Shafiy and M. Shebl, J. Mol. Struct., 2019, 1194, 187–203 CrossRef CAS.
- M. Shebl, S. M. E. Khalil, A. Taha and M. A. N. Mahdi, Spectrochim. Acta A, 2013, 113, 356–366 CrossRef CAS PubMed.
- B. S. Shyamala and V. Jayatyagaraju, Synth. React. Inorg. Met. Org. Chem., 2003, 33(1), 63–75 CrossRef CAS.
- P. Krishnamoorthy, P. Sathyadevi, K. Deepa and N. Dharmaraj, Spectrochim. Acta, Part A, 2010, 77(1), 258–263 CrossRef CAS PubMed.
- M. Shebl, S. M. E. Khalil, A. Taha and M. A. N. Mahdi, J. Mol. Struct., 2012, 1027, 140–149 CrossRef CAS.
- M. Shebl, J. Coord. Chem., 2016, 69(2), 199–214 CrossRef CAS.
- K. M. Raj, B. Vivekanand, G. Y. Nagesh and B. H. M. Mruthyunjayaswamy, J. Mol. Struct., 2014, 1059(1), 280–293 Search PubMed.
- F. C. Lima, Y. A. O. Só, R. Gargano, D. M. de Oliveira and C. C. Gatto, J. Inorg. Biochem., 2021, 224, 111559 CrossRef CAS PubMed.
- M. Shebl, Spectrochim. Acta, Part A, 2008, 70(4), 850–859 CrossRef PubMed.
- N. V. Kulkarni, M. P. Sathisha, S. Budagumpi, G. S. Kurdekar and V. K. Revankar, J. Coord. Chem., 2010, 63(8), 1451–1461 CrossRef CAS.
- S. Abdel Halim and M. Shebl, J. Coord. Chem., 2021, 74(17–20), 2984–3001 CrossRef CAS.
- M. Shebl, J. Coord. Chem., 2009, 62(19), 3217–3231 CrossRef CAS.
- W. H. Mahmoud, M. M. Omar, Y. M. Ahmed and G. G. Mohamed, Appl. Organomet. Chem., 2020, 34(4), e5528 CrossRef CAS.
- Y. M. Ahmed, W. H. Mahmoud, M. M. Omar and G. G. Mohamed, J. Inorg. Organomet. Polym. Mater., 2021, 31(6), 2339–2359 CrossRef CAS.
- A. A. A. Emara, B. A. El-Sayed and E.-S. A. E. Ahmed, Spectrochim. Acta, Part A, 2008, 69, 757–769 CrossRef PubMed.
- M. Shebl, Spectrochim. Acta, Part A, 2009, 73(2), 313–323 CrossRef PubMed.
- J. H. Pandya, R. N. Jadeja and K. J. Ganatra, J. Saudi Chem. Soc., 2014, 18(3), 190–199 CrossRef CAS.
- F. Samy and M. Shebl, Appl. Organomet. Chem., 2022, 36(5), e6650 CrossRef CAS.
- F. C. Lima, Y. A. O. Só, R. Gargano, M. Fujimori, E. L. França, A. C. Honorio-França and C. C. Gatto, J. Mol. Struct., 2020, 1212, 128083 CrossRef CAS.
- S. Rodríguez-Hermida, A. B. Lago, R. Carballo, O. Fabelo and E. M. Vázquez-López, Chem. – Eur. J., 2015, 21, 6605–6616 CrossRef PubMed.
- A. A. A. Emara and O. M. I. Adly, Transition Met. Chem., 2007, 32(7), 889–901 CrossRef CAS.
- S. G. Sanad and M. Shebl, J. Mol. Liq., 2019, 294, 111602 CrossRef CAS.
- C. M. Park and R. J. Shechan, Ullmann’s Encyclopedia of Industrial Chemistry, 1991, vol. 18, pp. 991–1043 Search PubMed.
- Kirk-Othmer Encyclopedia of Chemical Technology, ed. A. E. Williams, H. F. Mark, D. F. Othmer, C. G. Overberger and G. T. Seaborg, Wiley, New York, 3rd edn, 1978, vol. 3, pp. 778–792 Search PubMed.
- K. Yamamoto and N. Nishi, J. Am. Chem. Soc., 1990, 112(2), 549–558 CrossRef CAS.
- E. E. Schrier, M. Pottle and H. A. Scheraga, J. Am. Chem. Soc., 1964, 86(17), 3444–3449 CrossRef CAS.
- J. L. Opgrande, C. J. Dobratz, E. E. Brown, J. C. Liang, G. S. Conn, J. Wirth, J. Shelton, in Kirk-Othmer Encyclopedia of Chemical Technology, ed. J. I. Kroschwitz and M. Howe-Grant, Wiley, New York, 4th edn, 1992, vol. 4, pp. 103–115 Search PubMed.
- K. Suzuki, Y. Taniguchi and T. Watanabe, J. Phys. Chem., 1973, 77(15), 1918–1922 CrossRef CAS.
- D. E. Read and C. B. Purves, J. Am. Chem. Soc., 1952, 74(1), 116–119 CrossRef CAS.
- B. Heinrich, Ultrathin Magnetic Structure, Springer-Verlag, Berlin, 1994, vol. II, p. 216 Search PubMed.
- M. N. H. Hamed, E. A. Gomaa and S. G. Sanad, AASCIT J. Energy, 2015, 2(1), 1–8 Search PubMed.
- J. I. Kim and E. A. Gomaa, Bull. Soc. Chim. Belg., 1981, 90(4), 391–407 CrossRef CAS.
- F. C. Schmidt and W. E. Hoffmann, Proc. Schaap., Indiana, Acta, Sci., 1962, 72, 127–131 CAS.
- E. J. King, J. Phys. Chem., 1969, 73(5), 1220–1232 CrossRef CAS.
- I. Ali, W. A. Wani and K. Saleem, Synth. React. Inorg., Met.-Org., Nano-Met. Chem., 2013, 43(9), 1162–1170 CrossRef CAS.
- E. A. Gomaa and R. T. Rashad, Chem. Res. J., 2018, 3(2), 102–113 CAS.
- R. Gopal and M. A. Siddiqui, J. Phys. Chem., 1969, 73(10), 3390–3394 CrossRef CAS.
- P. Debye and H. Hückel, Phys. Z., 1923, 24, 305–325 CAS.
- A. M. Hafez, H. Sadek and N. H. El-Hammamy, Croat. Chem. Acta, 1977, 49(4), 713–716 CAS.
- M. N. H. Hamed, E. A. Gomaa and S. G. Sanad, AASCIT J. Eng. Technol., 2015, 2(2), 74–80 Search PubMed.
- Y. Li, V. P. Kotzeva and D. J. Fray, Mater. Lett., 2006, 60(21–22), 2743–2746 CrossRef CAS.
- E. A. Gomaa, Am. J. Syst.Sci., 2014, 3(1), 12–17 Search PubMed.
- L. Chen, L. Shen, A. Xie, J. Zhu, Z. Wu and L. Yang, Cryst. Res. Technol., 2007, 42(9), 886–889 CrossRef CAS.
- E. M. Abou Elleef and E. A. Gomaa, Int. J. Eng. Innovative Technol., 2013, 3(6), 308–313 Search PubMed.
- Y. Marcus, Pure Appl. Chem., 1990, 62(11), 2069–2076 CrossRef CAS.
- M. Sh. Ramadan, A. M. Hafez, H. Sadek and A. EI-Zyadi, J. Solution Chem., 1996, 25(8), 797–812 CrossRef CAS.
- N. M. Cleophase, Removal of aluminium and sulphate ions from alkaline medium using solvent extraction, Master of Technology, Faculty of Engineering, Cape Peninsula University of Technology, 2009 Search PubMed.
- P. Carreras, A. Antony, F. Rojas and J. Bertomeu, Thin Solid Film, 2011, 520(4), 1223–1227 CrossRef CAS.
- T. Shedlovsky, J. Franklin Inst., 1938, 225(6), 739–743 CrossRef CAS.
- R. E. Dickenson and I. Geis, Chemistry, Matter and the Universe An Integrated Approach to General Chemistry, Paperback, Inc., Benjamin-Cummings Publishing C, USA, 1st edn, 1976 Search PubMed.
- E. A. Gomaa, H. M. Abu El-Nader and Sh. E. Rashed, Int. J. Eng. Sci., 2014, 3, 64–73 Search PubMed.
- N. H. El-Hammamy, H. A. Hawaty, A. I. Kawanab, M. N. El-Hammamy and H. M. Moharem, J. Chem. Pharm. Res., 2011, 3(3), 729–733 CAS.
- D. J. G. Ives, Chemical Thermodynamics, University Chemistry, Macdonald Technical and Scientific Ltd., London, 1971 Search PubMed.
- K. M. Ibrahim, E. A. Gomaa, R. R. Zaky and M. N. Abdel El-Hady, Am. J. Chem., 2012, 2, 23–26 CrossRef.
|
This journal is © The Royal Society of Chemistry 2022 |