DOI:
10.1039/D2RA05758A
(Paper)
RSC Adv., 2022,
12, 28916-28928
Naphthylisoindolinone alkaloids: the first ring-contracted naphthylisoquinolines, from the tropical liana Ancistrocladus abbreviatus, with cytotoxic activity†‡§
Received
12th September 2022
, Accepted 3rd October 2022
First published on 12th October 2022
Abstract
The West African liana Ancistrocladus abbreviatus is a rich source of structurally most diverse naphthylisoquinoline alkaloids. From its roots, a series of four novel representatives, named ancistrobrevolines A–D (14–17) have now been isolated, displaying an unprecedented heterocyclic ring system, where the usual isoquinoline entity is replaced by a ring-contracted isoindolinone part. Their constitutions were elucidated by 1D and 2D NMR and HR-ESI-MS. The absolute configurations at the chiral axis and at the stereogenic center were assigned by using experimental and computational electronic circular dichroism (ECD) investigations and a ruthenium-mediated oxidative degradation, respectively. For the biosynthetic origin of the isoindolinones from ‘normal’ naphthyltetrahydroisoquinolines, a hypothetic pathway is presented. It involves oxidative decarboxylation steps leading to a ring contraction by a benzilic acid rearrangement. Ancistrobrevolines A (14) and B (15) were found to display moderate cytotoxic effects (up to 72%) against MCF-7 breast and A549 lung cancer cells and to reduce the formation of spheroids (mammospheres) in the breast cancer cell line.
Introduction
Ancistrocladus abbreviatus Airy Shaw (Ancistrocladaceae) is a woody liana native to the coastal rainforests of West Africa.1–4 It is characterized by a plethora of structurally most diverse secondary metabolites belonging to the emerging class of naphthylisoquinoline alkaloids, with 280 as yet known representatives.5–9 From this tropical plant, no less than 69 such compounds have so far been isolated, many of them with unique molecular scaffolds.5,10–18 With 33 examples,5,10,11,13,14,17 naphthyltetrahydroisoquinoline alkaloids such as ancistro-brevine A (1), its 6-O-demethyl analogue 2,5,14 and ancistro-brevine D (3)5,13 (Fig. 1) constitute the major portion of compounds produced by the plant. Their molecular moieties are coupled via their 5,1′-, 5,8′-, 7,1′-, or 7,8′-positions. In all these metabolites, the biaryl axis is rotationally hindered, due to the presence of mostly bulky ortho-substituents, leading to the phenomenon of atropisomerism.5–7,19,20 Many of the alkaloids of A. abbreviatus are typical Ancistrocladaceae-type compounds (i.e., with S-configuration at C-3 and an oxygen function at C-6), such as compounds 1–3. But also metabolites with structural characteristics typical of alkaloids occurring in plants of the related Dioncophyllaceae family21–23 were isolated, viz., 3R-configured and lacking an oxygen function at C-6, such as dioncophylline A (4)5,24,25 and its N-methyl analogue 5.5,10 Even mixed, hybrid-type compounds like ancistrobrevine M (7; 3R, 6-OH)17 and dioncoline A (6; 3S, 6-H),17 possessing the opposite characteristics, were discovered in the plant. Ancistrocladus abbreviatus is the only Ancistrocladus species known to contain naphthylisoquinoline alkaloids of all these four subclasses. Likewise reported was the occurrence of nine naphthyldihydroisoquinolines,5,12,14,15 six of which were 5,1′-coupled like ancistrobrevidine C (8),15 which was highly effective against the aggressive and metastatic growth of pancreatic cancer cells. The outstanding metabolite pattern of A. abbreviatus also comprises a unique series of seven alkaloids with a non-hydrogenated isoquinoline part, from all four coupling types mentioned above, with the biaryl axis as the only stereogenic element, among them ancistrobreveine D (9).16 Even though they are devoid of stereogenic centers, they are all optically active, occurring as enantiomerically pure compounds or as scalemic mixtures.16 The broad spectrum of alkaloids produced by A. abbreviatus is further enlarged by the occurrence of dimeric congeners like the C2-symmetric representative jozimine A2 (11; both halves 7,1′-coupled). In the roots of A. abbreviatus, three further symmetric and unsymmetric dimers were detected, with the parent compound jozimine A2 (11) being the most active agent against HT-29 colon cancer, HT-1080 fibrosarcoma, and MM.1S multiple myeloma cell lines.17 Likewise isolated were quinoid structures like ancistrobreviquinone A (10),15 possessing an ortho-naphthoquinone entity. Even more remarkable is the structure of the first 1-demethylated naphthylisoquinoline alkaloid, 1-nor-8-O-demethylancistro-brevine H (12),18 lacking the otherwise generally present methyl group at C-1. This compound was isolated along with a series of unprecedented seco-alkaloids from the roots of A. abbreviatus,18 having undergone a cleavage of the heterocyclic ring, with an elimination of the entire C-1/Me-1 unit, among them ancistrosecoline D (13) (Fig. 1). This alkaloid exerted potent, and selective, cytotoxicity against HeLa cervical cancer cells by inducing apoptosis.18
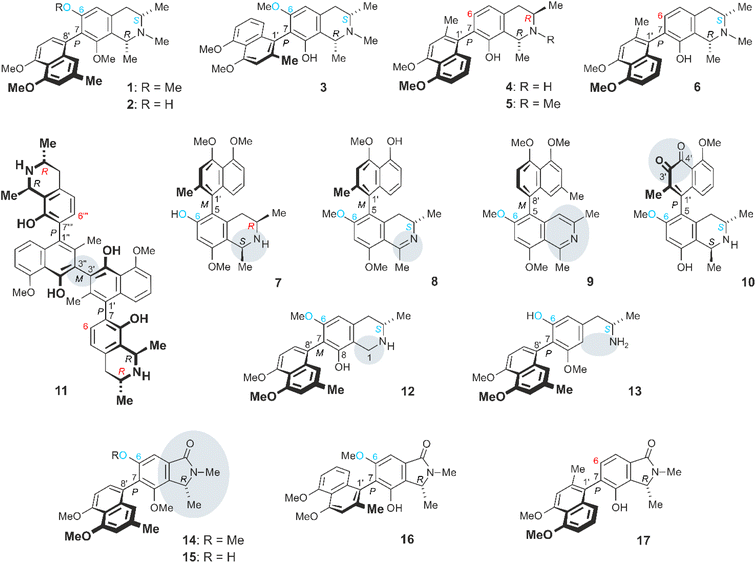 |
| Fig. 1 Secondary metabolites produced by A. abbreviatus: the naphthyltetrahydroisoquinolines ancistrobrevine A (1) and its 6-O-methyl analogue 2, ancistrobrevine D (3), dioncophylline A (4) and its N-methyl congener 5, dioncoline A (6), and ancistrobrevine M (7), the dihydroisoquinoline ancistrobrevidine C (8), the non-hydrogenated ancistrobreveine D (9), the naphthoquinone derivative ancistrobreviquinone A (10), the dimer jozimine A2 (11), the 1-unsubstituted 1-nor-8-O-demethylancistrobrevine H (12), the ring-cleaved ancistrosecoline D (13), and the novel ancistrobrevolines A–D (14–17). – Ancistrocladaceae-type alkaloids (with 6-OR and 3S) are labeled in “blue–blue”, Dioncophyllaceae-type representatives (6-H and 3R) are marked in “red–red”, the hybrid-type (6-OR and 3R) ancistrobrevine M (7) in “blue-red”, and the only known17 inverse-hybrid-type (6 H, 3S) dioncoline (6) in “red-blue”. | |
The ‘chemical creativity’ of A. abbreviatus is even larger, as shown in this report by the discovery of a series of metabolites constituting a novel subtype of axially chiral naphthylisoindolinone alkaloids, named ancistrobrevolines A–D (14–17). In these compounds, the six-membered heterocyclic part of the isoquinoline portion has undergone a ring contraction to give a five-membered heterocycle as part of an isoindolinone system, with loss of the whole C-3/Me-3 entity, which had so far been present in all other naphthylisoquinoline alkaloids. For the origin of the novel naphthylisoindolinones 14–17 in the plants, a biosynthetic concept is presented, involving the stepwise loss of two carbon atoms and a benzilic acid rearrangement, leading to the observed ring contraction. Ancistrobrevolines A (14) and B (15) showed moderate to good cytotoxic activities against lung and breast cancer cell lines.
Results and discussion
Isolation and structural elucidation of ancistrobrevolines A–D
Air-dried root bark material of A. abbreviatus collected in the Parc National de Taï in the Southwestern Côte d’Ivoire (Ivory Coast) was repeatedly extracted with MeOH. After filtration of the crude extract and evaporation of the solvent, the residual material was macerated with MeOH–H2O (9
:
1, v/v), followed by liquid–liquid partitioning with n-hexane and fractionation by column chromatography on silica gel. HPLC-UV guided analysis of an alkaloid-rich fraction showed the presence of metabolites exhibiting UV spectra closely similar to those of naphthylisoquinoline alkaloids. They showed a first UV maximum at 224–229 nm, yet with an additional second UV maximum at 259–261 nm, resembling that of alkaloids with a fully dehydrogenated ring like ancistrobreveine D (9).
Ancistrobrevoline A (14). The first isolated compound had a molecular formula of C25H27NO5, as indicated from its sodium adduct in HR-ESI-MS, C25H27NNaO5+, corresponding to m/z 444.17711 [M + Na]+. The 1H and 13C NMR spectra (Table 1) showed predominantly signals typical of conventional naphthylisoquinoline alkaloids (Fig. 2A), in particular for the naphthalene part, with its usual 4′,5′-dimethoxy-2′-methyl substitution pattern, coupled via C-8′. The NMR data revealed a spin system with four aromatic methines, H-1′ (δH 6.70), H-3′ (δH 6.76), H-6′ (δH 6.92), and H-7′ (δH 7.16), a three-proton singlet (δH,C 2.29, 22.0) evidencing the presence of an aryl-methyl group (Me-2′), and further singlets for two methoxy functions, MeO-4′ (δH,C 3.92, 56.9) and MeO-5′ (δH,C 3.95, 56.7). The two aromatic singlets (δH 6.70 and 6.76) and an AB spin system of two adjacent protons (δH 6.92 and 7.16) indicated that the coupling site of the biaryl axis was located in the methyl-free part of the naphthalene moiety, i.e., either at C-6′ or C-8′, which was in agreement with the normal, not highfield-shifted signal of Me-2′ (δH 2.29). The NOESY correlation sequence {MeO-5′ ↔ H-6′ ↔ H-7′} excluded the biaryl axis from being located at C-6′, thus establishing C-8′ to be the axis-bearing carbon atom. This assignment was confirmed by HMBC long-range couplings from H-1′ (δH 6.70) and H-6′ (δH 6.92) to C-8′ (δC 124.6), from H-7′ (δH 7.16) to C-9′ (δC 137.5), and from H-3′ (δH 6.76) to C-1′ (δC 118.8) (Fig. 2B).
Table 1 1H (600 MHz) and 13C (150 MHz) NMR data of ancistrobrevolines A (14), B (15), C (16), and D (17) in methanol-d4 (δ in ppm)
Position |
14 |
15 |
16 |
17 |
δH (J in Hz) |
δC |
δH (J in Hz) |
δC |
δH (J in Hz) |
δC |
δH (J in Hz) |
δC |
1 |
4.69, q |
58.4 |
4.65, q |
58.3 |
4.58, q |
58.3 |
4.67, q |
58.5 |
4 |
|
169.8 |
|
169.9 |
|
170.4 |
|
170.3 |
5 |
7.21, s |
101.2 |
7.05, s |
105.4 |
7.01, s |
97.4 |
7.37, d (7.6) |
115.3 |
6 |
|
161.1 |
|
158.4 |
|
160.5 |
7.10, d (7.5) |
133.6 |
7 |
|
128.1 |
|
126.7 |
|
120.5 |
|
131.9 |
8 |
|
155.6 |
|
155.8 |
|
151.9 |
|
151.5 |
9 |
|
132.6 |
|
131.0 |
|
128.2 |
|
135.3 |
10 |
|
134.0 |
|
133.9 |
|
133.8 |
|
133.6 |
1′ |
6.70, s |
118.8 |
6.80, s |
119.1 |
|
122.3 |
|
126.1 |
2′ |
|
137.3 |
|
137.9 |
|
137.9 |
|
137.2 |
3′ |
6.76, s |
109.6 |
6.77, s |
109.7 |
6.91, s |
110.4 |
6.92, s |
110.2 |
4′ |
|
158.4 |
|
158.4 |
|
158.2 |
|
158.2 |
5′ |
|
158.5 |
|
158.5 |
|
158.6 |
|
158.6 |
6′ |
6.92, d (8.0) |
106.3 |
6.94, d (7.9) |
106.4 |
6.85, d (6.9) |
107.0 |
6.87, d (7.2) |
107.0 |
7′ |
7.16, d (7.9) |
130.3 |
7.22, d (7.9) |
130.6 |
7.18, dd (7.8, 8.4) |
127.6 |
7.19, dd (7.8, 8.4) |
127.6 |
8′ |
|
124.6 |
|
124.6 |
6.81, dd (0.9, 8.4) |
119.1 |
6.80, dd (0.9, 8.5) |
119.5 |
9′ |
|
137.5 |
|
137.4 |
|
138.1 |
|
138.1 |
10′ |
|
117.1 |
|
117.3 |
|
117.8 |
|
117.8 |
1-Me |
1.54, d (6.6) |
17.2 |
3.20, d (6.6) |
17.2 |
1.55, d (6.6) |
16.8 |
1.58, d (6.6) |
16.4 |
2′-Me |
2.29, s |
22.0 |
2.30, s |
22.0 |
2.09, s |
20.5 |
2.16, s |
20.7 |
N–Me |
3.15, s |
27.1 |
3.13, s |
27.1 |
3.14, s |
27.3 |
3.15, s |
27.2 |
6-OMe |
3.67, s |
56.5 |
|
|
3.64, s |
56.3 |
3.64, s |
|
8-OMe |
3.24, s |
60.6 |
3.23, s |
60.5 |
|
|
|
|
4′-OMe |
3.92, s |
56.9 |
3.92, s |
56.9 |
3.97, s |
56.8 |
3.97, s |
56.8 |
5′-OMe |
3.95, s |
56.7 |
3.96, s |
56.7 |
3.92 s |
56.9 |
3.91, s |
56.9 |
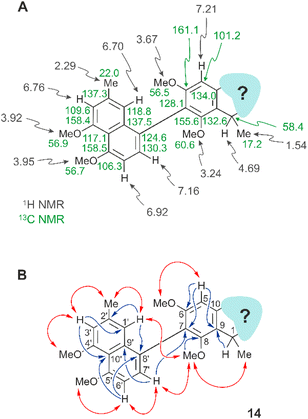 |
| Fig. 2 1D and 2D NMR data of the naphthalene moiety and the phenyl part of the heterocyclic molecular half of ancistrobrevoline A (14) closely resembling those of conventional naphthylisoquinoline alkaloids, while the novel-type, yet unknown structural elements of 14 are overlaid in pale blue. (A) 1H and 13C NMR data (in methanol-d4, δ in ppm). (B) HMBC (single blue arrows) and NOESY (double red arrows) interactions evidencing the constitution of the molecular units of 14 and the position of the biaryl axis between them. – For better comparability, the atom numbering follows the one usually applied for naphthylisoquinoline alkaloids. | |
Even the heterocyclic unit displayed, in part, the usual features as in other naphthylisoquinoline alkaloids, like the isocyclic benzene ring with the axis at C-7 (for the atom numbering applied, see ref. 26) and two methoxy functions at C-6 and C-8, as deduced from two singlets at δH 3.67 and 3.24, each corresponding to three protons, and from an aromatic singlet appearing at δH 7.21 (H-5) (Fig. 2A). The coupling pattern suggested the presence of a phenyl unit with no substituent at C-5, thus leaving C-7 (δC 128.1) as the axis-bearing carbon atom. This assumption was corroborated by HMBC interactions of H-5 (δH 7.21) to C-7 (δC 128.1) and C-9 (δC 155.6), and a 4J correlation of C-7 with H-7′ (δH 7.16) across the biaryl axis (Fig. 2B). Likewise present was the usual CHMe entity at C-1, as seen from a quartet (H-1, δH 4.69) in the aliphatic region of the 1H NMR spectrum, and from NOESY interactions between the protons of the methyl group appearing as a doublet at δH 1.54 and those of the OMe function at C-8 (δH 3.24), and between that O-methyl group and the two aromatic protons H-1′ (δH 6.70) and H-7′ (δH 7.16) (Fig. 2B), indicating that the methyl group at δH 1.54 was located at C-1 (δC 58.4), while the upfield-shifted OMe function resonating at δH 3.24 was at C-8 (δC 155.6).
The rest of the molecule, however, as overlaid in light blue (see Fig. 2 and structure I in Fig. 3), seemed substantially different from that of all other known naphthylisoquinoline alkaloids.
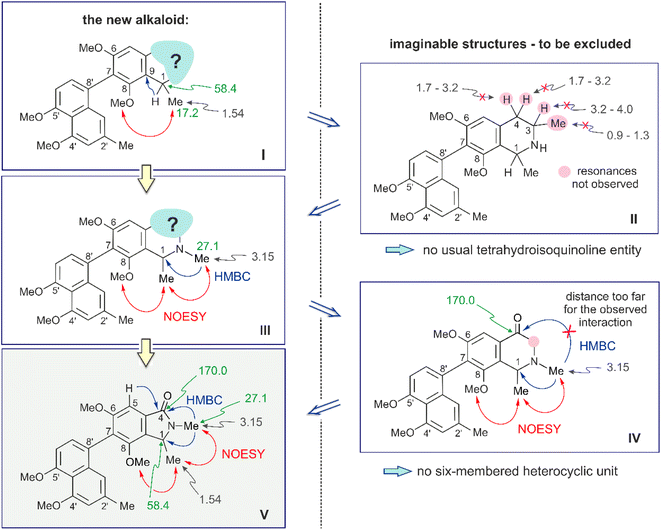 |
| Fig. 3 Decisive 1H and 13C NMR shifts (in methanol-d4, δ in ppm) and key NOESY (double red arrows) and HMBC (single blue arrows) interactions in structures I, III, and V, evidencing the structural elements of the heterocyclic ring in ancistrobrevoline A (14). The two geminal protons at C-4, the proton and the methyl group at C-3 (underlaid in pink in structure II), typical of usual naphthyltetrahydroisoquinoline alkaloids, were not observed in the 1H NMR spectrum of 14. Deletion of the carbon atom C-3 (marked in pink in structure IV) leads to the formation of the isoindolinone subunit of 14 as shown in structure V. | |
It was in particular the aliphatic region of the 1H NMR spectrum that diverged massively from that of a usual naphthyltetrahydroisoquinoline alkaloid, characterized by the lack of resonances for the methyl group at C-3 (which normally appears around δH 0.9 and 1.3), the missing usual multiplet of H-3 in the region between δH 3.2 and 4.0, and the likewise absent signals of the diastereotopic geminal protons at C-4, which usually appear as doublets of doublets resonating around δH 1.7–3.2 (see structure II in Fig. 3). The observation of a downfield-shifted three-proton singlet (δH 3.15) suggested the presence of an N-methyl group, which was in accordance with the observation of a NOESY correlation to the protons of the methyl group at C-1 and with an HMBC interaction from the protons of that methyl group (resonating at δH 3.15) to the aliphatic carbon atom C-1 (δC 58.4) (see structure III in Fig. 3).
A further most notable difference compared to usual naphthylisoquinolines was the presence of an additional oxygen function in that eastern part of the molecule. The 13C NMR data displayed a downfield-shifted signal at δC 170.0, typical of a carbonyl function. The observation of an HMBC cross-peak from the N-methyl group at δH 3.15 to that carbonyl C-atom, however, did not match with the structure of a six-membered tetrahydropyridinone as in structure IV (Fig. 3), since the distances between the two structural elements, N–Me and C-4, would have been two far for such an interaction.
The observed HMBC and NOESY interactions, while not fitting with the presence of an isoquinoline unit, gave conclusive evidence of a contracted ring in the new alkaloid, as a consequence of the loss of the carbon atom C-3, thus giving rise to a five-membered isoindolinone skeleton. This structural assignment presented in Fig. 3 (see structure V) was in agreement with the lack of the signal for C-3 in 13C NMR (normally resonating at δC 45–53) and with the appearance of a signal at 1673 cm−1 in the IR spectrum, which is characteristic of a carbonyl group as part of an amide function. In conclusion, the new alkaloid had to be a 7,8′-coupled biaryl alkaloid, structurally differing from a conventional naphthylisoquinoline by its unprecedented isoindolinone part.
The absolute configuration at the stereocenter of the new alkaloid at C-1 in the isoindolinone ring system was determined by a ruthenium-mediated oxidative degradation procedure (see the ESI†) developed by us earlier.6,27 Although this periodate oxidation method had initially been designed for the degradation of 1,3-disubstituted tetra- or dihydroisoquinolines, not for isoindolinones, with their stable C,N-bond, the reaction luckily succeeded in this case, too, affording the simple and easy-to-analyze chiral amino acid N-methyl-D-alanine, hence establishing the absolute configuration at the stereocenter as R.
Unfortunately, it was not possible to assign the configuration at the axis relative to the stereocenter at C-1 by NMR, because no significant long-range NOESY interactions across the biaryl axis were observed, like between Me-1 and/or H-1 in the isoindolinone ring and H-1′ or H-7′ in the naphthalene half. Apparently the small ring size of the heterocycle leads to enlarged distances between the substituent at C-1 and the respective H atoms attached to the naphthalene part. Therefore, quantum-chemical electronic circular dichroism (ECD) calculations were performed for both possible atropo-diastereomers.
The experimental ECD spectrum of the isolated naphthylisoindolinone (Fig. 4) was very similar to the one calculated for the P-isomer (in red) at the TDωB97XD3/def2-TZVP//B3LYP-D3/def2-TZVP level, while being virtually opposite to the one predicted for M (in blue), which unambiguously showed that the new compound was P-configured and, thus, had the full absolute stereostructure 14, as presented in Fig. 1. This novel natural product was given the name ancistrobrevoline A.
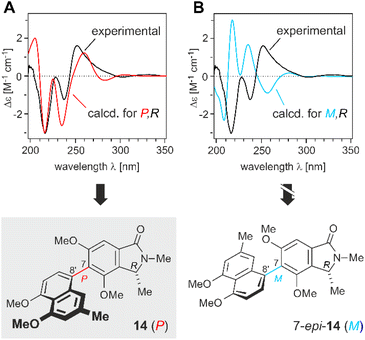 |
| Fig. 4 Assignment of the absolute configuration of ancistrobrevoline A (14) by comparison of its experimental ECD spectrum (in black, taken in methanol) with the ones calculated (A) for the (1R,7P)-isomer (ECD curve in red) and (B) for 1R,7M (in pale blue) using TDωB97XD3/def2-TZVP//B3LYP-D3/def2-TZVP. | |
Ancistrobrevoline B (15). The second new minor root bark metabolite was spectroscopically almost identical to ancistrobrevoline A (14), with only slightly different chemical shifts (Table 1), thus evidencing the presence of another naphthylisoindolinone, again 7,8′-coupled, as attributed by the observed key HMBC and NOESY interactions (see the ESI†). The 1H NMR spectrum displayed a three-proton singlet at δH 3.13, hinting at an N–Me group, and the 13C NMR data revealed the presence of a downfield-shifted signal at δC 169.9, typical of the amidic carbonyl entity of the isoindolinone unit. This was in accordance with a carbonyl signal at 1678 cm−1 in the IR spectrum of the new metabolite. HR-ESI-MS showed a protonated molecular-ion peak [M + H]+ at m/z 408.18150 (calcd for C24H26NO5+, 408.18055) and an [M + Na]+ peak at m/z 430.16117 (calcd for C24H25NNaO5+, 430.16249), thus having 14 mass units less than 14, suggesting that the isolated new metabolite might be an O-demethylated analogue of 14. In agreement with this assumption, the 1H NMR spectrum displayed singlets for only three O-methyl units, resonating at δH 3.23, 3.92, and 3.96 (Table 1). They were assigned to be located at C-8, C-4′, and C-5′, based on NOESY interactions with Me-1/H-1, H-3′, and H-6′, respectively, similar as for ancistrobrevoline A (14) (Fig. 2). Thus, different from 14, one of the oxygen functions in the new metabolite was a free hydroxy group at C-6. The ECD spectrum of the new compound (see the ESI†) matched well that of ancistrobrevoline A (14), proving that the absolute configuration at the biaryl axis was again P, as for 14. Likewise as in the case of 14, the degradation6,27 established the absolute configuration at the stereocenter at C-1 as R by the formation of N-methyl-D-alanine. The new alkaloid thus had the absolute stereostructure 15 as presented in Fig. 1, hence being the 6-O-demethyl analogue of 14. It was named ancistrobrevoline B.
Ancistrobrevoline C (16). The third new compound was obtained as a yellow amorphous powder. According to HRESIMS and 13C NMR, it had a molecular formula of C24H26NO5, as deduced from its monoprotonated molecular ion, [M + H]+, at m/z 408.17974, identical to that of ancistrobrevoline B (15). Again, the 1H NMR spectrum showed signals for three C-methyl groups (δH 1.55, 2.09, and 3.14), for three methoxy functions (δH 3.64, 3.92, and 3.97), and for one aliphatic proton (δH 4.58) and five aromatic ones (δH 6.81, 6.85, 6.91, 7.01, and 7.18) (Fig. 5A). This, together with an IR band at 1675 cm−1 and a downfield-shifted signal at δC 170.4 in 13C NMR, characteristic of an amidic carbonyl entity, indicated the new minor metabolite from the root bark of A. abbreviatus to be yet another naphthylisoindolinone alkaloid. Different from 15, the new compound exhibited a spin pattern of the aromatic protons consisting of two singlets, one doublet, and two doublets of doublets. This was in agreement either with a 5,1′- or a 7,1′-coupling, of which the 5,1′-linkage was excluded due to HMBC interactions of H-5 (δH 7.01) to C-4 (δC 170.4) and C-7 (δC 120.5) and a NOESY correlation between H-5 and MeO-6 (δH 3.64) in the isoindolinone part (Fig. 5B). This assignment was confirmed by NOESY correlation sequences in the series {H-8′ ↔ H-7′ ↔ MeO-5′} and {MeO-4′ ↔ H-3′ ↔ Me-2′} and by HMBC cross-peaks of Me-2′ (δH 2.09) and H-8′ (δH 6.81) to C-1′ (δC 58.3) in the naphthalene moiety. The three methoxy groups were determined to be located at C-6, C-4′, and C-5′, based on NOESY interactions with their neighboring protons, H-5, H-3′, and H-6′, respectively (Fig. 5B). Another oxygenated carbon atom was found to be located in the phenyl ring, at C-8 (δC 151.9), bearing a free hydroxy function. The ruthenium-mediated oxidative degradation6,27 of the new compound afforded the D-enantiomer of N-methylalanine, establishing the absolute configuration at the stereocenter as R.
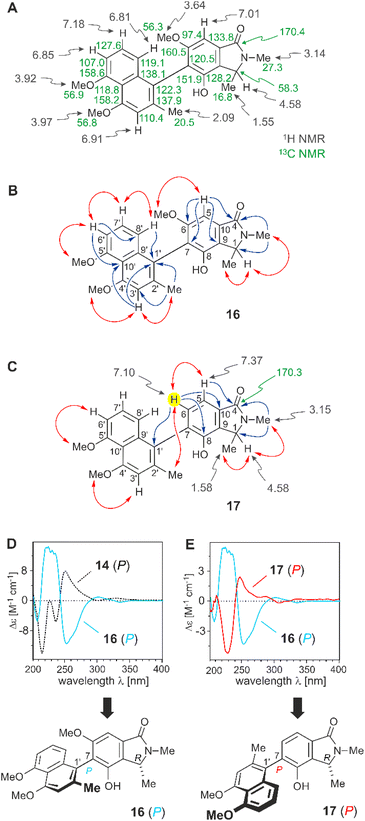 |
| Fig. 5 (A) 1H and 13C NMR data (in methanol-d4, δ in ppm) of ancistrobrevoline C (16). (B) Key HMBC (single blue arrows) and NOESY (double red arrows) interactions indicative of the constitution of 16. (C) Decisive 1D and 2D NMR data of ancistrobrevoline D (17) evidencing the presence of an additional aromatic proton (highlighted in yellow). (D) Assignment of the absolute axial configuration in 16 by comparison of its experimental ECD spectrum (in pale blue, full line) with the ECD curve (in black, dotted line) of ancistrobrevoline A (14, for its structure, see Fig. 4). (E) Comparison of the ECD spectrum of 17 (in red, full line) with that of the likewise 7,1′-coupled ancistrobrevoline C (16) (in pale blue, full line). | |
The absolute axial configuration of the new alkaloid was deduced by comparison of its experimental ECD spectrum (full line in blue) with that of ancistrobrevoline A (14, dotted line in black). The new alkaloid differed from 14 only by the position of the methyl group in the naphthalene part or, in other words, by the coupling type (7,1′ versus 7,8′), and by the substitution pattern at C-6 and C-8 (OMe/OH versus OH/OMe in 14). The ECD spectrum of the new alkaloid was virtually opposite to the one of 14 (Fig. 5D), suggesting that the larger part of the naphthalene unit should be directed down, i.e., with an opposite stereoarray as compared to 14 and 15. According to the Cahn-Ingold-Prelog priority rules, however, the descriptor was again assigned as P, i.e., formally the same as for 14 and 15. The new compound thus had the full stereostructure 16 as presented in Fig. 5D. It was named ancistrobrevoline C.
Ancistrobrevoline D (17). The fourth compound was isolated as a yellow amorphous solid with a molecular formula of C23H23NO4, as established from the corresponding [M + Na]+ adduct, C23H23NNaO4, at m/z 400.15033. 1H and 13C NMR data (Table 1) revealed the new metabolite to be yet another 7,1′-linked naphthylisoindolinone alkaloid, with a constitution strongly resembling that of the above-described ancistrobrevoline C (16). Similar to 16, it displayed a 4′,5′-dimethoxy-substituted naphthalene portion, as deduced by NOESY cross-peaks between MeO-4′ and H-3′ and between MeO-5′ and H-6′. Likewise observed in 1D and 2D NMR were the typical structural features of the heterocyclic ring in the isoindolinone moiety, as outlined in Fig. 5C. The molecular weight of the new metabolite, however, was lower than those of 14–16, hinting at the presence of only four oxygen atoms. This gave rise to the assumption that the new alkaloid might be derived from the subclass of dioncophyllaceae-type compounds, which are characterized by the lack of an oxygen function at C-6 (and the presence of an R-configuration at C-3, which, however, does not apply here). Indeed, the most significant difference in the NMR spectrum of the new metabolite compared to that of 16 was the appearance of an additional aromatic proton, which was supposed to be the hydrogen atom at C-6. The coupling pattern of the aromatic protons showed one singlet (H-3′), two doublets (H-6′ and H-8′), and one doublet of doublets (H-7′) in the naphthalene part (Table 1), and two doublets resonating at δH 7.10 and δH 7.37, located in the isoindolinone portion. This assignment was in accordance with COSY interactions between H-5 (δH 7.37) and H-6 (δH 7.10), which showed ortho-coupling to each other (J = 7.5 Hz), and it was also confirmed by HMBC cross-peaks from H-6 to C-1' (δC 126.1), C-10 (δC 133.6), and C-8 (δC 151.5) (Fig. 5C). Oxidative degradation6,27 established the absolute configuration at the stereocenter at C-1 as R. The ECD spectrum of the new alkaloid (Fig. 5E) was virtually opposite to that of ancistrobrevoline C (16), while resembling that of ancistrobrevolines A (14) and B (15) (see ESI†), hence establishing the absolute axial configuration as P. The new alkaloid consequently had the full absolute stereostructure 17 as presented in Fig. 5E. It was henceforth named ancistrobrevoline D.
Proposed biosynthetic origin of naphthylisoindolinone alkaloids
The structures of the discovered four novel alkaloids 14–17, with their isoindolinone parts connected to the naphthalene moieties via a stereogenic axis, are unprecedented, and differ substantially from previously described artificial naphthylisoindolinones.28–30 In the field of ‘normal’, amino-acid derived benzylisoquinoline alkaloids, some few other, yet naphthalene-devoid isoindolinones are known like aristoyagonine (18)30–32 and aristolactam I (19)30,33,34 (Fig. 6A). Biosynthetically, 18 and 19 are assumed to be formed from the corresponding benzyltetrahydroisoquinoline alkaloids, by 3,4-dioxygenation, followed by a benzilic acid rearrangement, with loss of a carbon atom and ring contraction.30–34
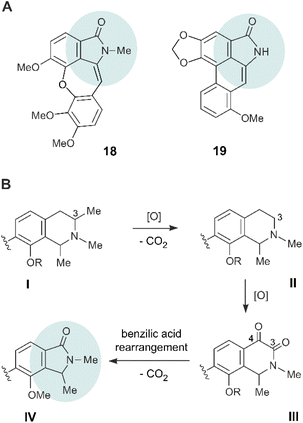 |
| Fig. 6 (A) Structures of the conventional, naphthalene-devoid isoindolinones, aristoyagonine (18) and aristolactam (19). (B) From ‘normal’ naphthyltetrahydroisoquinolines (I) to ancistrobrevolines (IV): Proposed biosynthetic pathway, with oxidation, decarboxylation, and ring contraction reactions. | |
In an analogous way, the four novel naphthylisoindolinones 14–17 (general partial structure IV, see Fig. 6B) might arise from the respective naphthyltetrahydroisoquinoline alkaloids I, via the corresponding 3,4-diketones III – yet with the additional necessity of eliminating the methyl group at C-3, maybe via II, or possibly via the 3-oxo analogues. The loss of the C-3 methyl group might occur by a stepwise oxidation to the respective carboxylic acid followed by decarboxylation. A similar elimination of a C-methyl group in naphthylisoquinoline alkaloids had already been observed at C-1 (see the structure of 1-nor-8-O-demethylancistrobrevine H, 12, in Fig. 1), as found to be produced by the same plant, A. abbreviatus,18 and also oxygenations at Me-3 (see ref. 5, 35 and 36) and at C-4 (see ref. 5, 37 and 38) are known to occur in the biosynthesis of naphthylisoquinoline alkaloids. As in the case of 18 and 19, the resulting diketones III could then undergo a benzilic acid rearrangement, with loss of CO2, to provide the isoindolinone unit IV.
Cytotoxic activity of ancistrobrevolines A and B against breast and lung cancer cells
Some of the mono- and dimeric naphthylisoquinoline alkaloids isolated from the West African liana A. abbreviatus display strong cytotoxic activities against various cancer cell lines, among them multiple myeloma,9,39 leukemia,16,39 cervical,15,18,40 fibrosarcoma,17 colon,17 and pancreatic14,15,40 cancer cells. Investigations regarding the specificity and effectiveness of the compounds on the viability of those cancer cells indicated that the alkaloids may have a substantial therapeutic potential and can thus be regarded as promising candidates for drug development.
And only recently, dioncophylline A (5), a main constituent of A. abbreviatus, and related compounds have been reported to exhibit pronounced antiproliferative activities in the submicromolar range against two breast cancer cell lines, MCF-7 and MDA-MB-231.41 Breast42,43 and lung44,45 cancer are among the most commonly diagnosed lethal cancer types worldwide. Although the death rate caused by cancer has been decreasing over the past years,46 as a result of improved diagnostic and therapeutic approaches to many cancer types, treatment of lung and breast cancer still suffers from a poor prospect to completely eradicate the tumor cells, which in turn leads to a recurrent and metastatic manifestation of the disease, and thus to high mortality rates.42–48 Therefore, the search for novel therapeutic agents to efficiently combat lung and breast cancer cells still remains an important task.
Given the pronounced antiproliferative activities of some of the naphthylisoquinoline alkaloids such as dioncophylline A (5),17,39,41 jozimine A2 (11),17,40 or ancistrobrevidine C (8),15 with their usual six-membered heterocyclic ring system, it seemed rewarding to likewise study the cytotoxic potential of the new ring-contracted naphthylisoindolinones. This was also promising in view of the fact that aristolactams (Fig. 6A) and some of their non-natural analogues,28,33 all equipped with an isoindolinone moiety similar to the one of ancistrobrevolines A–D (14–17), are known to exhibit remarkable anticancer properties, too.34,49,50
We here, therefore, report on the evaluation of ancistrobrevolines A (14) and B (15) for their growth-inhibitory effects against MCF-7 breast adenocarcinoma cells and against the non-small cell lung cancer (NSCLC) cell line A549. Due to the lack of isolated material of ancistrobrevines C (16) and D (17), only 14 and 15 were assessed.
These two alkaloids exhibited only low cytotoxic effects against MCF-7 breast cancer cells, as determined by the MTT assay (see Experimental Section). The results showed that exposure of MCF-7 cells to varying concentrations (10, 30, 50, 70, and 100 μM) of 14 and 15 resulted in growth-inhibitory activities of 19-38% (for 14) and 3.7–43% (for 15) (see Table 2). Although 14 and 15 did not reach the pronounced growth-inhibitory activities of the previously investigated naphthylisoquinoline alkaloids on breast cancer cells,41 the results are important contributions to our ongoing investigations on structure–activity (SAR) relationships.
Table 2 Concentration-dependent cytotoxic activity of ancistrobrevolines A (14) and B (15) against breast cancer cells (MCF-7)
Compound |
Cytotoxicity ± SDa (%) |
10 μM |
30 μM |
50 μM |
70 μM |
100 μM |
The results are shown as mean values ± standard deviation (SD); the experiments were done in triplicate (n = 3). |
14 |
19.1 ± 22.7 |
25.3 ± 17.0 |
27.1 ± 6.82 |
28.5 ± 12.2 |
37.7 ± 12.7 |
15 |
3.68 ± 11.5 |
17.6 ± 31.5 |
34.0 ± 8.47 |
37.4 ± 13.0 |
43.0 ± 6.73 |
Against A549 lung cancer cells, the cytotoxic activities of 14 and 15 were significantly higher, reaching ca. 8–72% and 36–68%, respectively (see Table 3), with IC50 values of 34.6 μM (for 14) and 9.05 μM (for 15) (Fig. 7). Thus, the IC50 value of ancistrobrevoline B (15) was markedly lower than that of ancistrobrevoline A (14), showing a higher sensitivity of A549 lung cancer cells to the growth inhibition induced by 15, which further evidenced the pronounced structure–function diversity in naphthylisoquinoline alkaloids. Studies on the immortalized human mammary epithelial cell line MCF-10A51 revealed ancistrobrevoline B (15) to display moderate cytotoxicity on these non-tumorigenic cells, which led to a selectivity index of 1.4 regarding the A549 cancer cell line, whereas ancistrobrevoline A (14) showed no selectivity towards the cancer cells compared to the normal epithelial MCF-10A cells (SI ≈ 1).
Table 3 Concentration-dependent antiproliferative activity of ancistrobrevolines A (14) and B (15) against lung cancer cells (A549)
Compound |
Cytotoxicity ± SD (%)a |
10 μM |
30 μM |
50 μM |
70 μM |
100 μM |
The results are shown as mean values ± standard deviation (SD); the experiments were done in triplicate (n = 3). |
14 |
8.27 ± 10.3 |
26.4 ± 4.01 |
54.3 ± 3.47 |
58.0 ± 3.66 |
72.7 ± 2.88 |
15 |
36.4 ± 9.62 |
61.9 ± 2.11 |
63.8 ± 9.52 |
64.0 ± 1.69 |
68.3 ± 3.88 |
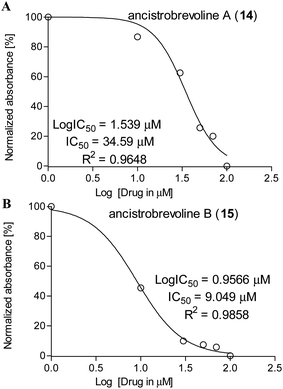 |
| Fig. 7 Treatment of lung cancer (A549) cells with ancistrobrevolines A (14) and B (15). Determination of IC50 and log IC50 values, and regression analysis of the effects of (A) 14 and (B) 15 (10, 30, 50, 70, and 100 μM each) on the A549 cells after 48 h of incubation; the results are expressed as mean ± SD. | |
Cytotoxic activity of ancistrobrevolines A and B against breast and lung cancer cells
The manifestation of breast cancer is strongly associated with the occurrence of a small atypical population of tumor-initiating cells that possess the capacity of unlimited propagation and multipotent differentiation.52–54 These cancer-stem-like cells have been identified in a variety of tumors and are under suspicion to be a causative factor of tumor growth and metastasis, eventually facilitating resistance to chemotherapies, and thus, leading to treatment failure and tumor recurrence.55 Therefore, targeting these cancer stem cells in breast cancer has become a promising approach.56
Cultivation of human breast epithelial adenocarcinoma cells MCF-7 under non-adherent non-differentiating conditions as described in the Experimental section results in the generation of discrete clusters of spheroids, termed mammospheres (Fig. 8A), which undergo asymmetric division and self-renewal, making them aggressive and highly metastatic.57,58 Before this background, we studied the effect of ancistrobrevines A (14) and B (15) on the sphere formation potential of MCF-7 cells after exposure to 10, 30, 50, 70, and 100 μM of the new naphthylisoindolinone alkaloids. After 5 d of treatment, the size of the spheroids was captured using a phase-contrast microscope. As shown in Fig. 8, compounds 14 and 15 significantly reduced the size of the spheres in MCF-7 culture compared to the non-treated control group, where large spheroids were observed. A dose-dependent spheroid inhibitory activity of ancistrobrevolines A (14) and B (15) was found in MCF-7-derived mammospheres. The results indicate the reduction of stemness and self-renewal of MCF-7 cells in the presence of 14 and 15.
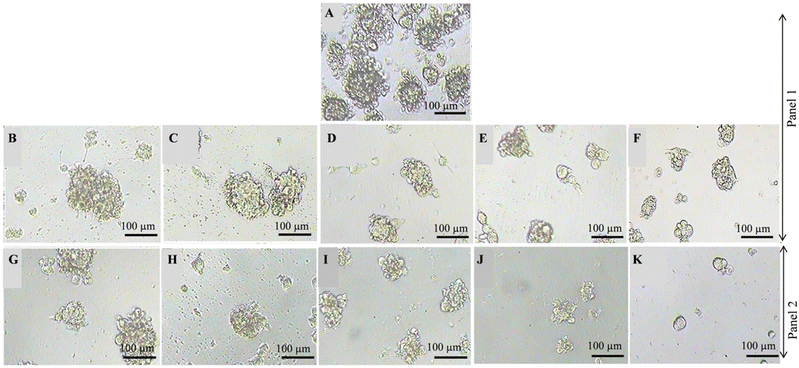 |
| Fig. 8 Cytotoxic effects of ancistrobrevolines A (14) and B (15) on the formation of MCF-7-derived breast cancer stem-like cells (mammosphere). (A) Mammospheres derived from untreated MCF-7 cells as a control. (B–F) MCF-7 cells derived from mammospheres treated with 14 at concentrations of 10 (B), 30 (C), 50 (D), 70 (E), and 100 μM (F). (G-K) MCF-7 cells derived from mammospheres treated with 15 at concentrations of 10 (G), 30 (H), 50 (I), 70 (J), and 100 μM (K). The mammospheres were cultured for 5 d to form spheres in ultra-low attachment surface plates. The images of the mammospheres were taken at 10 × resolution using phase-contrast microscopy. | |
Experimental
General experimental procedures
Optical rotations were recorded using a JASCO P-1020-polarimeter (JASCO, Gross-Umstadt, Germany) operating with a sodium light source (λ = 589 nm). UV spectra were measured on a Shimadzu UV-1800 spectrophotometer, and ECD spectra were recorded under nitrogen atmosphere on a JASCO J-715 spectrometer at room temperature, using a standard cell (0.02 cm), then processed using the SpecDis software.59,60 IR spectra were measured on a JASCO FT/IR-410 spectrometer. 1D and 2D NMR spectra were taken on a Bruker DMX 600 instrument at ambient temperature, using methanol-d4 as the solvent, with the 1H and 13C signals of the solvent (δH 3.31 and δC 49.15 ppm) as the internal reference. Chemical shifts (δ) are given in parts per million (ppm), and coupling constants (J) are reported in Hertz (Hz). Multiplicities of NMR signals are denoted as singlet (s), doublet (d), doublet of doublets (dd), or quartet (q). Spectra were processed using an the MestReNova NMR (Mestrelab Research) software. HRESIMS spectra were acquired on a Bruker Daltonics micrOTOF-focus mass instrument. GC-MSD analysis was done on a GCMS-QP 2010SE System (Shimadzu). Preparative HPLC was accomplished on a JASCO System (PU-1580 Plus) in combination with a PDA detector at 195–650 nm (JASCO MD-2010 Plus diode array detector). For the isolation and purification of the constituents of the root bark extracts, a SymmetryPrep C18 column (19 × 300 mm, 7 μm, Waters) was used, applying a flow rate of 10 mL min−1; mobile phases: (A) 90% H2O with 10% CH3CN (0.05% trifluoroacetic acid) and (B) 90% CH3CN with 10% H2O (0.05% trifluoroacetic acid). For further purification, a Waters X-Select HSS PFP column (10 × 250 mm, 5 μm) was applied; mobile phases: (A) 90% H2O with 10% CH3OH (0.05% trifluoroacetic acid) and (B) 90% CH3OH with 10% H2O (0.05% trifluoroacetic acid).
Plant material
Roots of Ancistrocladus abbreviatus Airy Shaw (Ancistrocladaceae) were collected by one of us (late Prof. L. Aké Assi) in May 1996, in the Parc National de Taï, in the Southwest of the Ivory Coast. A voucher specimen (no. 3) has been deposited at the Herbarium Bringmann, Institute of Organic Chemistry, University of Würzburg.
Chemical and reagents
For the purification of the plant fractions by preparative HPLC, HPLC-grade solvents MeCN, MeOH, purchased from Sigma-Aldrich, were utilized. Spectroscopic-grade methanol was used for the ECD and UV measurements of the pure alkaloids. Ultra-pure water for HPLC was obtained from an Elga Purelab Classic System. (S)-MTPA used for the degradation was purchased from Sigma-Aldrich (Steinheim, Germany).
Dulbecco's Modified Eagle Medium (DMEM), fetal bovine serum (FBS), trypsin, penicillin, streptomycin, horse serum, epidermal growth factor (EGF), fibroblast growth factor (FGF), B-27 supplements, hydrocortisone, and insulin were purchased from Thermo Fisher Scientific (Waltham, MA, USA). For cell proliferation assays, 3-(4,5-dimethylthiazol-2-yl)-2,5-diphenyl-tetrazolium bromide (MTT) and cholera toxin were obtained from Sigma-Aldrich (St. Louis, MO, USA).
Extraction and purification of naphthylisoindolinones
The air-dried powdered root bark material of A. abbreviatus (ca. 420 g) was macerated in methanol (2 × 3 L) at 40 °C, followed by ultrasonication for 1 h. The extract was filtered, and the solvent was evaporated under reduced pressure to give a brownish solid residue, which was re-dissolved in 90% aqueous methanol and partitioned with n-hexane. The aqueous methanolic layer was dried under reduced pressure to yield 17.5 g of an alkaloid-rich fraction, which was directly subjected to fractionation over a silica gel column using a linear solvent system consisting of CH3OH and CH2Cl2 with a gradient increase of CH3OH (0 → 90%), giving rise to five naphthylisoindolinone-enriched fractions (F1–F5). The alkaloid-containing subfractions were purified on the X-Select HSS PFP column by applying the following method: 0 min: 68% B, 38 min: 88% B, affording 0.7 mg of ancistrobrevoline C (16) (retention time 22.0 min), 0.6 mg of ancistrobrevoline B (15) (retention time 25.3 min), 0.5 mg of ancistrobrevoline D (17) (retention time 26.4 min), and 1.1 mg of ancistrobrevoline A (14) (retention time 28.1 min).
Ancistrobrevoline A (14). Yellow, amorphous powder; [α]23D +11.5 (c 0.05, MeOH); UV/Vis (MeOH) λmax (log
ε) 196 (4.1), 213 (3.7), 229 (3.6), 261 (3.0), 306 (3.0) nm; ECD (c 0.10; MeOH) λmax (log
ε) 213 (−2.7), 235 (−1.1), 249 (+1.5), 326 (+0.1) cm2 mol−1; IR (ATR) νmax 3400, 2967, 2929, 1673, 1585, 1463, 1421, 1383, 1198, 1132, 951, 808, 672 cm−1; 1H NMR and 13C NMR data, see Table 1; HR-ESI-MS m/z 444.17711 [M + Na]+ (calcd for C25H27NNaO5, 444.17814).
Ancistrobrevoline B (15). Yellow, amorphous solid; [α]23D –8.3 (c 0.07, MeOH); UV/Vis (MeOH) λmax (log ε) 196 (4.1), 213 (3.5), 227 (3.4), 261 (2.8), 306 (2.8) nm; ECD (c 0.10; MeOH) λmax (log
ε) 212 (−2.4), 232 (−1.1), 247 (+1.7), 301 (−0.1) cm2 mol−1; IR (ATR) νmax 2965, 1678, 1587, 1463, 1428, 1383, 1196, 1135, 836, 803, 673 cm−1; 1H NMR and 13C NMR data, see Table 1; HR-ESI-MS m/z 408.1815 [M + H]+ (calcd for C24H26NO5, 408.1805), and m/z 430.1611 [M + Na]+ (calcd for C24H25NNaO5, 430.1624).
Ancistrobrevoline C (16). Yellow, amorphous powder; [α]23D –18.9 (c 0.05, MeOH); UV/Vis (MeOH) λmax (log ε) 197 (4.1), 215 (3.5), 228 (2.4), 261 (2.8), 304 (2.7) nm; ECD (c 0.10; MeOH) λmax (log
ε) 205 (−0.6), 225 (+1.5), 250 (−1.3), 300 (+0.09) cm2 mol−1; IR (ATR) νmax 2927, 2850, 1675, 1589, 1459, 1387, 1264, 1200, 1135, 836, 805, 719 cm−1; 1H NMR and 13C NMR data, see Table 1; HR-ESI-MS m/z 408.1797 [M + H]+ (calcd for C24H26NO5, 408.1805), and m/z 430.1608 [M + Na]+ (calcd for C24H25NNaO5, 430.1624).
Ancistrobrevoline D (17). Yellow, amorphous powder; [α]23D –24.0 (c 0.04, MeOH); UV/Vis (MeOH) λmax (log ε) 197 (4.0), 229 (3.2), 295 (2.6), 334 (2.5) nm; ECD (c 0.10; MeOH) λmax (log
ε) 209 (+0.1), 226 (−2.7), 246 (+1.3), 286 (+0.08) cm2 mol−1; IR (ATR) νmax 2969, 2850, 1680, 1594, 1459, 1327, 1204, 1134, 1075, 725, 672 cm−1; 1H NMR and 13C NMR data, see Table 1; HR-ESI-MS m/z 400.1503 [M + Na]+ (calcd for C23H23NNaO4, 400.1519).
Oxidative degradation
The naphthylisoindolinone alkaloids 14–17 (ca. 0.5 mg each) were subjected to a ruthenium(VIII)-mediated periodate degradation,6,27 followed by derivatization of the resulting amino acids with CH3OH/HCl and (R)-α-trifluoromethylphenyl-acetylchloride [(R)-MTPA-Cl, prepared from (S)-MTPA]. The absolute configurations were assigned by GC on a dimethylpolysiloxane-coated capillary column coupled to a mass-selective detector and comparison with the corresponding derivatives of authentic amino acids of known absolute configuration.
Computational details
For the calculation of the ECD spectra, a simplified approach was selected. Only the main conformers of the possible configurations of compound 14 were optimized with B3LYP-D3//def2-TZVP.61–63 The conformational freedom of the methoxy groups was not taken into account because their influence on the ECD should be negligible. Excited states results were achieved utilizing TDωB97XD3/def2-TZVP(-f).64,65 All calculations were done using ORCA 4.1.0.2.66 For processing of the data, SpecDis 1.71 was used59,60 with a UV shift of 18 nm, finally providing the computed ECD spectra, which were compared with the experimental one of 14.
Cell culture
Human breast (MCF-7) and lung (A549) cancer cells were purchased from the National Centre for Cell Sciences, Pune, India. Normal, non-tumoral breast cells (MCF-10A) were from ATTC, University Boulevard, Manassas, VA, USA. Breast and lung cancer cells were cultured in DMEM supplemented with 10% (v/v) FBS (heat-inactivated), 2 mM L-glutamine, 100 μg mL−1 of streptomycin and 100 U mL−1 of penicillin. The normal breast cells (MCF-10A) were maintained in DMEM/F12 media supplemented with FBS (10%), horse serum (5%), cholera toxin (100 ng mL−1), EGF (20 ng mL−1), hydrocortisone (0.5 mg mL−1), and insulin (10 μg mL−1) and incubated at 37 °C and 5% CO2.
Cytotoxicity study using the MTT assay
Approximately 104 cells/well were seeded in 96-well plates and kept in the incubator at 37 °C and 5% CO2. The cells were kept overnight to allow them to adhere and were then fed with fresh medium containing different concentrations (10, 30, 50, 70, and 100 μM) of the test compounds, ancistrobrevolines A (15) and B (16). After incubation for 48 h, cell viability was assessed using the MTT assay.67 At the stipulated time following the treatment of the test sample, medium was aspirated; MTT (5 mg mL−1) was added in each well and incubation was continued at 37 °C for 2 h. The plates were spun, supernatants were discarded, and purple-colored precipitates of formazan were dissolved in 100 μL of dimethylsulfoxide (DMSO). The color absorbance was recorded at 590 nm using a microplate reader (BioTek Instruments, Inc. USA). IC50 calculations and regression analysis were performed using the GraphPad Prism 5.0 software.
The selectivity index (SI) of the test compounds was calculated using the following equation, where MCF-10A represents human normal cells, while A549 denotes lung cancer cells: SI = IC50 normal cells/IC50 cancer cells. The IC50 value indicates the concentration of the test drug required for 50% inhibition of the cancer cells. SI values > 1 indicate that the compound is selective against the respective cancer cells.68
Mammosphere formation and drug treatment
Mammospheres derived from MCF-7 cells were formed by seeding the cells in 6-well ultra-low-attachment surface plates (Corning, Tewksbury, MA, USA) at a density of 2 × 104 cells/well and cultured in serum-free DMEM-Ham's F12 nutrient mixture (1
:
1, v/v), supplemented with 5 mg mL−1 insulin, 0.5 mg mL−1 hydrocortisone, 2% B-27 supplements, 10 ng mL−1 fibroblast growth factor, and 20 ng mL−1 epidermal growth factor. The cells were incubated at 37 °C and 5% CO2 for 5 d to form mammospheres.69 To study the effect of ancistrobrevolines A (14) and B (15) on mammosphere formation, the cells were treated with different concentrations (10, 30, 50, 70, and 100 μM) of the agents and then allowed to form spheres for the next 5 d. The compound solutions were prepared using DMSO with its final non-toxic concentration (0.1%). An equal amount of DMSO was added to the non-treated control group. After 5 d, the mammospheres were photographed using phase-contrast microscopy, and the images were analyzed using the ImageJ analysis software.69
Conclusions
This paper describes the discovery of a structurally novel subclass of naphthylisoquinoline-related alkaloids, bearing a heterocyclic moiety that is ring-contracted to an isoindolinone entity. The results further highlight the “chemical creativity” of A. abbreviatus and demonstrate the outstanding phytochemical diversity of this productive West African liana. Already at the level of ‘normal’, standard-type naphthyltetrahydroisoquinoline alkaloids, the plant shows a remarkably broad variability,5,10,11,13,14,17 with no less than four out of seven known C,C-coupling types (5,1′, 7,1′, 5,8′, and 7,8′) and an otherwise very rare stereo-diversity, producing all four possible stereochemical combinations at C-1/C-3 (i.e. S,S, S,R, R,S, and R,R) (Fig. 1). In addition, it is the only plant that contains all four possible subclasses of naphthylisoquinoline alkaloids,5,17 namely Ancistrocladaceae- (6-OR, 3S), Dioncophyllaceae- (6-H, 3R), hybrid- (6-OR, 3R), and inverse hybrid-type (6-H, 3S) representatives, as outlined in Fig. 1.
Moreover, A. abbreviatus excels by the highest number of metabolic follow-up variations, resulting from – mostly oxidative – secondary modifications. Besides the phenol-oxidative coupling via the naphthalene portion, e.g. to give jozimine A2 (11),17 with its three stereogenic axes, and a nearly complete series of its numerous atropo-diastereomers, it is the only plant that produces naphthylisoquinoline alkaloids whose isocyclic moiety is further oxygenated to form an ortho-naphthoquinone as in ancistrobreviquinone A (10).15
Even more thrilling are the downstream variations of the tetrahydroisoquinoline portion, which can be dehydrogenated leading to the otherwise less frequently occurring 3,4-dihydroisoquinolines and to the (normally very rare) fully dehydrogenated naphthylisoquinoline alkaloids, with no less than seven representatives. Totally unprecedented even is the occurrence of 1-demethylated naphthylisoquinolines like 1-nor-8-O-demethylancistrobrevine H (12)18 and ring-cleaved representatives like ancistrosecoline D (13)18 (Fig. 1) – and, as described here, the discovery of oxidatively ring-contracted alkaloids, delivering new molecular scaffolds within the class of naphthylisoquinoline alkaloids.
These first four naphthylisoindolinones, ancistrobrevolines A–D (14–17), are all N-methylated, and they are all fully O-methylated in the naphthalene part and all are R-configured at C-1. In that respect they fit with the structural properties of the presumable precursors to 14, 15, 16, and 17, viz., ancistrobrevine A (1), its 6-O-demethyl analogue 2, ancistrobrevine D (3), and N-methyldioncophylline A (5) (Fig. 1), respectively, which, in agreement with our biosynthetic concept, all co-occur in A. abbreviatus, where they are even among the main alkaloids. Despite their structural similarities, the new metabolites do vary by their oxygenation degree at C-6, by the O-methylation degree at C-8, and by the stereo-orientation at the biaryl axis. This suggests that the structural requirements for the ring contraction are not particularly specific, evidencing that isoindolinone formation in naphthylisoquinoline alkaloids is possibly a more general principle. The work shows that naphthylisoquinoline alkaloids are not just metabolically stable bioactive end products, but can also be substrates for further – mainly oxidative – modifications.
Among all investigated naphthylisoquinoline-producing plants, A. abbreviatus shows the broadest diversity of secondary metabolites. It thus occupies a phytochemically – and phylogenetically – outstanding position within the Ancistro-cladaceae family, with a close relationship to Dioncophyllaceae lianas such as Triphyophyllum peltatum on the one hand and to geographically neighboring West and Central African Ancistrocladaceae plants on the other. Ancistrobrevolines A (14) and B (15) exhibited moderate antiproliferative activities against breast (MCF-7) and lung (A549) cancer cell lines. Furthermore, 14 induced a significant reduction of the formation of highly metastatic spheroid clusters in breast cancer-derived cancer-stem-like cells, worth further, more in-depth investigations on the antitumoral potential of naphthylisoquinoline alkaloids. The results presented here render phytochemical studies on the numerous further, as yet unidentified, trace compounds of Ancistrocladus plants and their biological evaluation rewarding goals.
Conflicts of interest
There are no conflicts to declare.
Acknowledgements
This work was supported by the Deutsche Forschungsgemeinschaft (DFG, Research Grant Br 699/14-2 “Molecular Phylogeny and Chemotaxonomy of the Ancistrocladaceae Plant Family”) and by a grant from the German Academic Exchange Service (Deutscher Akademischer Austauschdienst, DAAD) to one of us (S. F.). This publication was supported by the Open Access Publication Fund of the University of Würzburg. We thank Dr M. Grüne and Mrs. P. Altenberger for the NMR measurements, and Dr M. Büchner and Dr J. Adelmann for the MS analyses, all from the University of Würzburg. S. K. acknowledges DST-India for providing a DST-FIST grant to the Department of Biochemistry, Central University of Punjab, Bathinda, India.
Notes and references
- C. M. Taylor, R. E. Gereau and G. M. Walters, Ann. Mo. Bot. Gard., 2005, 92, 360–399 Search PubMed.
- M. Cheek, Kew Bull., 2000, 55, 871–882 CrossRef.
- H. K. Airy Shaw, Kew Bull., 1949, 4, 68–69 Search PubMed.
- H. K. Airy Shaw, Kew Bull., 1950, 5, 147–150 CrossRef.
- G. Bringmann and F. Pokorny, The naphthylisoquinoline alkaloids, in The Alkaloids, ed. G. A. Cordell, Academic Press Inc, New York, 1995, vol. 46, pp. 127–271 Search PubMed.
- B. K. Lombe, D. Feineis and G. Bringmann, Nat. Prod. Rep., 2019, 36, 1513–1545 RSC.
- N. Tajuddeen and G. Bringmann, Nat. Prod. Rep., 2021, 38, 2154–2186 RSC.
- S. R. M. Ibrahim and G. A. Mohamed, Fitoterapia, 2015, 106, 194–225 CrossRef CAS.
- X. F. Shang, C. J. Yang, S. L. Morris-Natschke, J. C. Li, X. D. Yin, Y. Q. Liu, J. W. Peng, M. Goto, J. Y. Zhang and K. H. Lee, Med. Res. Rev., 2020, 40, 2212–2289 CrossRef CAS PubMed.
- G. Bringmann, D. Lisch, H. Reuscher, L. Aké Assi and K. Günther, Phytochemistry, 1991, 30, 1307–1310 CrossRef CAS.
- G. Bringmann, R. Zagst, H. Reuscher and L. Aké Assi, Phytochemistry, 1992, 31, 4011–4014 CrossRef CAS.
- G. Bringmann, F. Pokorny, M. Stäblein, M. Schäffer and L. Aké Assi, Phytochemistry, 1993, 33, 1511–1515 CrossRef CAS.
- G. Bringmann, R. Weirich, D. Lisch and L. Aké Assi, Planta Med., 1992, 58, A703–A704 CrossRef.
- S. Fayez, D. Feineis, L. Aké Assi, M. Kaiser, R. Brun, S. Awale and G. Bringmann, Fitoterapia, 2018, 131, 245–259 CrossRef CAS PubMed.
- S. Fayez, A. Cacciatore, S. Sun, M. Kim, L. Aké Assi, D. Feineis, S. Awale and G. Bringmann, Bioorg. Med. Chem., 2021, 30, 115950 CrossRef CAS PubMed.
- S. Fayez, D. Feineis, L. Aké Assi, E. J. Seo, T. Efferth and G. Bringmann, RSC Adv., 2019, 9, 15738–15748 RSC.
- S. Fayez, J. Li, D. Feineis, L. Aké Assi, M. Kaiser, R. Brun, M. A. Anany, H. Wajant and G. Bringmann, J. Nat. Prod., 2019, 82, 3033–3046 CrossRef CAS.
- S. Fayez, T. Bruhn, D. Feineis, L. Aké Assi, S. Awale and G. Bringmann, J. Nat. Prod., 2020, 83, 1139–1151 CrossRef CAS PubMed.
- G. Bringmann, C. Günther, M. Ochse, O. Schupp and S. Tasler, in Progress in the Chemistry of Organic Natural Products, ed. W. Herz, H. Falk, G. W. Kirby and R. E. Moore, Springer, Wien, New York, 2001, vol. 82, pp. 111–124 Search PubMed.
- J. E. Smyth, N. M. Butler and P. A. Keller, Nat. Prod. Rep., 2015, 32, 1562–1583 RSC.
- H. K. Airy Shaw, Kew Bull., 1951, 6, 327–347 CrossRef.
- S. Porembski and W. Barthlott, in The Families and Genera of Vascular Plants. V. Flowering Plants – Dicotyledons, Malvales, Capparales
and Non-betain Caryophyllales, ed. K. Kubitzki and C. Bayer, Springer, Heidelberg, 2002, vol. 5, pp. 178–181 Search PubMed.
- G. Bringmann, G. François, L. Aké Assi and J. Schlauer, Chimia, 1998, 52, 18–28 CrossRef CAS.
- G. Bringmann, M. Rübenacker, J. R. Jansen, D. Scheutzow and L. Aké Assi, Tetrahedron Lett., 1990, 31, 639–642 CrossRef CAS.
- G. Bringmann, J. R. Jansen, H. Reuscher, M. Rübenacker, K. P. Peters and H. G. von Schnering, Tetrahedron Lett., 1990, 31, 643–646 CrossRef CAS.
- For better comparability, the atom numbering follows the one applied for normal, intact naphthylisoquinolines, as also previously used for the seco-type ancistrosecolines18 like e.g., compound 13.
- G. Bringmann, R. God and M. Schäffer, Phytochemistry, 1996, 43, 1393–1403 CrossRef CAS.
- T. Wu, Y. Wang, L. Li, J. Feng, Y. Liu, P. Ren and Y. Liu, US pat. 2017/055656, WO 2018/068017 A1 12.04.2018.
- M. Urbano, E.-K. Kim, C. Chen, Q. Li, J. Ayers, K. Nakamura, X. Zhu, H. Rosen, J. Rosenblum, M. C. Zhang, Y. Liu and M. Ueno, US pat. 2020/015683, WO 2020/160151 A1 06.08.2020.
- K. Speck and T. Magauer, Beilstein J. Org. Chem., 2013, 9, 2048–2078 CrossRef.
- M. J. Campello, L. Castedo, D. Dominguez, A. R. De Lera, J. M. Saá, R. Suau, E. Tojo and M. C. Vidal, Tetrahedron Lett., 1984, 25, 5933–5936 CrossRef CAS.
- E. Tojo, D. Dominguez and L. Castedo, Phytochemistry, 1991, 30, 1005–1010 CrossRef CAS.
- V. Kumar, Poonam, A. K. Prasad and V. S. Parmar, Nat. Prod. Rep., 2003, 20, 565–583 RSC.
- J. Michel, M. J. Ingrouille, M. S. J. Simmonds and M. Heinrich, Nat. Prod. Rep., 2014, 31, 676–693 RSC.
- G. Bringmann, M. Dreyer, H. Rischer, K. Wolf, H. A. Hadi, R. Brun, H. Meimberg and G. Heubl, J. Nat. Prod., 2004, 67, 2058–2062 CrossRef CAS.
- G. Bringmann, R. Seupel, D. Feineis, M. Xu, G. Zhang, J. Wu, M. Kaiser, R. Brun, E.-J. Seo and T. Efferth, Fitoterapia, 2017, 121, 76–85 CrossRef CAS.
- G. Bringmann, T. Ortmann, R. Zagst, B. Schöner, L. Aké Assi and C. Burschka, Phytochemistry, 1992, 31, 4015–4018 CrossRef CAS.
- G. Bringmann, C. Günther, W. Saeb, J. Mies, R. Brun and L. Aké Assi, Phytochemistry, 2000, 54, 337–346 CrossRef CAS.
- J. Li, R. Seupel, D. Feineis, V. Mudogo, M. Kaiser, R. Brun, D. Brünnert, M. Chatterjee, E.-J. Seo, T. Efferth and G. Bringmann, J. Nat. Prod., 2017, 80, 443–458 CrossRef CAS PubMed.
- J. Li, R. Seupel, T. Bruhn, D. Feineis, M. Kaiser, R. Brun, V. Mudogo, S. Awale and G. Bringmann, J. Nat. Prod., 2017, 80, 2807–2817 CrossRef CAS PubMed.
- P. P. Kushwaha, A. K. Singh, S. Prajapati, M. Shuaib, S. Fayez, G. Bringmann and S. Kumar, Toxicol. Appl. Pharmacol., 2020, 409, 115297 CrossRef CAS PubMed.
- N. Harbeck, F. Penault-Llorca, J. Cortes, M. Gnant, N. Houssami, P. Poortmans, K. Ruddy, J. Tsang and F. Cardoso, Nat. Rev. Dis. Primers, 2019, 5, 66 CrossRef PubMed.
- S. Łukasiewicz, M. Czeczelewski, A. Forma, J. Baj, R. Sitarz and A. Stanisławek, Cancers, 2021, 12, 4287 CrossRef.
- A. A. Thai, B. J. Solomon, L. V. Sequist, J. F. Gainor and R. S. Heist, Lancet, 2021, 398, 535–554 CrossRef.
- H. Lemjabbar-Alaoui, O. Hassan, Y. W. Yang and P. Buchanan, Biochim. Biophys. Acta, 2015, 1856, 189–210 CAS.
- R. L. Siegel, K. D. Miller, H. E. Fuchs and A. Jemal, Ca-Cancer J. Clin., 2022, 72, 7–33 CrossRef PubMed.
- A. G. Waks and E. P. Winer, JAMA, 2019, 32, 288–300 CrossRef PubMed.
- M. Yuan, L. L. Huang, J. H. Chen, J. Wu and Q. Xu, Signal Transduct. Target. Ther., 2019, 4, 61 CrossRef PubMed.
- W. Chanakul, P. Tuchinda, N. Anantachoke, M. Pohmakotr, P. Piyachaturawat, S. Jariyawat, K. Suksen, T. Jaipetch, N. Nuntasaen and V. Reutrakul, Fitoterapia, 2011, 82, 964–968 CrossRef CAS PubMed.
- S. Nayyatip, P. Thaichana, M. Buayairaksa, W. Tuntiwechapikul, P. Meepowpan, N. Nuntasaen and W. Pompimon, Int. J. Mol. Sci., 2012, 13, 5010–5018 CrossRef CAS PubMed.
- S. Liu and Y. C. Lin, Breast J., 2004, 10, 514–521 CrossRef CAS PubMed.
- M. J. Grimshaw, L. Cooper, K. Papazisis, J. A. Coleman, H. R. Bohnenkamp, L. Chiapero-Stanke, J. Taylor-Papdimitriou and J. M. Burchel, Breast Cancer Res., 2017, 10, R52 CrossRef.
- S. Yousefnia, K. Ghaedi, F. S. Forootan and M. H. N. Esfahani, Tumor Biol., 2019, 41, 1–14 Search PubMed.
- Y. Lombardo, A. de Giorgio, C. R. Coombes, J. Stebbing and L. Castellano, J. Visualized Exp., 2015, 97, e52671 Search PubMed.
- K. S. Prajapati, M. Shuaib, S. Gupta and S. Kumar, Mol. Carcinog., 2022, 61, 876–889 CrossRef CAS PubMed.
- M. Al-Hajj, M. S. Wicha, A. Benito-Hernandez, S. J. Morrison and M. F. Clarke, Proc. Natl. Acad. Sci. U. S. A., 2003, 100, 3983–3988 CrossRef CAS PubMed.
- C. T. Jordan, M. L. Guzman and M. Noble, N. Engl. J. Med., 2006, 355, 1253–1261 CrossRef CAS PubMed.
- A. Schulenburg, H. Ulrich-Pur, D. Thurnher, B. Erovic, S. Florian, W. R. Sperr, K. Kalhs, P. Marian, F. Wrba, C. C. Zielinkski and P. Valent, Cancer, 2006, 107, 2512–2520 CrossRef CAS.
- T. Bruhn, A. Schaumlöffel, Y. Hemberger and G. Bringmann, Chirality, 2013, 25, 243–249 CrossRef CAS.
- T. Bruhn, A. Schaumlöffel, Y. Hemberger and G. Pescitelli, SpecDis, Version 1.71, https://www.specdis-software.jimdo.com, Berlin, Germany, 2017 Search PubMed.
- S. Grimme, J. Antony, S. Ehrlich and H. Krieg, J. Chem. Phys., 2010, 132, 154104–154119 CrossRef PubMed.
- F. Weigend and R. Ahlrichs, Phys. Chem. Chem. Phys., 2005, 7, 3297–3305 RSC.
- F. Weigend, Phys. Chem. Chem. Phys., 2006, 8, 1057–1065 RSC.
- U. Ekström, L. Visscher, R. Bast, A. J. Thorvaldsen and K. Ruud, J. Chem. Theory Comput., 2010, 6, 1971–1980 CrossRef PubMed.
- Y. S. Lin, G. D. Li, S. P. Mao and J. D. Chai, J. Chem. Theory Comput., 2013, 9, 263–272 CrossRef CAS.
- F. Neese, Wiley Interdiscip. Rev.: Comput. Mol. Sci., 2018, 8, e1327 Search PubMed.
- P. P. Kushwaha, P. S. Vardhan, P. Kapewangolo, M. Shuaib, S. K. Prajapati, A. K. Singh and S. Kumar, Life Sci., 2019, 234, 116783 CrossRef CAS PubMed.
- Y. Santiago-Vázquez, U. Das, A. Varela-Ramirez, S. T. Baca, Y. Ayala-Marin, C. Lema, S. Das, A. Baryyan, J. R. Dimmock and R. J. Aguilera, Clin. Cancer Drugs, 2016, 3, 138–146 CrossRef PubMed.
- P. P. Kushwaha, A. K. Singh, M. Shuaib, K. S. Prajapati, P. S. Vardhan, S. Gupta and S. Kumar, Chem.-Biol. Interact., 2020, 328, 109200 CrossRef CAS PubMed.
Footnotes |
† Deceased on January 14, 2014. |
‡ Dedicated to Professor Günther Heubl, Ludwigs-Maximilians-Universität München (LMU Munich) on the occasion of this 70th birthday. |
§ Electronic supplementary information (ESI) available: Spectroscopic data include NMR (1H, 13C, 1H, 1H–COSY, HSQC, HMBC, NOESY, and ROESY), HRESIMS, IR, and ECD spectra of compounds 14–17 and GC-MSD chromatograms obtained from the oxidative degradation of 14–17. See DOI: https://doi.org/10.1039/d2ra05758a |
|
This journal is © The Royal Society of Chemistry 2022 |
Click here to see how this site uses Cookies. View our privacy policy here.