DOI:
10.1039/D2RA04937F
(Paper)
RSC Adv., 2022,
12, 28043-28051
Low-generation fluorescent polyurethane dendrimers via late-stage modification using azide–alkyne click chemistry†
Received
7th August 2022
, Accepted 22nd September 2022
First published on 3rd October 2022
Abstract
Protecting group free one-pot multicomponent Curtius reaction afforded a versatile MN2 type dendron, ensuring late-stage modification of both dendron and dendrimer to afford highly fluorescent symmetrical and unsymmetrical (Janus) polyurethane dendrimers. Fluorescence study of these dendrimers exhibited the Förster resonance energy transfer (FRET) between blue and mint green fluorophores.
Introduction
Fluorescent macromolecules have made an immense contribution to modern technology. In this context, fluorescent dendrimers are more compelling than the linear analogues (polymeric structures) owing to their inherently branched topological features.1–4 A dendrimer has its core encompassed by numerous branched dendrons preventing self-aggregation and concentration-quenching in the solid-state. Moreover, dendrimers possess excellent wet processibility in organic solvents compared to polymers.5–7 Polymeric materials suffer from wide molecular weight distribution and terminal defects, whereas dendritic macromolecules have a well-defined architecture and precise molecular weight. For this reason, photoactive dendrimers can be utilized as light-harvesting materials in organic light-emitting diodes (OLEDs).8,9 Changes in the photophysical properties of fluorescent dendritic molecules, on the other hand, can be exploited for sensing applications.10,11 Additionally, luminescence signals can be traced to obtain useful information during drug delivery12 and biological imaging.13,14
Late-stage modification (LSM) or post-synthetic modification is a powerful synthetic approach for synthesizing desired molecules, including dendrimers.15–17 This approach not only facilitates the formation of target materials that cannot be synthesized directly but also accelerates their formation. Click reactions are a useful tool in chemistry to afford both small and large molecules efficiently. In particular, copper-catalysed azide–alkyne cycloaddition (CuAAC),18,19 due to its versatility and biocompatibility, has been widely employed in the design, synthesis, and/or LSM of fluorescent dendrimers containing different types of linkages.20–27 However, the use of azide–alkyne click reaction in the synthesis and LSM of polyurethane dendrimers (PUDs)28,29 has not been reported to the best of our knowledge. Previously, we reported a model for LSM of PUDs using thiol–ene click reaction.30 Herein, we report the synthesis and LSM of generation-one fluorescent PUDs employing CuAAC.
Results and discussion
Synthesis of fluorescent dendrimers
Synthesis of dendron 3 was performed by adopting the method established previously.30 Briefly, the diisocyanate formed in situ from 1 employing one-pot multicomponent Curtius reaction was trapped by a nucleophile, 4-pentyn-1-ol to afford 2 (Scheme S1†). Subsequent SN2 attachment of a spacer group, 11-bromoundecanol, furnished 3 in an excellent yield (88%, Scheme 1).
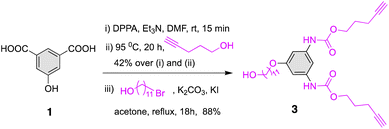 |
| Scheme 1 Synthesis of dendron 3 using one-pot multicomponent Curtius reaction followed by the spacer group attachment. | |
We used previously reported coumarin azides in this study.31 As shown in Scheme 2, compound 3 underwent CuAAC reaction with azidocoumarin 4 smoothly to afford highly fluorescent dendron 6 in an excellent yield (93%). The advantage of this reaction is that the product could be precipitated easily in water, or the crude can be passed through a small plug of silica rapidly to furnish pure product. The product can be easily tracked using UV light during chromatographic purification (Fig. S1†). In contrast to azidocoumarin 4, the azidocoumarin 5 gave a mixture of products containing one-clicked and two-clicked mint-green fluorescent products (7 and 8, Scheme 2). The reaction with 5 was extremely slow and did not form 8 completely even after three days of reaction.
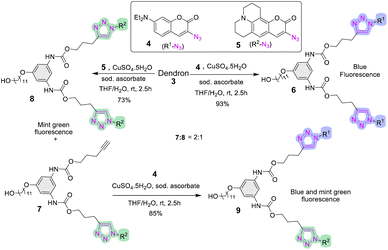 |
| Scheme 2 LSM of 3 using CuAAC to form fluorescent dendrons 6, 7, 8, and 9. | |
We investigated the Cu(I) stabilizing ligand tris(3-hydroxypropyltriazlylmethyl) amine (THPTA)32 for the complete formation of 8, but it did not improve the reaction significantly. The concomitantly formed product 7 was carried to the subsequent CuAAC reaction using coumarin 4 to synthesize the mixed labelled dendron 9 (Scheme 2).
With the fluorescent dendrons in hand, we commenced the synthesis of PUDs by attaching the focal point (–OH) of dendron to the difunctional core, hexamethylene diisocyanate (HMDI) 3a. Utilizing dibutyltin dilaurate (DBTDL) as a catalyst,33,34 9 was allowed to attach to 3a under stirring at room temperature to generate dendrimer 13 (Scheme 3a). This reaction was very sluggish. We reasoned that the Sn-catalyst (DBTDL) was trapped by a coordination sphere created by 1,2,3-triazole and the carbonyl group of coumarin35,36 leaving no catalyst available to coordinate with isocyanate. The addition of 4.2 eq. of DBTDL (assigning 1 eq. of catalyst to each triazole) to the reaction smoothly afforded alternating blue and mint-green fluorescent dendrimer 13 in 83% yield under similar reaction conditions. The excess DBTDL can be easily removed during workup by washing multiple times with saturated NaHCO3 or via flash chromatography before eluting the product.
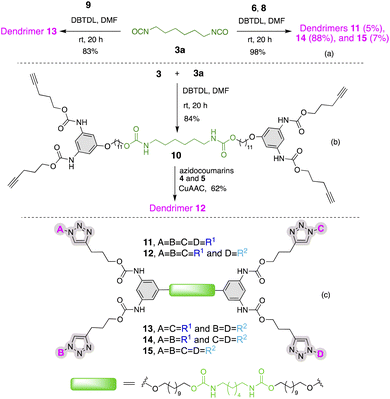 |
| Scheme 3 Synthesis of blue and mint green fluorescent PUDs by attachment of dendrons to the core (a) and by attachment followed to the core by click (b). (c) Structures of PUDs 11–15 with different fluorophore topologies. | |
To further synthesize PUDs with different structural features, core 3a was reacted with blue (6) and mint-green (8) fluorescent dendrons to afford a mixture of PUDs 11, 14, and 15 (Scheme 3a) in an excellent yield (98%). Surprisingly, 88% of the product mixture was the unsymmetrical dendrimer 14 (block-blue and mint green), contrary to statistical distribution.
In order to synthesize the fluorescent architectures with different structural features, we attached non-fluorescent dendron 3 to the core 3a to render a dendrimer 10 with four pentyne arms in 84% yield (Scheme 3b). The reaction preceded smoothly using 0.2 eq. of DBTDL catalyst under room temperature. Huisgen 1,3-dipolar cycloaddition of 10 was performed at its four-terminal positions carrying out CuAAC with azidocoumarins 4 and 5 (2.1 eq. each). The dendrimer 12 with three blue and one mint green fluorophore was obtained as 87% of the product. This agreed with the previously observed slow reactivity of 5 towards CuAAC. Although the dendrimers with fluorescence combinations – all blue (11) and two blue plus two mint green (13 or 14) were produced in negligible quantity, dendrimer 15 was not formed at all. Moreover, 13 and 14 were obtained as inseparable isomers. Scheme 3c shows five different types of fluorescent PUDs with varying topological features synthesized in this work.
Characterization of fluorescent dendrimers
The clicked fluorescent dendrimers were first characterized by homonuclear one-dimensional (1H and 13C) NMR spectroscopy and further investigated and assigned using two-dimensional homonuclear (COSY) and heteronuclear (HSQC) NMR spectroscopy. As depicted in Fig. 1a (and S21–S34†), the urethane NH peaks were observed at ∼7.23 ppm and ∼4.55 ppm for aromatic and aliphatic regions, respectively. The NH peak at ∼4.55 ppm confirmed the successful attachment of dendrons 6 and 8 to the core 3a. The urethane methylene peaks (NHCOOCH2) in the vicinity of the benzene ring were spotted more deshielded (∼4.25 ppm) than the aliphatic counterpart (∼3.23 ppm). The most downfield chemical shift at ∼8.32 ppm and a triplet at ∼2.94 ppm further evidenced the successful CuAAC reaction leading to the formation of these dendritic structures.
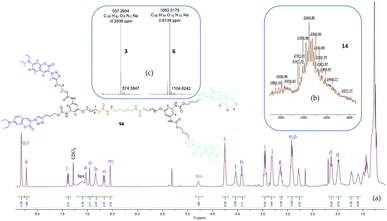 |
| Fig. 1 (a) A portion of 1H NMR spectrum (400 MHz, CDCl3, 298 K) of 14, (b) MALDI-TOF-MS spectrum (CCA matrix) of 14 showing [M + Na]+ peak, and (c) HRMS of nonfluorescent dendron 3 and blue fluorescent dendron 6. | |
Mass spectrometric investigation of both fluorescent and non-fluorescent small molecules was performed by high-resolution electrospray ionization mass spectrometry (HRESI-MS) using linear trap quadrupole (LTQ) orbitrap as shown in Fig. S35–S40.† Fluorescent PUDs (11–15), on the other hand, were studied by matrix-assisted laser desorption/ionization time-of-flight mass spectrometry (MALDI-TOF-MS) (Fig. 1b and S42–45†). In MALDI-TOF-MS spectrum, the peak of m/z = 2302.84 detected was consistent with theoretical mass of [M + Na]+ ion of dendrimer 14 (m/z = 2300.13) (Fig. 1b). Moreover, HRMS peaks of m/z = 537.2934 and 1053.5175 detected in LTQ-Orbitrap (Fig. 1c) corresponded to the [M + Na]+ ions of compound 3 and 6 respectively (theoretical m/z = 537.2941 and 1053.5169 respectively).
Photophysical properties
The photophysical properties of blue and mint-green fluorescent dendrimers 11–15 were first examined by UV-vis and fluorescence spectroscopy (Table 1; more details in ESI Fig. S46†). Fig. 2 visualizes the fluorescence of the dendrimers. As expected, blue fluorescent dendrimer 11 absorbed the shortest wavelength (414 nm), whereas mint-green fluorescent dendrimer 15 absorbed the longest wavelength (431 nm). Also, the blue and mint-green fluorescent dendrimers 13 (alternating) and 14 (block) exhibited identical absorption owing to their identical fluorophore entities. Fluorescence emission spectra of these urethane dendrimers were recorded in 50 μM DCM solution. Ongoing from completely blue fluorescent dendrimer 11 to completely mint-green fluorescent dendrimer 15, a bathochromic shift of 21 nm was observed. Surprisingly, the emission from 13 was almost identical to 15. Moreover, replacing one of the four blue coumarins with a mint green (12) resulted in identical fluorescence emission to that of 14.
Table 1 UV absorption and fluorescence emission wavelengths of PUDs 11–15
Dendrimers |
Absorption (nm) |
Emission (nm) |
11 (blue only) |
414 |
475 |
12 (3 blue, 1 mint green) |
415 |
489 |
13 (2 blue, 2 mint green, alternate) |
421 |
492 |
14 (2 blue, 2 mint green, block) |
418 |
488 |
15 (mint green only) |
431 |
492 |
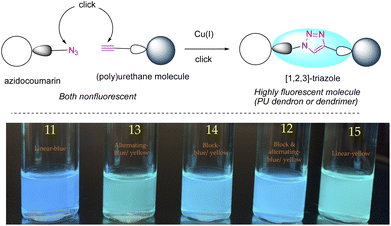 |
| Fig. 2 Schematic representation showing CuAAC of an azidocoumarin and a terminal alkyne-containing-PU forming a highly fluorescent molecule (top). Fluorescence observed in PUDs (50 μM solution in DCM) when illuminated under UV light (365 nm) (bottom). | |
The fluorescence behaviour observed in compounds 12 and 13 can be explained based on FRET (Förster or fluorescence resonance energy). The FRET process involves the transfer of energy (via virtual photon) from one fluorophore (donor) to a second fluorophore (acceptor) through nonradiative transfer of energy when the distance between them is less than 10 nm.37 The schematic representation of FRET is shown in Fig. 3(b) and (c) as a function of the distance between two fluorophores. The FRET efficiency is very sensitive to the distance between the fluorophores, as it is inversely proportional to the sixth power of that distance.38 This phenomenon is observed when one of the four blue fluorophores were replaced by a mint green fluorophore in compound 12, yielding fluorescence emission at a higher wavelength close to that of 14 (Fig. 3d). This is attributed to the distance between the blue and mint green fluorophores enough for transferring the radiationless energy from blue to the mint green fluorophore. Moreover, the FRET observation in a compound containing alternate blue and mint green fluorophores (13) is significantly higher than the one having block blue and mint green fluorophores (14). Clearly the distance between blue and mint green fluorophores in 13 is much less than their distance in 14 (Fig. 3a). The FRET process in 13 is so high that its fluorescence emission is similar to that of the dendrimer containing all mint green fluorophores (15).
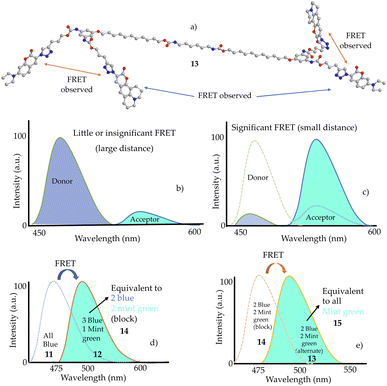 |
| Fig. 3 (a) Ball and stick 3D model of dendrimer 13 (hydrogens removed for simplicity) showing FRET between two fluorophores. Schematic representation of fluorescence resonance energy transfer (FRET) between a donor (blue) and an acceptor (mint green fluorophore) showing (b) little or insignificant FRET and (c) significant FRET as a function of distance between two fluorophores. (d) FRET observed in dendrimer containing 3 blue and 1 mint green fluorophores (12) compared to the dendrimer having blue fluorophores only.11 (e) FRET observed in dendrimer with alternate blue and mint green fluorophores (13) compared with the dendrimer with block blue and mint green fluorophores (14). The spectra show the fluorescence emissions only. | |
For efficient FRET to occur, there should be an overlap between the donor emission spectra and the acceptor absorption spectra. As shown in Fig. S46,† the emission of blue fluorescent dendrimer was observed at ∼430–600 nm, whereas the absorption of mint-green fluorescent dendrimer was found at ∼350–480 nm showing a substantial overlap of spectra in the 430–480 nm region. This caused the FRET process from blue fluorophore (donor) to mint-green fluorophore (acceptor).
Conclusions
In conclusion, we demonstrated an easy and efficient method for the synthesis of fluorescent polyurethane dendrimers employing a one-pot multicomponent Curtius reaction followed by late-stage modification using azide–alkyne click chemistry. Copper-catalysed azide–alkyne click reaction of a 3-azidocoumarin with a urethane containing terminal alkyne gave a fluorescent dendron, which underwent attachment to the difunctional core affording a well-defined dendrimer. The fluorescence properties can be tailored easily by installing various coumarins in the periphery via late-stage modification. The presence of coumarins offers tunable absorbance and fluorescence spectra. These properties can be exploited to furnish tailored macromolecular structures of interesting and versatile properties. Applications of these techniques to higher generations are underway and will be reported when complete.
Experimental section
General information
Starting materials were used as obtained from commercial sources: Sigma Aldrich (NaN3, AIBN, dibutyltin dilaurate (DBTDL), triethylamine, hexamethylene-1,6-diisocyanate), TCI (4-pentyn-1-ol, 1-bromoundecanol), and Alfa-Aesar (5-hydroxyisophthalic acid, DPPA). Whereas anhydrous solvents were used in the dendrimer synthesis, DMF (Acros Organics), DCM (Fischer Scientific), and acetone (Acros Organics) were used as received and toluene was used without distillation. Curtius reaction was set in a Carousel reactor and all other reactions were performed using classical batch process using oil bath (if heat needed). Melting points were determined using Thermo Scientific MelTemp 3.0 instrument.
1H, 13C, and 2D NMR spectra were recorded with a Bruker Advance 500 MHz NMR instrument at 298 K. NMR spectra were recorded using either acetone-d6 or CDCl3 as deuterated solvent and accordingly the solvent residual peaks were obtained at δ 2.05 ppm (qn) and δ 7.26 ppm (s) respectively in 1H NMR. In 13C NMR, solvent residual peaks were recorded at δ 206.68 ppm (s) and δ 29.92 ppm (septet) for acetone-d6 and δ 77.23 ppm (s) for CDCl3. Coupling constants (J) are given in hertz (Hz) whereas chemical shifts are given in δ scale (ppm). Moreover, the multiplicities are indicated as – s (singlet), d (doublet), t (triplet), q (quartet), qn (quintet), or m (multiplet).
HRMS spectra of small molecules including dendrons were obtained from ESI-LTQ-Orbitrap. MALDI of larger molecules were recorded with a Bruker Autoflex 3 instrument using α–cyano-4-hydroxycinnamic acid (CCA) as matrix in positive ion mode.
Purification of compounds were carried out using flash chromatography with irregular silica of 40–60 μm, 60 Å. Small scale purification was achieved using auto-column flash cartridges packed with 12 g or 40 g silica of 40–75 μm, 60 Å (obtained from Sorbtech and Supelco Technologies). Flow rate was 10–30 mL min−1. Mobile phase used in these separations was ethyl acetate, hexane, DCM or mixture of these solvents.
Synthesis of dendron 3
An oven-dried Carousel flask (250 mL) was charged with 5-hydroxyisophthalic acid 1 (5.0 g, 27.45 mmol, 1.0 eq.) and a magnetic stir bar. After flushing the air and backfilling with nitrogen, dry DMF (20 mL) was added to the flask to dissolve the compound. To this solution, triethylamine (7.7 mL, 54.90 mmol, 2.0 eq.) was added under stirring followed by slow addition of DPPA (12.5 mL, 57.65 mmol, 2.1 eq.). The solution was then stirred for 15 min at rt to ensure complete formation of acyl azide. Addition of DPPA is an exothermic reaction and turns the solution yellow. To this homogenous yellow solution, was added 4-pentyn-1-ol (5.1 mL, 54.90 mmol, 2.0 eq.) and heated to 95 °C for 20 h. The solution was then cooled to rt, diluted 20 times with water, extracted with EtOAc (4 × 100 mL), washed the combined organic layers multiple times with water, washed with brine (100 mL), dried over anhydrous MgSO4, filtered, and evaporated under reduced pressure. The crude was purified by flash chromatography using (3
:
2 hexane/EtOAc) to afford slightly pink solid as the product 2 (3.11 g, 9.0 mmol, 42%, Scheme S1†); mp 85–88 °C. TLC (40% EtOAc in hexane): Rf 0.37; 1H NMR (500 MHz, CD3COCD3): δ 8.57 (s, 2H, –NH–), 8.29 (s, 1H, –OH), 7.19 (t, J = 1.7 Hz, 1H), 6.90 (d, 2H), 4.20 (t, J = 6.3 Hz, 4H), 2.39 (t, J = 6.3 Hz, 2H), 2.31 (dt, J = 7.1, 2.6 Hz, 4H), 1.89–1.83 (m, 4H); 13C NMR (126 MHz, CD3COCD3): δ 158.1, 153.4, 140.6, 100.1, 99.9, 83.0, 69.5, 62.9, 27.9, 14.6; HRMS (ESI-LTQ-Orbitrap) (m/z): [M + Na]+ calcd for C18H20N2O5Na 367.1264; found 367.1266. (Caution: on heating, Curtius rearrangement proceeds immediately with a rapid evolution of N2 gas. At this point, the reaction vessel should not be sealed completely to prevent possible explosion).
An oven-dried RB flask (250 mL) equipped with a magnetic stir bar was charged with phenolic diurethane 2 (2.74 g, 7.95 mmol, 1.0 eq.), 11-bromoundecanol (3.00 g, 11.92 mmol, 1.5 eq.), K2CO3 (5.49 g, 39.74 mmol, 5.0 eq.), and KI (0.40 g, 2.38 mmol, 0.3 eq.). After flushing and backfilling the flask with N2, dry acetone (35 mL) was added, and the reaction mixture was refluxed for 20 h. When the reactant 2 was consumed completely (checked by TLC), the reaction was stopped, cooled to rt, and solvent was evaporated under reduced pressure. The residue was suspended in EtOAc/water mixture (200 mL, 1
:
1), organic layer was separated, aqueous layer was extracted with EtOAc (2 × 100 mL), combined organic layers washed with brine (90 mL), dried with anhydrous MgSO4, filtered, and evaporated under reduced pressure. The crude was purified by flash chromatography (3
:
2 hexane/EtOAc as mobile phase) to afford transparent and highly viscous oil as the dendron 3 (3.60 g, 6.99 mmol, 88%). TLC (40% EtOAc in hexane): Rf 0.31; 1H NMR (500 MHz, CD3COCD3): δ 8.63 (s, 2H, –NH–), 7.28 (s, 1H), 6.98 (s, 2H), 4.21 (t, J = 6.3 Hz, 4H), 3.95 (t, J = 6.5 Hz, 2H), 3.54 (q, J = 18.4, 5.2 Hz, 2H), 3.41 (t, J = 5.2 Hz, 1H, OH), 2.39 (t, J = 2.6 Hz, 2H), 2.32 (dt, J = 7.1, 2.6 Hz, 4H), 1.89–1.84 (m, 4H), 1.80–1.75 (m 2H), 1.53–1.46 (m, 4H), 1.44–1.33 (m, 12H); 13C NMR (126 MHz, CD3COCD3): δ 160.1, 153.3, 140.5, 100.7, 99.1, 82.9, 69.5, 67.5, 62.9, 61.6, 59.6, 54.5, 32.9, 27.9, 25.8, 25.8, 14.5; HRMS (ESI-LTQ-Orbitrap) (m/z): [M + Na]+ calcd for C29H42N2O6Na 537.2935; found 537.2934.
Synthesis of azidocoumarins
3-Azido-7-diethylaminocoumarin 4. Blue-fluorescent precursor 4 was synthesized from previously reported procedure.31 Briefly, the reaction mixture containing 4-diethylamino salicylaldehyde (2.86 g, 14.4 mmol, 1.0 eq.), ethyl nitroacetate (1.70 mL, 14.4 mmol, 1.0 eq.), piperidine (200 μL), acetic acid (400 μL), molecular sieves (200 mg), and 1-butanol (50 mL) was refluxed for 20 h. A bright yellow solid (glossy orange, needle shaped crystals) formed on cooling was collected by filtration, which was then redissolved in 30 mL of DMF at 80 °C and filtered to remove molecular sieves. The filtrate was poured into 200 mL of ice-cold water to obtain bright yellow solid 4a (2.22 g, 57%) (Scheme S2†).Without further purification, compound 4a (2.10 g, 7.98 mmol, 1 eq.) was dissolved in a solution of HCl (38 mL, 37.4%) and SnCl2 (12.2 g, 64.12 mmol, 9.0 eq.) slowly over 45 min under stirring at rt. Each portion was allowed to dissolve completely before adding the next portion. Stirring was continued for 4 h before pouring the solution into 152 g of ice. The solution was then made alkaline by adding 200 mL of 5 M NaOH at 15 °C. The granular yellow solid 4b formed was collected by filtration (1.40 g, 76%) (Scheme S2†).
Without further purification, compound 4b (1.20 g, 5.2 mmol, 1.0 eq.) was added in small portions at a time to HCl (50 mL, 17.2%) at rt under stirring. After cooling the solution in an ice bath, NaNO2 (373.0 mg, 5.2 mmol, 1.0 eq. in 10 mL water) was added dropwise and the stirring was continued for 20 min at the same temperature before adding NaOAc (48.0 g in 110 mL water) to bring the pH to 4.0. Then NaN3 was added in portions and the stirring was continued for an hour. The precipitated product was filtered, washed with ice-cold water, and dried under vacuum to afford a yellow solid 4 as the product (1.16 g, 87%). Spectra of the compounds were similar to those reported previously.
9-Azido-2,3,5,6-tetrahydro-1H,4H-11-oxa-3a-aza-benzo[de]anthracene-10-one 5. The first two steps were performed similar to above procedure. Compound 5b was converted to azidocoumarin 5 using previously reported procedure.3 In brief, aminocoumarin 5b (736.9 mg, 2.88 mmol, 1.0 eq.) was dissolved in portions to the stirred solution of p-TsOH·H2O (4.66 g, 25.88 mmol, 9.0 eq.) in water (40 mL). After stirring the solution for 15 min at rt, NaNO2 was added in portions followed by slow addition NaN3 (373.8 mg, 5.75 mmol, 2.0 eq.). The solution was then stirred for 30 min at rt. The precipitated solid was filtered, washed with water, and dried in vacuum to afford greenish yellow solid as azidocoumarin 5 (590.7 mg, 73%). Spectra were similar to those reported the literature previously.
General method of copper catalysed azide–alkyne cycloaddition (CuAAC)
Compound with terminal alkyne (1 eq.) was dissolved in THF in an RB flask to which azidocoumarin (1.2 eq./triple bond) was added. After adding an aqueous solution of CuSO4·5H2O (0.15 eq./N3) and sodium ascorbate (0.30 eq./N3) in minimum amount of water to the flask, the reaction mixture stirred vigorously at rt under dark. When the alkyne was completely consumed, the reaction mixture was diluted and extracted with DCM, combined organic layers were dried over anhydrous MgSO4, and the solvent was evaporated under reduced pressure. The crude was purified by flash chromatography.
Blue fluorescing dendron 6
General method of azide–alkyne click reaction was employed using dendron 3 (112.5 mg, 0.217 mmol, 1.0 eq.), azidocoumarin 4 (134.5 mg, 0.521 mmol, 2.4 eq.), CuSO4·5H2O (10.4 mg, 0.065 mmol, 0.3 eq.), sodium ascorbate (25.8 mg, 0.130 mmol, 0.6 eq.), THF (2 mL), and water (200 μL) to afford blue fluorescing yellow solid 6 (202.5 mg, 93%) on flash chromatography (40–70% EtOAc in hexane). Mp. 103–106 °C.
TLC (70% EtOAc in hexane): Rf 0.12; 1H NMR (500 MHz, CD3COCD3): δ 8.63 (s, 2H), 8.33 (d, J = 8.4 Hz, 4H), 7.29 (t, J = 1.9 Hz, 1H), 6.98 (s, 2H), 6.85 (dd, J = 8.9, 2.5 Hz, 2H), 6.63 (d, J = 2.5 Hz, 2H), 4.24 (t, J = 6.3 Hz, 4H), 3.94 (t, J = 6.5 Hz, 2H), 3.56 (dq, J = 12.5, 6.8 Hz, 10H), 3.46–3.34 (m, 1H), 2.91 (t, J = 7.5 Hz, 4H), 2.12 (d, J = 7.2 Hz, 4H), 1.82–1.70 (m, 2H), 1.49 (ddd, J = 15.2, 10.7, 7.1 Hz, 4H), 1.32 (s, 12H), 1.25 (t, J = 7.1 Hz, 12H); 13C NMR (126 MHz, CD3COCD3): δ 160.1, 156.7, 155.9, 153.5, 151.7, 146.5, 140.6, 135.0, 130.2, 122.2, 117.3, 109.9, 106.9, 99.1, 96.6, 67.5, 63.7, 61.6, 59.65, 44.5, 32.9, 25.9, 25.8, 21.9, 19.9, 13.6, 11.8; HRMS (ESI-LTQ-Orbitrap) (m/z): [M + Na]+ calcd for C55H70N10O10Na 1053.5169; found 1053.5175.
Mint-green fluorescing dendrons 7 and 8
General method of azide–alkyne click reaction was employed using dendron 3 (100 mg, 0.194 mmol, 1.0 eq.), azidocoumarin 5 (131.9 mg, 0.466 mmol, 2.4 eq.), CuSO4·5H2O (9.3 mg, 0.058 mmol, 0.3 eq.), sodium ascorbate (23.1 mg, 0.117 mmol, 0.6 eq.), THF (2 mL), and water (200 μL) to afford yellow fluorescing orange solid 7 (106.2 mg) and 8 (46.5 mg, 73% overall) on flash chromatography (40–70% EtOAc in hexane).
Dendron 7. Mp. 100–102 °C; TLC (70% EtOAc in hexane): Rf 0.62; 1H NMR (500 MHz, CD3COCD3): δ 8.63 (s, 2H), 8.31 (s, 1H), 8.20 (s, 1H), 7.28 (t, J = 1.9 Hz, 1H), 7.14 (s, 1H), 7.02–6.92 (m, 2H), 4.22 (dt, J = 14.8, 6.3 Hz, 4H), 3.94 (t, J = 6.5 Hz, 2H), 3.54 (td, J = 6.6, 5.0 Hz, 2H), 3.41 (t, J = 5.2 Hz, 1H), 3.37 (td, J = 5.9, 2.1 Hz, 4H), 2.94–2.74 (m, 8H), 2.40 (t, J = 2.7 Hz, 1H), 2.33 (td, J = 7.1, 2.7 Hz, 2H), 2.15–2.10 (m, 2H), 2.02–1.94 (m, 4H), 1.87 (p, J = 6.7 Hz, 2H), 1.81–1.72 (m, 2H), 1.56–1.43 (m, 4H), 1.39–1.30 (m, 12H); 13C NMR (126 MHz, CD3COCD3): δ 171.2, 160.3, 157.4, 154.3, 153.5, 153.4, 150.8, 149.1, 146.9, 146.7, 139.6, 139.4, 135.0, 125.9, 122.1, 119.8, 116.1, 107.0, 106.2, 100.9, 99.9, 83.1, 69.1, 68.0, 64.5, 63.6, 62.9, 60.4, 50.1, 49.6, 39.1, 32.8, 29.7, 29.5, 29.4, 29.4, 29.2, 29.1, 28.4, 27.8, 27.5, 25.9, 25.7, 22.4, 21.2, 21.0, 20.3, 20.2, 15.1, 14.2; HRMS (ESI-LTQ-Orbitrap) (m/z): [M + Na]+ calcd for C44H56N6O8Na 819.4052; found 819.4060.
Dendron 8. Mp. 122–124 °C; TLC (70% EtOAc in hexane): Rf 0.18; 1H NMR (400 MHz, CDCl3): δ 9.57 (s, 2H), 8.28 (d, J = 14.7 Hz, 4H), 7.21 (d, J = 1.9 Hz, 1H), 7.15 (s, 2H), 6.82–6.70 (m, 2H), 4.14 (t, J = 6.4 Hz, 4H), 3.83 (t, J = 6.5 Hz, 2H), 3.38 (d, J = 6.4 Hz, 2H), 3.29 (d, J = 5.9 Hz, 8H), 3.12 (s, 1H), 2.90–2.65 (m, 12H), 2.03 (q, J = 7.2 Hz, 4H), 1.95–1.81 (m, 8H), 1.67 (t, J = 7.2 Hz, 2H), 1.41–1.21 (m, 16H); 13C NMR (101 MHz, CDCl3): δ 162.6, 160.3, 157.3, 156.9, 153.4, 150.8, 146.9, 146.7, 139.5, 134.9, 125.9, 122.1, 119.7, 116.1, 107.0, 106.2, 99.9, 69.5, 67.9, 64.9, 64.5, 53.82, 50.1, 49.6, 40.7, 36.5, 31.9, 31.7, 31.4, 29.9, 29.7, 29.6, 29.4, 29.4, 29.33, 29.30, 29.28, 29.26, 29.23, 29.06, 28.5, 27.5, 26.2, 25.9, 25.87 22.7, 22.5, 21.2, 20.3, 20.2, 14.1; HRMS (ESI-LTQ-Orbitrap) (m/z): [M + Na]+ calcd for C59H70N10O10Na 1101.5169; found 1101.5173.
Blue and mint-green fluorescing dendron 9
General method of azide–alkyne click reaction was employed using 7 (129.0 mg, 0.162 mmol, 1.0 eq.), azidocoumarin 4 (50.2 mg, 0.194 mmol, 1.2 eq.), CuSO4·5H2O (4.0 mg, 0.0243 mmol, 0.15 eq.), sodium ascorbate (10.0 mg, 0.0486 mmol, 0.3 eq.), THF (2 mL), and water (200 μL) to afford blue fluorescing yellow solid 9 (124.0 mg, 73%) on flash chromatography (90% EtOAc in hexane). Mp. 121–125 °C; TLC (70% EtOAc in hexane): Rf 0.18; 1H NMR (400 MHz, CD3SOCD3): δ 9.57 (s, 2H), 8.41 (s, 1H), 8.34–8.24 (m, 3H), 7.61 (d, J = 8.9 Hz, 1H), 7.21 (d, J = 1.9 Hz, 1H), 7.18 (s, 1H), 6.82 (dd, J = 9.0, 2.4 Hz, 1H), 6.77 (d, J = 1.8 Hz, 2H), 6.66 (d, J = 2.4 Hz, 1H), 4.14 (t, J = 6.4 Hz, 4H), 3.84 (t, J = 6.5 Hz, 2H), 3.48 (q, J = 7.0 Hz, 4H), 3.37 (dd, J = 6.5, 5.3 Hz, 2H), 2.90–2.67 (m, 8H), 2.03 (q, J = 6.9 Hz, 4H), 1.89 (dq, J = 12.5, 6.0 Hz, 4H), 1.67 (p, J = 6.7 Hz, 2H), 1.42–1.24 (m, 16H), 1.15 (t, J = 6.0 Hz, 6H); 13C NMR (101 MHz, CD3SOCD3): δ 170.8, 159.6, 157.3, 157.25, 156.0, 153.8, 151.8, 150.9, 147.2, 146.5, 146.5, 140.8, 137.2, 137.0, 130.9, 126.6, 123.4, 123.3, 119.7, 116.8, 115.92, 110.4, 106.9, 106.7, 105.7, 99.4, 96.8, 67.7, 63.9, 61.2, 60.2, 49.8, 49.3, 44.6, 33.0, 29.5, 29.5, 29.4, 29.4, 29.2, 29.1, 28.7, 27.3, 25.9, 22.0, 21.9, 21.2, 21.1, 20.2, 14.5, 12.7; HRMS (ESI-LTQ-Orbitrap) (m/z): [M + Na]+ calcd for C57H70N10O10Na 1077.5169; found 1077.5165.
General method of attachment of dendrons to the core
An oven dried RB flask equipped with a magnetic stir bar was charged with dendrons (fluorescent or non-fluorescent). After flushing and backfilling with N2, dry DMF was added to the flask via syringe. Then hexamethylene-1,6-diisocyanate and dibutyltin dilaurate (DBTDL) were added successively. The reaction mixture was stirred vigorously at rt for 2–20 h before diluting and extracting with DCM. The combined organic layers were washed multiple times with water to get rid of DMF, washed with brine, dried over anhydrous MgSO4, filtered, and evaporated under educed pressure. The crude was then purified with flash chromatography.
Dendrimer with alkyne periphery 10
General method of dendron attachment was performed using dendron 3 (133.7 mg, 0.260 mmol, 2.1 eq.), hexamethylene diisocyanate (20 μL, 0.124 mmol, 1.0 eq.), DBTDL (22 μL, 0.037 mmol, 0.3 eq.), and DMF (1 mL) to afford white solid as the product 10 (124.0 mg, 84%) on flash chromatography (40% EtOAc in hexane). Mp. 83–86 °C; TLC (40% EtOAc in hexane): Rf 0.13; 1H NMR (400 MHz, CDCl3): δ 6.97 (d, J = 2.3 Hz, 2H), 6.80 (s, 4H), 6.75–6.58 (m, 4H), 4.65 (d, J = 6.3 Hz, 2H), 4.19 (t, J = 6.3 Hz, 8H), 3.97 (t, J = 6.7 Hz, 4H), 3.85 (t, J = 6.5 Hz, 4H), 3.16–2.98 (m, 4H), 2.24 (td, J = 7.0, 2.7 Hz, 8H), 1.92 (t, J = 2.7 Hz, 4H), 1.82 (p, J = 6.7 Hz, 8H), 1.66 (dd, J = 8.2, 6.3 Hz, 4H), 1.52 (t, J = 6.9 Hz, 4H), 1.43–1.20 (m, 34H); 13C NMR (101 MHz, CDCl3): δ 162.5, 160.3, 156.5, 153.3, 139.4, 100.9, 100.0, 83.0, 69.2, 69.1, 68.0, 64.9, 63.7, 60.4, 40.7, 36.5, 31.4, 29.9, 29.7, 29.4, 29.4, 29.28, 29.23, 29.1, 29.0, 27.8, 26.2, 25.9, 25.8, 21.0, 15.1, 14.2; MALDI-TOF-MS (CCA matrix) (m/z): [M + Na]+ calcd for C66H96N6O14Na 1219.69; found 1219.68.
Blue and mint-green fluorescing (alternate) dendrimer 13
General method of dendron attachment was performed using dendron 9 (92.6 mg, 0.088 mmol, 2.1 eq.), hexamethylene diisocyanate (7 μL, 0.042 mmol, 1.0 eq.), DBTDL (106 μL, 0.176 mmol, 4.2 eq.), and DMF (0.8 mL) to afford yellow solid as the product 10 (84.7 mg, 85%) on flash chromatography (10–20% acetone in DCM). Mp. 121–123 °C; TLC (24% acetone in DCM): Rf 0.16; 1H NMR (400 MHz, CDCl3): δ 8.38–8.15 (m, 8H), 7.40 (d, J = 8.9 Hz, 2H), 7.08–6.93 (m, 8H), 6.82 (d, J = 11.1 Hz, 4H), 6.68 (d, J = 8.8 Hz, 2H), 6.56 (d, J = 2.7 Hz, 2H), 4.76 (s, 2H), 4.27 (t, J = 6.2 Hz, 8H), 4.06 (d, J = 7.1 Hz, 4H), 3.93 (t, J = 6.6 Hz, 4H), 3.47 (q, J = 7.1 Hz, 8H), 3.33 (q, J = 5.7 Hz, 8H), 3.17 (d, J = 6.8 Hz, 4H), 2.93 (q, J = 7.1 Hz, 12H), 2.79 (t, J = 6.4 Hz, 4H), 2.15 (t, J = 6.9 Hz, 8H), 2.00 (q, J = 6.0 Hz, 8H), 1.80–1.55 (m, 18H), 1.53–1.23 (m, 66H); 13C NMR (101 MHz, CDCl3): δ 160.3, 157.3, 157.1, 156.9, 155.7, 153.44, 151.4, 150.8, 146.9, 146.8, 139.5, 137.4, 135.0, 134.6, 129.9, 125.9, 122.1, 122.1, 119.7, 117.1, 116.2, 115.32, 110.0, 107.1, 107.0, 106.2, 99.9, 97.0, 68.0, 64.9, 64.6, 64.5, 50.1, 49.7, 44.9, 30.9, 29.9, 29.7, 29.4, 29.4, 29.3, 29.3, 29.2, 29.2, 29.0, 28.5, 27.5, 26.2, 25.9, 25.8, 22.5, 22.4, 21.2, 20.3, 20.2, 12.4; MALDI-TOF-MS (CCA matrix) (m/z): [M + Na]+ calcd for C122H152N22O22Na 2300.13; found 2301.82.
Blue and mint-green fluorescing dendrimer 11, 14, and 15
General method of dendron attachment was performed using dendron 6 (80.0 mg, 0.078 mmol, 1.0 eq.), 8 (83.6 mg, 0.078 mmol, 1.0 eq.), hexamethylene diisocyanate (13 μL, 0.078 mmol, 1.0 eq.), DBTDL (203 μL, 0.341 mmol, 4.2 eq.), and DMF (1.6 mL) to afford yellow solid as the products 11 (7.5 mg), 14 (153.0 mg), and 15 (13.0 mg) on flash chromatography (10–24% acetone in DCM) (98%, overall yield).
Dendrimer 11. Mp. 130–132 °C; TLC (24% acetone in DCM): Rf 0.25; 1H NMR (500 MHz, CDCl3): δ 8.34 (d, J = 4.4 Hz, 7H), 7.40 (d, J = 8.9 Hz, 4H), 7.03 (d, J = 1.9 Hz, 6H), 6.84 (s, 4H), 6.68 (dd, J = 8.9, 2.5 Hz, 4H), 6.56 (d, J = 2.4 Hz, 4H), 4.77 (s, 2H), 4.27 (t, J = 6.1 Hz, 8H), 4.05 (t, J = 6.8 Hz, 4H), 3.93 (t, J = 6.5 Hz, 4H), 3.46 (q, J = 7.1 Hz, 16H), 3.17 (d, J = 6.8 Hz, 4H), 2.94 (t, J = 7.4 Hz, 8H), 2.15 (p, J = 6.7 Hz, 8H), 1.74 (p, J = 6.7 Hz, 8H), 1.59 (d, J = 7.4 Hz, 6H), 1.49 (d, J = 6.7 Hz, 4H), 1.45–1.23 (m, 74H); 13C NMR (101 MHz, CDCl3): δ 178.8, 160.3, 157.1, 156.9, 155.7, 153.5, 151.5, 146.8, 139.5, 134.7, 129.9, 122.1, 117.0, 110.0, 107.1, 99.9, 97.0, 69.6, 68.0, 64.9, 64.50, 53.7, 45.4, 44.9, 40., 34.04, 31.9, 31.8, 31.7, 31.6, 29.9, 29.7, 29.6, 29.6, 29.6, 29.4, 29.4, 29.4, 29.41, 29.3, 29.3, 29.2, 29.2, 29.1, 29.0, 28.9, 28.4, 26.2, 25.9, 25.8, 24.7, 22.6, 22.6, 22.6, 22.4, 14.1, 14.0, 12.4, 8.5; MALDI-TOF-MS (CCA matrix) (m/z): [M + Na]+ calcd for C118H152N22O22Na 2252.13; found 2251.41.
Dendrimer 14. Mp 131–134 °C; TLC (24% acetone in DCM): Rf 0.16; 1H NMR (400 MHz, CDCl3): δ 8.41–8.14 (m, 8H), 7.38 (d, J = 8.8 Hz, 3H), 7.25–6.99 (m, 6H), 6.95 (s, 2H), 6.83 (s, 4H), 6.67 (d, J = 9.0 Hz, 2H), 6.54 (d, J = 2.6 Hz, 2H), 4.81 (s, 1H), 4.25 (d, J = 6.5 Hz, 8H), 4.05 (d, J = 7.1 Hz, 3H), 3.91 (t, J = 6.3 Hz, 4H), 3.45 (q, J = 7.3 Hz, 10H), 3.31 (d, J = 6.1 Hz, 6H), 3.16 (d, J = 6.9 Hz, 3H), 2.98–2.71 (m, 14H), 2.13 (t, J = 7.3 Hz, 8H), 1.98 (d, J = 5.9 Hz, 6H), 1.76–1.54 (m, 10H), 1.52–1.22 (m, 64H); 13C NMR (101 MHz, CDCl3): δ 178.4, 160.3, 157.3, 157.1, 156.9, 155.76, 153.5, 151.4, 150.8, 146.9, 146.8, 146.7, 139.5, 135.0, 134.7, 129.9, 125.9, 122.1, 122.1, 119.7, 117.0, 116.20, 110.0, 107.1, 107.0, 106.2, 99.9, 97.0, 68.0, 64.9, 64.5, 64.5, 50.1, 49.6, 44.9, 44.7, 40.7, 34.4, 31.9, 31.8, 29.9, 29.7, 29.66, 29.62, 29.48, 29.43, 29.36, 29.34, 29.32, 29.26, 29.2, 29.0, 28.4, 27.5, 26.28 25.9, 25.8, 25.0, 22.6, 22.6, 22.1, 22.4, 21.2, 20.3, 20.2, 14.1, 14.0, 12.4, 8.6; MALDI-TOF-MS (CCA matrix) (m/z): [M + Na]+ calcd for C122H152N22O22Na 2300.13; found 2301.82.
Dendrimer 15. Mp 133–135 °C; TLC (24% acetone in DCM): Rf 0.09; 1H NMR (400 MHz, CDCl3): δ 8.37–8.15 (m, 8H), 7.25–6.99 (m, 6H), 6.95 (s, 4H), 6.84 (s, 4H), 4.80 (t, J = 6.2 Hz, 2H), 4.26 (t, J = 6.2 Hz, 8H), 4.05 (d, J = 7.0 Hz, 4H), 3.92 (t, J = 6.5 Hz, 4H), 3.32 (t, J = 5.6 Hz, 16H), 3.15 (t, J = 7.0 Hz, 4H), 2.91 (d, J = 8.6 Hz, 16H), 2.78 (t, J = 6.3 Hz, 8H), 2.13 (p, J = 7.0 Hz, 8H), 1.99 (h, J = 6.0 Hz, 16H), 1.76–1.56 (m, 10H), 1.52–1.24 (m, 50H); 13C NMR (101 MHz, CDCl3): δ 162.6, 160.3, 157.3, 156.9, 153.4, 150.81, 146.9, 146.7, 139.5, 134.9, 125.9, 122.1, 119.7, 116.1, 107.0, 106.2, 99.91, 69.5, 67.9, 64.9, 64.5, 53.8, 50.10, 49.6, 40.7, 36.5, 31.9, 31.7, 31.4, 29.9, 29.7, 29.6, 29.4, 29.4, 29.3, 29.3, 29.2, 29.2, 29.2, 29.0, 28.4, 27.5, 26.2, 25.9, 25.8, 22.7, 22.5, 21.2, 20.3, 20.2, 14.1; MALDI-TOF-MS (CCA matrix) (m/z): [M + Na]+ calcd for C126H152N22O22Na 2348.13; found 2349.21.
Surface modification of dendrimer 10 using different azidocoumarins
General method of azide–alkyne click reaction was employed using PU dendrimer 10 (95.8 mg, 0.080 mmol, 1.0 eq.), azidocoumarin 4 (50.0 mg, 0.192 mmol, 2.4 eq.), azidocoumarin 5 (54.2 mg, 0.192 mmol, 2.4 eq.), CuSO4·5H2O (8.0 mg, 0.048 mmol, 0.6 eq.), sodium ascorbate (19.0 mg, 0.0.96 mmol, 1.2 eq.), THF (1.5 mL), and water (200 μL) to afford solids as the product (112.3 mg, 62%) on flash chromatography (10–24% acetone in DCM). Dendrimer 12 was exclusively formed (90.0 mg). Mp 129–133 °C; TLC (26% acetone in DCM): Rf 0.17; 1H NMR (400 MHz, CDCl3): δ 8.33 (t, J = 2.9 Hz, 7H), 8.23 (s, 1H), 7.39 (d, J = 8.8 Hz, 3H), 7.15–6.91 (m, 6H), 6.84 (s, 4H), 6.67 (d, J = 9.0 Hz, 3H), 6.55 (s, 3H), 4.77 (s, 2H), 4.26 (t, J = 6.2 Hz, 8H), 4.04 (t, J = 6.9 Hz, 4H), 3.93 (t, J = 6.6 Hz, 4H), 3.46 (q, J = 7.2 Hz, 12H), 3.32 (q, J = 5.7 Hz, 4H), 3.17 (q, J = 6.9 Hz, 4H), 2.93 (t, J = 7.6 Hz, 10H), 2.79 (t, J = 6.4 Hz, 2H), 2.14 (t, J = 7.0 Hz, 7H), 1.99 (d, J = 5.9 Hz, 3H), 1.81–1.55 (m, 12H), 1.51–1.22 (m, 56H); 13C NMR (101 MHz, CDCl3): δ 160.3, 157.1, 156.9, 155.7, 153.4, 151.4, 146.9, 139.5, 135.0, 134.6, 129.9, 125.9, 122.1, 119.7, 117.1, 110.0, 107.1, 107.0, 106.2, 99.95, 97.0, 68.0, 64.9, 64.5, 50.1, 49.6, 44.9, 40.7, 30.9, 29.9, 29.4, 29.4, 29.3, 29.2, 29.2, 29.0, 28.5, 27.5, 26.2, 25.9, 25.8, 22.4, 21.2, 20.3, 20.2, 12.4; MALDI-TOF-MS (CCA matrix) (m/z): [M + Na]+ calcd for C120H152N22O22Na 2276.13; found 2276.77.
Author contributions
D. P. planned and conducted the synthetic experiments; isolated and characterized the products and wrote the manuscript. R. T. T. supervised the project and edited the manuscript. All authors approved the final version of the manuscript.
Conflicts of interest
There are no conflicts to declare.
Acknowledgements
We acknowledge the Department of Chemistry and Biochemistry of Miami University for the support and guidance.
Notes and references
- K. T. Al-Jamal, P. Ruenraroengsak, N. Hartell and A. T. Florence, An intrinsically fluorescent dendrimer as a nanoprobe of cell transport, J. Drug Targeting, 2006, 14, 405–412 CrossRef CAS PubMed.
- V. Balzani, P. Ceroni, S. Gestermann, M. Gorka, C. Kauffmann and F. Vögtle, Fluorescent guests hosted in fluorescent dendrimers, Tetrahedron, 2002, 58, 629–637 CrossRef CAS.
- C. M. Cardona, J. Alvarez, A. E. Kaifer, T. D. McCarley, S. Pandey and G. A. Baker, et al, Dendrimers functionalized with a single fluorescent dansyl group attached “off center”: Synthesis and photophysical studies, J. Am. Chem. Soc., 2000, 122, 6139–6144 CrossRef CAS.
- D. Liu, S. De Feyter, M. Cotlet, U. M. Wiesler, T. Weil and A. Herrmann, et al, Fluorescent self-assembled polyphenylene dendrimer nanofibers, Macromolecules, 2003, 36, 8489–8498 CrossRef CAS.
- X. Ban, W. Jiang, T. Lu, X. Jing, Q. Tang and S. Huang, et al, Self-host thermally activated delayed fluorescent dendrimers with flexible chains : an effective strategy for non-doped electroluminescent devices based on solution processing, J. Mater. Chem. C, 2016, 4, 8810–8816 RSC.
- J. Li, Q. Li and D. Liu, Novel thieno-[3,4-b]-pyrazines cored dendrimers with carbazole dendrons: Design, synthesis, and application in solution-processed red organic light-emitting diodes, ACS Appl. Mater. Interfaces, 2011, 3, 2099–2107 CrossRef CAS PubMed.
- Z. Ma, Y. Wan, W. Dong, Z. Si, Q. Duan and S. Shao, Alkoxy encapsulation of carbazole-based thermally activated delayed fluorescent dendrimers for highly efficient solution-processed organic light-emitting diodes, Chin. Chem. Lett., 2021, 32, 703–707 CrossRef CAS.
- K. Sun, D. Chu, Y. Cui, W. Tian, Y. Sun and W. Jiang, Near-infrared thermally activated delayed fluorescent dendrimers for the efficient non-doped solution-processed organic light-emitting diodes, Org. Electron., 2017, 48, 389–396 CrossRef CAS.
- L. Zhao, S. Wang, J. Ding and L. Wang, Solution-Processible Blue Fluorescent Dendrimers with Carbazole/Diphenylamine Hybrid Dendrons for Power-Efficient Organic Light-Emitting Diodes, ACS Omega, 2019, 4, 15923–15928 CrossRef CAS PubMed.
- A. J. Clulow, P. L. Burn, P. Meredith and P. E. Shaw, Fluorescent carbazole dendrimers for the detection of nitroaliphatic taggants and accelerants, J. Mater. Chem., 2012, 22, 12507–12516 RSC.
- I. Grabchev, D. Staneva and R. Betcheva, Fluorescent Dendrimers As Sensors for Biologically Important Metal Cations, Curr. Med. Chem., 2012, 19, 4976–4983 CrossRef CAS PubMed.
- S. Fuchs, H. Otto, S. Jehle, P. Henklein and A. D. Schlüter, Fluorescent dendrimers with a peptide cathepsin B cleavage site for drug delivery applications, Chem. Commun., 2005, 1, 1830–1832 RSC.
- Y. Cai, C. Ji, S. Zhang, Z. Su and M. Yin, Synthesis of water-soluble dye-cored poly(amidoamine) dendrimers for long-term live cell imaging, Sci. China Mater., 2018, 61, 1475–1483 CrossRef CAS.
- W. Luo, R. Jiang, M. Liu, Q. Wan, J. Tian and Y. Wen, et al, Synthesis of fluorescent dendrimers with aggregation-induced emission features through a one-pot multi-component reaction and their utilization for biological imaging, J. Colloid Interface Sci., 2018, 509, 327–333 CrossRef CAS PubMed.
- L. J. Twyman, Post synthetic modification of the hydrophobic interior of a water-soluble dendrimer, Tetrahedron Lett., 2000, 41, 6875–6878 CrossRef CAS.
- S. R. Woodruff and N. V. Tsarevsky. Synthesis of Star Polymers with Epoxide-Containing Highly Branched Cores by Low-Catalyst Concentration Atom Transfer Radical Polymerization and Post-Polymerization Modifications. Controlled Radical Polymerization: Materials, 2015, ch. 11, pp. 149–167 Search PubMed.
- M. Zhu, N. Hao, M. Zaheer, J. Yang and L. Li, Preparation of Functional Long-Subchain Hyperbranched Polystyrenes via Post-polymerization Modification: Study on the Critical Role of Chemical Stability of Branching Linkage, ChemistryOpen, 2020, 9, 967–974 CrossRef CAS PubMed.
- V. V. Rostovtsev, L. G. Green, V. V. Fokin and K. B. Sharpless, A stepwise huisgen cycloaddition process: Copper(I)-catalyzed regioselective “ligation” of azides and terminal alkynes, Angew. Chem., Int. Ed., 2002, 41, 2596–2599 CrossRef CAS.
- C. W. Tornøe, C. Christensen and M. Meldal, Peptidotriazoles on solid phase: [1,2,3]-Triazoles by regiospecific copper(I)-catalyzed 1,3-dipolar cycloadditions of terminal alkynes to azides, J. Org. Chem., 2002, 67, 3057–3064 CrossRef.
- R. Das and B. Mukhopadhyay, Use of “click chemistry” for the synthesis of carbohydrate-porphyrin dendrimers and their multivalent approach toward lectin sensing, Tetrahedron Lett., 2016, 57, 1775–1781 CrossRef CAS.
- C. Li, H. Li and G. Jie, Click chemistry reaction-triggered DNA walker amplification coupled with hyperbranched DNA nanostructure for versatile fluorescence detection and drug delivery to cancer cells, Microchim. Acta, 2020, 187, 625 CrossRef CAS PubMed.
- N. T. Nguyen, J. Hofkens, I. G. Scheblykin, M. Kruk and W. Dehaen, Click reaction synthesis and photophysical studies of dendritic metalloporphyrins, Eur. J. Org. Chem., 2014, 2014, 1766–1777 CrossRef CAS.
- S. C. Han, S. H. Jin and J. W. Lee, Synthesis of dendrimer containing carbazole unit as a core chromophore, Bull. Korean Chem. Soc., 2012, 33, 3451–3454 CrossRef CAS.
- Q. M. Kainz, A. Schätz, A. Zöpfl, W. J. Stark and O. Reiser, Combined covalent and noncovalent functionalization of nanomagnetic carbon surfaces with dendrimers and BODIPY fluorescent dye, Chem. Mater., 2011, 23, 3606–3613 CrossRef CAS.
- S. Xiao, N. Fu, K. Peckham and B. D. Smith, Efficient synthesis of fluorescent squaraine rotaxane dendrimers, Org. Lett., 2010, 12, 140–143 CrossRef CAS PubMed.
- P. Rajakumar and R. Raja, Synthesis and photophysical properties of chiral dendrimers with quinoline surface group via click chemistry, Tetrahedron Lett., 2010, 51, 4365–4370 CrossRef CAS.
- S. K. Yang, X. Shi, S. Park, S. Doganay, T. Ha and S. C. Zimmerman, Monovalent, clickable, uncharged, water-soluble perylenediimide-cored dendrimers for target-specific fluorescent biolabeling, J. Am. Chem. Soc., 2011, 133, 9964–9967 CrossRef CAS PubMed.
- B. Bruchmann, Dendritic polymers based on urethane chemistry – Syntheses and applications, Macromol. Mater. Eng., 2007, 292, 981–992 CrossRef CAS.
- S. Matsumura, A. R. Hlil, C. Lepiller, J. Gaudet, D. Guay and Z. Shi, et al, Stability and Utility of Pyridyl Disulfide Functionality in RAFT and Conventional Radical Polymerizations, J. Polym. Sci., Part A: Polym. Chem., 2008, 46, 7207–7224 CrossRef.
- D. P. Poudel and R. T. Taylor, A Model for Late-Stage Modification of Polyurethane Dendrimers Using Thiol–Ene Click Chemistry, ACS Omega, 2021, 6, 12375–12381 CrossRef CAS.
- K. Sivakumar, F. Xie, B. M. Cash, S. Long, H. N. Barnhill and Q. Wang, A fluorogenic 1,3-dipolar cycloaddition reaction of 3-azidocoumarins and acetylenes, Org. Lett., 2004, 6, 4603–4606 CrossRef CAS.
- V. Hong, S. I. Presolski, C. Ma and M. G. Finn, Analysis and optimization of copper-catalyzed azide-alkyne cycloaddition for bioconjugation, Angew. Chem., Int. Ed., 2009, 48, 9879–9883 CrossRef CAS PubMed.
- S. Niyogi, S. Sarkar and B. Adhikari, The Modification of Polyurethanes by Highly Ordered Coordination Compounds of Transition Metals, Indian J. Chem. Technol., 2012, 9, 330–333 Search PubMed.
- H. Sardon, A. Pascual, D. Mecerreyes, D. Taton, H. Cramail and J. L. Hedrick, Synthesis of polyurethanes using organocatalysis: A perspective, Macromolecules, 2015, 48, 3153–3165 CrossRef CAS.
- N. Delhi, Tin(II and IV) Complexes with Nitrogen Ligands: Review of Recent Developments, Main Group Met. Chem., 2003, 26, 155–211 Search PubMed.
- P. I. P. Elliott, Organometallic complexes with 1,2,3-triazole-derived ligands, Organomet. Chem., 2014, 39, 1–25 CAS.
- J. A. Broussard, B. Rappaz, D. J. Webb and C. M. Brown, Fluorescence resonance energy transfer microscopy as demonstrated by measuring the activation of the serine/threonine kinase Akt, Nat. Protoc., 2013, 8, 265–281 CrossRef CAS.
- D. C. Harris, Quantitative Chemical Analysis, W. H. Freman and Company, New York, 2012 Search PubMed.
|
This journal is © The Royal Society of Chemistry 2022 |