DOI:
10.1039/D2RA04730F
(Paper)
RSC Adv., 2022,
12, 32097-32101
Lewis acid-catalyzed [4 + 2] cycloaddition of donor–acceptor cyclobutanes with iminooxindoles: access to spiro[piperidine-3,2′-oxindoles]†
Received
29th July 2022
, Accepted 27th October 2022
First published on 9th November 2022
Abstract
A new approach is described for the synthesis of spiro[piperidine-3,2′-oxindoles] in 35–82 yields with excellent stereoselectivity via the [4 + 2] cycloaddition reaction of donor–acceptor cyclobutanes with iminooxindoles in the presence of 10–30 mol% Sc(OTf)3 at room temperature. This methodology provides great potential for building spiro-heterocycle compounds from simple building blocks.
Spirocyclic oxindole skeletons are present in numerous naturally occurring alkaloids and medicinal active molecules.1 Among them, spiro[piperidine-3,2′-oxindoles] molecular frameworks are attractive scaffolds because of their usefulness in potential biological activities. For instance, the drug KAE609 (formerly known as NITD609 or cipargamin), which has been identified as a potential treatment for malaria,2 and quinoline spirooxindoles, which is a potential antitumour molecular.3 Furthermore, some spiro[piperidine-3,2′-oxindoles] are being investigated as peptide isosteres that can mimetic type II β-turn in the search for new enzyme inhibitors (Fig. 1).4 Given the importance of this structural class, the great advances that have occurred,1d,g–j the continuous development of catalytic and versatile synthetic strategies that allow the direct formation of these frameworks is still needed. Herein, we report a general and efficient method for the synthesis of highly substituted spiro[piperidine-3,2′-oxindoles] from donor–acceptor (D–A) cyclobutanes and iminooxindoles catalyzed by Lewis acid under mild conditions.
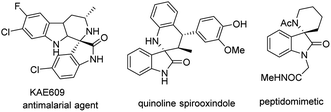 |
| Fig. 1 Some examples of bioactive spiro[piperidine-3,2′-oxindoles]. | |
Cycloaddition reactions of strained cycloalkanes represent one of the most efficient ways for the synthesis of carbo- and heterocycles in organic chemistry.5 Cyclobutanes are found in a large number of biologically active natural products.6 Moreover, as an important type of four-membered all-carbon building block, donor–acceptor (D–A) cyclobutanes have been used in the ring-opening reaction7 and applied in the construction of various cyclic molecular frameworks by [4 + n] cycloaddition8 under the catalysis of Lewis acids.9 For example, in 2010, Pagenkopf group reported a Yb(OTf)3 catalyzed [4 + 2] cycloaddition reaction of D–A cyclobutanes and imines, giving highly substituted piperidines (Scheme 1a). However, to the best of our knowledge, examples of cycloaddition of D–A cyclobutanes with iminooxindoles for constructing spiro[piperidine-3,2′-oxindoles] under Lewis acid have not been reported. Thus, the development of new synthetic protocols to access such spiro-heterocycle skeleton is still desirable (Scheme 1b).
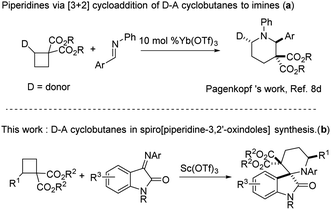 |
| Scheme 1 Previous work and our strategy for this study. | |
Recently, we reported a Lu(OTf)3-catalyzed [4 + 4] cycloaddition reaction of D–A cyclobutanes with anthranils to deliver oxa-bridged eight-membered heterocycles.10 Owing to our continuous interest in Lewis acid catalyzed reactions,11 particularly in D–A cyclobutanes involved transformations, we envisioned that, upon suitable activation by acid, the reaction of D–A cyclobutanes and iminooxindoles would provide a new approach to deliver diverse spiro[piperidine-3,2′-oxindoles] (Scheme 1b).
We tested our hypothesis using iminooxindole 1a and dimethyl 2-(4 methoxyphenyl)cyclobutane-1,1-dicarboxylate 2a as model substrates. Initially, the use of 10 mol% Yb(OTf)3 as a catalyst did not yield good results (Table 1, entries 1 and 2). To our delight, spiro[piperidine-3,2′-oxindoles] 3aa was isolated in 82% yield as a single isomer and a trace amount of unidentified product when the reaction was carried out under the catalysis of 10 mol% Sc(OTf)3 at room temperature (Table 1, entry 3). Catalysts such as Y(OTf)3, Hf(OTf)4, In(OTf)3, Cu(OTf)2, Lu(OTf)3 and Nd(OTf)3 result in low efficiency (Table 1, entries 4–9). To improve the yield, Lewis acids, including iron salts, tin salts and other kinds of Lewis acids, were also tested, and the results are unsatisfactory (Table 1, entries 10–19). The yield decreased when the reaction was carried out in DCE, toluene and CHCl3 (Table 1, entries 20–22). Polar solvents were unsuitable for this cycloaddition reaction (Table 1, entries 23 and 24). Only a 32% isolated yield was obtained when the reaction was performed without 4 Å molecular sieves (MS), indicating that a trace amount of water might result in a side product (Table 1, entry 25).
Table 1 Screening the reaction conditionsa
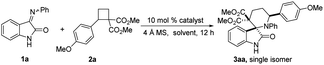
|
Entry |
Catalyst |
Solvent |
Isolated yield (%) |
Reaction conditions: 1a (0.22 mmol), 2a (0.2 mmol), 10 mol% catalyst, and 60 mg of activated 4 Å MS in 2.0 mL of solvent at room temperature, DCM = CH2Cl2, DCE = ClCH2CH2Cl, n.r. = no reaction. The reaction was performed according to the ref. 8d, at −50 °C for 1 h, then at 0 °C overnight. Without 4 Å MS. |
1 |
Yb(OTf)3 |
DCM |
Trace |
2b |
Yb(OTf)3 |
DCM |
n.r. |
3 |
Sc(OTf)3 |
DCM |
82 |
4 |
Y(OTf)3 |
DCM |
Trace |
5 |
Hf(OTf)4 |
DCM |
Trace |
6 |
In(OTf)3 |
DCM |
Trace |
7 |
Cu(OTf)2 |
DCM |
Trace |
8 |
Lu(OTf)3 |
DCM |
Trace |
9 |
Nd(OTf)3 |
DCM |
Trace |
10 |
Fe(OTf)3 |
DCM |
n.r. |
11 |
FeCl3 |
DCM |
30 |
12 |
SnCl2 |
DCM |
n.r. |
13 |
SnCl4 |
DCM |
Complex |
14 |
Bi(OTf)3 |
DCM |
n.r. |
15 |
Tm(OTf)3 |
DCM |
Trace |
16 |
Gd(OTf)3 |
DCM |
Trace |
17 |
Er(OTf)3 |
DCM |
Trace |
18 |
Ho(OTf)3 |
DCM |
Trace |
19 |
MgI2 |
DCM |
Trace |
20 |
Sc(OTf)3 |
DCE |
72 |
21 |
Sc(OTf)3 |
Toluene |
40 |
22 |
Sc(OTf)3 |
CHCl3 |
28 |
23 |
Sc(OTf)3 |
CH3CN |
Trace |
24 |
Sc(OTf)3 |
Acetone |
Trace |
25c |
Sc(OTf)3 |
DCM |
32 |
With the optimized reaction conditions in hand, the scope of this Lewis-acid catalyzed [4 + 2] cycloaddition reaction was explored with iminooxindole 1a and various D–A cyclobutanes 2, and the results are summarized in Table 2. 4-Phenyl D–A cyclobutane with an ethoxyl or benzyloxyl on the phenyl ring afforded the corresponding products in 67–72% yield (Table 2, 3ab–3ac). However, cyclobutane 2d without a substituent on the phenyl ring (R1 position) and 2e with a weak electron-withdrawing group (Br) on the phenyl ring (R1 position) could not undergo the cycloaddition reaction with 1a, and no desired cycloadducts were detected (Table 2, 3ad–3ae). The reaction of iminooxindole 1a and cyclobutanes 2f (R1 being 4-methylphenyl) also failed (Table 2, 3f). Di- and trisubstituted cyclobutanes 2g–2i with electron-donating groups on the phenyl ring (R1 position) also worked in this reaction, furnishing [4 + 2] cycloadditon products in 41–80% isolated yields albeit higher catalyst loading and longer reaction time were needed in some cases (Table 2, 3ag–3ai). For thienyl-substituted cyclobutane 3i, the desired [4 + 2] cycloaddition product 3aj was obtained in 46% yield (Table 2, 3aj). The aforementioned results indicate that the nature and position of the substituents on the aromatic rings significantly affect the reaction activity. Surprisingly, the dicarboxylates can be switched to other esters to give the desired spiro[piperidine-3,2′-oxindoles] 3ak–3al in 58–72% yields (Table 2, 3ak–3al). The structure and the relative stereochemistry of the products were established by X-ray crystallography analysis of 3aa (Fig. 2).
Reaction conditions: 1a (0.22 mmol), 2 (0.2 mmol), 10 mol% Sc(OTf)3, and 60 mg of activated 4 Å MS in 2.0 mL of DCM at rt, isolated yield. 100 mol% Sc(OTf)3 was used at 90 °C, and no desired product was detected. 20 mol% Sc(OTf)3 was used. 30 mol% Sc(OTf)3 was used. |
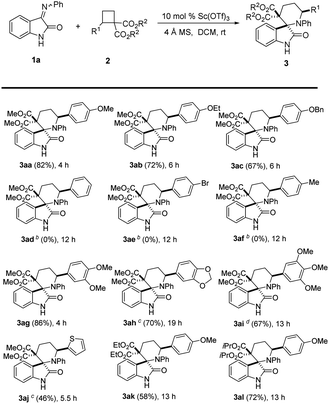 |
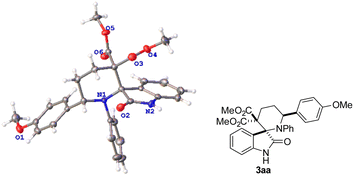 |
| Fig. 2 X-ray crystal structure of compound 3aa (CCDC 2143778†). | |
We studied the scope of this reaction due to the variation of the iminooxindole component (Table 3). The data in Table 3 showed that various functional groups were introduced into the oxindole fragment of iminooxindole 1b–1g (R3 position), such as halides (F, Cl, Br), methyl, methoxyl and nitro group, were compatible, affording the desired [4 + 2] cycloaddition products 3ba–3ga in 67–76% isolated yields (Table 3, 3ba–3ga). Similarly, iminooxindole 1h–1m, bearing both electron-withdrawing and electron-donating groups in the aromatic substituent at the imine N atom (R′ group), also reacted with D–A cyclobutane 2a and yielded corresponding products 3ha–3ma (Table 3, 3ha–3ma). Unfortunately, when we used iminooxindole 1 with alkyl groups (e.g., Bu, 1n) at the imine N atom, no desired cycloadduct 3na was detected. The reaction of N-protected iminooxindole 1o (R = Me) with cyclobutane 2a, affording the desired product in 35% yield (Table 3, 3oa). It is noteworthy to mention that no other stereoisomer was detectable in all the reactions we carried out.
Reaction conditions: 1a (0.22 mmol), 2 (0.2 mmol), 10 mol% Sc(OTf)3, and 60 mg of activated 4 Å MS in 2.0 mL of DCM at rt, isolated yield. 100 mol% Sc(OTf)3 was used at 90 °C, and no desired product was detected. |
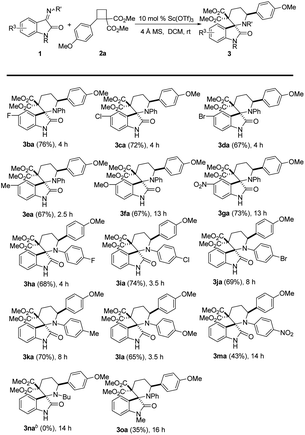 |
Based on these experimental results, a plausible mechanism that accounts for these results is proposed in Scheme 2. Initially, the Lewis acid Sc(OTf)3 binds to the ester moiety to produce intermediate IA. The direct C–C heterolysis of IA would yield intermediate IB (path a), which would readily react with iminooxindole to yield a zwitterionic intermediate IC. Subsequent cyclization via the favored conformation TS would afford cycloadducts 3 and regenerate the catalyst. The alternative reaction pathway (path b) to generate IC from IA is via an SN2-like nucleophilic attack of iminooxindole to the activated D–A cyclobutane offers the zwitterion IC, thereby causing the inversion of the stereochemistry at the activated R1 substituted carbon center of cyclobutane, which has been explored by Johnson group in donor–acceptor cyclopropanes.12 Thus, the reaction of enantioenriched cyclobutane would give an enantioenriched cycloadduct. To know which reaction pathway is the real one, enantioenriched 2a was prepared based on the method developed by Tang et al.13 After running for 4 h under standard reaction conditions, the reaction affords a racemic cycloadduct 3aa (Scheme 3), indicating that reaction path a may be a more possible reaction pathway.
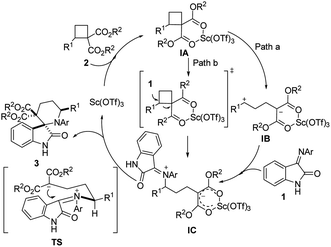 |
| Scheme 2 Plausible mechanism. | |
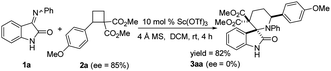 |
| Scheme 3 Stereospecificity of the [4 + 2] cycloaddition reaction of enantioenriched cyclobutane 2a. | |
Finally, the derivatization of spiro[piperidine-3,2′-oxindoles] 3 was investigated by the selective transformations of the representative compound 3aa. The diester group of 3aa can be reduced by LiAlH4 in THF, yielding the desired product 4 in an 89% isolated yield (Scheme 4).
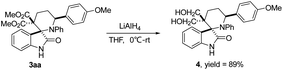 |
| Scheme 4 Transformations of product 3aa. | |
In conclusion, we have developed an Sc(OTf)3-catalyzed [4 + 2] cycloaddition reaction from D–A cyclobutanes and N-unprotected iminooxindoles under mild reaction conditions, providing the corresponding spiro[piperidine-3,2′-oxindoles] with good yields and excellent diastereoselectivity. Further studies on Lewis acid-catalyzed cycloaddition reactions are ongoing in our laboratory.
Conflicts of interest
There are no conflicts to declare.
Acknowledgements
This research was supported by National Natural Science Foundation of China (Grant No. 21861041) and Research Start-up Funding in University, China.
Notes and references
-
(a) A. A. Akaev, S. I. Bezzubov, V. G. Desyatkin, N. S. Vorobyeva, A. G. Majouga, M. Y. Melnikov and E. M. Budynina, J. Org. Chem., 2019, 84, 3340–3356 CrossRef CAS PubMed;
(b) A. J. Boddy and J. A. Bull, Org. Chem. Front., 2021, 8, 1026–1084 RSC;
(c) B. Yu, D.-Q. Yu and H.-M. Liu, Eur. J. Med. Chem., 2015, 97, 673–698 CrossRef CAS PubMed;
(d) G. S. Singh and Z. Y. Desta, Chem. Rev., 2012, 112, 6104–6155 CrossRef CAS PubMed;
(e) M. M. M. Santos, Tetrahedron, 2014, 70, 9735–9757 CrossRef CAS;
(f) T. L. Pavlovska, R. G. Redkin, V. V. Lipson and D. V. Atamanuk, Mol. Diversity, 2016, 20, 299–344 CrossRef CAS PubMed;
(g) G.-J. Mei and F. Shi, Chem. Commun., 2018, 54, 6607–6621 RSC;
(h) L. Hong and R. Wang, Adv. Synth. Catal., 2013, 355, 1023–1052 CrossRef CAS;
(i) Z.-Y. Cao, F. Zhou and J. Zhou, Acc. Chem. Res., 2018, 51, 1443–1454 CrossRef CAS PubMed;
(j) N. R. Ball-Jones, J. J. Badillo and A. K. Franz, Org. Biomol. Chem., 2012, 10, 5165–5181 RSC.
-
(a) H. Takada, N. Kumagai and M. Shibasaki, Org. Lett., 2015, 17, 4762–4765 CrossRef CAS PubMed;
(b) M. Rottmann, C. McNamara, B. K. S. Yeung, M. C. S. Lee, B. Zou, B. Russell, P. Seitz, D. M. Plouffe, N. V. Dharia, J. Tan, S. B. Cohen, K. R. Spencer, G. E. González-Páez, S. B. Lakshminarayana, A. Goh, R. Suwanarusk, T. Jegla, E. K. Schmitt, H.-P. Beck, R. Brun, F. Nosten, L. Renia, V. Dartois, T. H. Keller, D. A. Fidock, E. A. Winzeler and T. T. Diagana, Science, 2010, 329, 1175–1180 CrossRef CAS PubMed;
(c) B. K. S. Yeung, B. Zou, M. Rottmann, S. B. Lakshminarayana, S. H. Ang, S. Y. Leong, J. Tan, J. Wong, S. Keller-Maerki, C. Fischli, A. Goh, E. K. Schmitt, P. Krastel, E. Francotte, K. Kuhen, D. Plouffe, K. Henson, T. Wagner, E. A. Winzeler, F. Petersen, R. Brun, V. Dartois, T. T. Diagana and T. H. Keller, J. Med. Chem., 2010, 53, 5155–5164 CrossRef CAS PubMed.
- V. V. Kouznetsov, J. S. Bello Forero and D. F. Amado Torres, Tetrahedron Lett., 2008, 49, 5855–5857 CrossRef CAS.
- G. Lesma, N. Landoni, A. Sacchetti and A. Silvani, Tetrahedron, 2010, 66, 4474–4478 CrossRef CAS.
-
(a) H. K. Grover, M. R. Emmett and M. A. Kerr, Org. Biomol. Chem., 2015, 13, 655–671 RSC;
(b) N. Vemula and B. L. Pagenkopf, Org. Chem. Front., 2016, 3, 1205–1212 RSC;
(c) T. F. Schneider, J. Kaschel and D. B. Werz, Angew. Chem., Int. Ed., 2014, 53, 5504–5523 CrossRef CAS PubMed;
(d) V. A. Rassadin and Y. Six, Tetrahedron, 2016, 72, 4701–4757 CrossRef CAS;
(e) B. L. Pagenkopf and N. Vemula, Eur. J. Org. Chem., 2017, 2017, 2561–2567 CrossRef CAS;
(f) F. de Nanteuil, F. De Simone, R. Frei, F. Benfatti, E. Serrano and J. Waser, Chem. Commun., 2014, 50, 10912–10928 RSC;
(g) N. De and E. J. Yoo, ACS Catal., 2018, 8, 48–58 CrossRef CAS;
(h) D. B. Werz and A. T. Biju, Angew. Chem., Int. Ed., 2020, 59, 3385–3398 CrossRef CAS PubMed;
(i) V. Pirenne, B. Muriel and J. Waser, Chem. Rev., 2021, 121, 227–263 CrossRef CAS PubMed;
(j) J. C. Namyslo and D. E. Kaufmann, Chem. Rev., 2003, 103, 1485–1538 CrossRef CAS PubMed;
(k) H.-U. Reissig and R. Zimmer, Angew. Chem., Int. Ed., 2015, 54, 5009–5011 CrossRef CAS PubMed.
-
(a) M. Wang and P. Lu, Org. Chem. Front., 2018, 5, 254–259 RSC;
(b) Y.-Y. Fan, X.-H. Gao and J.-M. Yue, Sci. China: Chem., 2016, 59, 1126–1141 CrossRef CAS;
(c) J. Li, K. Gao, M. Bian and H. Ding, Org. Chem. Front., 2020, 7, 136–154 RSC.
-
(a) S. Kolb, M. Petzold, F. Brandt, P. G. Jones, C. R. Jacob and D. B. Werz, Angew. Chem., Int. Ed., 2021, 60, 15928–15934 CrossRef CAS PubMed;
(b) A. Kreft, S. Ehlers, P. G. Jones and D. B. Werz, Org. Lett., 2019, 21, 6315–6319 CrossRef CAS PubMed;
(c) N. L. Ahlburg, T. Freese, S. Kolb, S. Mummel, A. Schmidt and D. B. Werz, Eur. J. Org. Chem., 2021, 2021, 1603–1606 CrossRef CAS;
(d) S. Kolb, N. L. Ahlburg and D. B. Werz, Org. Lett., 2021, 23, 5549–5553 CrossRef CAS PubMed;
(e) B. Mondal, D. Das and J. Saha, Org. Lett., 2020, 22, 5115–5120 CrossRef CAS PubMed.
-
(a) S. Shigeru, S. Kazuhiko, N. Hideyuki and H. Masaki, Chem. Lett., 1991, 20, 1149–1152 CrossRef;
(b) E. A. Allart, S. D. R. Christie, G. J. Pritchard and M. R. J. Elsegood, Chem. Commun., 2009, 7339–7341 RSC;
(c) A. T. Parsons and J. S. Johnson, J. Am. Chem. Soc., 2009, 131, 14202–14203 CrossRef CAS PubMed;
(d) M. M. A. R. Moustafa and B. L. Pagenkopf, Org. Lett., 2010, 12, 4732–4735 CrossRef CAS PubMed;
(e) M. M. A. R. Moustafa, A. C. Stevens, B. P. Machin and B. L. Pagenkopf, Org. Lett., 2010, 12, 4736–4738 CrossRef CAS PubMed;
(f) A. C. Stevens, C. Palmer and B. L. Pagenkopf, Org. Lett., 2011, 13, 1528–1531 CrossRef CAS PubMed;
(g) F. de Nanteuil and J. Waser, Angew. Chem., Int. Ed., 2013, 52, 9009–9013 CrossRef CAS PubMed;
(h) M. Kawano, T. Kiuchi, S. Negishi, H. Tanaka, T. Hoshikawa, J.-i. Matsuo and H. Ishibashi, Angew. Chem., Int. Ed., 2013, 52, 906–910 CrossRef CAS PubMed;
(i) N. Vemula, A. C. Stevens, T. B. Schon and B. L. Pagenkopf, Chem. Commun., 2014, 50, 1668–1670 RSC;
(j) J.-L. Hu, L. Wang, H. Xu, Z. Xie and Y. Tang, Org. Lett., 2015, 17, 2680–2683 CrossRef CAS PubMed;
(k) D. Perrotta, S. Racine, J. Vuilleumier, F. de Nanteuil and J. Waser, Org. Lett., 2015, 17, 1030–1033 CrossRef CAS PubMed;
(l) N. Vemula and B. L. Pagenkopf, Eur. J. Org. Chem., 2015, 2015, 4900–4906 CrossRef CAS;
(m) L.-W. Feng, H. Ren, H. Xiong, P. Wang, L. Wang and Y. Tang, Angew. Chem., Int. Ed., 2017, 56, 3055–3058 CrossRef CAS PubMed;
(n) L. K. B. Garve, A. Kreft, P. G. Jones and D. B. Werz, J. Org. Chem., 2017, 82, 9235–9242 CrossRef CAS PubMed;
(o) X.-K. Kuang, J. Zhu, L. Zhou, L. Wang, S. R. Wang and Y. Tang, ACS Catal., 2018, 8, 4991–4995 CrossRef CAS;
(p) E. Igarashi, K. Sakamoto, T. Yoshimura and J.-i. Matsuo, Tetrahedron Lett., 2019, 60, 13–15 CrossRef CAS;
(q) D. Tong, J. Wu, N. Bazinski, D. Koo, N. Vemula and B. L. Pagenkopf, Chem.–Eur. J., 2019, 25, 15244–15247 CrossRef CAS PubMed;
(r) S. Wei, L. Yin, S. R. Wang and Y. Tang, Org. Lett., 2019, 21, 1458–1462 CrossRef CAS PubMed;
(s) J. Wu, P. Winiarz, D. Patel, J. de Jong, D. Tong, T. Chidley, N. Vemula and B. L. Pagenkopf, Org. Lett., 2020, 22, 3140–3144 CrossRef CAS PubMed;
(t) A. Manel, J. Berreur, F. R. Leroux and A. Panossian, Org. Chem. Front., 2021, 8, 5289–5295 RSC.
-
(a) R. Dalpozzo, G. Bartoli, L. Sambri and P. Melchiorre, Chem. Rev., 2010, 110, 3501–3551 CrossRef CAS PubMed;
(b) S. Kobayashi, M. Sugiura, H. Kitagawa and W. W. L. Lam, Chem. Rev., 2002, 102, 2227–2302 CrossRef CAS PubMed;
(c) X.-M. Wang, P. Zhang, Q. Xu, C.-Q. Guo, D.-B. Zhang, C.-J. Lu and R.-R. Liu, J. Am. Chem. Soc., 2021, 143, 15005–15010 CrossRef CAS PubMed;
(d) Z. Chen, Z. Tian, J. Zhang, J. Ma and J. Zhang, Chem.–Eur. J., 2012, 18, 8591–8595 CrossRef CAS PubMed;
(e) D. B. Baudry, A. Dormond, F. Duris, J. M. Bernard and J. R. Desmurs, J. Fluorine Chem., 2003, 121, 233–238 CrossRef CAS;
(f) P. Zhang, X.-M. Wang, Q. Xu, C.-Q. Guo, P. Wang, C.-J. Lu and R.-R. Liu, Angew. Chem., Int. Ed., 2021, 60, 21718–21722 CrossRef CAS PubMed;
(g) S. Antoniotti, V. Dalla and E. Duñach, Angew. Chem., Int. Ed., 2010, 49, 7860–7888 CrossRef CAS PubMed.
- M. Hou, J. Li, F. Rao, Z. Chen and Y. Wei, Chem. Commun., 2022, 58, 5865–5868 RSC.
-
(a) J. Yan, H. Luo, Z. Chen, Y. Wei, H. Zhan and Y. Mei, Tetrahedron Lett., 2020, 61, 151453 CrossRef CAS;
(b) H. Luo, J. Yan, Z. Chen, Y. Wei, B. Chen and Y. Liu, ChemistrySelect, 2020, 5, 4074–4077 CrossRef CAS;
(c) H. Zhan, M. Hou, Y. Li, Z. Chen, Y. Wei and S. Liu, ChemistrySelect, 2021, 6, 11537–11540 CrossRef CAS.
- P. D. Pohlhaus, S. D. Sanders, A. T. Parsons, W. Li and J. S. Johnson, J. Am. Chem. Soc., 2008, 130, 8642–8650 CrossRef CAS PubMed.
- J.-L. Hu, L.-W. Feng, L. Wang, Z. Xie, Y. Tang and X. Li, J. Am. Chem. Soc., 2016, 138, 13151–13154 CrossRef CAS PubMed.
Footnotes |
† Electronic supplementary information (ESI) available. CCDC 2143778. For ESI and crystallographic data in CIF or other electronic format see DOI: https://doi.org/10.1039/d2ra04730f |
‡ These authors contributed equally to this work. |
|
This journal is © The Royal Society of Chemistry 2022 |
Click here to see how this site uses Cookies. View our privacy policy here.