DOI:
10.1039/D2RA04276B
(Paper)
RSC Adv., 2022,
12, 26975-26988
Extraction of flavonoids from Citri Reticulatae Pericarpium Viride using a deep eutectic solvent†
Received
11th July 2022
, Accepted 12th September 2022
First published on 21st September 2022
Abstract
Flavonoids are the main active ingredient in Citri Reticulatae Pericarpium Viride (CRPV). In this study, a deep eutectic solvent (DES) was explored to extract the main flavonoids from CRPV, including narirutin (NAR) and hesperidin (HES). A total of 30 DESs were prepared, and DES-20 (proline
:
urea) was selected as the optimal solvent. According to the single factor and response surface methodology experiments, the optimal extraction conditions for the flavonoids included a molar ratio of 1
:
2, water content of 30%, extraction time of 28 min, extraction power of 240 W, and a liquid-to-solid ratio of 70 mL g−1 and the optimal extraction yields of NAR and HES were 21 ± 2 mg g−1 and 60 ± 2 mg g−1, respectively. The recovery rate of NAR and HES with macroporous resin SP825 were 88 ± 3% and 86 ± 4%, respectively. Compared with traditional extraction methods, this novel method greatly improved extraction efficiency and saved time. Scanning electron microscopy results showed that this novel method could destroy the plant cell wall to enhance the extraction efficiency. Then, 21 flavonoids were identified through an ultra-high-performance liquid chromatography-quadrupole time-of-flight mass spectrometry (UPLC-Q-TOF-MS) technique and nine of them were discovered for the first time in CRPV extracts. Furthermore, a high-performance liquid chromatography (HPLC) method was simultaneously used to determine NAR and HES in CRPV extracts upon methodological validation. Finally, antioxidant and cytotoxic experiments in vitro showed that the flavonoids extracted from the CRPV exhibited certain antioxidant and cytotoxic activities. The above results indicated that the DES was a green solvent, which can effectively extract NAR and HES from CRPV.
1. Introduction
Citrus reticulata Blanco, a fruit belonging to the Rutaceae family, is one of the most produced and consumed fruits in the world.1 In 2021, approximately 6.82 million hectares of land were used to cultivate 98 million tons of citrus worldwide.2 China is a major producer of citrus and its citrus industrial system is relatively complete, including fruits, beverages, canned goods, cosmetics, medicines, and additives.3 In particular, citrus has become one of the sources of medicine in the Chinese medicine industry. Citri Reticulatae Pericarpium Viride (CRPV), derived from the peel of Citrus reticulata Blanco, has been widely used as a traditional Chinese medicine for treating chest distension, hernia, nucleus pulposus, and canker sores.4 Several studies have revealed flavonoids, such as narirutin (NAR) and hesperidin (HES), as the main chemical components of CRPV.5 Flavonoids exhibit pharmacological activities, including antitumor, antioxidant, anti-inflammatory, antibacterial, and hypoglycemic.6,7 In the contemporary world, the flavonoids extracted from CRPV are important precursors for drugs and health products. Therefore, it is necessary to develop an efficient extraction method for extracting flavonoids from CRPV.
Usually, it is found that some extraction factors will exert a huge impact on the extraction process of flavonoids. Therefore, one classic process design method, response surface methodology (RSM), is generally chosen by previous researchers to obtain the optimal extraction process.8 The response surface methodology (RSM) refers to a statistical and mathematical tool for evaluating the relationship between factors and response values. Compared with other optimization methods (orthogonal experimental design and uniform test design), RSM can not only continuously analyze each level of the experiment, but also reduce the number of experiments and avoid wasting time.9 For these reasons, RSM is widely used to optimize the extraction process of natural products. Tarun et al.10 used RSM to optimize the extraction of phenolics in barberry fruit, and the results showed that the optimal extraction process were methanol concentration of 80%, pH of 3, liquid–solid ratio of 50
:
1 mL g−1, temperature of 80 °C, time of 30 min, and the extraction rate could reach 4659 ± 1%. In addition, in another study, RSM was used to optimize the extraction of flavonoids from Angelica sinensis, and the results showed that the optimal extraction conditions were a temperature of 80 °C, a time of 4 min, an ethanol concentration of 78%, and a liquid–solid ratio of 35 mL g−1, and the best extraction rate was 8 mg g−1.11
Generally, acetone, ethanol, and acetonitrile are widely used as extraction solvents in natural products such as flavonoids, polyphenols and styrene acrylic. However, these organic solvents are volatile, toxic and environmentally harmful.12 It is necessary to explore a green, environmentally friendly and efficient extraction solvent to extract active ingredients from natural products. Deep eutectic solvent (DES), firstly proposed by Abbott in 2003, is the eutectic mixture of hydrogen bond donors (HBDs) and hydrogen bond acceptors (HBAs).13 DES is characterized by easy preparation, low cost, low-vapor pressure, and good thermal stability, thus, DES is considered as an alternative to traditional solvents.14 According to the excellent characteristics of DES, a large number of complex natural products are fully extracted, including flavonoids, polyphenols and polysaccharides.15–17 Moreover, ultrasound-assisted DES extraction (UAE) possesses the advantages of both ultrasound extraction and DES extraction, which is able to maximize the yield of natural active ingredients as well as saving time and energy.18 All of the above points suggest that DES expands the approach to natural active substance extraction.
In this study, the extraction of flavonoids from the CRPV using UAE and the biological activity of CRPV extracts were investigated. First, 30 DESs were prepared and the best performed DESs were selected. Second, the recovery of flavonoids from DES extract was investigated. Then, the extraction conditions were optimized using single factor and response surface methodology (RSM). In addition, the UAE method was compared with the conventional extraction methods (ME and PE). The morphological alterations of the CRPV powder using the UAE and conventional extraction methods were initially evaluated via scanning electron microscopy (SEM) characterization. Moreover, the number of flavonoids in CRPV extracts was identified using the ultra-high-performance liquid chromatography-quadrupole time-of-flight mass spectrometry (UPLC-Q-TOF-MS) technique. Subsequently, the high-performance liquid chromatography (HPLC) method was simultaneously used to determine NAR and HES upon methodological validation. Finally, the biological activities of the flavonoid extract, including in vitro antioxidant and cytotoxic activities, were investigated.
2. Materials and methods
2.1. Reagents
The CRPV sample was collected from Zunyi in October 2020 and identified as genuine by the Pharmacy Teaching and Research Office of Zunyi Medical University. The samples (voucher number: CRPV-20211015) were kept at room temperature in a storage cabinet at 2–605, School of Pharmacy.
Choline chloride and betaine were purchased from Shanghai Yuanye Biotechnology Co., Ltd. (Shanghai, China, purity >98%). NAR and HES standards were obtained from Shanghai Merrill Chemical Technology Co., Ltd. (Chengdu, China, purity >98%). Chromatographic grade methanol and acetonitrile were purchased from Shuangju Chemical Co., Ltd. (Zunyi, Guizhou). CRPV was collected from Zunyi, Guizhou, China, and stored in a cool (light-free place).
2.2. Preparation of DES
The DESs were synthesized via the “one-pot method” described in previous reports.19 Generally, HBAs and HBDs with an appropriate amount of water were mixed and then stirred at 80 °C until a clear mixture was formed to prepare DESs. Then, the mixture was cooled at 25 °C and stored for subsequent experiments. The 30 DESs were prepared in this experiment (Table 1) and its appearances at room temperature were displayed in Fig. S1.†
Table 1 The viscosity of 30 DESs
Number |
Abbreviation |
HBA |
HBD |
Molar ratio |
Viscosity (Pa s) |
DES-1 |
Chcl : Lac |
Choline |
Lactic acid |
1 : 2 |
3 |
DES-2 |
Chcl : Ery |
Choline |
Erythritol |
1 : 2 |
13 |
DES-3 |
Chcl : Ure |
Choline |
Urea |
1 : 2 |
4 |
DES-4 |
Chcl : Gly |
Choline |
Glycerol |
1 : 2 |
3 |
DES-5 |
Chcl : But |
Choline |
1,2,4-Butanetriol |
1 : 2 |
4 |
DES-6 |
Chcl : Xyl |
Choline |
Xylitol |
1 : 2 |
4 |
DES-7 |
Chcl : Mal |
Choline |
L-Malic acid |
1 : 2 |
6 |
DES-8 |
Chcl : Cit |
Chloride |
Citric acid |
1 : 2 |
7 |
DES-9 |
Chcl : Glu |
Chloride |
D-Glucose |
1 : 2 |
7 |
DES-10 |
Bet : Lac |
Betaine |
Lactic acid |
1 : 2 |
3 |
DES-11 |
Bet : Gly |
Betaine |
Glycerol |
1 : 2 |
3 |
DES-12 |
Bet : But |
Betaine |
1,2,4-Butanetriol |
1 : 2 |
5 |
DES-13 |
Bet : Ery |
Betaine |
Erythritol |
1 : 2 |
5 |
DES-14 |
Bet : Mal |
Betaine |
L-Malic acid |
1 : 2 |
11 |
DES-15 |
Bet : Glu |
Betaine |
D-Glucose |
1 : 2 |
14 |
DES-16 |
Bet : Xyl |
Betaine |
Xylitol |
1 : 2 |
13 |
DES-17 |
Bet : Cit |
Betaine |
Citric acid |
1 : 2 |
10 |
DES-18 |
Bet : Fru |
Betaine |
D-Fructose |
1 : 2 |
33 |
DES-19 |
Bet : Sor |
Betaine |
D-Sorbitol |
1 : 2 |
6 |
DES-20 |
Pro : Ure |
L-Proline |
Urea |
1 : 2 |
2 |
DES-21 |
Pro : Lac |
L-Proline |
Lactic acid |
1 : 2 |
4 |
DES-22 |
Pro : But |
L-Proline |
1,2,4-Butanetriol |
1 : 2 |
9 |
DES-23 |
Pro : Ery |
L-Proline |
Erythritol |
1 : 2 |
7 |
DES-24 |
Pro : Gly |
L-Proline |
Glycerol |
1 : 2 |
5 |
DES-25 |
Pro : Mal |
L-Proline |
L-Malic acid |
1 : 2 |
20 |
DES-26 |
Pro : Cit |
L-Proline |
Citric acid |
1 : 2 |
23 |
DES-27 |
Pro : Xyl |
L-Proline |
Xylitol |
1 : 2 |
23 |
DES-28 |
Pro : Glu |
L-Proline |
D-Glucose |
1 : 2 |
14 |
DES-29 |
Lac : Glu |
Lactic acid |
D-Glucose |
1 : 2 |
8 |
DES-30 |
Lac : Fru |
Lactic acid |
D-Fructose |
1 : 2 |
16 |
2.3. Quantitative analysis of CRPV extract
2.3.1. Determination of NAR and HES content. NAR and HES were identified as the main flavonoids in CRPV, and their contents were determined via HPLC. The HPLC (Agilent 1260, Agilent, USA) was operated under the following conditions: a Phenomenex C18 column (5 μm, 250 × 4.6 mm, Guangzhou FLM Scientific Instrument Co., Ltd., China); mobile phases of acetonitrile (A) and 0.1% formic acid in water (B); flow rate, column temperature, and injection volume of 1 mL min−1, 30 °C and 20 μL, respectively; and detection wavelength of 283 nm. The elution procedure is listed in Table 2. As shown in Fig. 1, the peaks in the CRPV extract were determined according to the retention times of the HES standard (12.63 min) and NAR standard (13.86 min).
Table 2 Elution procedure
Time (min) |
Acetonitrile (%) |
0.1% aqueous formic acid (%) |
0 |
20 |
80 |
15 |
30 |
70 |
20 |
50 |
50 |
35 |
100 |
0 |
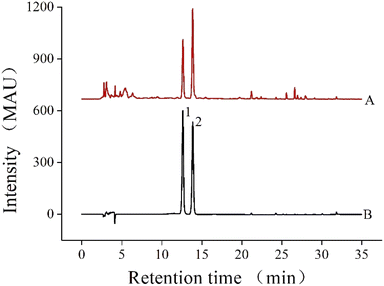 |
| Fig. 1 The HPLC chromatograms of the extracts of CRPV and the standard mixture solution ((A) CRPV extract, (B) the standard mixture solution; (1) NAR, (2) HES). | |
2.3.2. Preparation of CRPV extract. The dried CRPV was pulverized with a multifunctional pulverizer (2000C, Dongguan Fangtai Electric Co., Ltd., China) and passed through an 80-mesh sieve. Then, 1 g of CRPV powder and 70 mL of DES (30% water content) were added to an Erlenmeyer flask and the mixture was stirred. The extraction was performed with an ultrasonic cleaning instrument (KQ5200, Kunshan Ultrasonic Instruments Co., Ltd., China) at a power of 240 W for 30 min. After the extraction, the mixture was separated via centrifugation (3000 rpm) for 10 min. The supernatant was collected, diluted with methanol (to 100 mL), and filtered through a 0.45 μm microporous membrane for HPLC analysis. The contents of HES and NAR were determined using formula (1). |
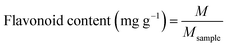 | (1) |
where M (mg) was the mass of HES and HES, Msample (mg) was the mass of CRPV extract.
2.4. Optimal DES screening
A total of 30 DESs listed in Table 1 were prepared to extract the flavonoids from CRPV via the method described in Section 2.3.2. Taking the content of flavonoids as the response, the DES with high extraction efficiency was selected through HPLC analysis.
2.5. Single factors experiment
Setting the content of two flavonoids in CRPV extract as indexes, the effects of DES molar ratio, DES water content, extraction time, solid–liquid ratio, and ultrasonic power on the indexes were investigated. The detailed parameters of the single-factor experiment are listed below. The extraction of flavonoids from CRPV was performed at different operating conditions: DES molar ratios (2
:
1, 1
:
1, 1
:
2, 1
:
3, and 1
:
4, HBA
:
HBD), DES water contents (10, 20, 30, 40, and 50%), extraction times (10, 30, 50, 70, and 90 min), solid–liquid ratios (10
:
1, 30
:
1, 50
:
1, 70
:
1, and 90
:
1 mL g−1), and ultrasonic power (160, 200, 240, 280, and 320 W).
2.6. Optimization of extraction process
Combined with the results of single-factor experiments, the extraction conditions of flavonoids in CRPV were optimized using Box–Behnken experimental design (BBD) experiments. DES molar ratio (1
:
1, 1
:
2 and 1
:
3), DES water content (20, 30, and 40%), and extraction time (10, 30, and 50 min) were selected as the main influencing factors and coded at three levels (−1, 0, 1) (Table 3). In addition, the extraction effect of NAR (Y1) and HES (Y2) were considered as the response value. The equation between factors and response is presented in eqn (2). |
Y = β0 + β1X1 + β2X2 + β3X3 + β11X12 + β22X22 + β33X32 + β12X1X2 + β23X2X3 + β13X1X3
| (2) |
where Y was the response value predicted by model, X was the independent variable, β0, β1, β2, β3 were the linear coefficients, β11, β22, β33 were the quadratic coefficients, β12, β13, β23 were the interaction coefficients.
Table 3 Factors and levels of Box–Behnken design
Factors |
Experimental level |
Low (−1) |
Medium (0) |
High (+1) |
X1: molar ratio |
1 |
2 |
3 |
X2: water content, % |
20 |
30 |
40 |
X3: extraction time, min |
10 |
30 |
50 |
2.7. Recovery of flavonoids from DES extracts
Recovery of flavonoids from DES extracts was achieved using macroporous resin. The details were as follows: 5 mL of CRPV extracts were adsorbed with 1 g of different pretreated macroporous resin (AB-8, D101, NAK-9, HPD600 and SP825) on a constant temperature shaker (SHA-C, Changzhou Guoyu Instrument Manufacturing Co., Ltd., China) at a speed of 100 rpm and 25 °C for 24 h. Subsequently, the filtered macroporous resin was desorbed with 20 mL of methanol under the same conditions. Finally, the mixture was filtered, and the filtrate was collected to determine the content of flavonoids through HPLC and calculate the recovery yield.
2.8. Comparison between traditional extraction methods
2.8.1. Maceration extraction (ME). In line with the previous report,20 1.0 g of CRPV powder and 70 mL of DES were blended and then soaked for 12 h. Then, the extract was collected through centrifugation (3000 rpm, 10 min) and concentrated under reduced pressure. The contents of flavonoids in the extracts were detected via HPLC.
2.8.2. Percolation extraction (PE). According to Hashemi et al.,21 1.0 g of CRPV powder was wetted with optimum DES and kept under seal for 30 min. Then, the mixture was percolated with DES at a flow rate of two drops per minute. After the percolation, the extract was collected and concentrated under reduced pressure, and the flavonoid contents were determined using HPLC.
2.9. Identification of CRPV extract
According to previous studies,22 the identification of flavonoids in CRPV extracts is usually carried out using UPLC-Q-TOF-MS. The specific analytical parameters were as follows: the system was an Acquity UPLC system (Waters Corp., Milford, MA, USA); the column was BEH C18 (100 × 2.1 mm, 1.7 μm); the mobile phase was 0.1% formic acid solution (A) and 0.1% formic acid acetonitrile (B), and the elution procedure is displayed in Table 4; and the flow rate, temperature, and injection volume were 0.4 mL min−1, 45 °C, and 10 μL, respectively.
Table 4 Elution procedure of UPLC-Q-TOF-MS
Time (min) |
0.1% formic acid (A) |
0.1% formic acid acetonitrile (B) |
0 |
95 |
5 |
3 |
80 |
20 |
10 |
0 |
100 |
12 |
0 |
100 |
15 |
95 |
5 |
20 |
95 |
5 |
Additionally, the Q-TOF was operated in the acquisition mode of the MSE model and ionization mode of ESI positive/negative. The MS data for 0–20 min was collected within the acquisition range of 5–1000 m/z at a scan rate of 0.2 s. Meanwhile, other pivotal parameters were set: the positive capillary voltage, negative capillary voltage, and cone voltage were defined at 2 kV, 2 kV, and 40 V, respectively, and the desolvation gas and temperature were set as 900 L h−1 and 450 °C, respectively, and cone gas and source temperatures were 50 L h−1 and 115 °C, respectively.
2.10. Antioxidant activity of flavonoid extract
2.10.1. 1,1-Diphenyl-2-picrylhydrazyl (DPPH) radical scavenging activity. In line with the report of Jiang et al.,23 2 mL of CRPV extract at different concentrations (0–1 mg mL−1) were reacted with 0.2 mmol L−1 DPPH solution for 30 min. Then, the absorbance was recorded at 517 nm, and the DPPH radical scavenging rate was calculated. Vc (in the range of 0–1 mg mL−1) were used as a standard. |
 | (3) |
in this formula, A0 represented the absorbance of the blank group and Ax referred the absorbance of the sample group.
2.10.2. 2,2-Azino-bis-3-ethybenzothiazoline-6-sulfonic acid (ABTS) radical scavenging activity. In line with the method presented by Lei et al.,24 7 mmol L−1 of ABTS stock solution and 2.45 mmol L−1 of potassium persulfate solution were mixed in equal volumes, and the mixture was shaken at room temperature for 16 h. The ABTS solution was diluted with anhydrous ethanol until the absorbance reached 0.7 at 734 nm. Then, 2 mL of different concentrations of CRPV extract and ABTS solution were reacted in the dark for 6 min and the ABTS radical clearance was detected at 734 nm and calculated using eqn (4). Vc (in the range of 0–1 mg mL−1) were used as a standard. |
 | (4) |
where A0 was the absorbance of the blank group, Ax indicated the absorbance of sample group.
2.10.3. Hydroxyl radical scavenging activity. The hydroxyl radical scavenging activity of CRPV extract was determined according to the method of Ke et al.25 A volume of 2 mL of CRPV extract, 0.3 mL of 1.5 mmol L−1 ferrous sulfate solution, 0.4 mL of 20 mmol L−1 sodium salicylate solution, and 0.3 mL of 6 mmol L−1 hydrogen peroxide were mixed and reacted for 30 min at 37 °C under water bath. Then, the absorbance was measured at 734 nm, and the hydroxyl radical scavenging rate was calculated using eqn (5). |
 | (5) |
where A1 was the absorbance of sample group, A2 referred to the control group, A0 represented the blank group.
2.11. Cytotoxic activity of flavonoids extract
The MTT method was adopted to examine the inhibitory effect of CRPV extract on CT-26, HCT-116, HT-29, and MCF-7 cells. The procedures were as follows: first, cancer cells at a logarithmic growth stage were spread in 96-well plates (cell density of 5 × 103 cells per well, 100 μL per well). The cells were grouped (control, 10, 100, 500, 1000, and 2000 μg mL−1 groups) when they reached approximately 50% growth. Then, CRPV extract was mixed with the cells and reacted for 48 h. A 20 μL of MTT (5 mg mL−1) was added to each well and incubated for 4 h. After the incubation, the culture medium was removed, and 150 μL of dimethyl sulfoxide (DMSO) was added to each well and shaken at low speed for 15 min. The absorbance was measured at 490 nm, and the cell inhibition rate was calculated through eqn (6). |
 | (6) |
where control group was the group of cells without adding CRPV extract, the blank group referred to the culture medium without cells and the test group is the group of cells with addition of the CRPV extract.
2.12. Statistical analysis
All of the experiments were repeated three times in this work, and the experimental data were expressed as mean ± standard deviation (±SD). Design Expert 8.0.6 was used to optimize the extraction process, and SPSS18.0 statistical software was used for statistical analysis. P < 0.05 indicated a statistical difference.
3. Results and discussion
3.1. Optimal DES screening
Various DESs possessed distinct physical properties, such as viscosity, density, which greatly impacted the extraction of natural products.26 Among them, viscosity is the key factor affecting the extraction effect. To this end, we further measured the viscosity of 30 DESs. As shown in Table 1, the viscosity of DES-20 (pro
:
ure) was the lowest among the 30 DESs, indicating a positive performance of DES-20 in extraction process. To verify this theory, 30 DESs were used to extract flavonoids from CRPV. As shown in Fig. 2, DES-20 exhibited the strongest extraction efficiency of NAR and HES among the 30 DESs. The high efficiency of DES-20 can be attributed to its polarity, similar to that of flavonoids. Moreover, the DES-20 exhibited a stronger extraction effect compared to traditional solvents (ethanol, 50% aqueous ethanol, acetone, 50% aqueous acetone, acetonitrile, and 50% aqueous acetonitrile). Thus, DES-20 was selected as the preferred solvent for the extraction of flavonoids from CRPV.
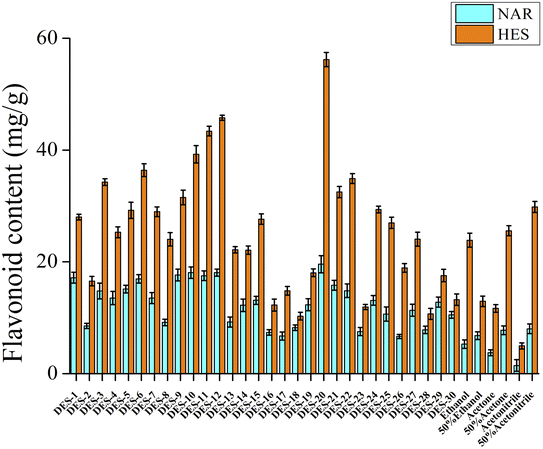 |
| Fig. 2 Effects of different DESs on the contents of NAR and HES in CRPV. | |
3.2. Single-factor experiment
To improve the extraction effect of flavonoids from CRPV, the DES molar ratio, DES water content, extraction time, liquid–solid ratio, and ultrasonic power were further examined. As shown in Fig. 3A–E, the DES molar ratio, DES water content, extraction time, liquid–solid ratio, and ultrasonic power significantly influenced the extraction of NAR and HES. In addition, DES molar ratio (1
:
1, 1
:
2, and 1
:
3), DES water content (20%, 30%, and 40%), extraction time (10, 30, and 50 min), liquid–solid ratio (70 mL g−1), and ultrasonic power (240 W) were applied in the RSM.
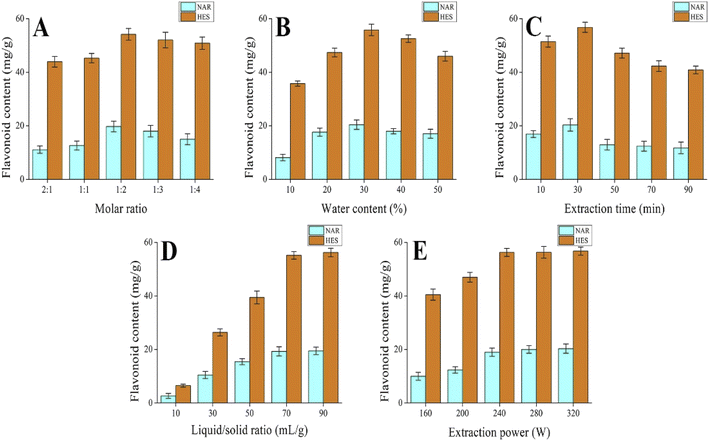 |
| Fig. 3 The effect of extraction parameters on the extraction rate of NAR and HES in CRPV (A) molar ratio, (B) water content, (C) extraction time, (D) solid–liquid ratio and (E) extraction power. | |
3.3. Optimization of extraction process
Based on the single-factor experimental results, the interaction effects between molar ratio (X1), water content (X2), and extraction time (X3) were performed via BBD in Design-Expert software (Stat-Ease Inc., Minneapolis, MN, USA) and the results were displayed in Table 5. BBD experiment method was adopted as an excellent tool to optimize the extraction parameter of the target components, which explored the interaction effects between variables with fewer experiments.27 Therefore, the BBD experimental method has been adopted by a large number of scholars to optimize the extraction process of natural products, including flavonoids, polyphenols, polysaccharides, etc.
Table 5 Results of Box–Behnken experiment
Run |
X1 |
X2 (%) |
X3 (min) |
Y1 (mg g−1) |
Y2 (mg g−1) |
1 |
2 |
30 |
30 |
19 |
56 |
2 |
1 |
40 |
30 |
17 |
50 |
3 |
3 |
40 |
30 |
10 |
36 |
4 |
2 |
40 |
10 |
15 |
45 |
5 |
2 |
20 |
50 |
16 |
47 |
6 |
1 |
30 |
50 |
16 |
48 |
7 |
1 |
30 |
10 |
17 |
49 |
8 |
2 |
40 |
50 |
13 |
40 |
9 |
3 |
20 |
30 |
14 |
43 |
10 |
2 |
30 |
30 |
19 |
56 |
11 |
1 |
20 |
30 |
15 |
48 |
12 |
2 |
30 |
30 |
19 |
56 |
13 |
2 |
30 |
30 |
20 |
57 |
14 |
3 |
30 |
50 |
12 |
38 |
15 |
3 |
30 |
10 |
14 |
43 |
16 |
2 |
30 |
30 |
20 |
57 |
17 |
2 |
20 |
10 |
15 |
44 |
The ANOVA analysis result (see Tables S1–S2†) showed that the R2 and RAdj2 of the two models were 0.9897 and 0.9920 and 0.9764 and 0.9818, respectively, indicating that these models were reasonable agreement and reliable, reflecting the relationship between response values and independent variables. Meanwhile, the high F values (74.56 and 96.92) and low P values (<0.05) indicated that the models were stable and significant. Moreover, all the three factors significantly influenced response values, with the P values less than 0.05. Additionally, the interaction factors X1X2 and X2X3 were significant (P < 0.05), illustrating that they could significantly impact the DES extraction process. Furthermore, the P values of lack of fit were all greater than 0.05 (insignificant), illustrating the reliability of the model. Moreover, multiple regression of the independent variable (X1, X2, and X3) and the dependent variable (Y1 and Y2) were analyzed to obtain second-order equations.
Y1 = 19.34 − 1.68X1 − 0.53X2 − 0.41X3 − 1.35X1X2 − 0.24X1X3 − 0.62X2X3 − 2.56X12 − 2.77X22 − 2.08X32 |
Y2 = 56.46 − 4.15X1 − 1.18X2 − 1.06X3 − 2.54X1X2 − 1.01X1X3 − 1.77X2X3 − 5.86X12 − 6.48X22 − 5.95X32 |
To obtain optimal extraction effects of NAR and HES, 3D response surface plots were observed. As shown in Fig. 4, all of the 3D response surface curves were upper-phase convex with maximum values, which suggested that it was reliable for the level range. Based on the RSM model, the optimal extraction process was selected with the predicted maximum extraction effects (Table 6). Upon further verification, the experimental and predicted values were in reasonable agreement, indicating that the models were reliable and significant.
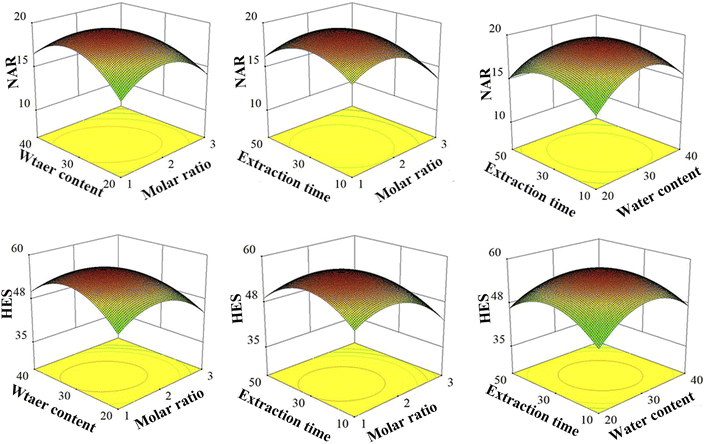 |
| Fig. 4 3D response surface plots of NAR and HES. | |
Table 6 Optimal extraction parameters for NAR and HES
|
Molar ratio |
Water content (%) |
Extraction time (min) |
Predicted values (mg g−1) |
Experimental values (mg g−1) |
NAR |
1 : 2 |
30 |
28 |
20 |
21 ± 2 |
HES |
1 : 2 |
30 |
28 |
57 |
60 ± 2 |
3.4. Recovery of flavonoids from DES extracts
The recovery of target components from DES extracts is vital and urgent. Previously, some materials, such as macroporous resins, have been applied to recover target substances from DES extracts.28 In this work, different macroporous resin (AB-8, D101, NAK-9, HPD600 and SP825) were explored for the recovery of flavonoids from DES extracts. As shown in Fig. 5, the adsorption and desorption of NAR and HES by the macroporous resin SP825 were the greatest among all the macroporous resins (higher than 80%). Thus, the macroporous resin SP825 was used as a material for the recovery of flavonoids from DES extracts and the recovery rates of NAR as well as HES reached 88 ± 3% and 86 ± 4%, respectively. Moreover, the recovered DES could extract NAR and HES for the second time, the extraction rates were 18 ± 1 mg g−1 and 53 ± 2 mg g−1.
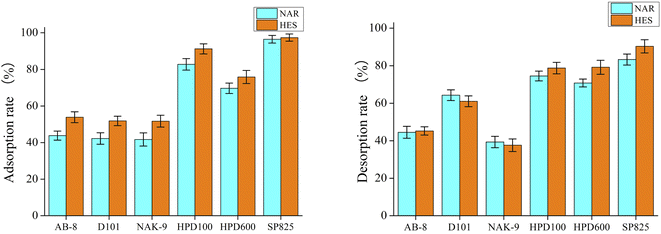 |
| Fig. 5 Adsorption and desorption diagrams of macroporous resins. | |
3.5. Comparison between traditional extraction methods
The traditional methods were compared to confirm the efficiency of flavonoid extractions using UAE. As shown in Fig. 6, the efficiency of NAR and HES extraction using UAE was higher than that of ME and PE. Meanwhile, the extraction time of UAE was significantly lesser than that of ME and PE. The results indicated that UAE was more efficient than the conventional method and possessed great potential value for the extraction of NAR and HES from CRPV.
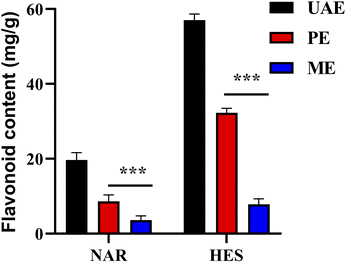 |
| Fig. 6 Comparison of different extraction methods. | |
3.6. Surface morphological characterization of CRPV
It is widely known that DES can accelerate the leaching of active substances through the disruption of plant cell walls.29 Thus, to investigate the morphological alterations of the CRPV powder, the CRPV powder and the extracts using different methods (UAE, ME, and PE) were analyzed through SEM. Fig. 7A showed the SEM image of CRPV powder without extraction, where cell walls were tightly arranged. However, the CRPV powder after UAE extraction was filled with holes and debris, indicating that a large number of cell walls were damaged (see Fig. 7B). Nevertheless, the cell surface of CRPV powder after ME and PE extraction shrank and caused slight damage (Fig. 7C and D). The above results implied that the mechanism of UAE extraction was to dissolve the active substances by destroying the plant cell wall.
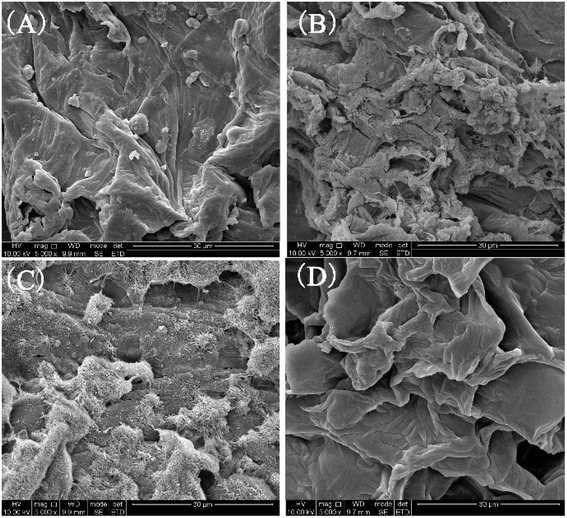 |
| Fig. 7 SEM images of green skin ((A) was unextracted CRPV powder, (B) was the CRPV powder extracted by DES method, (C) was the ME method extracted CRPV powder, (D) was the powdered CRPV extracted by PE method). | |
3.7. UPLC-Q-TOF-MS
Among the well-known techniques, UPLC-Q-TOF-MS has been developed as a reliable technique to identify the target components of natural products.30 In this study, the flavonoids in CRPV extracts were identified using the UPLC-Q-TOF-MS technique.
A total of 21 flavonoids, including two chalcones, six dihydroflavonoids, six flavonoids, and seven flavonols, were identified from CRPV extracts based on retention times, mass spectrometry excimer ion peaks, and SciFinder database, as presented in Table 7 and Fig. 8. In particular, chalcones comprised isoliquiritin apioside and isoliquiritigenin; dihydroflavonoids comprised hesperetin, naringenin, narirutin, liquiritigenin, hesperidin, and naringenin 7-O-β-D-glucopyranoside; flavonoids comprised apigenin, luteolin, tangeretin, nobiletin, genkwanin; and flavonols comprised quercetin, quercetin 3-O-neohesperidoside, myricetin, quercetin 3-O-robinobioside, kaempferide-7-glucoside, rutin, and isorhammetin. Notably, nine of these components were found for the first time in CRPV extracts, and they were quercetin 3-O-neohesperidoside, quercetin 3-O-robinobioside, petunidin, isoliquiritin apioside, liquiritigenin, isoliquiritigenin, kaempferide-7-glucoside, genkwanin, naringenin 7-O-β-D-glucopyranoside, respectively.
Table 7 The chemical constituents from CRPV
No. |
Retention time (min) |
Analyte |
Chemical structure |
Neutral mass (Da) |
Determined [M − H]− (m/z) |
Product ions (m/z) |
Mass error (mDa) |
1 |
3.51 |
Quercetin |
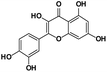 |
302.1533 |
301.1463 |
138.2652, 137.8510, 41.2310 |
−0.5 |
2 |
4.25 |
Hesperetin |
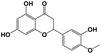 |
302.0790 |
301.0714 |
150.2851, 148.3691, 120.8501 |
−0.2 |
3 |
4.55 |
Quercetin 3-O-neohesperidoside |
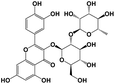 |
610.1533 |
609.1463 |
444.8574, 268.6530, 152.2010, 136.9202, 108.9541 |
0.2 |
4 |
4.73 |
Naringenin |
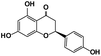 |
272.0684 |
271.0608 |
154.2134, 119.8540, 126.8412 |
−0.4 |
5 |
4.82 |
Myricetin |
 |
318.0375 |
317.0275 |
155.2341, 156.8541, 124.2845, 43.1201 |
−2.8 |
6 |
5.88 |
Narirutin |
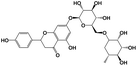 |
580.1792 |
579.1719 |
427.3851, 250.3612, 152.1025, 134.8463, 123.3601 |
−0.2 |
7 |
6.31 |
Quercetin 3-O-robinobioside |
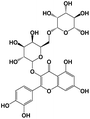 |
610.1533 |
609.1461 |
446.2150, 284.1236, 155.2140, 137.8450, 110.3201 |
1.7 |
8 |
6.47 |
Petunidin |
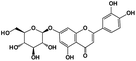 |
448.1005 |
447.0935 |
133.0219, 151.2214, 285.0511 |
0.6 |
9 |
6.64 |
Apigenin |
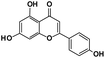 |
270.0528 |
269.0452 |
117.0256, 151.2310, 123.3265 |
−0.3 |
10 |
7.05 |
Isoliquiritin apioside |
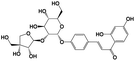 |
550.1686 |
595.167 |
417.1418, 297.0184, 255.0312, 254.0321, 135.9845, 119.7456 |
0.1 |
11 |
7.83 |
Liquiritigenin |
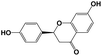 |
256.0735 |
255.0659 |
135.0023, 119.0224, 107.8112 |
−0.4 |
12 |
8.39 |
Isoliquiritigenin |
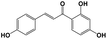 |
256.0735 |
255.0708 |
153.1214, 124.2826, 120.3210 |
−0.2 |
13 |
9.34 |
Hesperidin |
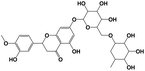 |
610.1897 |
609.1819 |
450.3211, 288.1210, 152.1236, 149.2530, 124.0121 |
−0.6 |
14 |
9.69 |
Kaempferide-7-glucoside |
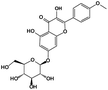 |
462.1162 |
461.1091 |
399.2354, 154.9321, 136.3820, 108.5240 |
−0.1 |
15 |
10.32 |
Rutin |
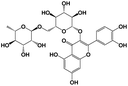 |
580.1792 |
579.1463 |
463.2744, 301.2213, 155.2181, 138.2540, 110.254 |
0 |
16 |
10.64 |
Luteolin |
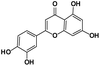 |
286.0477 |
285.0403 |
155.9123, 134.2201, 106.2134 |
−0.2 |
17 |
10.85 |
Tangeretin |
 |
372.9654 |
371.9854 |
243.9541, 133.8541, 215.5489 |
−0.3 |
18 |
11.67 |
Isorhammetin |
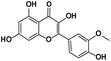 |
462.1162 |
461.0509 |
153.2018, 152.0841, 124.2816, 42.3261 |
−0.1 |
19 |
11.89 |
Nobiletin |
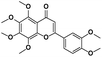 |
402.3912 |
401.2586 |
244.1234, 216.3250, 148.1020 |
1.1 |
20 |
12.25 |
Genkwanin |
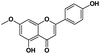 |
284.0684 |
283.0666 |
168.4562, 119.8630, 141.2111 |
0.2 |
21 |
12.51 |
Naringenin 7-O-β-D-glucopyranoside |
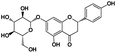 |
434.1213 |
433.1143 |
271.8540, 151.2564, 124.6590, 120.9201 |
−1.8 |
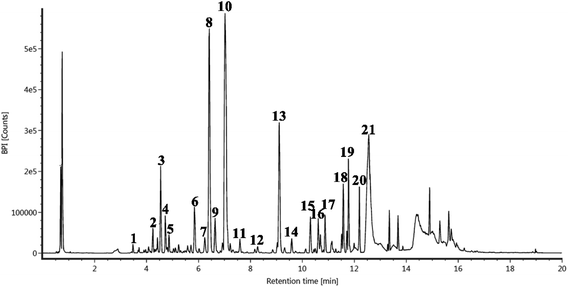 |
| Fig. 8 UPLC-Q-TOF-MS extracted chromatograms of the compounds from CRPV. | |
3.8. Method validation
Fig. 1 shows that the main active components of the CRPV extract are NAR and HES. The methodological validation of NAR and HES contributed to the quality determination of the CRPV extract. The standard equations for NAR and HES were Y = 388
91X − 80.579 and Y = 347
39X + 194.48, respectively. As listed in Table 8, the NAR and HES showed favorable linearity ranging from 5–200 μg mL−1 (R2 > 0.999).
Table 8 Method validation for NAR and HES
Analyte |
Calibration curve |
R2 |
LOD (μg mL−1) |
LOQ (μg mL−1) |
Intraday precision (n = 6)a |
Interday precision (n = 9)a |
Stability (n = 6)a |
Repeatability (n = 6)a |
Recovery (n = 9)a |
Intraday precision, interday precision, stability, repeatability and recovery are expressed as the RSD (%) of peak area. |
NAR |
Y = 388 91X − 80.579 |
0.9996 |
0.61 |
1.87 |
1.12 |
1.94 |
1.55 |
1.81 |
1.51 |
HES |
Y = 347 39X + 194.48 |
0.9993 |
0.58 |
1.69 |
0.77 |
1.95 |
1.46 |
1.68 |
1.62 |
The intraday precision was investigated by repeating the same sample test six times in one day, and interday precision was investigated by measuring the same sample for three consecutive days (three replicate injections per injection). The stability of the DES was analyzed by conducting the sample tests at 0, 2, 4, 8, 12, and 24 h. The reproducibility of the experiment was determined by repeating the preparation of six samples on the same day. Three concentration grades (80%, 100%, and 120%) of NAR and HES standards were spiked into nine samples to evaluate their recovery rates. The result revealed that the method exhibited good precision (RSD < 2%, Table 8). In addition, the stability result showed that the samples were stable at 25 °C for 12 h (RSD < 2%). Moreover, the RSD of reproducibility was less than two, which indicated that this method showed good reproducibility. Furthermore, the recovery rate, limits of detection, and limits of quantification of the method are presented in Table 8. The above results indicated that the method was fit for determining NAR and HES in CRPV extract.
3.9. Antioxidant activity
To explore natural antioxidants, three methods (DPPH, ABTS, and hydroxyl radical scavenging assay) were adopted to evaluate the antioxidant activity of flavonoid extracts. Fig. 9A–C showed that the flavonoids extract exhibited relatively strong DPPH, ABTS, and hydroxyl radical scavenging abilities at a concentration of 0.2–1.0 mg mL−1. At 1.0 mg mL−1, the DPPH, ABTS, and hydroxyl radical scavenging rates of the samples were maximum at 71 ± 2%, 96 ± 2%, and 62 ± 2%, respectively. Hence, the samples exhibited great scavenging rates on DPPH, ABTS, and hydroxyl radicals.
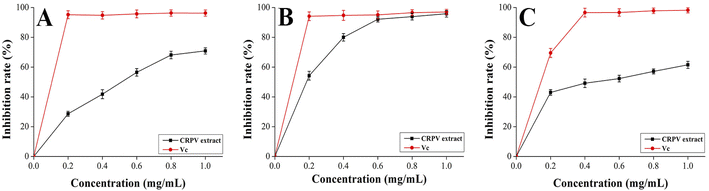 |
| Fig. 9 Antioxidant activities of flavonoids extract ((A) DPPH radical scavenging activity, (B) ABTS radical scavenging activity, (C) hydroxyl radical scavenging activity). | |
3.10. Cytotoxic activity
Cancer has become a critical disease impacting the health of humans across the world.31 To find natural cytotoxic agents, the cytotoxic activity of flavonoid extracts was further investigated using the MTT method. The influence of flavonoid extract on the viability of the six cancer cells was presented in Fig. 10. As extract concentration increased from 0 μg mL−1 to 500 μg mL−1, cancer cell viability decreased significantly for HCT-116, HT-29, CT-26, MCF-7 and HepG2 cells. In particular, for HT-29 cell line, cell survival was below 50% when the extract concentration was 100 μg mL−1. However, it is not significant for the inhibition of A549 cancer cells when the extract concentration was increased from 0 μg mL−1 to 50 μg mL−1, but while the sample concentration continued to increase, the cell survival rate decreased significantly. All the results showed that the flavonoid extract from CRPV has a good inhibitory effect on the six cancer cells selected in the experiment.
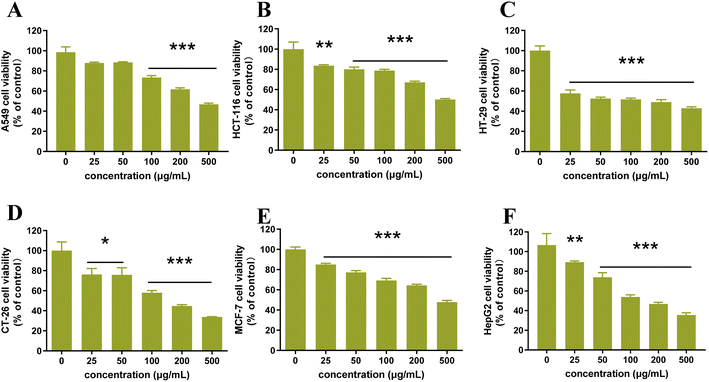 |
| Fig. 10 Antitumor activity of flavonoids extract ((A) A549 cell, (B) HCT-116 cell, (C) HT-29 cell, (D) CT-26 cell, (E) MCF-7 cell, (F) HepG2 cell). | |
4. Conclusion
In this paper, a novel, green, and efficient method with DES was developed and successfully applied to extract flavonoids from CRPV. DES-20 (proline
:
urea) was screened as the preferred extraction solvent, and it was green, safe, and meets the development needs of green chemistry. The single-factor and RSM experiments were employed to obtained the optimal extraction conditions with the extraction yields of NAR and HES as index. Meanwhile, the UAE–DES method has various advantages including short time-consuming, solvent saving, environmental protection, etc. compared to conventional extraction methods (ME and PE). In addition, UAE–DES was able to disrupt the plant cell wall to enhance the solubilization of active ingredients, as revealed via SEM characterization. Moreover, 21 flavonoids were detected using the UPLC-Q-TOF-MS technique and nine of them were newly identified in CRPV extract. Furthermore, HPLC methods were used simultaneously to determine NAR and HES, and the macroporous resin SP825 was used to recover flavonoids from DES extracts. Finally, CRPV extracts exhibited positive antioxidant and cytotoxic activities. Hence, the DES as an extractant was developed and implemented successfully for the extraction of flavonoids in CRPV.
Data availability
Research data are not shared.
Conflicts of interest
The authors declare that they have no known competing financial interests or personal relationships that could have appeared to influence the work reported in this paper.
Acknowledgements
This work was supported by the 2018 National Science Foundation of China 81860697, Zunyi Talent Team Project (2020) No. 10(E-361), Guizhou Province Science and Technology Plan Project [2020]4Y086.
References
- H. G. Jia, Y. Z. Zhang and V. Orbovic, et al., Genome editing of the disease susceptibility gene CsLOB1 in citrus confers resistance to citrus canker, Plant Biotechnol. J., 2017, 15(7), 817–823 CrossRef CAS.
- L. F. Sun and K. F. Z. Nasrullah, et al., Citrus Genetic Engineering for Disease Resistance: Past, Present and Future, Int. J. Mol. Sci., 2019, 20(21), 5256 CrossRef CAS PubMed.
- C. Y. Zhou, The status of citrus Huanglongbing in China, Tropical Plant Pathology, 2020, 45(3), 279–284 CrossRef.
- X. F. He, S. S. Huang and M. M. Wu, et al., Simultaneous quantitative analysis of ten bioactive flavonoids in Citri Reticulatae Pericarpium Viride (Qing Pi) by ultrahigh-performance liquid chromatography and high-resolution mass spectrometry combined with chemometric methods, Phytochem. Anal., 2021, 32(6), 1152–1161 CrossRef CAS PubMed.
- Y. Wang, W. L. Cui and G. W. Pang, et al., Analyses of Physical and Chemical Compositions of Different Medicinal Specifications of CRPV by Use of Multiple Instrumental Techniques Combined with Multivariate Statistical Analysis, Molecules, 2022, 27(10), 3285 CrossRef CAS.
- S. Mitra, M. S. Lami and T. M. Uddin, et al., Prospective multifunctional roles and pharmacological potential of dietary flavonoid narirutin, Biomed. Pharmacother., 2022, 150, 112932 CrossRef CAS PubMed.
- A. Ganeshpurkar and A. Saluja, The pharmacological potential of hesperidin, Indian J. Biochem. Biophys., 2019, 56(4), 287–300 CAS.
- K. Rewatkar, D. Z. Shende and K. L. Wasewar, Modeling and Optimization of Reactive Extraction of Gallic Acid Using RSM, Chem. Eng. Commun., 2017, 204(4), 522–528 CrossRef CAS.
- B. Guldiken, D. Boyacioglu and E. Capanoglu, Optimization of Extraction of Bioactive Compounds from Black Carrot Using Response Surface Methodology (RSM), Food Analytical Methods, 2016, 9(7), 1876–1886 CrossRef.
- T. Belwal, P. Dhyani and I. D. Bhatt, et al., Optimization extraction conditions for improving phenolic content and antioxidant activity in Berberis asiatica fruits using response surface methodology (RSM), Food Chem., 2016, 207, 115–124 CrossRef CAS PubMed.
- L. Zhang, Y. H. Jiang and X. N. Pang, et al., Simultaneous Optimization of Ultrasound-Assisted Extraction for Flavonoids and Antioxidant Activity of Angelica keiskei Using Response Surface Methodology (RSM), Molecules, 2019, 24(19), 3461 CrossRef CAS PubMed.
- B. Socas-Rodriguez, M. V. Torres-Cornejo and G. Alvarez-Rivera, et al., Deep Eutectic Solvents for the Extraction of Bioactive Compounds from Natural Sources and Agricultural By-Products, Appl. Sci., 2021, 11(11), 4897 CrossRef CAS.
- P. Yu, Q. Li and Y. Feng, et al., Extraction and Analysis of Six Effective Components in Glycyrrhiza uralensis Fisch by Deep Eutectic Solvents (DES) Combined with Quantitative Analysis of Multi-Components by Single Marker (QAMS) Method, Molecules, 2021, 26(5), 1310 CrossRef CAS PubMed.
- N. F. Sukor, V. P. Selvam and R. Jusoh, et al., Intensified DES mediated ultrasound extraction of tannic acid from onion peel, J. Food Eng., 2021, 296, 110437 CrossRef CAS.
- T. Frangopoulos, A Green, Efficient Approach on Extraction of Polyphenols from Fenugreek Seeds (Trigonella foenum-graecum): DES and beta-Cyclodextrin Assisted Extraction, Waste Biomass Valorization, 2022 DOI:10.1007/s12649-022-01785-y.
- L. Sillero, R. Prado and T. Welton, et al., Extraction of flavonoid compounds from bark using sustainable deep eutectic solvents, Sustainable Chem. Pharm., 2021, 24, 100544 CrossRef CAS.
- P. L. Tang, E. Hao and Z. C. Du, et al., Polysaccharide extraction from sugarcane leaves: combined effects of different cellulolytic pretreatment and extraction methods, Cellulose, 2019, 26(18), 9423–9438 CrossRef CAS.
- X. S. Wang, Y. F. Wu and J. Li, et al., Ultrasound-assisted deep eutectic solvent extraction of echinacoside and oleuropein from Syringa pubescens Turcz, Ind. Crops Prod., 2020, 151, 112442 CrossRef CAS.
- A. Grudniewska and J. Poplonski, Simple and green method for the extraction of xanthohumol from spent hops using deep eutectic solvents, Sep. Purif. Technol., 2020, 250, 117196 CrossRef CAS.
- E. Kostic, B. Arsic and M. Mitic, et al., Optimization of the Solid-Liquid Extraction Process of Phenolic Compounds from Mulberry Fruit, Not. Bot. Horti Agrobot. Cluj-Napoca, 2019, 47(3), 629–633 CAS.
- Z. Hashemi and M. A. Ebrahimzadeh, Evaluation of three methods for the extraction of antioxidants from Vicia faba L. bean and hulls, Lat. Am. Appl. Res., 2014, 44(3), 203–208 CAS.
- J. Yang, X. Han and H. Y. Wang, et al., Comparison of metabolomics of Dendrobium officinale in different habitats by UPLC-Q-TOF-MS, Biochem. Syst. Ecol., 2020, 89, 104007 CrossRef CAS.
- Y. M. Jiang, D. K. Li and X. Q. Ma, et al., Ionic Liquid-Ultrasound-Based Extraction of Biflavonoids from Selaginella helvetica and Investigation of Their Antioxidant Activity, Molecules, 2018, 23(12), 3284 CrossRef PubMed.
- J. Lei, Y. M. Wang and W. W. Li, et al., Natural green deep eutectic solvents-based eco-friendly and efficient extraction of flavonoids from Selaginella moellendorffii: Process optimization, composition identification and biological activity, Sep. Purif. Technol., 2022, 283, 120203 CrossRef CAS.
- L. Q. Ke, H. F. Ke and Y. Liu, Preparation of Flavonoids from Loquat Flowers (Eriobotrya japonica (Thunb.) Lindl) and Investigation of Their Antioxidant Activities, Lat. Am. J. Pharm., 2020, 39(9), 1734–1738 CAS.
- H. W. Guo, S. Liu and S. F. Li, et al., Deep eutectic solvent combined with ultrasound-assisted extraction as high efficient extractive media for extraction and quality evaluation of Herba Epimedii, J. Pharm. Biomed. Anal., 2020, 185, 113228 CrossRef CAS.
- B. Sun, Y. L. Zheng and S. K. Yang, et al., One-pot method based on deep eutectic solvent for extraction and conversion of polydatin to resveratrol from Polygonum cuspidatum, Food Chem., 2021, 343, 128498 CrossRef CAS PubMed.
- X. L. Qi, X. Peng and Y. Y. Huang, et al., Green and efficient extraction of bioactive flavonoids from Equisetum palustre L. by deep eutectic solvents-based negative pressure cavitation method combined with macroporous resin enrichment, Ind. Crops Prod., 2015, 70, 142–148 CrossRef CAS.
- P. V. D. Pontes, I. A. Shiwaku and G. J. Maximo, et al., Choline chloride-based deep eutectic solvents as potential solvent for extraction of phenolic compounds from olive leaves: Extraction optimization and solvent characterization, Food Chem., 2021, 352, 129346 CrossRef PubMed.
- S. Y. Zhao, Z. L. Liu and Y. S. Shu, et al., Chemotaxonomic Classification Applied to the Identification of Two Closely-Related Citrus TCMs Using UPLC-Q-TOF-MS-Based Metabolomics, Molecules, 2017, 22(10), 1721 CrossRef.
- X. Y. Wang, X. L. Yang and J. X. Li, et al., Impact of healthy lifestyles on cancer risk in the Chinese population, Cancer, 2019, 125(12), 2099–2106 CrossRef PubMed.
|
This journal is © The Royal Society of Chemistry 2022 |
Click here to see how this site uses Cookies. View our privacy policy here.