DOI:
10.1039/D2RA04191J
(Paper)
RSC Adv., 2022,
12, 21022-21025
Metal-free synthesis of C2-quaternary indolinones by (NH4)2S2O8 mediated oxidative dearomatization of indoles†
Received
7th July 2022
, Accepted 15th July 2022
First published on 21st July 2022
Abstract
An efficient metal-free, (NH4)2S2O8 mediated oxidative dearomatization of indoles for the construction of C2-quaternary indolinones was disclosed. A series of C2-quaternary indolinones derivatives with good functional group tolerance were obtained in moderate to excellent yields. This methodology provides an alternative approach for the direct generation of all-carbon quaternary centers at the C2 position of indoles. This catalytic approach represents a step-economic and convenient strategy for the oxidative dearomatization of indoles.
Introduction
N-Bonded heterocycles are privileged moieties in the molecular skeleton of various natural products and pharmaceuticals.1 Indole and its derivatives are important compounds, a subset of N-bonded heterocycles, are widespread in nature and exhibit significant biological activity2 and oxindole derivatives (Fig. 1) are known to possess a variety of biological activities.3 Oxidative dearomatization reaction of indole leads to the formation of a diverse class of products such as 2/3-oxoindoles, indirubin, indigo, isatin and indoline derivatives.4 Among them, the transformation of indoles to C2/C3-quaternary indolinone derivatives is synthetically quite valuable since it converts structurally simpler, planar indole skeleton to a complex three-dimensional architecture. For example, 2-(1H-indol-3-yl)-2,3′-biindolin-3-one 1 was isolated as the product of indole oxidation by a strain of Claviceps purpurea.5 This compound has also been characterised from natural (bacterial) sources such as Vibrio parahaemolyticus6 and Haemophilus influenzae.7 In addition, isatisine A 2, an oxindole system having indole 2-substituents, is present in the roots and leaves of Isatis indigotica Fort (Cruciferae). This biennial herbaceous plant is widely cultivated in China and East Asia for the prevention and treatment of viral diseases such as influenza, viral pneumonia, mumps, and hepatitis.8 Therefore, we believe that synthesis of 2,2-disubstituted indolin-3-ones derivatives is valuable.
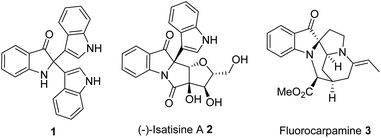 |
| Fig. 1 Some important bioactive synthetic derivatives with 2,2-disubstituted indolin-3-one structural unit. | |
In 2012, Liu and coworkers reported the TEMPO [(2,2,6,6-tetramethylpiperidin-1-yl)oxyl] mediated synthesis of C2-quaternary indolinones from free (NH)-indoles (Scheme 1a).9 However, the reaction required three days and the high loading of TEMPO for completion. Subsequently, in 2013, Liu and coworkers reported a tandem oxidative homocoupling reaction for the generation of all-carbon quaternary centers at the C2 position of indoles by using NaNO2 with CH3SO3H in pyridine (Scheme 1b).10 In 2018, Ganesan and coworkers reported one pot oxidative dearomatization reaction of indole leading to the formation of the corresponding C2/C3-quaternary indolinones (Scheme 1c).11 Free (NH)-indoles gave C2-quaternary indolinone derivatives whilst (NR)-indoles yielded C3-quaternary indolinones as the major product. In 2020, Thakur and coworkers reported ‘on-water’ synthesis of 2,2-bis(indoly-3-yl)indoline-3-ones via N2-selective dearomatization of ‘(N–H) protection-free’ indole derivatives (Scheme 1d).12 To the best of our knowledge, there is only limited precedent are available for the one-pot transformation of indole to the corresponding C2 quaternary indolinone derivatives, with no reported study for (NH4)2S2O8-catalysed methodologies. One relevant piece of work is by Ganesan et al. (Scheme 1), who described the TBHP reagent system to convert free indoles to the corresponding C2-quaternary indolinone derivatives.11 Therefore, development of methods to meet the challenges of optimum reaction conditions would significantly enhance the utility of 2,2-diaryloxindoles. Herein, we describe successful implementation of the strategy shown in Scheme 1, which affords 2,2-bis(indoly-3-yl)indoline-3-ones from indoles using (NH4)2S2O8 in DMF as oxidant.
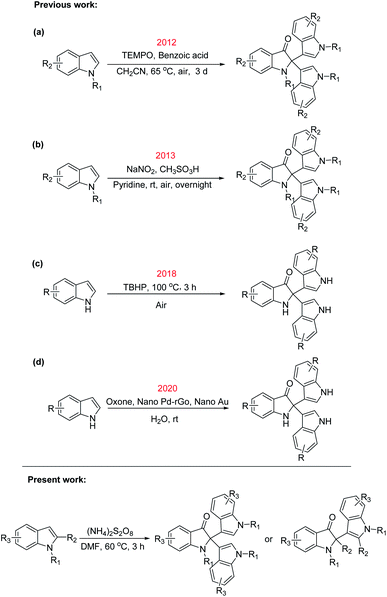 |
| Scheme 1 Previous and present approaches for the synthesis of 2,2-diaryloxindole (R = alkyl or halide group). | |
Results and discussion
The reaction conditions were firstly optimized by 1-methyl-1H-indole 1a as the template substrates. As shown in Table 1, the model reaction employed (NH4)2S2O8 as oxidant in DMF (2 ml) as the solvent at 60 °C for 3 h, giving the desired product of 2,2-bis(indoly-3-yl)indoline-3-ones 2a in a yield of 89% (entry 1). Subsequently, the effects of different oxidants on the reaction were investigated. For instance, K2S2O8, oxone, H2O2, and TBHP were applied as oxidants for the one-pot reaction, respectively (entries 2–5). Among those, (NH4)2S2O8 displayed the highest activity, and the yield of 2a was 89% (entry 1). In addition, 4a was not observed in the absence of the oxidant, suggesting the importance of (NH4)2S2O8 as oxidant (entry 6). Increasing or decreasing the amount of (NH4)2S2O8 resulted in the decrease of yield (entry 7 and 8). Next, the solvent was screened. DMF was proved to be more efficient than others, such as DCM, DCE, PhCH3, MeCN, DMSO or EtOH (entries 9–14). Changing the amount of DMF led to the reduced of the product yield (entries 15 and 16). The heating effect was also evaluated for this reaction and the reaction led to maximum yield at 60 °C while increasing or decreasing the temperature was not fruitful for this process (entry 17 and 18). Further investigation of the reaction for a shorter or longer time period (1 or 5 h) did not give a higher yield of 2a (entries 19 and 20). Finally, the approach employed (NH4)2S2O8 as oxidant in DMF (2 ml) as the solvent at 60 °C for 3 h, giving the desired product of 2,2-bis(indoly-3-yl)indoline-3-ones 2a in a yield of 89% (entry 1).
Table 1 Optimization of the reaction conditionsa
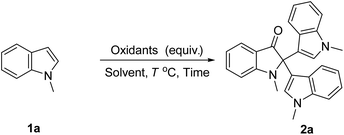
|
Entry |
[Cat.] |
Solvent |
Temp. (°C) |
Yieldb (%) |
Reaction conditions: 1-methyl-1H-indole 1a (0.3 mmol), [oxidants] (equiv.), solvent (mL), T °C, air tube. Isolated yields. N.D. = no detected. NR means not reaction. Reaction for 1 h. Reaction for 5 h. H2O2: 30% aqueous solution. TBHP: 70% aqueous solution. |
1 |
(NH4)2S2O8 (2) |
DMF (2) |
60 |
89 |
2 |
K2S2O8 (2) |
DMF (2) |
60 |
72 |
3 |
Oxone (2) |
DMF (2) |
60 |
38 |
4 |
H2O2 (2) |
DMF (2) |
60 |
NDc |
5 |
TBHP (2) |
DMF (2) |
60 |
NRd |
6 |
No |
DMF (2) |
60 |
NR |
7 |
(NH4)2S2O8 (1) |
DMF (2) |
60 |
46 |
8 |
(NH4)2S2O8 (3) |
DMF (2) |
60 |
52 |
9 |
(NH4)2S2O8 (2) |
DCM (2) |
60 |
Trace |
10 |
(NH4)2S2O8 (2) |
DCE (2) |
60 |
10 |
11 |
(NH4)2S2O8 (2) |
PhCH3 (2) |
60 |
22 |
12 |
(NH4)2S2O8 (2) |
MeCN (2) |
60 |
ND |
13 |
(NH4)2S2O8 (2) |
DMSO (2) |
60 |
NR |
14 |
(NH4)2S2O8 (2) |
EtOH (2) |
60 |
71 |
15 |
(NH4)2S2O8 (2) |
DMF (1) |
60 |
60 |
16 |
(NH4)2S2O8 (2) |
DMF (3) |
60 |
32 |
17 |
(NH4)2S2O8 (2) |
DMF (2) |
40 |
42 |
18 |
(NH4)2S2O8 (2) |
DMF (2) |
80 |
45 |
19e |
(NH4)2S2O8 (2) |
DMF (2) |
60 |
28 |
20f |
(NH4)2S2O8 (2) |
DMF (2) |
60 |
50 |
After achieving the optimized conditions, a series of substituted indole derivatives were tested for the oxidative dearomatization (Table 2). As summarized in Table 2, the reaction was compatible with a variety of indole moieties (1a–1u) bearing electron-donating and electron-withdrawing substituents to produce the desired C2-quaternary indolinones products (2a–2u) in moderate to good yields (73–89%). The structure of the desired C2-quaternary indolinones 2a was confirmed by X-ray analysis. Notably, the gram-scale synthesis afforded 1.05 g of 2a in 86% yield. To our delight, the reaction also showed good compatibility with a wide range of valuable functional groups such as chloro (2n and 2r) and bromo (2o and 2u). Tolerance to the halogen atoms was noteworthy since they have been frequently used for further modification. N-Alkylindoles can smoothly proceed to give the corresponding products in good yields (2a–2h). The 2-methylindoles and 2-phenylindoles gave the corresponding unsymmetrically substituted C2-quaternary indolinone product 2i–2l in 74–80% yield. Moreover, we were pleased to find that the position of the substituent on the indole moiety showed no obvious influence on the reaction outcome, and substitutions at the C5-(2m–2q), C6-(2r and 2s), or C7-(2t and 2u) were all well tolerated in the reaction.
Table 2 Scope for indolesa
Reaction conditions: 1 (0.3 mmol), (NH4)2S2O8 (2 equiv.), DMF (2 mL), 60 °C, air tube for 3 h. Isolated yields. In a 9 mmol scale. |
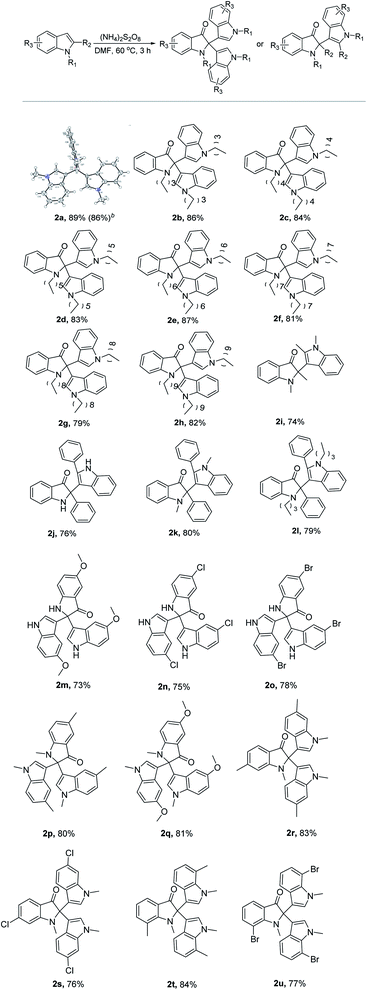 |
On the basis of substrate diversity and previous reports,13 the attack of the (NH4)2S2O8 onto the nucleophilic centre C-3 of indole (1a) generates intermediate (B) in situ through the various intermediate compounds (Scheme 2). As depicted in Scheme 2, the electrophilic intermediate (B) easily facilitates nucleophilic attack by the indole molecule (1a) to form intermediate compound C on oxidation condition. Subsequently, the electrophilic intermediate (C) easily facilitates nucleophilic attack by the indole molecule (1a) to form the C2-quaternary indolinone 2a.14
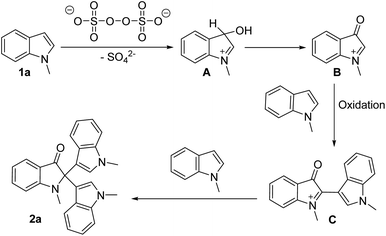 |
| Scheme 2 Plausible reaction mechanism. | |
Conclusions
In conclusion, we have successfully demonstrated an efficient metal-free, (NH4)2S2O8 mediated oxidative dearomatization of indoles for the construction of C2-quaternary indolinones derivatives in moderate to good yields. The significant aspects of our work allows modest functional group tolerance, were compatible under the current methodology. This methodology provides an alternative approach for the direct generation of all-carbon quaternary centers at the C2 position of indoles. This catalytic approach represents a step-economic and convenient strategy for the oxidative dearomatization of indoles.
Conflicts of interest
There are no conflicts to declare.
Acknowledgements
We are grateful for the financial support from the project supported by Chengdu Normal University Project (2021CS21ZCY02) and National undergraduate training program for innovation and entrepreneurship (Grant No. 202114389016 and 202114389005), the Foundation of Chengdu Normal University Talent Introduction Research Funding (2021YJRC202020).
Notes and references
-
(a) E. Vitaku, D. T. Smith and J. T. Njardarson, Analysis of the structural diversity, substitution patterns, and frequency of nitrogen heterocycles among US FDA approved pharmaceuticals: miniperspective, J. Med. Chem., 2014, 57, 10257–10274 CrossRef CAS PubMed;
(b) R. D. Taylor, M. MacCoss and A. D. Lawson, Rings in drugs: Miniperspective, J. Med. Chem., 2014, 57, 5845–5859 CrossRef CAS PubMed.
-
(a) R. Sundberg, Indoles, Academic Press, San Diego, 1996, p. 113 Search PubMed;
(b) S. Cacchi and G. Fabrizi, Synthesis and functionalization of indoles through palladium-catalyzed reactions, Chem. Rev., 2005, 105, 2873–2920 CrossRef CAS PubMed;
(c) L. Joucla and L. Djakovitch, Transition Metal-Catalysed, Direct and Site-Selective N1-, C2-or C3-Arylation of the Indole Nucleus: 20 Years of Improvements, Adv. Synth. Catal., 2009, 351, 673–714 CrossRef CAS;
(d) M. Bandini and A. Eichholzer, Catalytic functionalization of indoles in a new dimension, Angew. Chem., Int. Ed., 2009, 48, 9608–9644 CrossRef CAS PubMed;
(e) G. Bartoli, G. Bencivenni and R. Dalpozzo, Organocatalytic strategies for the asymmetric functionalization of indoles, Chem. Soc. Rev., 2010, 39, 4449–4465 RSC.
-
(a) A. Gazit, N. Osherov, I. Posner, P. Yaish, E. Poradosu, C. Gilon and A. Levitzki, Tyrphostins. II. Heterocyclic and. alpha.-substituted benzylidenemalononitrile tyrphostins as potent inhibitors of EGF receptor and ErbB2/neu tyrosine kinases, J. Med. Chem., 1991, 34, 1896–1907 CrossRef CAS PubMed;
(b) S. Dilber, M. Saban, J. Jelaca, A. Gelineo, L. Arsenijević and M. Bogavac, Investigation of antimicrobial activity of some isatin derivatives, Pharmazie, 1989, 44, 649–650 CAS;
(c) A. Wetzel and F. Gagosz, Gold-Catalyzed Transformation of 2-Alkynyl Arylazides: Efficient Access to the Valuable Pseudoindoxyl and Indolyl Frameworks, Angew. Chem., Int. Ed., 2011, 123, 7492–7496 CrossRef;
(d) C. V. S. Kumar, V. G. Puranik and C. V. Ramana, InCl3-Mediated Addition of Indole to Isatogens: An Expeditious Synthesis of 13-deoxy-Isatisine A, Chem.–Eur. J., 2012, 18, 9601–9611 CrossRef CAS PubMed.
- M. Linhares, S. L. Rebelo, M. M. Simoes, A. M. Silva, M. G. P. Neves, J. A. Cavaleiro and C. Freire, Biomimetic oxidation of indole by Mn (III) porphyrins, Appl. Catal., 2014, 470, 427–433 CrossRef CAS.
- R. Bell, S. Carmeli and N. Sar, Vibrindole A, a metabolite of the marine bacterium, Vibrio parahaemolyticus, isolated from the toxic mucus of the boxfish Ostracion cubicus, J. Nat. Prod., 1994, 57, 1587–1590 CrossRef CAS PubMed.
- A. Gazit, N. Osherov, I. Posner, P. Yaish, E. Poradosu, C. Gilon and A. Levitzki, Tyrphostins. II. Heterocyclic and. alpha.-substituted benzylidenemalononitrile tyrphostins as potent inhibitors of EGF receptor and ErbB2/neu tyrosine kinases, J. Med. Chem., 1991, 34, 1896–1907 CrossRef CAS PubMed.
- T. L. Stull, L. Hyun, C. Sharetzsky, J. Wooten, J. P. McCauley and A. B. Smith, Production and Oxidation of Indole by Haemophilus influenzae, J. Biol. Chem., 1995, 270, 5–8 CrossRef CAS PubMed.
-
(a) J.-F. Liu, Z.-Y. Jiang, R.-R. Wang, Y.-T. Zheng, J.-J. Chen, X.-M. Zhang and Y.-B. Ma, Isatisine A, a novel alkaloid with an unprecedented skeleton from leaves of Isatis indigotica, Org. Lett., 2007, 9, 4127–4129 CrossRef CAS PubMed;
(b) J. Lee and J. S. Panek, Total synthesis of (+)-isatisine A, Org. Lett., 2011, 13, 502–505 CrossRef CAS PubMed;
(c) H.-Z. Zheng, Z.-H. Dong and Q. Yu, Modern Study of Traditional Chinese Medicine, Xueyaun Press, Beijing China, 1997, vol. 1, pp. 328–334 Search PubMed.
-
(a) W.-B. Qin, Q. Chang, Y.-H. Bao, N. Wang, Z.-W. Chen and L.-X. Liu, Metal-free catalyzed oxidative trimerization of indoles by using TEMPO in air: a biomimetic approach to 2-(1H-indol-3-yl)-2, 3′-biindolin-3-ones, Org. Biomol. Chem., 2012, 10, 8814–8821 RSC;
(b) Y.-B. Kong, J.-Y. Zhu, Z.-W. Chen and L.-X. Liu, Copper-catalyzed oxidative trimerization of indoles by using TEMPO to construct quaternary carbon centers: the synthesis of 2-(1H-indol-3-yl)-2, 3′-biindolin-3-ones, Can. J. Chem., 2014, 92, 269–273 CrossRef CAS.
- J. Xue, Y. Bao, W. Qin, J. Zhu, Y. Kong, H. Qu, Z. Chen and L. Liu, Metal-Free–Catalyzed Oxidative Trimerization of Indoles Using NaNO2 to Construct Quaternary Carbon Centers: Synthesis of 2-(1H-Indol-3-yl)-2, 3′-biindolin-3-ones, Synth. Commun., 2014, 44, 2215–2221 CrossRef CAS.
- J. Kothandapani, S. M. K. Reddy, S. Thamotharan, S. M. Kumar, K. Byrappa and S. S. Ganesan, TBHP Mediated Substrate Controlled Oxidative Dearomatization of Indoles to C2/C3-Quaternary Indolinones, Eur. J. Org. Chem., 2018, 2018, 2762–2767 CrossRef CAS.
- S. B. Gohain, M. Basumatary, P. K. Boruah, M. R. Das and A. J. Thakur, Nano Au/Pd-catalysed ‘on-water’synthesis of C3–C3′ diaryl-oxindole scaffolds via N 2-selective dearomatization of indole, Green Chem., 2020, 22, 170–179 RSC.
-
(a) S. Meenakshisundaram and N. Sarathi, Kinetics and mechanism of oxidation of indole by HSO, Int. J. Chem. Kinet., 2007, 39, 46–51 CrossRef CAS;
(b) See ref. 11..
- A. Bahuguna, A. Kumar, S. Kumar, T. Chhabra and V. Krishnan, 2D-2D nanocomposite of MoS2-graphitic carbon nitride as multifunctional catalyst for sustainable synthesis of C3-functionalized indoles, ChemCatChem, 2018, 10, 3121–3132 CrossRef CAS.
|
This journal is © The Royal Society of Chemistry 2022 |