DOI:
10.1039/D2RA03882J
(Review Article)
RSC Adv., 2022,
12, 25977-25991
An overview of biomedical applications of choline geranate (CAGE): a major breakthrough in drug delivery
Received
23rd June 2022
, Accepted 28th August 2022
First published on 13th September 2022
Abstract
A number of studies are on the way to advancing the field of biomedical sciences using ionic liquids (ILs) and deep eutectic solvents (DESs) in view of their unique properties and inherent tunability. These significant solvents tend to enhance the physical properties of the drug, increase their bioavailability and promote the delivery of recalcitrant drugs to the body. One such widely investigated tempting multipurpose IL/DES system is choline geranate (CAGE), which has gained significant interest due to its biocompatible and highly potent antiseptic behavior, which also facilitates its sanitizing ability to combat the coronavirus. This review focuses on total advancements in biomedical applications of CAGE. This biocompatible IL/DES has made facile the solubilization of hydrophobic and hydrophilic drugs and delivery of intractable drugs through physiological barriers by stabilizing proteins and nucleic acids. Therefore, it has been used as a transdermal, subcutaneous, and oral delivery carrier and as an antimicrobial agent to treat infectious diseases and wounds as approved by laboratory and clinical translations. Moreover, current challenges and future outlooks are also highlighted to explore them more purposefully.
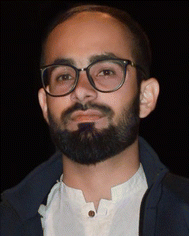 Mubeshar Riaz | Mubeshar Riaz earned his BSc degree in Chemistry from Islamia University of Bahawalpur and later his MSc degree with distinction from University of Multan where he worked on organic synthesis. Currently, he is about to complete his M.Phil. degree under the supervision of Dr Maliha Uroos from the Centre for Research in Ionic Liquids (CRIL), School of Chemistry, University of the Punjab. His research project is based on synthesis of biocompatible ionic liquids and their biological activities. |
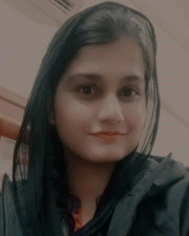 Maida Akhlaq | Maida Akhlaq pursued her Bachelor and MPhil degrees in Chemistry from School of Chemistry, University of the Punjab, Lahore. Currently, she is doing her PhD in Organic Chemistry from the same school. Along with this, she is working as Research Officer in HEC funded Technology Development Fund (TDF) project at Centre for Research in Ionic Liquids (CRIL) with Dr Maliha Uroos. Her research interests are entailing broad canvas from synthesis of organic compounds to the preservation of environment from lethal chemicals. Her recent project circumscribes pretreatment and processing of lignocellulosic waste materials and their conversion into ecofriendly and value-added chemicals. |
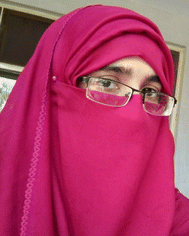 Sadia Naz | Sadia Naz obtained her BS Chemistry (4 years) in 2016 from Lahore College for Women University, Lahore, where she worked on synthesis of organic compounds. Her next research interest was ionic liquids assisted processing of lignocellulosic biomass, which she pursued during her MPhil studies (2016–18) at School of Chemistry, University of the Punjab. Currently, she is a final year PhD candidate at Centre for Research in Ionic Liquids, School of Chemistry, University of the Punjab (2018 to onwards) and is also working as a research assistant in HEC NRPU project. She is working on the synthesis of less-toxic and cost-effective ionic liquids and sustainable conversion of crop residues into industrially important platform chemicals using ionic liquids. |
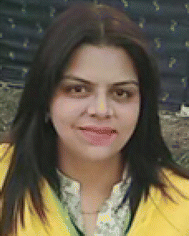 Maliha Uroos | Dr. Maliha Uroos obtained her MSc (Chemistry) in 2003 from School of Chemistry, University of the Punjab. She completed her PhD in synthetic organic chemistry in 2012 from the University of Nottingham under HEC Faculty Development Scholarship. She was awarded EPSRC postdoctoral fellowship (2012–13) at the University of Nottingham, UK. She was appointed as a lecturer at the University of Punjab in 2007 and is currently working as an associate professor (2020 to date) and Director of Centre for Research in Ionic Liquids, School of Chemistry, University of the Punjab. She has received the following awards: President Talent Forming Scholarship (2000), PhD Scholarship (2008), Leslie Crombie Prize UK for best PhD student (2011), and EPSRC Fellowship (2012). She has secured two major research grants from HEC under NRPU and TDF. She has several publications in leading international journals including patents and presented her work at various national and international conferences. Her main focus is synthetic organic chemistry, and research interests include synthesis and applications of ionic liquids, synthesis of biologically active molecules, sustainable energy production, green chemistry protocols, and nanochemistry. |
1. Introduction
In today's world, people suffer from plethora of diseases in tandem with the challenges in curing them. Scientists are searching, thoroughly, for a beacon to nullify all chronic illnesses. Insights into the human genome and a surge in scientific development have encouraged the use of biologics. Their usage can be estimated from the fact that these biologics contributed to one-third of the newly recommended drugs in the last decade.1 The problem is that many drug candidates have low solubility in FDA (Food and Drug Administration) approved solvents as well as biological fluids, leading to limited bioactivity and clinical applications.2 It is the need of the hour to confront new approaches to enhance the effective usage of drugs by addressing this solubility issue. In this context, biological ionic liquids or deep eutectic solvents with their application-driven investigations have become vibrant and emerging frontiers in therapeutics, especially in drug delivery. They efficiently deliver or solvate a wide range of components in the body. The novel concept of active pharmaceutical ingredient-ionic liquids (API-ILs) has been developed to instill biologically active anions or cations into the structure of ionic liquid to make it more effective for its biological applications. API-ILs possess high solvation power for hydrophobic/hydrophilic solid-drug ingredients that are insoluble in water and other solvents. Solvation, in turn, increases their bioavailability and facilitates the route of delivery and the mode of action.
From all the API-ILs synthesized and tested so far for drug delivery applications, choline geranate (CAGE) is leading over the others due to its biocompatible nature; CAGE-based deep eutectic solvents are in high demand as clinical agents due to their safety and superior efficacy. They are highly safe for biological use, as evident by their history. The first ingredient choline has been used as a pregnancy supplicant as it provides support to fetal development and inhibits organ malfunction. The second ingredient, geranic acid is ubiquitously present in tea extracts. While, mutually, CAGE has a wide range of possibilities in recalcitrant infections of the body, such as infections in the lungs, skin, mucosal membranes, and oral cavity.3
Owing to its outstanding profile, its toxicity is extremely low to negligible,3,4 and it is enriched with a number of therapeutic properties such as antimicrobial activity against drug-resistant bacteria, fungi, and viruses and improvement in the transdermal delivery of both hydrophobic and hydrophilic molecules.5 The synthesis of CAGE with a ratio of choline and geranic acid 1
:
2 has proven to be highly efficient for the applications of various drugs in the laboratory and in the clinic.4,6 It has been used as an antibiotic, antiseptic and antifungal agent for transdermal, oral, and subcutaneous deliveries to treat various diseases and wounds. Moreover, its clinical translation encourages its usage in pharmaceutical formulations.
Here, we present CAGE as a potential multipurpose expedient in drug delivery and treatment of numerous infections. Further, we have discussed its different activities as a drug delivery carrier and as an antimicrobial agent. We are well aware of many excellent reviews on the use of ILs in drug delivery.7–9 But to the best of our knowledge, there is no review existed in biomedical applications of CAGE. Fig. 1 represents the growing interest and scientific contributions for biomedical applications of CAGE; the annual number of publications is presented from 2014 to date.
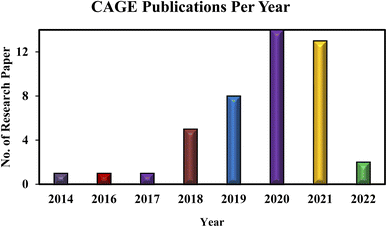 |
| Fig. 1 The annual number of publications on CAGE since 2014 using the search term ‘Choline geranate (CAGE)’ and further refined by the word ‘CAGE biomedical applications’, dated 25 May 2022. | |
2. Synthesis of CAGE
In the laboratory, CAGE has been synthesized by salt metathesis reaction using commercial and GRAS (Generally Recognized as Safe) present in the list of FDA (Food and Drug Administration) reagents; choline bicarbonate and geranic acid. Choline is a water-soluble crucial nutrient present in the liver and in phospholipids redundant in cell membranes, while geranic acid occurs in lemon grass and is used as a flavoring agent.10
CAGE is basically a deep eutectic solvent (DES) and/or ionic liquid (IL) formed at room temperature (RTIL) and is considered a promising material for active pharmaceutical ingredients (API) and additive formulations.11,12 DES comprises properties in correspondence with conventional ILs. Whether it is RTIL or DES, is determined by the ratio of both of its components in the mixture. Neat ILs or RTILs are organic salts, having a 1
:
1 component ratio of cations and anions while DES are prepared by varying ratios of cation of charged or neutral species. Fig. 2 presents the synthesis of CAGE mostly reported in the literature by 1
:
1 and 1
:
2 combination ratios of choline and geranic acid and are termed RTIL and DES, respectively.13,14
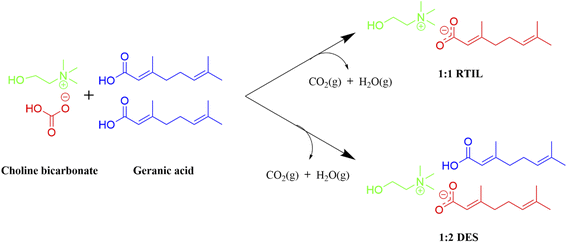 |
| Fig. 2 Schematic diagram of CAGE synthesis; 1 : 1 molar ratio choline bicarbonate and geranic acid (RTIL) and 1 : 2 molar ratio choline bicarbonate and geranic acid (DES). | |
3. Physicochemical properties of CAGE
CAGE comprises primarily ionic species and the properties of its composition depend nonmonotonically on the complex interaction between ionic and neutral species of different ratios of choline and geranic acid. The most effective 1
:
2 ratio of CAGE has a viscosity 569 ± 19 mPa s, conductivity 13.79 ± 0.28 mS m−1 with diffusion coefficient 2.2 × 10−12 m2 s−1.6 Moreover, CAGE has a molecular weight 440.32 g mL−1 with a density 0.989
:
0.001 g mL−1 at 25 °C.15,16
4. Bioactive potential of CAGE
As CAGE is synthesized using biologically active precursors, it is a highly biologically active system. It has shown significant antimicrobial activities against microbes and pathogens. It has been employed in the preparation of antiseptic agents against bacterial biofilms,3 in wound healing scaffolds against bacterial colonies,17 as an antifungal drug carrier, and in the preparation of long-lasting sanitizers to prevent coronavirus and other viral infections.18 All these bioactivities and biological applications are thoroughly penned in subsequent sections.
4.1. Antimicrobial potential
CAGE-based IL/DES systems show high antimicrobial activities not only against bacteria but also, against fungi19 and viruses.18 It is highly active against microbes clinically isolated from Candida albicans, Staphylococcus aureus and Mycobacterium tuberculosis as well as laboratory strains of herpes simplex virus. Studies performed on human keratinocytes and mice show an iota of CAGE toxicity with enhanced efficacy/toxicity ratio compared to currently used antiseptics.3
Antiseptics are the most important tools against bacterial biofilms. In hospitals, they are commonly used for disinfecting and cleaning.20 In April 2019, the U.S. FDA listed 28 of 31 ingredients for re-evaluation prior to use in antiseptics. As these ingredients play a crucial role, the development of efficient antiseptics is now on top of the list. Potent antiseptics should be investigated that can deal with biofilms21 having a shield or protective layer of exopolymeric substances (EPS) consisting of polysaccharides, nucleic acids, humic acids, and peptides.22,23 Thorough knowledge of antibiotic action is crucial to developing highly efficient antiseptic agents to infuse resistance in microbes.
CAGE has a unique property of skin penetration due to its biocompatible nature and can deliver antibiotics, effectively. The penetrating power of CAGE is not limited to just dermis, but it can treat deep skin pathogens, exhibiting the potential to treat an infection such as Propionibacterium acnes, as observed through laboratory experiments. These data clearly confirm its therapeutic and antiseptic applications.3
CAGE showed efficient elimination of biofilms at low concentrations (3.65 mM) in just 2 hours. Thus, it exhibits a high capacity for biofilm removal compared to the other reported antibacterial agents. When this time-kill study was applied to one-day-old Staphylococcus aureus biofilms, 0.1% CAGE solution was observed to efficiently reduce the vulnerability of biofilms by three orders of magnitude in 15 minutes. While the outer polymeric layer of the biofilms was ruptured. By mechanism, the ions of geranate and geranic acid in CAGE are predicted to penetrate pathogenic membranes by perturbing membrane homeostasis, as predicted by molecular modeling studies.24 In the last of the 20th century, two scientists, Pernak and Skrzypczak, described a relationship between the concentration of IL (imidazolium chloride) and its effective inhibitory ratio against bacteria.25 After that, other studies followed the suit, resulting in a second mechanistic approach stating that the antibacterial activity of ILs is related to the alkyl chain length. Other researchers developed a mechanism similar to the action of pesticides and surfactants, in which an aliphatic chain gets inserted into the bacterial membrane.26 Thus, without an accurate mechanism, the development of the desired antimicrobial IL is not possible. There must be a thorough knowledge of ILs facts and properties, such as polarity, density, viscosity, hydrophobic and hydrophilic nature, and conductivity. Moreover, the treasure of ILs could be explored by understanding their tunable nature.
The mechanism of antimicrobial action of CAGE was also inferred with combined simulation and experimental techniques using 1
:
1, 1
:
2, 2
:
1, and 1
:
4 choline bicarbonate and geranic acid ratios and Gram-negative bacteria (E. coli). Fluorescence flow cytometry showed the loss of integrity of the cell membrane due to its rupture by CAGE. It was also evidenced by Fourier Transform Infrared spectroscopy (FTIR) that showed the alternation of membrane lipid profile antibacterial resistance. The disruption of the membrane is the linchpin of interactions between the ionic species. The presence of a negative charge on the outer membrane of E. coli is different from that of phospholipid bilayers and it makes the membrane vulnerable to the penetration of CAGE. The simulation results confirmed that choline efficiently screens the negative charge and facilitates the geranate or geranic acid to penetrate the membrane (Fig. 3). Among the other variants, CAGE with 1
:
4 choline bicarbonate and geranic acid has the highest penetration ability as well as the highest toxicity. It has been shown that by increasing geranic acid content, biocidal activity is also increased. This basic mechanism further stated that low CAGE concentrations (6.5–13 mM) are bacteriostatic, and the cells can flourish for a few hours at this concentration, while higher concentrations (≥26 mM) are bactericidal.4
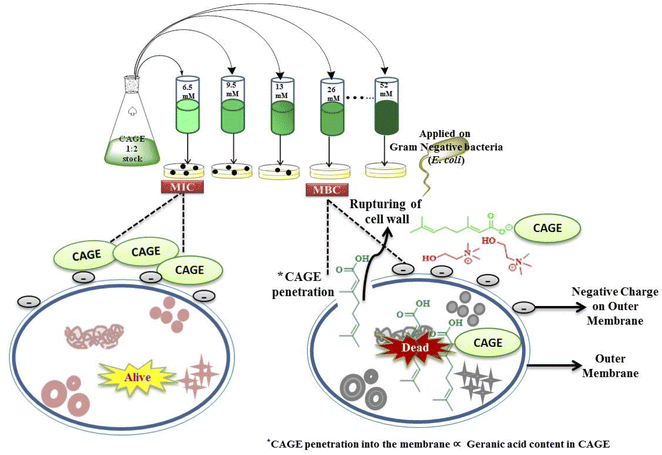 |
| Fig. 3 The mechanism of antimicrobial action of CAGE. | |
Moreover, recently in 2019, coronavirus disease (COVID-19) broke out, demanding immediate protection from infections and pathogens. Ethanol-based sanitizers are short-lived due to the rapid evaporation of ethanol. A long-lasting sanitizer has been developed using IL/DES CAGE (CG-101, 5% w/w), which is biocompatible and GRAS material, named IonLAST™. A single application of the gel protects for 4 hours for tolerance studied on human volunteers. Lab studies also confirmed its ability to keep microbes at bay for several hours. The mechanism of its antimicrobial action is the same lipid extraction and membrane disruption, as already reported in the literature,18,24 There is a need to explore more about the antiviral properties of CAGE, its pros and cons for skin application, and many more like the CAGE-based antiviral drugs.
4.2. Wound healing potential of CAGE scaffolds
Wound healing is crucial to protect the tissues from foreign agents and pathogens, which could be dangerous to the patient.27 Pathogens can form microbial biofilms on and in the compromised tissue that is vulnerable to bacterial colonies.28 These microbial biofilms could lead to chronic infections44 and are quite resistant towards antibiotic therapy29,30. In 2018, chronic wounds have affected 8.2 million Medicare beneficiaries. According to an estimate, the cost of wound care in the United States ranges from 31.7 to $96.8 billion, solely for Medicare beneficiaries.31 Recently, electrospun scaffolds containing CAGE have come in trend to diminish the bacterial colonies, efficiently.32 Opportunistic bacterial pathogens are the main cause to hamper the wound healing process. These pathogens form biofilms that lead to chronic infections. Electrospun proteins are the main ingredients of the skin wound healing devices (Fig. 4). When the CAGE was employed in electrospinning devices as an electrospun scaffold, it caused the inactivation of both types of bacteria, Gram-positive (Enterococcus specie) as well as Gram-negative (Pseudomonas aeruginosa) by diminishing colonies. Ceftazidime, a third-generation antibiotic, is effective against wounds with biofilm; P. aeruginosa and S. enterica are biofilm-forming pathogens, often present in chronic wounds. But this drug has a plethora of limitations, including water solubility, skin permeability, and shelf-life. The CAGE comes here to rescue as it disrupts the biofilm of pathogens and makes drug delivery facile and gentle.14 Thus, it is an interesting alternative to conventional wound care.17
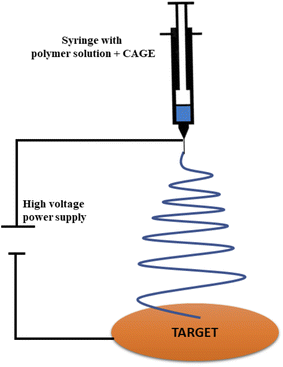 |
| Fig. 4 Representative diagram of the electrospinning process used to create CAGE scaffolds for wound healing. | |
Moreover, the capacity of antifungal drugs can be inferred from the penetration in the stratum coronium (SC) or not. To improve the efficacy of these drugs, various penetration enhancers can be used.33 The use of antifungal drugs with CAGE has proved to be a potential carrier for antifungal drug delivery. Furthermore, CAGE has been discovered as a novel transdermal delivery material that has proved to be efficient in the penetration of drugs and macromolecules through the skin without causing any irritation.34 As an amphiphilic DES, CAGE is a potential excipient in subcutaneous delivery.14 Because the CAGE is a viscous clear liquid that can improve the permeation of proteins, peptides, and small molecules through oral and transdermal delivery barriers4,6,35 the concept is elaborated in the subsequent sections.
Superficial fungal infections of the stratum corneum (SC) are treated using topical applications.36,37 CAGE is equally effective for skin fungal infections occurring in deep skin layers like the dermis and epidermis.38 It can enhance the efficiency of high molecular weight and poorly water-soluble antifungal drug ketoconazole (KCZ). The solubility of the drug is enhanced 100-fold. In vitro studies show the synergistic action of CAGE with KCZ, effective in impeding the activity of a fungus, T. interdigitale. CAGE acts in both ways, skin layer penetration, and antifungal activity because it is able to strip the wounded skin in a flaky manner to irradicate the fungi completely. The skin became normal after treatment with no irritation.19 The hydroxyethylcellulose (HEC) gel integrating CAGE, as a permeation enhancer is used to convey bacteriophage particles in the cutaneous layer for the antimicrobial treatment of pyoderma. CAGE helps to dissolve amphipathic molecules such as protein, while the use of CAGE-integrated HEC gel is desired for structural and functional stability of a cocktail of separated lytic bacteriophage particles for the treatment of animal pyoderma. The results showed the potential of CAGE in transdermal delivery enhancement for the treatment of animal skin infections39
5. CAGE in drug delivery systems
ILs and DESs are have recently been proposed as biocompatible solvents for facile drug delivery and CAGE is one of them. It has proven to be an efficient drug delivery model for transdermal,40 buccal, and subcutaneous delivery of intractable drugs with the least toxicity41,42 efficient skin permeation,43 less irritation, and the ability to achieve target therapeutic goals with minimum side effects. Fig. 5 shows some of the drugs reported to be delivered successfully via CAGE.
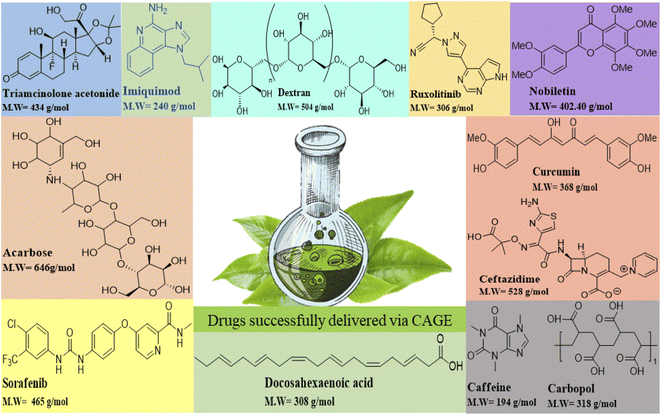 |
| Fig. 5 Drugs delivered successfully by CAGE. | |
5.1. Transdermal drug delivery
Transdermal drug delivery is gaining ground as a non-invasive alternative to injectable formulations. It ensures systematic, controlled, and painless delivery of therapeutics. Although oral delivery has several advantages over transdermal delivery, it has plenty of disadvantages as well, including large-dosage intake, low oral absorption, and irritation in the gastrointestinal tract. While passive transdermal delivery is confined within the framework of small molecules by estimating the partition coefficient (log
P) value between 1 and 4 in the octanol–water model.44 The massive hydrophilic molecules including proteins have low permeability in the skin. To modify the permeation, chemical and physical enhancers are employed, such as microneedle, urea, dimethyl sulfoxide, ultrasound, fatty acids, and alcohols.34,45–47. But these typical methods can cause irritation and toxicity. Neat CAGE is proven to be a concerted agent in antimicrobial activity and transdermal drug delivery because of its multipurpose formula, in accordance with the permeation of drug delivery with minimum toxicity to skin and epithelial cells (Fig. 6).
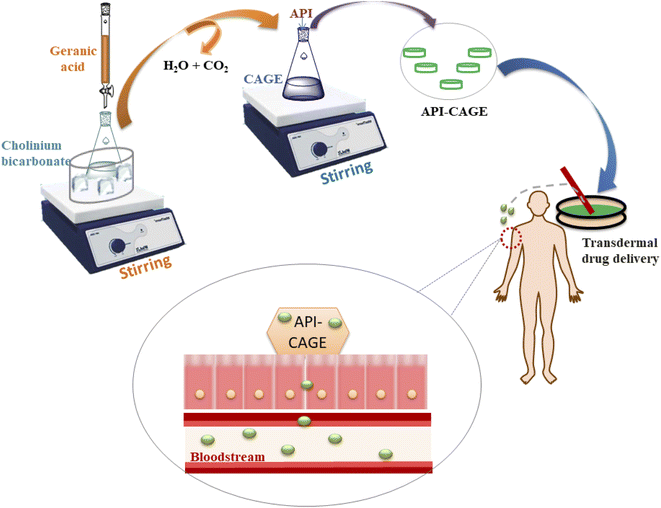 |
| Fig. 6 Schematic representation of transdermal delivery of CAGE in the body. | |
5.1.1. Skin permeation of different drugs by CAGE. Designing and screening of ILs for transdermal drug delivery are quintessential. When the transport of the two model drug acarbose (used in the treatment of type 2 diabetes)48 and ruxolitinib (Janus kinase inhibitor)49 with 16 different ILs was studied, it was found that this delivery relies on molecular composition and ion stoichiometry. The maximum transport enhancement was observed by CAGE (choline and geranic acid, 1
:
2). The systematic study with different anions confirmed that ILs with fewer interionic interactions were efficient for transdermal delivery because anions could freely enter the skin. Side by side, the modification of the cation can reduce the inter-ionic interaction, a blessing for improved drug efficacy. Thus, the combination of effectual cation and anion for a specifically designed IL efficiently enhances the transport of drugs. One of the examples is the transport of ruxolitinib drug using CAGE.50 Table 1 presents a comprehensive summary of outcomes of transdermal delivery studies of various drugs via CAGE performed so far. An FTIR study was conducted to compare, in-vogue ammonium-based ILs/DESs with conventional chemical permeation enhancers (CPEs) on the skin stratum corneum for transdermal delivery safety and efficacy. The 31 ILs/DESs including CAGE were observed leading over 44 CPEs in terms of capacity and efficacy. Moreover, Hansen solubility standard also proved the superiority of ILs/DESs over CPEs.51,52 Nobiletin (NOB), a flavonoid extracted from citrus fruits,53 has potential applications as anti-inflammatory,54 antidiabetic,55 anticancer56 and anti-Alzheimer.83 But its solubility (16.2 μg mL−1) and oral bioavailability are extremely poor. By using CAGE, a biocompatible material, transdermal delivery of NOB is highly facilitated. The efficacy of this delivery method has been tested both in vitro and in vivo. Moreover, the solubility is also enhanced due to multiple intermolecular and intramolecular hydrogen bonding interactions between NOB and CAGE (Fig. 7). NOB-CAGE also caused a considerable reduction in blood glucose levels in rats resulting from NOB hypoglycemic capacity. In vitro, Franz diffusion cell emphasizes that CAGE as a transdermal absorption enhancer is leading over other penetration enhancers. Thus, the delivery of NOB by employing CAGE as a vehicle is facile for transdermal administration.57
Table 1 Transdermal drug delivery using CAGE
CAGE the ratio of choline and geranic acid |
Formulation |
Drug/active pharmaceutical ingredient (API) |
Dermal penetration (fold increase per reported methods) |
Biological activity/applications |
Ref. |
Year |
1 : 2 |
API-DES combination |
Mannitol |
∼05 |
(i) Antimicrobial against P. aeruginosa and S. enterica (wound healing activity), (ii) drug permeation enhancement |
58 |
2014 |
Cefadoxil |
∼16 |
1 : 2 |
Protein-DES |
Bovine serum albumin |
<15.4 |
Effective delivery of polypeptide chains of high molecular weight ≈ 66 kDa, ≈45 kDa, ≈5.8 kDa |
59 |
2017 |
Ovalbumin |
<15.4 |
Insulin |
∼15.4 |
1 : 4 |
Insulin-DES |
Insulin |
Mild enhancer |
Skin penetration of insulin by applying distinct ratios of CAGE was assessed, it has proven that excessive ratios of geranic acid provide better insulin delivery thus, delivery is composition dependent |
60 |
2018 |
1 : 2 |
Very effective |
1 : 1 |
Not effective |
2 : 1 |
Verse effect |
1 : 2 |
DES as API |
CAGE |
Rapidly eradicate mature biofilms |
(i) Antiseptic against ESKAPE pathogens, (ii) thinning, interaction, and penetration mechanism of CAGE within the cell membrane (computational study) |
61 |
2019 |
1 : 2 |
API-DES/ILs |
Acarbose |
Delivered better |
Various ILs investigated to deliver hydrophilic drugs, but 1 : 2 CAGE stands out in showing effective skin permeability |
62 |
2019 |
Ruxolitinib |
Delivered good |
1 : 2 |
Dextran-DES |
Dextrans with a high molecular weight |
>40 for 20 kDa, 2 for 4 kDa |
Markedly improved transdermal delivery of large-dextran molecules having molecular weights of 4, 20, 40, 70 and 1150 k |
63 |
2019 |
1 : 2 |
NOB-DES |
Nobiletin (NOB) |
1.9–3.7 |
Enhance the bioavailability of poorly water-soluble polymath-oxy flavonoid (NOB) |
64 |
2019 |
1 : 2 |
GLP-1-DES |
Glucagon like peptide |
3–4 |
Glucagon permanency, action, evacuation, and pharmacokinetics using changed concentrations of CAGE 1 : 2 (20, 50, and 100%) |
65 |
2021 |
1 : 2 |
CPVA-DES |
Insulin via chitosan-based patches |
7 |
Oral insulin delivery results in effective blood glucose level |
66 |
2020 |
1 : 2 |
SRF-DES |
Sorafenib (SRF) |
5 |
Anti-tumor activity synergistic increase in drug efficiency |
67 |
2020 |
1 : 2 |
KCZ-DES |
Ketoconazole (KCZ) |
2–5 |
Better carrier for an antifungal drug KCZ, better recovery from fungus, and found no skin irritation synergically |
68 |
2020 |
1 : 2 |
IMQ-DES |
Imiquimod (IMQ) |
>10 |
Immunomodulator drugs showed better solubility, skin saturation, and safer way using gel-DES as well as liquid-DES formulations in CAGE |
69 |
2021 |
TCA-DES |
Triamcinolone acetonide (TCA) |
3.5–5.5 |
1 : 2 |
Pha-HEC-DES |
Bacteriophage (Pha) |
— |
Lytic bacteriophage isolated and unified with CAGE encumbered at hydroxy-ethyl-cellulose (HEC) to cure animal pyoderma |
70 |
2020 |
1 : 2 |
CAF-DES |
Caffeine (CAF) |
High |
A cocktail of CAGE, carbopol, and caffeine clinically trialed to treat skin cellulite |
71 |
2021 |
1 : 2 |
CUR-DES |
Curcumin (CUR) |
Enhanced |
Effectively delivers the natural bioactive flavonoid curcumin |
72 |
2022 |
1 : 2 |
APO-DES-PEG |
Apomorphine (APO) |
Enhanced drug delivery |
(i) Effective delivery of apomorphine for the cure of Parkinson's disease, (ii) it enhanced the drug emulsification process by increasing drug concentration |
73 |
2022 |
1 : 2 |
40% w/w CAGE |
CAGE |
1.8 folds |
Translational journey of CAGE for the treatment of rosacea (successful clinical application) |
74 |
2021 |
1 : 2 |
siRNA-IL-BDOA |
siRNA |
Enhanced |
Enhanced dermal and epidermal permeation of siRNA by CAGE |
75 |
2020 |
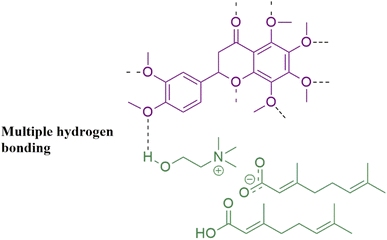 |
| Fig. 7 Hydrogen bonding interaction of Nobiletin (NOB) with CAGE. | |
Curcumin transdermal delivery in porcine ear skin has been enhanced by CAGE due to its ability to facilitate the passage of bioactive molecules. This study is one of its kind for the histological translation of CAGE to alter the structure of outer skin barriers.43 In another study, an in-depth analysis was performed to improve the transdermal permeation of curcumin to infer the effect of CAGE concentration. The model used was non-linear regression analysis, which proved that a low concentration of CAGE (2% w/w) helps disrupt the skin in a transient fashion and facilitates the curcumin dissolution passage.58
CAGE is also effective in controlling obesity by interacting with fat molecules to form particles of micrometer size, which hinders fat from penetrating the intestine, resulting in lower body weight gain.59,60 Ginoid hydrolipodystrophy (HDLG) or cellulite, a common issue among the female population, comprises an alteration of the cutaneous relief. Different treatments work by stimulation of lipolysis (caffeine). The therapeutic ‘CAGE’ facilitates the topical permeation of caffeine. CAGE (1%) was converted into a gel with two other components 2% carbopol, and 5% caffeine. Preliminary and stability tests were performed by checking various characteristics, including spreadability, organoleptic properties, and pH. A Franz cell permeation assay has confirmed the transdermal permeation of caffeine. The clinical trial was also performed for the treatment of cellulite using CAGE. The gel formulation was stable after 180 days of storage. Moreover, the gel has high permeation in the skin with bit cytotoxicity. The clinical application proved that the gel with CAGE is superior to a gel without IL due to its cutaneous permeation of caffeine.40
5.1.2. Skin permeation of macromolecules using CAGE. Traditional methods for transdermal delivery of biological macromolecules such as peptides and other hydrophilic and hydrophobic molecules face serious limitations because the permeability of the skin is low. Recently, deep eutectic solvents of CAGE have been observed to improve protein delivery such as insulin (INS), ovalbumin (OVA), and bovine serum albumin (BSA). CAGE tends to enhance the permeation of these proteins into porcine skin ex vivo, accelerating their penetration into the dermis and epidermis. Further studies illustrate that the titanium-coated BSA and insulin coated with fluorescein isothiocyanate have improved the delivery of proteins by showing a time-dependent manner of penetration. Fourier transform IR spectrum of porcine skin shows the extraction of lipids from the stratum corneum (SC) of porcine. Moreover, circular dichroism emphasizes that CAGE has no effect on insulin's secondary conformation. When studies were conducted on rats using insulin dispersed in CAGE, there was a 40% fall in blood glucose levels. Thus, these studies have confirmed that the employment of CAGE in the transdermal delivery of proteins is showing promising results, worth mentioning, non-invasive alternatives to insulin delivery for treating diabetes.61Giant hydrophilic molecules are used in transdermal delivery posing a challenge due to the diffusion barrier properties of the skin. By employing the CAGE (1
:
2), dextrans with molecular weight (MW) up to 150 KDa was observed to deliver significantly. Moreover, for the very first time, CAGE has limited the size-dependence barriers for transport and has broadened the horizon for different solutes. FTIR suggested that CAGE facilitates the process of lipid extraction while permeability is improved due to faster diffusion of macromolecules.4 Similarly, the CAGE is equally effective for the hydrophobic drug sorafenib (SRF); 0.5 mg mL−1 of CAGE significantly enhances the cytotoxic efficacy of SRF without influencing the internal structure of the cell. CAGE was observed to enhance the effectiveness of SRF up to five-fold with increased SRF retention and impeding exocytosis when delivered to cancerous cells as well as intestinal epithelial cells. Furthermore, apoptosis and blockage of cell cycle growth by CAGE inclusion in SRF improved its anti-tumor effect. Multiple mechanisms are involved in the penetration of SRF into and across cells due to the CAGE. Taken together, CAGE as a synergistic agent of SRF increasing the potential of novel therapeutic efficacy for treating cancerous cells.62
5.1.3. Delivery of immunomodulators via CAGE. The skin has the potential to offer an excellent environment for the introduction of immunomodulators63,64 which are biological or synthetic substances that can tune the immune system for both adaptive and innate arms of the immune response.65 But the skin barriers hamper their delivery. By employing CAGE, their delivery has been made facile. Two drugs, imiquimod (IMQ) and triamcinolone acetonide (TCA) are used as immuno-active and immunosuppressive drugs, respectively. With respect to typical comparators, CAGE was observed to outperform in the delivery of immunomodulators to deep skin layers. When a comparison of lead IMQ formulation was performed using commercial IMQ (5%) cream, the former showed a 10-fold better delivery of IMQ than the latter. The lead TCA came at par with intralesional injections available clinically. Thus, the ILs-based CAGE strategy is an easy and effective method of drug delivery to the skin.66
5.1.4. Efficient delivery of small interference RNA (siRNA). The growth of the undesired protein can be impeded by employing small interference RNA (siRNA), which hampers the effect of the allele-specific gene for treating various diseases. But the delivery of siRNA is a daunting challenge because the large size and negative charge of siRNA make its interaction poor with the cell membrane. Moreover, it often degrades into the bloodstream and causes inflammation.67,68 Thus, its transport and delivery to target tissues are lagging behind. CAGE helps the skin penetration of siRNA for designed drug delivery. By employing CAGE, the delivery became facile because of the non-covalent interaction of CAGE with siRNA to improve dermal penetration. The synergistic effect with enhanced dermal and epidermal penetration was confirmed by applying conditions on porcine skin and SKH-1E hairless mice with no toxicity.69
5.2. CAGE in the oral delivery systems
Drug delivery through the buccal route (mouth) is facilitated due to the abundance of blood vessels and the absence of any tight junctions in the buccal epithelium.42,70 Along with the dramatic enhancement of the recalcitrant hydrophilic therapeutics, CAGE also modifies the drug absorption capacity. It can improve the drug's half-life and its pharma properties. Moreover, it changes the bio-distribution of the drug by improving its capacity to target the organs. CAGE is an efficient drug delivery model for enhancing the oral absorption of hydrophobic drugs with varying bio-distribution to achieve the target therapeutic goal and minimize the side effects (Fig. 8). It has been explored for oral delivery of insulin as well as some other water-insoluble drugs described later.
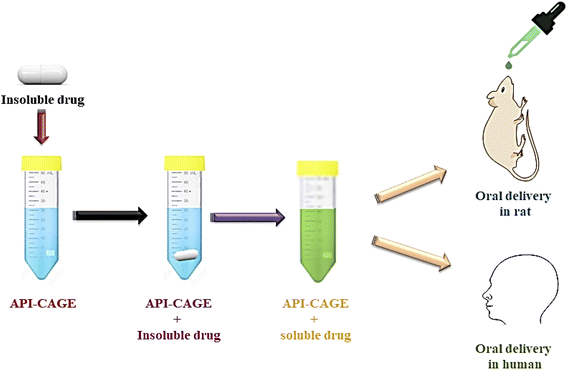 |
| Fig. 8 The scheme of study for oral drug delivery in rats and humans. | |
The increase in the number of patients with diabetes worldwide and the reluctance to injectable insulin is demanding an effective oral insulin formulation apart from the efficient transdermal delivery method. The main hindrance in the oral delivery of drugs is the gastrointestinal tract. However, CAGE is proven effective in the oral delivery of drugs without causing any enzymatic degradation and thinning of the mucus layer. When insulin-CAGE was administered in the jejuna of rats, it proved exceptional both pharmacokinetically and pharmacodynamically. Due to the slow administration of insulin (3–10 U Kg−1) and exposure for a longer period of time (up to 12 hours), there was a considerable reduction in blood glucose levels. When coated capsules were used for oral delivery of insulin-CAGE (10 U Kg−1), a 45% decrease in blood glucose level was observed. This formula was highly biocompatible and showed stability for almost 60 days at room temperature and 120 days under refrigeration.13
Buccal drug delivery is a localized way to deliver the drug, but the delivery of macromolecules by buccal administration into systematic circulation remains a cumbersome challenge. When the CAGE was employed as a transport facilitator with a biodegradable polymer patch of chitosan as the mucoadhesive matrix, insulin formed a gel with CAGE that was sandwiched between layers of the biodegradable polymer. CAGE gel behaved viscoelastically in concurrence with elastic modulus. It was confirmed by confocal microscopy that the transport of insulin (26% loading) was increased seven-fold by employing CAGE. The CAGE-insulin patches lowered blood glucose levels with no significant tissue damage. This showed a sustainable profile as the pleated level of serum insulin remained stable for 3 h.71
PVA-based ionogels loaded with CAGE are also effective for the oral administration of insulin. The solubility of the drug and absorption in the intestine are improved due to the CAGE. By using PVA, CAGE was encapsulated into a gel form, which overall formed a mucoadhesive-ionogel patch (CAGE-patch) to stick to the intestine. Repeated freeze–thaw cycles of CAGE patches have increased the swelling, mucoadhesive strength, and desired delivery of insulin. In vitro, studies indicated an overall >30% enhanced transport.72
Clinical trials were also performed for using CAGE as a vehicle for the transfer of insulin into the body due to the inability of insulin to cross the translational barriers.73 Employing the insulin-CAGE model for oral delivery has, unprecedently, enhanced the oral bioavailability, biocompatibility, oral efficacy, and long-term stability of the drug.3,61
Besides insulin, CAGE is also a potent DES/IL for other water-insoluble drugs. It facilitates their delivery and enhances their bioavailability by efficiently solubilizing them. One such drug is SRF tosylate, which showed higher solubility in CAGE (>500 mg mL−1). When an oral dose was given to rats, CAGE increased the amount of SRF in the blood by 2.2-fold. The elimination of half-life was also doubly enhanced with an increase in the mean absorption time to 1.6-fold. The mechanistic pathway illustrated that the combined solution of SRF and CAGE forms a self-assembled structure that supports the varying bio-distribution of CAGE in the laboratory. Ultra-High Performance Liquid Chromatography with Mass Spectrometry detection (UPLC-MS) endorsed the micellar/emulsion structure of combined choline and geranate that further break down into molecular species.74
Obesity is a common problem that leads to various secondary complications, including hypertension and diabetes. Besides the other factors, high fat and carbohydrate content in food is responsible for weight gain. CAGE can help in this regard by reduction of body weight with lower fat absorption through the intestine. In vitro studies conducted on a model fat molecule docosahexaenoic acid (DHA) illustrated that CAGE can link with fat molecules by forming particles of 2 to 4 μm in diameter to hinder their absorption through the intestine with satiety. When studies were conducted on rats, it showed that large particles lower fat absorption in the intestine. In the laboratory, CAGE lowered the absorption of the model fat molecules by about 60–70%. When rats were given a high amount of fat with 10 μL daily oral dose of CAGE, <12% weight gain was observed as compared with the control group that was given high-fat content for 1 month without CAGE.75
5.3. Subcutaneous drug delivery via CAGE
Subcutaneous injections are used for low and continuous delivery of hormones, antibodies, peptides, and small molecules. The preference for injection depends upon combinations of principles such as effectiveness, safety, patient choice, and pharmaco-economics.76 Instead of intravenous injection, the subcutaneous method is easy and cost-effective and offers relative bioavailability for some drugs.77 Besides CAGE usage as DES/IL in transdermal delivery and oral administration, one of its attributes is subcutaneous delivery (Fig. 9). To test its efficiency, a common glucagon-like peptide-1 (GLP-1) was employed to be delivered subcutaneously. Generally, it is degraded in the presence of the enzyme ‘dipeptidyl peptidase-4 (DPP-4)’ and is cleared rapidly from the circulatory system. But when delivery was performed through a neat or diluted CAGE, the peptide concentration was observed to increase in the circulatory system. Further mechanistic studies illustrate that CAGE hampers enzymatic degradation due to its self-assembly and helps in the entrapment and facile release of GLP-1.78
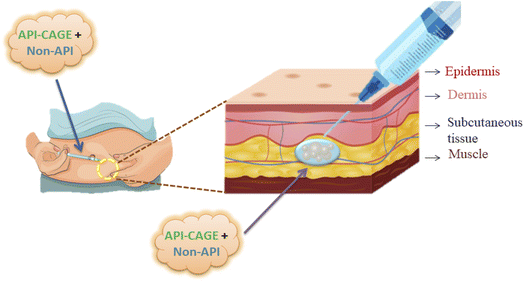 |
| Fig. 9 Schematic illustration of subcutaneous drug delivery by CAGE. | |
In a recent study, CAGE was used in the effective delivery of apomorphine (a dopamine agonist), used to treat Parkinson's disease. The half-life of apomorphine was improved by emulsification with CAGE in the subcutaneous space, as observed by ex vivo studies in rats. In vivo studies on rats and pigs further confirmed the superiority of CAGE over the clinical comparator apokyn. Thus, DES maintains its drug concentration in plasma by substituting three injections per day with three injections per week.41
6. Interaction of CAGE with body fluids
The drug delivery application of CAGE requires an understanding of its microscopic structure in the presence of water, which is the medium for all reactions occurring in the body (Fig. 10). As, in physiological fluids, water and CAGE occur together for biomedical applications and water is crucial for the physiochemical properties of CAGE. The interaction of CAGE (choline: geranic acid, 1
:
1) with water was studied through experimental and computational studies focusing on different parameters such as conductivity, viscosity, and the self-diffusion coefficient. It was reported that under stored conditions, neat CAGE comprises a 0.2 mole fraction of water, and the properties of CAGE stay constant with intra-ionic interaction until the water fraction does not exceed 0.65 mole fraction. Geranate ions are proposed to undergo rearrangement to minimize the interactions between the hydrophobic tail and water molecules, as evidenced by increased interactions of ions in the tails and plateau in the viscosity. Thus, pre-drying of CAGE for different applications is not mandatory but it can be diluted for the reorganization of anions.79
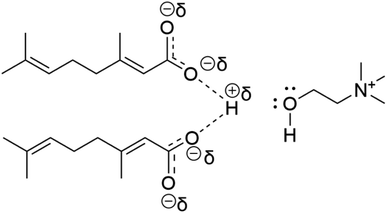 |
| Fig. 10 The interaction of CAGE with water. | |
Moreover, the influence of physiological fluids (intestinal and intradermal fluids) on the structure of CAGE is not obvious in transdermal and oral delivery. The molecular structure of CAGE with varying water ratios was investigated by employing the small-angle X-ray scattering (SAXS) and nuclear magnetic resonance (NMR) spectroscopy. The SAXS showed a transition to the lamellar phase of CAGE with broad peaks (17 vol% water) and narrow peaks (25–50 vol% water), overall CAGE micelles with more than 67 vol% water. The proton NMR showed a peak at a higher magnetic field and increased integral content due to water molecules. This indicated that the presence of a single proton peak of H2O, choline (–OH), and geranic acid (–COOH) is due to the proton exchange. Carbon NMR also showed the shifting of the carbonyl peak with added water. Thus, it is confirmed that CAGE maintains its structure with water up to 17 vol% and this information is useful to understand the drug delivery applications of CAGE.15
Recently, surface tension and thermal expansion coefficients of various aqueous solutions of CAGE have been investigated, providing a new perspective on CAGE behavior; the liquid–vapor interface, and its mechanism in a sophisticated way. This will inspire scientists to explore other applications of CAGE and related materials.80
7. Clinical trials of CAGE
The scope of academic discoveries in the clinical stages is often delayed by the difference in the practical and fundamental laboratory research. Usually, academic innovations are not compatible with clinical due to the issues of scale-up, stability, and human safety. Therefore, academics and clinical discoveries are not in concurrence with each other. Contrarily, CAGE exhibited a remarkable potential to be used in both translations; it has been investigated for dermatological applications in the lab as well as in clinical trials for rosacea,81 which is an inflammatory heterogeneous skin disease affecting millions of people, especially Caucasian adults.82 It has varying symptoms and manifestations that make its diagnosis tricky.83,84 CAGE gel (CGB400) was applied to facial rosacea in 26 patients for a 12 weeks open-label phase and a significant reduction in inflammation was observed.85 Using the CAGE for the cure has been observed to cause a significant reduction in the number of inflammatory lesions, clinically.
A major issue in the successful pharmaceutical application of most of the active compounds or drugs such as benzalkonium chloride,86 chlorhexidine,87 and povidone-iodine is their toxicity against human cells and tissues. Although they exhibit surface effective cleaning, pre-surgical-sanitizing properties and curing agents for eye infections88 and chronic wounds.89 Contrarily, the CAGE has lower cytotoxicity when it was tested on mammalian cells.90 In mice and rats, the same results were verified, and no skin irritation and toxicity were observed. Similarly, when compared with intradermal injection of sterile saline, CAGE showed superiority due to its biocompatibility, as evidenced by the in vivo studies. These superior attributes of CAGE over other traditional drugs appeal to its use in the clinical treatment of infections.
The clinical potential of CAGE was also tested on animal models against various infectious diseases; one of them is P. acnes infection. This bacterial infection affects the deepest layers of the skin and demands treatment accordingly. Similar to P. acnes, there is no optimal treatment method for chronic acne vulgaris. One method, Over-the-Counter (OTC) is used for acne treatment due to its non-irritating nature.91,92 However, medications are preferred over this treatment due to their site-clearing limitation. But medications come with irritation and side effects such as rash, itching, dryness, and skin sensitivity. Moreover, the stringent Gram-positive bacterial cell wall inhibits the delivery of drugs in doses with a number of other problems that may activate the immune system for increased inflammatory responses and sensitivity to clinical agents due to the release of pro-inflammatory molecules in the skin. In contrast, CAGE has proven more effective than 1% w/v of the typically prescribed clindamycin, which is a water-soluble drug with poor skin penetration while most pathogenic microbes reside in deep layers of tissues. A saline solution of 1% clindamycin and neat CAGE was used for comparative analysis after a single treatment with CAGE; there was a significant reduction in skin inflammation, while no observable reduction was detected with clindamycin usage.93
The effectiveness of CAGE was due to its high skin penetration ability. To obtain an insight into CAGE penetration as a carrier with solute, the fluorescent calcein was employed to model clindamycin migration in the deepest layers of skin. Calcein is quite compatible with clindamycin in solubility and molecular weight. Encouragingly, the migration of both drugs was significantly enhanced due to CAGE. The dissolved drug in CAGE performs two functions, neutralization of pathogens and significant transport enhancement in the deepest layers of tissue. According to an estimate, CAGE can penetrate 3.5 mm into the intact skin, so it is effective for the treatment of mild infections when used alone as well as in combination with other antibiotics in lethal infections.94
8. Conclusion
CAGE provided a new perspective on drug delivery. Compared with other ILs, CAGE is a multifunctional IL/DES that has been used in buccal, dermal and subcutaneous delivery of drugs. It promotes the dissolution of poorly soluble recalcitrant drugs and improves their delivery by bypassing the stratum coronium barrier and disrupting cell integrity. Along with these, CAGE has emerged as a delivery carrier for large bioactive molecules such as peptides and siRNA, which have been proven to challenge other therapeutics. Moreover, CAGE biocompatibility, chemical stability, bioavailability, and efficacy have attracted its exploitation as a drug delivery carrier. It is a potent and broad-spectrum antimicrobial agent with the potential to surpass many conventional antimicrobial agents. Future research on CAGE and other API-ILs must focus on the following:
(1) Their multi-functionality for delivery of small and large drugs.
(2) Their tunability for controlled drug release and prolonged safety in the body guarantees better efficacy.
(3) Except for the delivery of small molecules and proteins, more bioactive peptides provide good application prospects in the field of drug delivery.
(4) The stability of CAGE and other API-ILs must be further improved, instead of relying on storage in an inert atmosphere.
(5) Laboratory and clinical trials for CAGE and other API-ILs must be encouraged to utilize the full potential of these therapeutics practically.
Conflicts of interest
All the authors declare no conflict of interest.
Acknowledgements
The Higher Education Commission of Pakistan is acknowledged for providing funding through TDF03-294 and HEC/NRPU-8639. The School of Chemistry is acknowledged for its support of this project.
References
- O. Bruyaka, H. K. Zeitzmann, I. Chalamon, R. E. Wokutch and P. Thakur, Strategic corporate social responsibility and orphan drug development: Insights from the US and the EU biopharmaceutical industry, J. Bus. Ethics, 2013, 117, 45–65 CrossRef.
- R. Kellar, N. C. Nieto, A. Koppisch and R. Del Sesto, Ionic liquids that sterilize and prevent biofilm formation in skin wound healing devices, US Pat., US 2020/0289421 Al, 2019 Search PubMed.
- M. Zakrewsky, A. Banerjee, S. Apte, T. L. Kern, M. R. Jones, R. E. D. Sesto, A. T. Koppisch, D. T. Fox and S. Mitragotri, Choline and geranate deep eutectic solvent as a broad-spectrum antiseptic agent for preventive and therapeutic applications, Adv. Healthcare Mater., 2016, 5, 1282–1289 CrossRef CAS PubMed.
- K. N. Ibsen, H. Ma, A. Banerjee, E. E. Tanner, S. Nangia and S. Mitragotri, Mechanism of antibacterial activity of choline-based ionic liquids (CAGE), ACS Biomater. Sci. Eng., 2018, 4, 2370–2379 CrossRef CAS.
- Q. M. Qi and S. Mitragotri, Mechanistic study of transdermal delivery of macromolecules assisted by ionic liquids, J. Controlled Release, 2019, 311, 162–169 CrossRef PubMed.
- E. E. Tanner, K. N. Ibsen and S. Mitragotri, Transdermal insulin delivery using choline-based ionic liquids (CAGE), J. Controlled Release, 2018, 286, 137–144 CrossRef CAS PubMed.
- B. Lu, T. Liu, H. Wang, C. Wu, H. Chen, Z. Liu and J. Zhang, Ionic Liquid Transdermal Delivery System: Progress, Prospects, and Challenges, J. Mol. Liq., 2022, 118643 CrossRef CAS.
- A. M. Curreri, S. Mitragotri and E. E. Tanner, Recent advances in ionic liquids in biomedicine, Adv. Sci., 2021, 8, 2004819 CrossRef CAS.
- C. Agatemor, K. N. Ibsen, E. E. Tanner and S. Mitragotri, Ionic liquids for addressing unmet needs in healthcare, Bioeng. Transl. Med., 2018, 3, 7–25 CrossRef PubMed.
- M. Friedman, P. R. Henika, C. E. Levin and R. E. Mandrell, Antibacterial activities of plant essential oils and their components against Escherichia coli O157: H7 and Salmonella enterica in apple juice, J. Agric. Food Chem., 2004, 52, 6042–6048 CrossRef CAS PubMed.
- E. L. Smith, A. P. Abbott and K. S. Ryder, Deep eutectic solvents (DESs) and their applications, Chem. Rev., 2014, 114, 11060–11082 CrossRef CAS.
- I. Marrucho, L. Branco and L. Rebelo, Ionic liquids in pharmaceutical applications, Annu. Rev. Chem. Biomol. Eng., 2014, 5, 527–546 CrossRef CAS PubMed.
- Q. Zhang, K. D. O. Vigier, S. Royer and F. Jérôme, Deep eutectic solvents: syntheses, properties and applications, Chem. Soc. Rev., 2012, 41, 7108–7146 RSC.
- M. Zakrewsky, K. S. Lovejoy, T. L. Kern, T. E. Miller, V. Le, A. Nagy, A. M. Goumas, R. S. Iyer, R. E. Del Sesto and A. T. Koppisch, Ionic liquids as a class of materials for transdermal delivery and pathogen neutralization, Proc. Natl. Acad. Sci. U. S. A., 2014, 111, 13313–13318 CrossRef CAS PubMed.
- J. Takeda, Y. Iwao, M. Karashima, K. Yamamoto and Y. Ikeda, Structural evaluation of the choline and geranic acid/water complex by SAXS and NMR analyses, ACS Biomater. Sci. Eng., 2021, 7, 595–604 CrossRef CAS.
- S. Mitragotri, A. Banerjee, T. Brown, K. Ibsen and C. Agatemor, Ionic liquids for internal delivery, 2020, WO2019099837A1.
- T. A. Bardsley, C. L. Evans, J. R. Greene, R. Audet, M. J. Harrison, M. Zimmerman, N. C. Nieto, R. E. Del Sesto, A. T. Koppisch and R. S. Kellar, Integration of choline geranate into electrospun protein scaffolds affords antimicrobial activity to biomaterials
used for cutaneous wound healing, J. Biomed. Mater. Res., Part B, 2021, 109, 1271–1282 CrossRef CAS.
- M. Shevachman, A. Mandal, S. Mitragotri and N. Joshi, A long-lasting sanitizing skin protectant based on CAGE, a choline and geranic acid eutectic, medRxiv, 2020 Search PubMed.
- X. Wu, Q. Yu, J. Wu, T. Li, N. Ding, W. Wu, Y. Lu, Q. Zhu, Z. Chen and J. Qi, Ionic liquids containing ketoconazole improving topical treatment of T. Interdigitale infection by synergistic action, Int. J. Pharm., 2020, 589, 119842 CrossRef CAS PubMed.
- Department of Health And Human Services Food And Drug Administration, Safety and effectiveness of health care antiseptics; topical antimicrobial drug products for over-the-counter human use. Final rule, Fed. Regist., 2017, 82, 60474–60503 Search PubMed.
-
(a) J. Costerton, Introduction to biofilm, Int. J. Antimicrob. Agents, 1999, 11, 217–221 CrossRef CAS;
(b) J. W. Costerton, P. S. Stewart and E. P. Greenberg, Bacterial biofilms: a common cause of persistent infections, Science, 1999, 284, 1318–1322 CrossRef CAS PubMed.
- D. Davies, Understanding biofilm resistance to antibacterial agents, Nat. Rev. Drug Discovery, 2003, 2, 114–122 CrossRef CAS.
- P. Stewart and J. William Costerton, Lancet, 2001, 358, 135–138 CrossRef CAS.
- J. R. Greene, K. L. Merrett, A. J. Heyert, L. F. Simmons, C. M. Migliori, K. C. Vogt, R. S. Castro, P. D. Phillips, J. L. Baker and G. E. Lindberg, Scope and efficacy of the broad-spectrum topical antiseptic choline geranate, PLoS One, 2019, 14, e0222211 CrossRef CAS PubMed.
- J. Pernak and A. Skrzypczak, 3-Alkylthiomethyl-1-ethylimidazolium chlorides. Correlation between critical micelle concentrations and minimum inhibitory concentrations, Eur. J. Med. Chem., 1996, 31, 901–903 CrossRef CAS.
- S. Jeong, S. H. Ha, S.-H. Han, M.-C. Lim, S. M. Kim, Y.-R. Kim, Y.-M. Koo, J.-S. So and T.-J. Jeon, Elucidation of molecular interactions between lipid membranes and ionic liquids using model cell membranes, Soft Matter, 2012, 8, 5501–5506 RSC.
- A. B. Wysocki, Evaluating and managing open skin wounds: colonization versus infection, AACN Adv. Crit. Care, 2002, 13, 382–397 Search PubMed.
- J. C. Barker, I. Khansa and G. M. Gordillo, A formidable foe is sabotaging your results: what you should know about biofilms and wound healing, Plast. Reconstr. Surg., 2017, 139, 1184e CrossRef CAS.
- M. Gompelman, S. A. van Asten and E. J. Peters, Update on the role of infection and biofilms in wound healing: pathophysiology and treatment, Plast. Reconstr. Surg., 2016, 138, 61S–70S CrossRef CAS PubMed.
- K. R. Kirker and G. A. James, In vitro studies evaluating the effects of biofilms on wound-healing cells: a review, Apmis, 2017, 125, 344–352 CrossRef PubMed.
- S. R. Nussbaum, M. J. Carter, C. E. Fife, J. DaVanzo, R. Haught, M. Nusgart and D. Cartwright, An economic evaluation of the impact, cost, and medicare policy implications of chronic nonhealing wounds, Value Health, 2018, 21, 27–32 CrossRef.
- J. N. Pendleton, S. P. Gorman and B. F. Gilmore, Clinical relevance of the ESKAPE pathogens, Expert Rev. Anti-Infect. Ther., 2013, 11, 297–308 CrossRef CAS PubMed.
- P. Boonme, J. Kaewbanjong, T. Amnuaikit, T. Andreani, A. M Silva and E. B Souto, Microemulsion and microemulsion-based gels for topical antifungal therapy with phytochemicals, Curr. Pharm. Des., 2016, 22, 4257–4263 CrossRef CAS PubMed.
- J. J. Escobar-Chavez, I. M. Rodriguez-Cruz and C. L. Dominguez-Delgado, Chemical and physical enhancers for transdermal drug delivery, Pharmacology, 2012, 7, 398–435 Search PubMed.
- A. Banerjee, K. Ibsen, T. Brown, R. Chen, C. Agatemor and S. Mitragotri, Ionic liquids for oral insulin delivery, Proc. Natl. Acad. Sci. U. S. A., 2018, 115, 7296–7301 CrossRef CAS.
- N. Akhtar, A. Verma and K. Pathak, Topical delivery of drugs for the effective treatment of fungal infections of skin, Curr. Pharm. Des., 2015, 21, 2892–2913 CrossRef CAS.
- L. H. Kircik, Advancements in Topical Antifungal Vehicles, J. Drugs Dermatol., 2016, 15, s44–s48 CAS.
- T. Sato, Practical management of deep cutaneous fungal infections, Med. Mycol. J., 2017, 58, E71–E77 CrossRef PubMed.
- E. C. Silva, T. J. Oliveira, F. C. Moreli, L. K. Harada, M. M. Vila and V. M. Balcão, Newly isolated lytic bacteriophages for Staphylococcus intermedius, structurally and functionally stabilized in a hydroxyethylcellulose gel containing choline geranate: Potential for transdermal permeation in veterinary phage therapy, Res. Vet. Sci., 2021, 135, 42–58 CrossRef CAS PubMed.
- A. N. Hernandes, R. Boscariol, V. M. Balcão and M. M. Vila, Transdermal Permeation of Caffeine Aided by Ionic Liquids: Potential for Enhanced Treatment of Cellulite, AAPS PharmSciTech, 2021, 22, 1–9 CrossRef PubMed.
- J. Kim, Y. Gao, Z. Zhao, D. Rodrigues, E. E. Tanner, K. Ibsen, P. K. Sasmal, R. Jaladi, S. Alikunju and S. Mitragotri, A deep eutectic-based, self-emulsifying subcutaneous depot system for apomorphine therapy in Parkinson's disease, Proc. Natl. Acad. Sci. U. S. A., 2022, 119, e2110450119 CrossRef CAS.
- J. O. Morales and D. J. Brayden, Buccal delivery of small molecules and biologics: of mucoadhesive polymers, films, and nanoparticles, Curr. Opin. Pharmacol., 2017, 36, 22–28 CrossRef CAS PubMed.
- R. Boscariol, É. A. Caetano, E. C. Silva, T. J. Oliveira, R. M. Rosa-Castro, M. M. Vila and V. M. Balcão, Performance of choline geranate deep eutectic solvent as transdermal permeation enhancer: An in vitro skin histological study, Pharmaceutics, 2021, 13, 540 CrossRef CAS.
- N. Chandrashekar and R. S. Rani, Physicochemical and pharmacokinetic parameters in drug selection and loading for transdermal drug delivery, Indian J. Pharm. Sci., 2008, 70, 94 CrossRef CAS PubMed.
- A. C. Williams and B. W. Barry, Penetration enhancers, Adv. Drug Delivery Rev., 2012, 64, 128–137 CrossRef.
- I. B. Pathan and C. M. Setty, Chemical penetration enhancers for transdermal drug delivery systems, Trop. J. Pharm. Res., 2009, 8 CAS.
- Y. Chen, P. Quan, X. Liu, M. Wang and L. Fang, Novel chemical permeation enhancers for transdermal drug delivery, Asian J. Pharm. Sci., 2014, 9, 51–64 CrossRef.
- R. R. Holman, C. A. Cull and R. C. Turner, A randomized double-blind trial of acarbose in type 2 diabetes shows improved glycemic control over 3 years (UK Prospective Diabetes Study 44), Diabetes Care, 1999, 22, 960–964 CrossRef CAS PubMed.
- S. Verstovsek, R. A. Mesa, J. Gotlib, R. S. Levy, V. Gupta, J. F. DiPersio, J. V. Catalano, M. Deininger, C. Miller and R. T. Silver, A double-blind, placebo-controlled trial of ruxolitinib for myelofibrosis, N. Engl. J. Med., 2012, 366, 799–807 CrossRef CAS PubMed.
- E. E. Tanner, A. M. Curreri, J. P. Balkaran, N. C. Selig-Wober, A. B. Yang, C. Kendig, M. P. Fluhr, N. Kim and S. Mitragotri, Design principles of ionic liquids for transdermal drug delivery, Adv. Mater., 2019, 31, 1901103 CrossRef.
- P. Karande, A. Jain, K. Ergun, V. Kispersky and S. Mitragotri, Design principles of chemical penetration enhancers for transdermal drug delivery, Proc. Natl. Acad. Sci. U. S. A., 2005, 102, 4688–4693 CrossRef CAS.
- Q. M. Qi, M. Duffy, A. M. Curreri, J. P. Balkaran, E. E. Tanner and S. Mitragotri, Comparison of ionic liquids and chemical permeation enhancers for transdermal drug delivery, Adv.
Funct. Mater., 2020, 30, 2004257 CrossRef CAS.
- T. Seki, T. Kamiya, K. Furukawa, M. Azumi, S. Ishizuka, S. Takayama, S. Nagase, H. Arai, T. Yamakuni and N. Yaegashi, Nobiletin-rich Citrus reticulata peels, a kampo medicine for Alzheimer's disease: A case series, Geriatr. Gerontol. Int., 2013, 13, 236–238 CrossRef PubMed.
- N. Lin, T. Sato, Y. Takayama, Y. Mimaki, Y. Sashida, M. Yano and A. Ito, Novel anti-inflammatory actions of nobiletin, a citrus polymethoxy flavonoid, on human synovial fibroblasts and mouse macrophages, Biochem. Pharmacol., 2003, 65, 2065–2071 CrossRef CAS PubMed.
- H. Nagase, N. Omae, A. Omori, O. Nakagawasai, T. Tadano, A. Yokosuka, Y. Sashida, Y. Mimaki, T. Yamakuni and Y. Ohizumi, Nobiletin and its related flavonoids with CRE-dependent transcription-stimulating and neuritegenic activities, Biochem. Biophys. Res. Commun., 2005, 337, 1330–1336 CrossRef CAS.
- K. Kunimasa, M. Ikekita, M. Sato, T. Ohta, Y. Yamori, M. Ikeda, S. Kuranuki and T. Oikawa, Nobiletin, a citrus polymethoxyflavonoid, suppresses multiple angiogenesis-related endothelial cell functions and angiogenesis in vivo, Cancer Sci., 2010, 101, 2462–2469 CrossRef CAS.
- T. Hattori, H. Tagawa, M. Inai, T. Kan, S.-i. Kimura, S. Itai, S. Mitragotri and Y. Iwao, Transdermal delivery of nobiletin using ionic liquids, Sci. Rep., 2019, 9, 1–11 CrossRef PubMed.
- R. Boscariol, J. M. O. Junior, D. A. Baldo, V. M. Balcão and M. M. Vila, Transdermal permeation of curcumin promoted by choline geranate ionic liquid: Potential for the treatment of skin diseases, Saudi Pharm. J., 2022 Search PubMed.
- J.-W. Shin, I.-C. Seol and C.-G. Son, Interpretation of animal dose and human equivalent dose for drug development, J. Korean Med., 2010, 31, 1–7 Search PubMed.
- A. B. Nair and S. Jacob, A simple practice guide for dose conversion between animals and human, J. Basic Clin. Pharm., 2016, 7, 27 CrossRef PubMed.
- A. Banerjee, K. Ibsen, Y. Iwao, M. Zakrewsky and S. Mitragotri, Transdermal protein delivery using choline and geranate (CAGE) deep eutectic solvent, Adv. Healthcare Mater., 2017, 6, 1601411 CrossRef PubMed.
- Y. Shi, Z. Zhao, K. Peng, Y. Gao, D. Wu, J. Kim and S. Mitragotri, Enhancement of Anticancer Efficacy and Tumor Penetration of Sorafenib by Ionic Liquids, Adv. Healthcare Mater., 2021, 10, 2001455 CrossRef CAS.
- Z. Zhao, A. Ukidve, A. Dasgupta and S. Mitragotri, Transdermal immunomodulation: Principles, advances and perspectives, Adv. Drug Delivery Rev., 2018, 127, 3–19 CrossRef CAS PubMed.
- P. Di Meglio, G. K. Perera and F. O. Nestle, The multitasking organ: recent insights into skin immune function, Immunity, 2011, 35, 857–869 CrossRef CAS PubMed.
- H. A. El Enshasy and R. Hatti-Kaul, Mushroom immunomodulators: unique molecules with unlimited applications, Trends Biotechnol., 2013, 31, 668–677 CrossRef CAS PubMed.
- Z. Zhao, E. E. Tanner, J. Kim, K. Ibsen, Y. Gao and S. Mitragotri, Ionic Liquid-Enabled Topical Delivery of Immunomodulators, ACS Biomater. Sci. Eng., 2021, 7, 2783–2790 CrossRef CAS PubMed.
- J. Wang, Z. Lu, M. G. Wientjes and J. L.-S. Au, Delivery of siRNA therapeutics: barriers and carriers, AAPS J., 2010, 12, 492–503 CrossRef CAS PubMed.
- Y. Dong, D. J. Siegwart and D. G. Anderson, Strategies, design, and chemistry in siRNA delivery systems, Adv. Drug Delivery Rev., 2019, 144, 133–147 CrossRef CAS PubMed.
- V. Dharamdasani, A. Mandal, Q. M. Qi, I. Suzuki, M. V. L. B. Bentley and S. Mitragotri, Topical delivery of siRNA into skin using ionic liquids, J. Controlled Release, 2020, 323, 475–482 CrossRef CAS.
- M. Sattar, O. M. Sayed and M. E. Lane, Oral transmucosal drug delivery-current status and future prospects, Int. J. Pharm., 2014, 471, 498–506 CrossRef CAS PubMed.
- A. Vaidya and S. Mitragotri, Ionic liquid-mediated delivery of insulin to buccal mucosa, J. Controlled Release, 2020, 327, 26–34 CrossRef CAS PubMed.
- K. Peng, Y. Shi, A. LaBarbiera and S. Mitragotri, Mucoadhesive ionic liquid gel patches for oral delivery, ACS Biomater. Sci. Eng., 2020 Search PubMed.
- G. Campisi, C. Paderni, R. Saccone, O. Fede, A. Wolff and L. Giannola, Human buccal mucosa as an innovative site of drug delivery, Curr. Pharm. Des., 2010, 16, 641 CrossRef CAS PubMed.
- Y. Shi, Z. Zhao, Y. Gao, D. C. Pan, A. K. Salinas, E. E. Tanner, J. Guo and S. Mitragotri, Oral delivery of sorafenib through spontaneous formation of ionic liquid nanocomplexes, J. Controlled Release, 2020, 322, 602–609 CrossRef CAS PubMed.
- M. Nurunnabi, K. N. Ibsen, E. E. Tanner and S. Mitragotri, Oral ionic liquid for the treatment of diet-induced obesity, Proc. Natl. Acad. Sci. U. S. A., 2019, 116, 25042–25047 CrossRef CAS.
- M. Viola, J. Sequeira, R. Seiça, F. Veiga, J. Serra, A. C. Santos and A. J. Ribeiro, Subcutaneous delivery of monoclonal antibodies: how do we get there?, J. Controlled Release, 2018, 286, 301–314 CrossRef CAS PubMed.
- J.-f. Jin, L.-l. Zhu, M. Chen, H.-m. Xu, H.-f. Wang, X.-q. Feng, X.-p. Zhu and Q. Zhou, The optimal choice of medication administration route regarding intravenous, intramuscular, and subcutaneous injection, Patient Prefer. Adherence, 2015, 9, 923 Search PubMed.
- C. Agatemor, T. D. Brown, Y. Gao, N. Ohmori, K. N. Ibsen and S. Mitragotri, Choline-Geranate Deep Eutectic Solvent Improves Stability and Half-Life of Glucagon-Like Peptide-1, Adv. Ther., 2021, 4, 2000180 CrossRef CAS.
- E. E. Tanner, K. M. Piston, H. Ma, K. N. Ibsen, S. Nangia and S. Mitragotri, The influence of water on choline-based ionic liquids, ACS Biomater. Sci. Eng., 2019, 5, 3645–3653 CrossRef CAS.
- A. Felipe, C. A. Lovenduski, J. L. Baker and G. E. Lindberg, Long-ranged heterogeneous structure in aqueous solutions of the deep eutectic solvent choline and geranate at the liquid–vapor interface, Phys. Chem. Chem. Phys., 2022, 24, 13720–13729 RSC.
- B. M. Rainer, S. Kang and A. L. Chien, Rosacea: Epidemiology, pathogenesis, and treatment, Derm.-Endocrinol., 2017, 9, e1361574 CrossRef PubMed.
- L. Gether, L. Overgaard, A. Egeberg and J. Thyssen, Incidence and prevalence of rosacea: a systematic review and meta-analysis, Br. J. Dermatol., 2018, 179, 282–289 CAS.
- T. T. Huynh, Burden of disease: the psychosocial impact of rosacea on a patient's quality of life, Am. Health Drug Benefits, 2013, 6, 348 Search PubMed.
- E. J. van Zuuren, Z. Fedorowicz, J. Tan, M. M. van der Linden, B. W. Arents, B. Carter and L. Charland, Interventions for rosacea based on the phenotype approach: an updated systematic review including GRADE assessments, Br. J. Dermatol., 2019, 181, 65–79 CrossRef CAS PubMed.
- J. Ko, A. Mandal, S. Dhawan, M. Shevachman, S. Mitragotri and N. Joshi, Clinical translation of choline and geranic acid deep eutectic solvent, Bioeng. Transl. Med., 2021, 6, e10191 CAS.
- B. Britton, R. Hervey, K. Kasten, S. Gregg and T. McDonald, Intraocular irritation evaluation of benzalkonium chloride in rabbits, SLACK Incorporated Thorofare, NJ, 1976, vol. 7, pp. 46–55 Search PubMed.
- M. o. t. W. Party, J. Campbell, F. Plaat, M. Checketts, D. Bogod, S. Tighe, A. Moriarty and R. Koerner, Safety guideline: skin antisepsis for central neuraxial blockade: Association of Anaesthetists of Great Britain and Ireland Obstetric Anaesthetists' Association Regional Anaesthesia UK Association of Paediatric Anaesthetists of Great Britain and Ireland, Anaesthesia, 2014, 69, 1279–1286 CrossRef PubMed.
- A. Grzybowski, P. Kanclerz and W. G. Myers, The use of povidone–iodine in ophthalmology, Curr. Opin. Ophthalmol., 2018, 29, 19–32 CrossRef PubMed.
- P. Vogt, K. Reimer, J. Hauser, O. Rossbach, H. Steinau, B. Bosse, S. Muller, T. Schmidt and W. Fleischer, PVP-iodine in hydrosomes and hydrogel—a novel concept in wound therapy leads to enhanced epithelialization and reduced loss of skin grafts, Burns, 2006, 32, 698–705 CrossRef CAS PubMed.
- I. R. SANCHEZ, K. E. NUSBAUM, S. F. SWAIM, A. S. HALE, R. A. HENDERSON and J. A. McGUIRE, Chlorhexidine diacetate and povidone-iodine cytotoxicity to canine embryonic fibroblasts and Staphylococcus aureus, Vet. Surg., 1988, 17, 182–185 CrossRef CAS PubMed.
- K. M. Whitney and C. M. Ditre, Anti-inflammatory properties of clindamycin: a review of its use in the treatment of acne vulgaris, Clin. Med. Insights: Dermatol., 2011, 4, 27 CAS.
- R. A. Bojar and K. T. Holland, Acne and Propionibacterium acnes, Clin. Dermatol., 2004, 22, 375–379 CrossRef.
- D. R. Guay, Topical clindamycin in the management of acne vulgaris, Expert Opin. Pharmacother., 2007, 8, 2625–2664 CrossRef CAS PubMed.
- R. L. Bronaugh, R. F. Stewart and E. R. Congdon, Methods for in vitro percutaneous absorption studies II. Animal models for human skin, Toxicol. Appl. Pharmacol., 1982, 62, 481–488 CrossRef CAS.
Footnote |
† Joint First authors. |
|
This journal is © The Royal Society of Chemistry 2022 |
Click here to see how this site uses Cookies. View our privacy policy here.