DOI:
10.1039/D2RA03547B
(Paper)
RSC Adv., 2022,
12, 19412-19416
Late-stage ortho-C–H alkenylation of 2-arylindazoles in aqueous medium by Manganese(I)-catalysis†
Received
8th June 2022
, Accepted 27th June 2022
First published on 4th July 2022
Abstract
Earth-abundant and water-tolerant manganese(I) catalyzed alkenylation of 2-arylindazole with alkyl and aryl alkynes through C–H bond activation is described with a unique level of E-selectivity. The reaction proceeds through the control of C3 nucleophilicity of 2-aryl indazoles. This method is applied to the late-stage functionalization of complex molecules including ethinylestradiol, norethisterone, and N-protected amino acid derivatives. The kinetic isotope studies suggest that the C–H bond activation step may not be the rate-determining step.
Introduction
Direct functionalization of inert C–H bonds is still one of the most attractive strategies due to the formation of the versatile fundamental linkage in organic compounds.1 In this context, noble metals such as iridium,2a,b ruthenium,2c–e rhodium,2f,g and palladium2h–k have been extensively employed for C–C and C–hetero atom bond formation reactions in the past two decades because of their high catalytic performance. However, these noble metal catalysts usually suffer from toxicity, low natural abundance, and high cost. In recent years, remarkable progress in 3d-transition metals such as Mn,3a Fe,3b,c Co,3d,e Ni,3f and Cu3g based C–H activations have been observed due to the metals' low price, high earth abundance, low toxicity, and potential to develop unique reactivity. In this topic, Mn-complexes typically exhibit low toxicity, thus the utilization in C–H functionalization is highly desirable.4 The Wang group first reported the alkenyation of hetero-arene with alkynes via C–H bond activation using Mn(CO)5Br as a catalyst.5 Afterward, various research groups such as Ackermann,6a Glorius,6b Kuninobu6c and others6d–j independently developed Mn-catalyzed C–H functionalization. Even though C–H bond activation in an aqueous medium is sought after, only a few examples of such reactions are known that occur in water.7
Indazole, a nitrogen-containing heterocycle is widely used in pharmaceutical and material science. In particular, antitumor, antiplatelet, anticancer, anti-inflammatory, HIV-protease inhibition, etc., are shown by indazole.8 Various marketed drugs contain indazole moiety, such as pazopanib, gamendazole, anticancer agent (MK-4827), bendazac (tyrosine kinase inhibitor, votrient), etc.9 Therefore, various methodologies have been developed for the synthesis and functionalization of indazoles because of their extensive biological applications.10
Recently few methodologies of ortho C–H activation in 2-arylindazole moiety have been developed.11 However, C–H activation of 2-aryl indazoles using water-tolerant metal-catalyst has not been explored yet. We concentrated our focus on Mn-catalyzed selective ortho-C–H functionalization of 2-aryl indazoles through the control of C3 nucleophilicity.12 Herein, we have described manganese-catalyzed ortho-C–H alkenylation of 2-arylindazole with alkyl and aryl alkynes in water (Scheme 1).
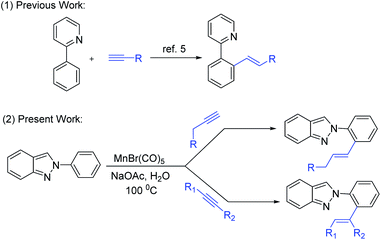 |
| Scheme 1 Indazole-Directed C–H Alkenylation. | |
Results and discussion
The study was initiated by investigating the reaction of 2-phenyl-2H-indazole (1a) and prop-2-yn-1-yl 4-methylbenzoate (2a) in the presence of Mn-catalyst. The reaction was performed using 10 mol% MnBr(CO)5 as a catalyst and 20 mol% NaOAc as an additive in 1,4-dioxane at 100 °C under N2 atmosphere. Gratifyingly, the coupling product (E)-3-(2-(2H-indazol-2-yl)phenyl)allyl 4-methylbenzoate (3aa) was obtained in 85% yield after 8 h (Table 1, entry 1). Next, we screened the effect of various Mn-catalyst like Mn2(CO)10, MnCl2, and Mn(OAc)2. But in all the cases, unsatisfactory results were observed (Table 1, entries 2–4). Further optimization was carried out using various solvents such as 1,2-DCE, THF, Toluene, DMF, CH3CN, and H2O (Table 1, entries 5–10). It was found that polar protic solvent H2O afforded the desired product in 91% yield (Table 1, entry 10). No significant improvement of the yield was observed on further screening of additives like KOAc, K2CO3, K3PO4, and Et3N (Table 1, entries 11–14). However, no product was formed in the absence of catalyst (Table 1, entry 15). Next, we checked the reaction without additives (Table 1, entry 16). In addition, varying the reaction temperature did not improve the yield of the product (Table 1, entry 17). The reaction yield did not enhance under air atmosphere, and no difunctionalized product was obtained in the presence of 2.0 equiv. of 2a (Table 1, entry 18). From these results, the optimum reaction conditions was determined to be those in entry 10.
Table 1 Optimization of the reaction conditionsa
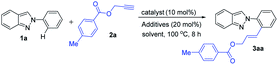
|
Entry |
Catalyst (10 mol%) |
Additives (20 mol%) |
Solvent (2.0 mL) |
Yield (%) |
Reaction conditions: All reactions were carried out with 0.25 mmol of 1a, 0.3 mmol of 2a, 10 mol% of MnBr(CO)5, 20 mol% NaOAc in 2.0 mL solvent for 8 h at 100 °C under N2. Stirred at 120 °C. Stirred at 80 °C. Under air. 2.0 equiv. of 2a was used. nr = no reaction. |
1 |
MnBr(CO)5 |
NaOAc |
1,4-Dioxane |
85 |
2 |
Mn2(CO)10 |
NaOAc |
1,4-Dioxane |
65 |
3 |
MnCl2 |
NaOAc |
1,4-Dioxane |
nr |
4 |
Mn(OAc)2 |
NaOAc |
1,4-Dioxane |
nr |
5 |
MnBr(CO)5 |
NaOAc |
1,2-DCE |
61 |
6 |
MnBr(CO)5 |
NaOAc |
THF |
46 |
7 |
MnBr(CO)5 |
NaOAc |
Toluene |
52 |
8 |
MnBr(CO)5 |
NaOAc |
DMF |
21 |
9 |
MnBr(CO)5 |
NaOAc |
CH3CN |
10 |
10 |
MnBr(CO)5 |
NaOAc |
H2O |
91 |
11 |
MnBr(CO)5 |
KOAc |
H2O |
78 |
12 |
MnBr(CO)5 |
K2CO3 |
H2O |
62 |
13 |
MnBr(CO)5 |
K3PO4 |
H2O |
57 |
14 |
MnBr(CO)5 |
Et3N |
H2O |
45 |
15 |
— |
NaOAc |
H2O |
nr |
16 |
MnBr(CO)5 |
— |
H2O |
36 |
17 |
MnBr(CO)5 |
NaOAc |
H2O |
67b, 62c |
18 |
MnBr(CO)5 |
NaOAc |
H2O |
69d, 72e |
After getting the optimized reaction conditions for the manganese-catalyzed C–H alkenylation in hand, we next investigated its versatility (Table 2). The electron-donating (–Me), halogen (–Cl), and electron-withdrawing (–CN) substituted benzoic acid derived alkynes smoothly underwent with indazole (1a) through C–H activation, effectively providing the expected products (3aa–3ac) with excellent yields even on a gram scale of 3aa. Nicotinic acid derived alkyne was examined, furnishing the corresponding alkenylated product 3ad in 78% yield. It is noteworthy that aliphatic acids like pivalic acid, cyclohexanecarboxylic acid, and adamantane-1-carboxylic acid derived alkynes were successfully employed, leading to the desired products 3ae–3ag in good to excellent yields of 89–96%. Pent-4-yn-1-yl benzoate (2h) was treated with 2-phenyl-2H-indazole (1a), and the expected alkenylation reaction occurred with moderate yield. Remarkably, phenol, aniline, and thiophenol derivatives alkynes were efficiently converted to the desired products 3ai–3ak with complete E-selectivity. Specifically, N-Boc-protected amino acid derivatives 2l and 2m were well tolerated, which will be precious for further post-synthetic application. Furthermore, N-Boc-protected indoline-2-carboxylic acid derived alkyne (2n) was converted to the desired product in 72% yield. Inspired by the incredible versatility of Mn(I)-catalyzed alkenyation, we became interested in performing this alkenylation reaction with more challenging steroid structures. In this regard, ethinylestradiol and norethisterone took part effectively in the reaction to deliver 3ao and 3ap in 68% and 60% yields, respectively. Again, 1-octyne was smoothly reacted, giving the desired product 3aq in 57% yield with excellent E-selectivity. Moreover, 3-hexyne was reacted with 2-phenyl-2H-indazole (1a) under the standard reaction conditions, but unfortunately obtained an inseparable mixture.
Table 2 Substrate Scope of Alkynesa
Reaction conditions: 0.25 mmol of 1, 0.3 mmol of 2 in presence of 10 mol% of MnBr(CO)5, 20 mol% NaOAc in 2.0 mL of H2O at 100 °C for 8 h under N2. 5 mmol scale. |
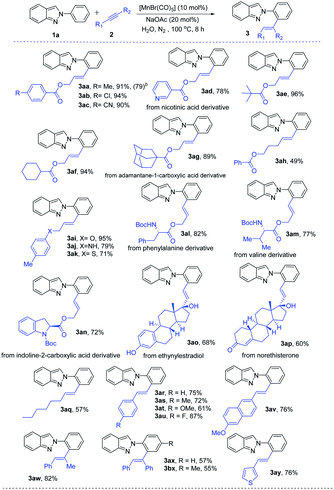 |
A number of electron-neutral and -donating (–Me, –OMe) and electron-deficient (–F) phenylacetylenes (2) were taken part in the reaction with 2-arylindazole (1a) to afford the desired products (3ar–3au) up to 87% yields. 2-Ethynyl-6-methoxynaphthalene (2v) was also amenable to this method. Moreover, internal alkyne (2w and 2x) also provided ortho-alkenyated product 3aw–3bx in moderated yields (55–82%). Interestingly, heteroaryl substituted alkyne such as 3-ethynyl thiophene (2y) was successfully converted into the corresponding alkenylated product 3ay in decent yields. The regioselectivity of unsymmetrical alkynes (direction of alkynes) have been determined from the 1H-NMR data and coupling constant (J) values.
Motivated by the versatility of the Mn(I)-catalysis, a variety of indazoles were examined. Electron-donating group (–Me) at the phenyl ring of 2-arylindazole gave the corresponding alkenylated product 3br in excellent yields. Halogen (–F, –Cl, and –Br) containing substrates produced the desired products (3cr–3er) in moderate to good yields. An electron-withdrawing (–COOEt) group containing 2H-indazole was well tolerated in this method and gave the expected products in moderate yields (3fr). meta-Substituted 2-arylindazoles (1g and 1h) were also reacted under the optimal reaction conditions (3gr and 3hr) with good yields. 2H-indazoles, bearing halogens (–F and –Cl) on the C-5 position of arene, successfully reacted to produce the desired products (3ir and 3kr). Furthermore, disubstituted indazoles (1j, 1l, and 1m) were explored and gave the corresponding products without any difficulties (3jr, 3lr, and 3mr). On the other side, due to the difficulty in the formation of a five-membered mangancyclic intermediate, indazole substituted at the ortho-position did not produce the desired product. Interestingly, similar N-containing heterocycles such as 2-phenylpyridine (1n) and 1-phenyl-1H-pyrazole (1o) also reacted with N-Boc-protected amino acid derivative alkyne (2l) and provided their corresponding products 3nl and 3ol in 86% and 88% yields, respectively (Table 3).
Table 3 Substrate Scope of Hetrocyclesa
Reaction conditions: 0.25 mmol of 1, 0.3 mmol of 2 in presence of 10 mol% of MnBr(CO)5, 20 mol% NaOAc in 2.0 mL of H2O at 100 °C for 8 h under N2. |
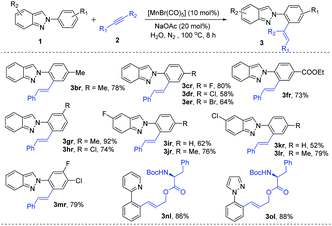 |
The deuterium labeling experiment, kinetic isotope effect (KIE), and parallel experiments were performed to realize the mechanistic pathway of this reaction (Scheme 2). Initially, a H/D exchange experiment was executed under the optimized reaction conditions using D2O as a co-solvent which led to the incorporation of deuterium at ortho-position of the phenyl ring (Scheme 2a). This result indicated that the reversible cleavage of C–H bonds might be involved in the reaction. In addition, the intermolecular competition reaction (Scheme 2b) and parallel reaction (Scheme 2c) gave low KIE values of 1.0 and 1.1, respectively. This data suggested that the C–H bond cleavage step was unlikely to be the rate-determining step.
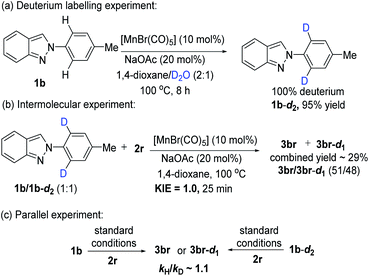 |
| Scheme 2 Control experiments. | |
Based on the above results and previous reports,4,5 a plausible reaction mechanism is shown in Scheme 3. At first, NaOAc-assisted cyclomanganation of 2H-indazole (1b) forms a five-membered manganacycle A. Coordination of alkyne (2) with A gives intermediate B. Subsequently, migratory insertion of alkyne generates intermediate C. Finally, protonation of C furnishes the desired product, and regenerate the active Mn(I) complex to complete the catalytic cycle.
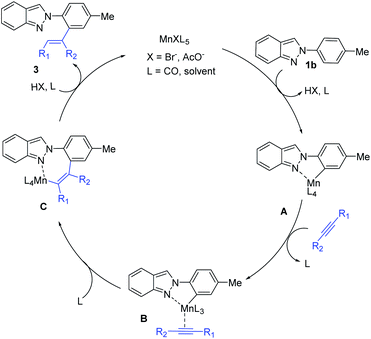 |
| Scheme 3 Plausible mechanistic pathway. | |
Conclusions
In summary, we have successfully developed a highly stereo- and regioselective Mn(I)-catalyzed ortho-alkenylation of indazole with various alkynes. The salient features of this methodology includes (1) robust C–H activation in H2O, (2) Mn(I)-catalyzed C–H functionalization, (3) broad substrate scope and good to excellent yields, (4) excellent E-stereoselectivity, (5) the late-stage functionalization of complex molecules, (6) scalable. We believe this method will gain much attraction in organic synthesis, pharmaceutical chemistry, and as well as in material sciences.
Conflicts of interest
There are no conflicts to declare.
Acknowledgements
A. H. acknowledges the financial support from SERB, New Delhi (Grant no. CRG/2020/000362). K.K.D (CSIR-JRF) and A.K.G (CSIR-SRF) thanks CSIR-New Delhi for their fellowship.
Notes and references
-
(a) L. McMurray, F. O'Hara and M. J. Gaunt, Chem. Soc. Rev., 2011, 40, 1885 RSC;
(b) U. Dutta, S. Maiti, T. Bhattacharya and D. Maiti, Science, 2021, 372, 701 CrossRef PubMed.
-
(a) Ł. Woźniak, J.-F. Tan, Q.-H. Nguyen, A. Madron du Vigné, V. Smal, Y.-X. Cao and N. Cramer, Chem. Rev., 2020, 120, 10516 CrossRef PubMed;
(b) M. Nagai, M. Nagamoto, T. Nishimura and H. Yorimitsu, Chem. Lett., 2017, 46, 1176 CrossRef CAS;
(c) P. B. Arockiam, C. Bruneau and P. H. Dixneuf, Chem. Rev., 2012, 112, 5879 CrossRef CAS PubMed;
(d) A. Mandal, R. Bera and M. Baidya, J. Org. Chem., 2021, 86(1), 62 CrossRef CAS PubMed;
(e) P. Kumar and M. Kapur, Org. Lett., 2019, 21, 2134 CrossRef CAS PubMed;
(f) D. A. Colby, R. G. Bergman and J. A. Ellman, Chem. Rev., 2010, 110, 624 CrossRef CAS PubMed;
(g) W. Zhu and T. B. Gunnoe, Acc. Chem. Res., 2020, 53, 920 CrossRef CAS PubMed;
(h) T. W. Lyons and M. S. Sanford, Chem. Rev., 2010, 110, 1147 CrossRef CAS PubMed;
(i) Z. Zhang, K. Tanaka and J.-Q. Yu, Nature, 2017, 543, 538 CrossRef CAS PubMed;
(j) S. Porey, X. Zhang, S. Bhowmick, V. Kumar Singh, S. Guin, R. S. Paton and D. Maiti, J. Am. Chem. Soc., 2020, 142(8), 3762 CrossRef CAS PubMed;
(k) L. Jin, P. Zhang, Y. Li, X. Yu and B.-F. Shi, J. Am. Chem. Soc., 2021, 143, 12335 CrossRef CAS PubMed.
-
(a) W. Liu and L. Ackermann, ACS Catal., 2016, 6, 3743 CrossRef CAS;
(b) R. Shang, L. Ilies and E. Nakamura, Chem. Rev., 2017, 117, 9086 CrossRef CAS PubMed;
(c) E. Nakamura, T. Hatakeyama, S. Ito, K. Ishizuka, L. Ilies and M. Nakamura, Org. React., 2004, 83, 1 Search PubMed;
(d) K. Gao and N. Yoshikai, Acc. Chem. Res., 2014, 47, 1208 CrossRef CAS PubMed;
(e) S. Debbarma, S. S. Bera and M. S. Maji, J. Org. Chem., 2016, 81, 11716 CrossRef CAS PubMed;
(f) N. A. Harry, S. Saranya, S. M. Ujwaldev and G. Anilkumar, Catal. Sci. Technol., 2019, 9, 1726 RSC;
(g) M. L. Kantam, C. Gadipelly, G. Deshmukh, K. R. Reddy and S. Bhargava, Chem. Rec., 2019, 19, 1302 CrossRef CAS PubMed.
-
(a) S. Ghosh, T. Khandelia and B. K. Patel, Org. Lett., 2021, 23, 7370 CrossRef CAS PubMed;
(b) L. Shi, X. Zhong, H. She, Z. Lei and F. Li, Chem. Commun., 2015, 51, 7136 RSC;
(c) T. Jia and C. Wang, ChemCatChem, 2019, 11, 5292 CrossRef CAS;
(d) S. Wan, Z. Luo, X. Xu, H. Yu, J. Li, Y. Pan, X. Zhang, L. Xu and R. Cao, Adv. Synth. Catal., 2021, 363, 2586 CrossRef CAS;
(e) D. Das, A. A. Bhosle, P. C. Panjikar, A. Chatterjee and M. Banerjee, ACS Sustainable Chem. Eng., 2020, 8, 19105 CrossRef CAS;
(f) J. Son, Beilstein J. Org. Chem., 2021, 17, 1733 CrossRef CAS PubMed.
- B. Zhou, H. Chen and C. Wang, J. Am. Chem. Soc., 2013, 135, 1264 CrossRef CAS PubMed.
-
(a) N. Kaplaneris, J. Son, L. Mendive-Tapia, A. Kopp, N. Barth, I. Maksso, M. Vendrell and L. Ackermann, Nat. Commun., 2021, 12, 3389 CrossRef CAS PubMed;
(b) S. Cembellín, T. Dalton, T. Pinkert, F. Schäfers and F. Glorius, ACS Catal., 2020, 10, 197 CrossRef;
(c) B. Liu, J. Li, P. Hu, X. Zhou, D. Bai and X. Li, ACS Catal., 2018, 8, 9463 CrossRef CAS;
(d) A. Kumar, N. Muniraj and K. R. Prabhu, Adv. Synth. Catal., 2019, 361, 4933 CrossRef CAS;
(e) Y.-X. Tan, X.-Y. Liu, Y.-S. Zhao, P. Tian and G.-Q. Lin, Org. Lett., 2019, 21, 5 CrossRef PubMed;
(f) S. Sueki, Z. Wang and Y. Kuninobu, Org. Lett., 2016, 18, 304 CrossRef CAS PubMed;
(g) H. Khatua, S. K. Das, S. Roy and B. Chattopadhyay, Angew. Chem., Int. Ed., 2021, 60, 304 CrossRef CAS PubMed;
(h) C. Wang and M. Rueping, ChemCatChem, 2018, 10, 2681 CrossRef CAS;
(i) X. Yang, X. Jin and C. Wang, Adv. Synth. Catal., 2016, 358, 2436 CrossRef CAS;
(j) G.-X. Kong, J.-N. Han, D. Yang, J.-L. Niu and M.-P. Song, Org. Biomol. Chem., 2019, 17, 10167 RSC.
-
(a) H. Wang, M. M. Lorion and L. Ackermann, Angew. Chem., Int. Ed., 2017, 56, 6339 CrossRef CAS PubMed;
(b) X. Kong, L. Lin and B. Xu, Adv. Synth. Catal., 2018, 360, 2801 CrossRef CAS.
-
(a) W. Han, J. C. Pelletier and C. N. Hodge, Bioorg. Med. Chem. Lett., 1998, 8, 3615 CrossRef CAS PubMed;
(b) F.-Y. Lee, J.-C. Lien, L.-J. Huang, T.-M. Huang, S.-C. Tsai, C.-M. Teng, C.-C. Wu, F.-C. Cheng and S.-C. Kuo, J. Med. Chem., 2001, 44, 3746 CrossRef CAS PubMed;
(c) M. D. De Lena, V. Lorusso, A. Latorre, G. Fanizza, G. Gargano, L. Caporusso, M. Guida, A. Catino, E. Crucitta, D. Sambiasi and A. Mazzei, Eur. J. Cancer, 2001, 37, 364 CrossRef CAS PubMed.
-
(a) H. Shen, S. Gou, J. Shen, Y. Zhu, Y. Zhang and X. Chen, Bioorg. Med. Chem. Lett., 2010, 20, 2115 CrossRef CAS PubMed;
(b) A. Veerareddy, G. Surendrareddy and P. K. Dubey, Synth. Commun., 2013, 43, 2236 CrossRef CAS.
-
(a) Y. Lian, R. G. Bergman, L. D. Lavis and J. A. Ellman, J. Am. Chem. Soc., 2013, 135, 7122 CrossRef CAS PubMed;
(b) S. Vidyacharan, A. Murugan and D. S. Sharada, J. Org. Chem., 2016, 81, 2837 CrossRef CAS PubMed;
(c) S. Vivek Kumar, S. Ellairaja, V. Satheesh, V. Sivasamy Vasantha and T. Punniyamurthy, Org. Chem. Front., 2018, 5, 2630 RSC;
(d) S. Guo, L. Sun, X. Li, X. Zhang and X. Fan, Adv. Synth. Catal., 2020, 362, 913 CrossRef CAS;
(e) K. Mahanty, D. Maiti and S. De Sarkar, J. Org. Chem., 2020, 85, 3699 CrossRef CAS PubMed;
(f) S. Neogi, A. K. Ghosh, K. Majhi, S. Samanta, G. Kibriya and A. Hajra, Org. Lett., 2020, 22, 5605 CrossRef CAS PubMed;
(g) S. Ghosh, S. Mondal and A. Hajra, Adv. Synth. Catal., 2020, 362, 3768 CrossRef CAS.
-
(a) P. Ghosh, S. Samanta and A. Hajra, Org. Biomol. Chem., 2020, 18, 1728 RSC;
(b) A. K. Ghosh, S. Samanta, P. Ghosh, S. Neogi and A. Hajra, Org. Biomol. Chem., 2020, 18, 3093 RSC;
(c) J. Li, L. Shi, S.-P. Zhang, X.-Y. Wang, X. Zhu, X.-Q. Hao and M.-P. Song, J. Org. Chem., 2020, 85, 10835 CrossRef CAS PubMed;
(d) A. K. Ghosh, P. Ghosh and A. Hajra, J. Org. Chem., 2020, 85, 15752 CrossRef CAS PubMed.
- A. K. Ghosh, K. K. Das and A. Hajra, Adv. Synth. Catal., 2021, 363, 4974 CrossRef.
|
This journal is © The Royal Society of Chemistry 2022 |
Click here to see how this site uses Cookies. View our privacy policy here.