DOI:
10.1039/D2RA03077B
(Paper)
RSC Adv., 2022,
12, 19108-19114
Extraction and preconcentration of Ni(II), Pb(II), and Cd(II) ions using a nanocomposite of the type Fe3O4@SiO2@polypyrrole-polyaniline†
Received
16th May 2022
, Accepted 13th June 2022
First published on 30th June 2022
Abstract
In this study, the application of Fe3O4@SiO2@polypyrrole-polyaniline magnetic nanocomposite was studied for Ni(II), Cd(II), and Pb(II) ions preconcentration extraction. In this regard, the silica layer prevents the Fe3O4 nanoparticles (NPs) from aggregating over a broad pH range value and simultaneously improves chemical stability and hydrophilicity. By using a Box–Behnken design, the effect of various parameters affecting the preconcentration was studied. FAAS was employed to quantify the eluted analytes. The detection limits are 0.09, 1.1, and 0.3 ng mL−1 for Ni(II), Cd(II) and Pb(II), ions, respectively. The relative standard deviations (RSDs%) were calculated for determining the method's precision, lower than 7.5%. The capacities of sorption are 75, 84, and 98 mg g−1, respectively. With the usage of a certified reference material, the developed method was validated. After that, the validated method was employed to rapidly extract trace target ions from food samples and gave satisfactory results.
1 Introduction
Heavy metals, which have been extensively utilized in diverse manufacturing processes such as combustion of fossil fuels, plating steel, metal forging, electronics, metallurgy, smelting, mining, electroplating, manufacturing of alkaline storage batteries, photographic materials, explosive manufacturing traffic, metal finishing, textiles, ceramics, printing, pigments, fuels and many others are unavoidably discharged into the environmental waters.1–3 These species can enter the human body via the food chain and endanger human health even at a low concentration.2,3 The biological molecules containing N, O, and S elements show a reasonable propensity for forming complexes to heavy metals.4,5 These interplays result in the breaking of hydrogen bonds, causing changes in the molecular structure of proteins and enzymes inhibition.4 The carcinogen and toxicity effects of heavy metal ions like those affecting the central nervous system (As(III), Hg(II) and Pb(II)); or skin, bones, or teeth (Ni(II), Cu(II), Cd(II), Cr(III)); the kidneys or liver (Hg(II), Pb(II), Cu(II), Cd(II));6–8 can be explained with these interactions.4
Consequently, monitoring and determining the proportion of heavy metal ions in food samples for an analytical chemist is necessary for identifying and monitoring toxicants in environmental samples. Analytical chemists. Identifying and monitoring these toxicants in the mentioned samples. Therefore, developing a quick, simple, sensitive, green, and reliable method for monitoring heavy metals and determination is crucial.9–11
Various preconcentration techniques such as ion exchange,13 chemical precipitation,14 liquid–liquid extraction,15 cloud point extraction,16 and solid-phase extraction (SPE) engineered for preconcentration of natural matrices, and heavy metal extraction,17–20 since the complexity of matrices, are mainly an issue, and proportion of the heavy metals in the actual samples is considerably low.12
SPE is the most widely employed extraction method for pre-concentrating heavy metals from actual samples among the mentioned techniques. Simplicity, rapidity, cost-effectiveness, and low consumption of reagents are the main reasons for widespread SPE utilization.21 By the emergence of SPE, a wide range of sorbents like carbon nanotubes and graphene oxide,22–25 modified porous materials,26,27 and magnetic nanoparticles28–30 have been used.
In the past decades, utilizing nanostructured materials in analytical chemistry, specifically, the usage of Fe3O4 as a magnetic nanoparticle, attracted great attention since magnetic Fe3O4 nanoparticles (Fe3O4 NPs) not only has the nanometer-sized materials general features but also demonstrates magnetic property.31–33 In many cases, major environmental problems arose due to the difficulties of completely removing and separating sorbents from sample solutions.34 Magnetic materials are capable of employing an external magnetic field; thus, they can simplify the solid sorbent's isolation from the sample solution and facilitate sample preparation procedures.35 However, Fe3O4 nanoparticles which tend to oxidize, are not selective regarding complex matrices and prone to aggregate. Thus, surface functionalization of bare Fe3O4 NPS allows a reduction in aggregation and leads to higher selectivity. Another way to address this problem is core–shell nanostructures. The strong superparamagnetic properties of Fe3O4 NPs make them a perfect candidate for the synthesis of core–shell nanostructures in which these NPs act as the core. Among the various shells, due to the several unique characteristics of silica, it is an ideal selection: (a) silica shell can solve the aggregation problem of magnetite NPs in a broad pH range and reinforces their chemical stability, (b) silane coupling agents are able to modify the silica surface, and consequently, they can be modified by diverse chelators and (c) the layers of SiO2 also have an excellent hydrophilicity behavior.36,37 From the theory of hard–soft–acid–base (HSAB), most heavy metal ions are soft acids, and they are capable of forming strong bonds with molecules/ions having S and N atoms which behave as a soft base.
The Fe3O4@SiO2@polypyrrole-polyaniline (Fe3O4@SiO2@PPy-PANI) magnetic nanocomposite is used in this work as a nano-sorbent for the purpose of preconcentration and rapid separation of Cd(II), Ni(II), and Pb(II) ions from numerous food samples. Characterization tests of sorbent were carried out with the Fourier transform infrared spectroscopy (FT-IR), elemental analysis, vibrating sample magnetometer (VSM), and transmission electron microscopy (TEM). Due to the superparamagnetic behavior of the nano sorbent, quick and straightforward separation of the solid materials is possible. Coating the magnetite NPs with PANI-PPy copolymer enhanced the selectivity and sorption capacity of the sorbents towards target analytes. Also, sorption capacity and surface area enhanced due to the nanosized property of sorbent. The optimum extraction conditions exploration was done with designing experiments approach with the help of the response surface methodology. In the final step, the extractive preconcentration and quantification of the target ions in diverse agricultural and sea food samples, conducted with the usage of the nano sorbent.
2 Experimental
2.1 Solutions and reagents
All analytical grade reagents including HCl, tetraethyl orthosilicate (TEOS), KCl, ammonium hydroxide (25%), FeCl2, HNO3, ammonium persulfate (APS), NaOH, FeCl3, aniline (Ani), pyrrole (Py), (ethanol, methanol, and acetone) provided by the Merck company or Fluka and were utilized without any additional purification procedure. Stock solutions (1000 mg L−1) of Pb(II), Cd(II) and Ni(II) ions were acquired from Merck (Darmstadt, Germany). The distilled water used for the preparation of samples was double distilled water.
2.2 Instrumentation
All information about the instrumentation are mentioned in ESI.†
2.3 Solutions
In a microwave digestion chamber, the reference material was prepared by using 6 mL HCl (37% (w/w)) and 2 mL HNO3 (65% (w/w)). Solutions were kept at room temperature, and the following program was accomplished: 2 min at 250 W, no radiation for 2 min, 6 min at 250 W, 5 min at 400 W, 8 min at 550 W the venting process was done for 8 min.38 Afterward, double distilled water was utilized for dilution of the residue.38 1000 mg L−1 solutions of K+, Al(III), Zn(II), Sn(II), Mn(II), Fe(III), Hg(II), Na+, PO43−, Ca(II), Mg(II), CrO42−, Co(II), Cu(II), and Cr(III) were prepared in the solution of nitric acid 2% (v/v). The preparation of the working solution with double distilled water was done by stepwise dilution of the stock solution.
2.4 Fe3O4@SiO2@polypyrrole-polyaniline magnetic nanocomposite synthesis
2.4.1 Fe3O4@silica synthesis. Previously we reported the NPs to synthesize procedure in which we used 25 mL 0.4 mol L−1 HCl solution to dissolve 5.2 g of FeCl3·6H2O and 2.0 g of FeCl2.35 In the next step, under N2 atmosphere, the iron salts mixture was added into 250 mL of 1.5 mol L−1 sodium hydroxide dropwise and heated for 30 min at 80 °C.35 Afterward, the black precipitate was isolated by a strong magnet with the size of 15 cm × 12 cm × 5 cm (1.4 Tesla). With the usage of distilled water and ethanol, the black precipitate was washed three times. As the next step, under vacuum conditions, magnetite NPs were dried for about six h. Then, in a 200 mL deionized water solution, 50 mL ethanol, 1.0 g of synthesized magnetite NPs and 3 mL NH4OH (25%) were mixed. Afterward, under vigorous stirring, 2.5 mL of TEOS was added steadily to synthesize the Fe3O4@SiO2 core–shell NPs into the reaction mixture.39 Using a permanent magnet, the Fe3O4@SiO2 NPs were isolated after 12 h stirring at 40 °C, then at room temperature, it was washed with ethanol and finally dried (Fig. 1).
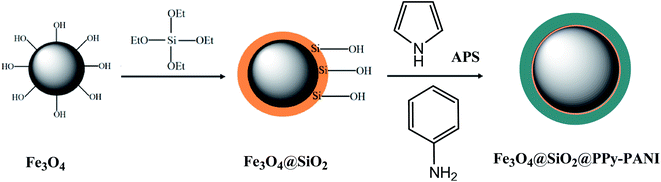 |
| Fig. 1 A schematic diagram for the synthesis of Fe3O4@SiO2@PPy-PANI magnetic nanocomposite. | |
2.4.2 Fe3O4@SiO2 nanoparticles modification with copolymer. The Fe3O4@SiO2 core–shell nanoparticles modifying procedure is given in two steps: At first, in 150 mL of HCl solution (1.0 mol L−1), 1.0 g of Fe3O4@silica was dispersed and sonication was conducted for about 15 min. Then 1.0 mL Py and 1.3 mL Ani were added to the system and stirred for 30 min. Next, the temperature of the solution dropped to 0 °C as the next step, and in 1.0 mol L−1 HCl solution, the stoichiometric concentration of APS was added dropwise. The polymerization process continued for about 12 h, and finally. As a result, a precipitate was required.40 Subsequently, utilizing a strong magnet, the Fe3O4@SiO2@PPy-PANI nano-material was isolated and washed with H2O and CH3OH continuously to ensure no possible unreacted reagents in the system and finally, at room temperature, they dried. A schematic diagram is illustrated in Fig. 1 for the Fe3O4@SiO2@PPy-PANI nanocomposite synthesis.
2.5 Extraction process
In the batch mode, extraction of target ions was accomplished. By employing 50 mL 0.5 μg mL−1 to mixture solutions, the sorption process was accomplished. Briefly, 1.0 mol L−1 HCL and 1.0 mol L−1 NH3 were added dropwise to adjust the solutions' pH. After that, Fe3O4@SiO2@PPy-PANI nanocomposite was added to the solutions. For complete extraction of the metal ions from the solution, it was agitated for a predetermined time.41 Based on concentration level change before and after the process with the help of FAAS, the absorbed amounts of the ions were calculated. The following equation determined sorption percentage:
Each analyte's initial and final concentrations (mg L−1) represented by CA and CB, respectively. The elution process was accomplished by preparing 5.4 mL of 1.7 mol L−l HNO3 solution as the eluent. After that, with the help of the strong magnet, the sorbent was isolated from the solution. At the end, the metal ions clear solution was injected into FAAS to determine each ion's recovery.
2.6 Real sample pretreatment
The actual sample and certified reference materials pretreatment details are mentioned in ESI.†
3 Results and discussion
3.1 Material selection
Extraction recovery of the target ions is affected by the sorbent type. In this context, firstly, Fe3O4 NPs are covered with a silica layer to prevent aggregation of Fe3O4 NPs and improve their acid resistance. In this case, a protecting layer such as SiO2 is needed since solutions of strong acids are used as eluent for solid-phase extraction of metal ions, which may dissolve Fe3O4 NPs. Moreover, the surface of silica has the silanol moieties, which silane coupling agents can functionalize, and subsequently, various ligands can be immobilized on its surface. In the next step, Fe3O4@SiO2 NPs are coated with a copolymer of aniline and pyrrole, which enhances the selectivity and sorption capacity of the nano-material towards the target species. Coordinating performance in the copolymer coating is enhanced due to its atoms being utilized as a proper functionalizing agent. With a simple and economical method, Fe3O4 can be synthesized in large quantities. Owing to the high surface area, these NPs have high sorption capacity. They are superparamagnetic, which means their separation from the extraction medium can be simply performed by employing a permanent magnet.5 Hence, the extraction ability of magnetite, Fe3O4@silica, Fe3O4@silica@PANI, Fe3O4@silica@PPy, and Fe3O4@silica@PANI-PPy sorbents was compared. The results indicated the efficiency of extraction. The Fe3O4@silica@PANI-PPy sorbent is higher than the others. The higher performance of Fe3O4@silica@PANI-PPy may be related to the possibility of chelate formation (more efficient interaction) with metal ions. Thus, it was considered as the best sorbent in this study.
3.2 Characterization
3.2.1 CHN analysis and FT-IR spectra. The KBr pellet method was utilized for recording the FT-IR spectra of Fe3O4@SiO2@PANI-PPy nanocomposite. Owing to the coating of Fe3O4@SiO2 NPs with PANI-PPy copolymer, the absorption peaks can be observed at 3403 cm−1 (N–H), 1579 cm−1 (C
N), and 1495 cm−1 (C
C) additionally, CHN analysis exhibited the presence of 2.1% N in the structure of Fe3O4@SiO2@PANI-PPy nanocomposite which proves the successful modification of NPs with PPy-PANI copolymer.
3.2.2 Transmission electron microscopy. TEM characterization technique was used (Fig. 2a) for studying the dimension of Fe3O4@SiO2@PANI-PPy NPs and its surface morphology. Fig. 2a demonstrates a relatively thin layer of SiO2 successfully coated Fe3O4 nanoparticles. Two recognizable regions with distinct electron densities can be observed in this figure which verifies that a core–shell structure is formed successfully.36 The electron-dense region is related to average-sized magnetite cores around 15 nm (Fig. 1S†). The more translucent and less-dense region around the cores is the SiO2 shell thickness of around 15 nm (Fig. 1S†).
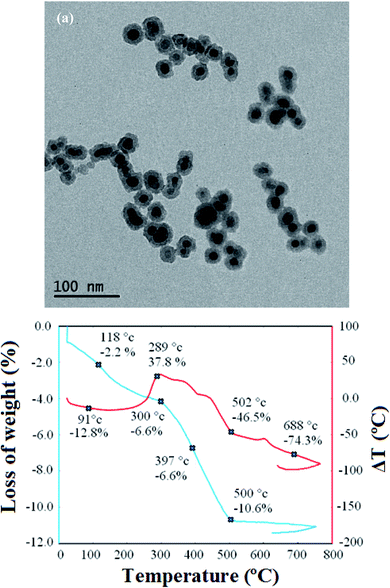 |
| Fig. 2 (a) The TEM image and (b) the TG plot of the Fe3O4@SiO2@PPy-PANI magnetic nanocomposite. | |
3.2.3 Thermogravimetric analysis. The thermal analysis explored the thermal behavior and stability of the modified Fe3O4@SiO2 NPS. Fig. 2b depicts the thermogravimetric (TG) plot of the nanocomposite. There were two thermal phenomena which the first one was related to the desorption of water molecules and took place up to 100 °C. From the TG curve also, it can be seen that the sorbent weight loss is around 10.2% when the sample is heated up to 500 °C, and subsequently, it is safe to say that the fabricated nano-material is stable. The weight loss of 6.6% in the range of 300–500 °C is related to the degradation of polymer layer. The results confirm that the formation of Fe3O4@SiO2@PANI-PPy nanocomposite was performed successfully.42
3.2.4 VSM and dynamic light scattering analyses. The sorbents must have superparamagnetic properties to separate under a magnetic field quickly. At room temperature, the magnetic properties of Fe3O4@SiO2, Fe3O4, and Fe3O4@SiO2@PANI-PPy with utilizing the magnetic property measurement were investigated, and the correlating curves can be seen in Fig. 3. As illustrated in this figure, the saturation magnetization of Fe3O4, Fe3O4@SiO2, and Fe3O4@SiO2@PANI-PPy is 70, 40, and 26 emu g−1, respectively, which is enough for MSPE process. It is known that the magnetization is zero when the external field approaches zero for superparamagnetic sorbent. In this work, the curve for hysteresis of Fe3O4@SiO2@PANI-PPy NPs passed through the zero point and exhibited super-paramagnetic features.43
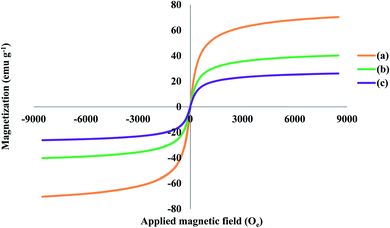 |
| Fig. 3 Vibrating sample magnetometry plots of (a) Fe3O4 (b) Fe3O4@SiO2@ and (c) Fe3O4@SiO2@PPy-PANI NPs. | |
The size of Fe3O4@SiO2@PANI-PPy NPS also was explored by the DLS technique. The DLS size distribution of the sorbent is illustrated in Fig. 2S (ESI†). As depicted, the average diameter of Fe3O4@SiO2@PANI-PPy NPs was about 35 nm with a relatively narrow distribution which demonstrates that there are no severe aggregates.
3.3 Method optimization
The parameters of (a) the eluent, (b) the sorption and (c) the elution step was optimized. In the ESI, the figures and data are represented (Fig. 3S and 4S, Table 1S†). The sorption time of samples are in the order of 9.0 min, and pH value is 6.2; application of 10 mg Fe3O4@SiO2@PANI-PPy NPs in the step of sorption, and the usage of a 1.7 mol L−l HNO3 as eluent with the volume of 5.4 mL; and finally for the elution time of 11.5 min had the best sorption and extraction recoveries.
3.4 Breakthrough volume
The preconcentration factor is affected in this regard, by spiking 1 mg Ni(II), Pb(II) and Cd(II) ions in the range of 250–1750 mL solution, samples volume influence was studied thoroughly. After that, the SPE process was accomplished, and the results revealed that the recovery of the analytes remained quantitative (>95%) up to 1000 mL. Thereby, the simultaneous quantitative recovery of target ions on Fe3O4@SiO2@PANI-PPy nano sorbent can be obtained up to 1000 mL of sample volumes. Based on the sample and eluent volume, an enrichment factor of 185 is obtained that is desirable.
3.5 Interference assay
For the study of Fe3O4@SiO2@PANI-PPy nano sorbent selectivity, the effect of various metal ions on the target ions extraction recovery was investigated. In Table 2S,† we summarized the tolerance of these potentially interfering ions (ESI,† ESM). From this information at pH 6.2, it is safe to say that high concentrations of potentially interfering species indicated no significant effect on the recovery of the analytes. Thereby, the developed extraction method can be employed for the determination of the target analytes in complicated samples reasonably.
3.6 Sorption capacity study
Fe3O4@SiO2@PANI-PPy nano sorbent's sorption capacity (AC) was studied by using a known concentration of each ion. After sorption, the equilibrium amounts of the target metals were established by FAAS. Accordingly the maximum AC of the new nano-material for Pb(II), Ni(II), and Cd(II) ions, was 84, 98, and 75 mg g−1, respectively.
3.7 Analytical features and method validation
Under the optimum conditions, calibration plots were attained to determine target ions. The linear range was 0.3–100 ng mL−1 for Cd(II), 3–150 ng mL−1 for Pb(II), and 1–130 ng mL−1 for Ni(II). The determination correlation (r2) was around 0.994 for Pb(II), 0.995 for Cd(II) and 0.996 for Ni(II) ions. Based on LOD = 3Sb/m, the detection limits were calculated. Ten replicate blank signals of the standard deviation, represented by Sb and calibration plot slope is m after the extraction process. When samples volume was 1000 mL, LOD calculated for Pb(II) about 1.1 ng mL−1 and for Ni(II) ions and Cd(II) is around 0.3 ng mL−1 and 0.09 ng mL−1, respectively. The repeatability of proposed MSPE method was evaluated in the 30 ng mL−1 of Ni(II), Pb(II), and Cd(II) ions (n = 3) standard solution and the relative standard deviations (RSDs%) are 6.8, 5.4 and 7.5% respectively.
To determine the reliability of the new method, the 02-2932 CRM seafood mix used, under the optimal conditions, target ions were determined in this sample. A reasonable correlation was observed between the actual value of the mentioned ions in CRM (Table 1) and the obtained value by the developed method. Thereby, the present method can be employed as an accurate and reliable procedure for the preconcentration and determination of target analytes in actual samples.
Table 1 Determination of Cd(II), Pb(II) and Ni(II) in sea food mix certified reference material
Concentration (mg kg−1) |
Relative error (%) |
Element |
Certified |
Found |
Cd |
4.764 |
4.63 |
−2.8 |
Pb |
2.779 |
2.68 |
−3.6 |
Ni |
3.071 |
2.90 |
−5.6 |
Besides, the performance of the developed extraction method is compared with other methods (ESI†). The comparison exhibited that the present method has suitable LODs, enrichment factors, and sorption capacities compared to the former works. The compared features of the developed method are similar or, in some cases are, act superior to the other methods. Thus, this method is acceptable in terms of analytical efficiency.
3.8 Analytical application to the real sample
To reduce the undesirable matrix influence in the case of accurate sample analysis, the developed extraction method was applied for the extraction of target analytes at pH 6.2. Table 2 summarizes the relative recovery of these ions in various samples, which are almost quantitative in all cases.
Table 2 Determination of Cd(II), Pb(II) and Ni(II) ions in sea food and agricultural samples
Sample |
Element |
Real sample (ng g−1) |
Added (ng g−1) |
Found (ng g−1) |
Recovery (%) |
RSD (%) |
Fish 1 (Persian Gulf) |
Cd |
6.7 |
10.0 |
15.6 |
89.0 |
6.8 |
Pb |
15.1 |
10.0 |
24.7 |
96.0 |
5.0 |
Ni |
21.3 |
10.0 |
30.5 |
92.0 |
4.9 |
Fish 2 (Persian Gulf) |
Cd |
8.0 |
10.0 |
17.0 |
90.0 |
7.3 |
Pb |
29.8 |
10.0 |
38.5 |
88.0 |
6.4 |
Ni |
55.3 |
10.0 |
64.0 |
87.0 |
7.9 |
Shrimp |
Cd |
23.5 |
10.0 |
32.1 |
86.0 |
3.9 |
Pb |
25.0 |
10.0 |
36.9 |
119 |
6.5 |
Ni |
33.4 |
10.0 |
42.2 |
88.0 |
7.8 |
Canned tuna |
Cd |
5.1 |
10.0 |
14.6 |
95.0 |
6.0 |
Pb |
75.4 |
10.0 |
84.9 |
95.0 |
8.5 |
Ni |
40.1 |
10.0 |
48.5 |
84.0 |
5.2 |
Fish (Caspian Sea) |
Cd |
19.6 |
10.0 |
29.0 |
94.0 |
4.6 |
Pb |
60.5 |
10.0 |
71.6 |
111 |
5.9 |
Ni |
98.4 |
10.0 |
107 |
86.0 |
6.1 |
Fish (Siahroud River) |
Cd |
32.2 |
10.0 |
41.5 |
93.0 |
5.9 |
Pb |
100 |
10.0 |
109 |
90.0 |
9.4 |
Ni |
25.3 |
10.0 |
36.0 |
107 |
8.3 |
Broccoli |
Cd |
3.2 |
10.0 |
13.5 |
103 |
7.1 |
Pb |
8.9 |
10.0 |
17.8 |
89.0 |
7.4 |
Ni |
6.3 |
10.0 |
15.9 |
96.0 |
6.5 |
Lettuce |
Cd |
3.3 |
10.0 |
12.9 |
96.0 |
6.9 |
Pb |
12.6 |
10.0 |
23.0 |
104 |
5.0 |
Ni |
10.0 |
10.0 |
18.9 |
89.0 |
6.7 |
Mushroom |
Cd |
2.3 |
10.0 |
12.2 |
99.0 |
8.5 |
Pb |
5.5 |
10.0 |
15.6 |
101 |
4.1 |
Ni |
9.7 |
10.0 |
18.7 |
90.0 |
6.6 |
4 Conclusion
Based on the application of Fe3O4@SiO2@PPy-PANI nano sorbent in magnetic solid-phase, a reproducible, rapid, selective and straightforward extraction method for Ni(II), Pb(II), and Cd(II) ions determination was developed. Compared to the traditional adsorbents, Fe3O4@SiO2@PPy-PANI nano sorbent exhibited several advantages, such as high sorption capacities, low detection limits, and high preconcentration factors. Also, besides the nanostructure characteristics, the nano sorbent showed magnetically-assisted separation, which leads to obtaining satisfactory results employing lower amounts of the nano-sorbent and solvent, and the method is not time-consuming.
Conflicts of interest
The authors declare that they have no conflict of interest.
References
- S. Özdemir, V. O. A. Dündar and E. Kılınç, Preconcentration of metal ions using microbacteria, Microchim. Acta, 2013, 180, 719–739 CrossRef.
- M. Mehdizadeh, F. Kermanian and G. Farjah, Schwann cell injuries of radial nerve after lead (Pb) exposure in rats, Pathophysiology, 2008, 15, 13–17 CrossRef CAS.
- L. Suo, X. Dong, X. Gao, J. Xu, Z. Huang, J. Ye, X. Lu and L. Zhao, Silica-coated magnetic graphene oxide nanocomposite based magnetic solid phase extraction of trace amounts of heavy metals in water samples prior to determination by inductively coupled plasma mass spectrometry, Microchem. J., 2019, 149, 104039 CrossRef CAS.
- E. Ghorbani-Kalhor, A metal–organic framework nanocomposite made from functionalized magnetite nanoparticles and HKUST-1 (MOF-199) for preconcentration of Cd(II), Pb(II), and Ni(II), Microchim. Acta, 2016, 183(9), 2639–2647 CrossRef CAS.
- H. Bagheri, A. Afkhami, M. Saber-Tehrani and H. Khoshsafar, Preparation and characterization of magnetic nanocomposite of Schiff base/silica/magnetite as a preconcentration phase for the trace determination of heavy metal ions in water, food and biological samples using atomic absorption spectrometry, Talanta, 2012, 97, 87–95 CrossRef CAS PubMed.
- J. W. Hamilton, R. C. Kaltreider, O. V. Bajenova, M. A. Ihnat, J. McCaffrey, B. W. Turpie, E. E. Rowell, J. Oh, M. J. Nemeth, C. A. Pesce and J. P. Lariviere, Molecular basis for effects of carcinogenic heavy metals on inducible gene expression, Environ. Health Perspect., 1998, 106, 1005 CAS.
- B. L. Vallee and D. D. Ulmer, Biochemical effects of mercury, cadmium, and lead, Annu. Rev. Biochem., 1972, 41, 91–128 CrossRef CAS PubMed.
- T. Partanen, P. Heikkilä, S. Hernberg, T. Kauppinen, G. Moneta and A. Ojajärvi, Renal cell cancer and occupational exposure to chemical agents, Scand. J. Work, Environ. Health, 1991, 1, 231–239 CrossRef.
- M. H. Khah, P. Jamshidi and F. Shemirani, Applying Fe3O4-MoS2-chitosan nanocomposite to preconcentrate heavy metals from dairy products prior quantifying by FAAS, Res. Chem. Intermed., 2021, 1–15 Search PubMed.
- N. Kanani, M. Bayat, F. Shemirani, J. B. Ghasemi, Z. Bahrami and A. Badiei, Synthesis of magnetically modified mesoporous nanoparticles and their application in simultaneous determination of Pb(II), Cd(II) and Cu(II), Res. Chem. Intermed., 2018, 44(3), 1689–1709 CrossRef CAS.
- M. Ghaedi, H. R. Noormohamadi, A. Asfaram, M. Montazerozohori, J. Tashkhourian and M. Soylak, Modification of platinum nanoparticles loaded on activated carbon and activated carbon with a new chelating agent for solid phase extraction of some metal ions, J. Mol. Liq., 2016, 221, 748–754 CrossRef CAS.
- P. Bruno, M. Caselli, G. Gennaro, P. Ielpo, T. Ladisa and C. M. Placentino, Ion chromatography determination of heavy metals in airborne particulate with preconcentration and large volume direct injection, Chromatographia, 2006, 64, 537–542 CrossRef CAS.
- M. C. Yebra-Biurrun, A. Bermejo-Barrera, M. P. Bermejo-Barrera and M. C. Barciela-Alonso, Atomic absorption spectrometry determination of trace metals in natural waters by flame atomic absorption spectrometry following on-line ion-exchange preconcentration, Anal. Chim. Acta, 1995, 303, 341–345 CrossRef CAS.
- M. M. Matlock, B. S. Howerton and D. A. Atwood, Chemical precipitation of heavy metals from acid mine drainage, Water Res., 2002, 36, 4757–4764 CrossRef CAS.
- S. Abe, K. Fuji and T. Sono, Liquid-liquid extraction of manganese(II), copper(II) and zinc(II) with acyclic and macrocyclic Schiff bases containing bisphenol A subunits, Anal. Chim. Acta, 1994, 293, 325–330 CrossRef CAS.
- A. Afkhami and M. Bahram, Cloud point extraction simultaneous spectrophotometric determination of Zn(II), Co(II) and Ni(II) in water and urine samples by 1-(2-pyridylazo) 2-naphthol using partial least squares regression, Microchim. Acta, 2006, 155, 403–408 CrossRef CAS.
- A. A. Asgharinezhad, N. Jalilian, H. Ebrahimzadeh and Z. Panjali, A simple and fast method based on new magnetic ion imprinted polymer nanoparticles for the selective extraction of Ni(II) ions in different food samples, RSC Adv., 2015, 5(56), 45510–45519 RSC.
- S. H. Mousavi, M. Manoochehri and F. A. Taromi, Fabrication of a novel magnetic metal–organic framework functionalized with 2-aminothiophenol for preconcentration of trace silver amounts in water and wastewater, RSC Adv., 2021, 11(23), 13867–13875 RSC.
- A. A. Asgharinezhad, M. Rezvani, H. Ebrahimzadeh, N. Shekari, N. Ahmadinasab and M. Loni, Solid phase extraction of Pb(II) and Cd(II) ions based on murexide functionalized magnetic nanoparticles with the aid of experimental design methodology, Anal. Methods, 2015, 7, 10350–10358 RSC.
- M. Babazadeh, R. Hosseinzadeh-Khanmiri, J. Abolhasani, E. Ghorbani-Kalhor and A. Hassanpour, Solid phase extraction of heavy metal ions from agricultural samples with the aid of a novel functionalized magnetic metal–organic framework, RSC Adv., 2015, 5(26), 19884–19892 RSC.
- M. Manoochehri and L. Naghibzadeh, A nanocomposite based on Dipyridylamine functionalized magnetic Multiwalled carbon nanotubes for separation and Preconcentration of toxic elements in black tea leaves and drinking water, Food Anal. Methods, 2017, 10(6), 1777–1786 CrossRef.
- A. A. ALOthman, M. Habila, E. Yilmaz and M. Soylak, Solid phase extraction of Cd(II), Pb(II), Zn(II) and Ni(II) from food samples using multiwalled carbon nanotubes impregnated with 4-(2-thiazolylazo) resorcinol, Microchim. Acta, 2012, 177, 397–403 CrossRef.
- P. O. Patil, P. V. Bhandari, P. K. Deshmukh, S. S. Mahale, A. G. Patil, H. R. Bafna, K. V. Patel and S. B. Bari, Green fabrication of graphene-based silver nanocomposites using agro-waste for sensing of heavy metals, Res. Chem. Intermed., 2017, 43(7), 3757–3773 CrossRef CAS.
- F. Faryadras, S. M. Yousefi, P. Jamshidi and F. Shemirani, Application of magnetic graphene-based bucky gel as an efficient green sorbent for determination of mercury in fish and water samples, Res. Chem. Intermed., 2020, 46(4), 2055–2068 CrossRef CAS.
- M. Taghizadeh, A. A. Asgharinezhad, N. Samkhaniany, A. Tadjarodi, A. Abbaszadeh and M. Pooladi, Solid phase extraction of heavy metal ions based on a novel functionalized magnetic multi-walled carbon nanotube composite with the aid of experimental design methodology, Microchim. Acta, 2014, 181, 597–605 CrossRef CAS.
- M. R. Sohrabi, Z. Matbouie, A. A. Asgharinezhad and A. Dehghani, Solid phase extraction of Cd(II) and Pb(II) using a magnetic metal–organic framework, and their determination by FAAS, Microchim. Acta, 2013, 180, 589–597 CrossRef CAS.
- M. Taghizadeh, A. A. Asgharinezhad, M. Pooladi, M. Barzin, A. Abbaszadeh and A. Tadjarodi, A novel magnetic metal organic framework nanocomposite for extraction and preconcentration of heavy metal ions, and its optimization via experimental design methodology, Microchim. Acta, 2013, 180, 1073–1084 CrossRef CAS.
- J. S. Suleiman, B. Hu, H. Peng and C. Huang, Separation/preconcentration of trace amounts of Cr, Cu and Pb in environmental samples by magnetic solid-phase extraction with Bismuthiol-II immobilized magnetic nanoparticles and their determination by ICP-OES, Talanta, 2009, 77, 1579–1583 CrossRef CAS.
- S. Karami, H. Ebrahimzadeh and A. A. Asgharinezhad, A simple and fast method based on functionalized magnetic nanoparticles for the determination of Ag(I), Au(III) and Pd(II) in mine stone, road dust and water samples, Anal. Methods, 2017, 9(19), 2873–2882 RSC.
- H. Bagheri, A. A. Asgharinezhad and H. Ebrahimzadeh, Determination of trace amounts of Cd(II), Cu(II), and Ni(II) in food samples using a novel functionalized magnetic nanosorbent, Food Analytical Methods, 2016, 9(4), 876–888 CrossRef.
- M. Ghaemi and G. Absalan, Study on the adsorption of DNA on Fe3O4 nanoparticles and ionic liquid-modified Fe3O4 nanoparticles, Microchim. Acta, 2014, 181, 45–53 CrossRef CAS.
- M. Faraji, Y. Yamini and M. Rezaee, Extraction of trace amounts of mercury with sodium dodecyle sulphate-coated magnetite nanoparticles and its determination by flow injection inductively coupled plasma-optical emission spectrometry, Talanta, 2010, 81, 831–836 CrossRef CAS PubMed.
- H. Bagheri, A. A. Asgharinezhad and H. Ebrahimzadeh, Determination of trace amounts of Cd(II), Cu(II), and Ni(II) in food samples using a novel functionalized magnetic nanosorbent, Food Analytical Methods, 2016, 9, 876–888 CrossRef.
- Y. Wang, H. Chen, J. Tang, G. Ye, H. Ge and X. Hu, Preparation of magnetic metal organic frameworks adsorbent modified with mercapto groups for the extraction and analysis of lead in food samples by flame atomic absorption spectrometry, Food Chem., 2015, 181, 191–197 CrossRef CAS.
- M. Rezvani, A. A. Asgharinezhad, H. Ebrahimzadeh and N. Shekari, A polyaniline-magnetite nanocomposite as an anion exchange sorbent for solid-phase extraction of chromium(VI) ions, Microchim. Acta, 2014, 181, 1887–1895 CrossRef CAS.
- M. Helmi, R. Farimani, N. Shahtahmassebi, M. R. Roknabadi and N. Ghows, Synthesis and study of structural and magnetic properties of super paramagnetic Fe3O4@SiO2 core/shell nanocomposite for biomedical applications, Nanomed. J., 2014, 1, 71–78 Search PubMed.
- A. A. Asgharinezhad, H. Ebrahimzadeh, M. Rezvani, N. Shekari and M. Loni, A novel 4-(2-pyridylazo) resorcinol functionalized magnetic nanosorbent for selective extraction of Cu(II) and Pb(II) ions from food and water samples, Food Addit. Contam., Part A, 2014, 31, 1196–1204 CAS.
- A. B. Yilmaz, Levels of heavy metals (Fe, Cu, Ni, Cr, Pb, and Zn) in tissue of Mugil cephalus and Trachurus mediterraneus from Iskenderun Bay, Turkey, Environ. Res., 2003, 92, 277–281 CrossRef CAS.
- S. Sadeghi and E. Aboobakri, Magnetic nanoparticles with an imprinted polymer coating for the selective extraction of uranyl ions, Microchim. Acta, 2012, 178, 89–97 CrossRef CAS.
- P. Xu, X. Han, C. Wang, B. Zhang, X. Wang and H.-L. Wang, Facile Synthesis of Polyaniline-Polypyrrole Nanofibers for Application in Chemical Deposition of Metal Nanoparticles, Macromol. Rapid Commun., 2008, 29, 1392–1397 CrossRef CAS.
- E. Roy, S. Patra, R. Madhuri and P. K. Sharma, Simultaneous determination of heavy metals in biological samples by a multiple-template imprinting technique: an electrochemical study, RSC Adv., 2014, 4(100), 56690–56700 RSC.
- A. A. Asgharinezhad, S. Karami, H. Ebrahimzadeh, N. Shekari and N. Jalilian, Polypyrrole/magnetic nanoparticles composite as an efficient sorbent for dispersive micro-solid-phase extraction of antidepressant drugs from biological fluids, Int. J. Pharm., 2015, 494(1), 102–112 CrossRef CAS PubMed.
- A. A. Asgharinezhad and H. Ebrahimzadeh, A simple and fast method based on mixed hemimicelles coated magnetite nanoparticles for simultaneous extraction of acidic and basic pollutants, Anal. Bioanal. Chem., 2016, 408, 473–486 CrossRef CAS.
|
This journal is © The Royal Society of Chemistry 2022 |
Click here to see how this site uses Cookies. View our privacy policy here.