DOI:
10.1039/D2RA02962F
(Paper)
RSC Adv., 2022,
12, 20876-20885
Spatial and temporal variability of contaminants of emerging concern in a drinking water source†
Received
10th May 2022
, Accepted 12th July 2022
First published on 21st July 2022
Abstract
The spatial–temporal behaviour of contaminants of emerging concern (CECs) are not well-documented in drinking water sources, including in Quebec, Canada. In this study, a set of seven contaminants, which are more frequently reported in water sources, were monitored from May 2016 to August 2017 at ten locations within an urbanized watershed (lakes and rivers) in Quebec, Canada. Samples were collected between a wastewater plant discharge (upstream) and the intake of a drinking water plant (downstream). The results showed that three (acetaminophen, salicylic acid, caffeine) out of seven CECs were consistently detected at a range of up to a few hundred ng L−1 at all sampling stations throughout the sampling period with a detection frequency between 51% and 94%. Upstream of two wastewater plant discharge locations, six CECs were measured above the detection limit compared to other locations where only three CECs (acetaminophen, salicylic acid, caffeine) were detected. Most of the CEC concentrations were higher (a few ng L−1) during late winter in comparison with the summer, in both years 2016 and 2017. The results highlight that the wastewater effluents and septic system effluents are significant sources of CECs that are released into the surface water. Moreover, the results help to identify the spatio-temporal patterns, which is a crucial element to understand the fate of CECs in water sources submitted to extreme weather conditions during the year. This research also provides baseline data for CEC occurrence at different points across lakes, rivers, and tributaries which will be useful for future ecotoxicological studies.
1 Introduction
Various studies have investigated the presence of contaminants of emerging concern (CECs) from sources such as pharmaceuticals, personal care products, hormones and other chemicals in wastewater effluents worldwide.1–3 The occurrence of CECs at trace levels in natural water sources is mainly due to the discharge of wastewater effluent and septic run-off into surface water (rivers, lakes, and marine waters).4,5 The CEC load in surface waters depends on the flow rate of the wastewater effluents, surface runoff, and tributaries, as well as the contribution of CECs from septic field runoff, and urbanization.6–8 Further, the occurrence and fate of contaminants in surface water depending on the various characteristics of the receiving water body, including flow rate, temperature, flow from surrounding tributaries, and the physicochemical and biological composition of the surface water.9,10 In addition, partitioning, biotic mechanisms (uptake by biota and biodegradation), and abiotic processes (photodegradation, volatilization, and hydrolysis) also influence the fate of contaminants.3,8 Moreover, the release of CECs via wastewater effluents is also influenced by dilution factors from receiving water bodies, which may affect contaminant occurrence and detection levels.7,11
CECs are constantly being added to aquatic ecosystems at trace levels (e.g. ng L−1). The degradation rates of these contaminants often compare to their addition rates, making CECs pseudo-persistent, resulting in continuous exposure for humans and aquatic life. The quantitative assessment of CECs is a key aspect of obtaining data on their widespread distribution and mobility in water bodies. This data provide the information that is required for the risk assessment of long-term exposure to CECs at low concentrations.12 Worldwide receiving bodies have been evaluated nationwide to monitor and collect data on CEC levels. For example, Focazio et al. 2008 assessed 100 CECs, of which 63 were detected, including acetaminophen, ibuprofen, carbamazepine, and sulfamethoxazole in 49 surface and drinking water samples across several states in the U.S.13 In 2017, a nationwide study in the U.S. reported that out of 247 examined contaminants, 148 were detected in surface water, including caffeine, carbamazepine, and sulfamethoxazole. In Spain, Valcárcel et al. (2011) investigated the presence of 33 pharmaceuticals in rivers and tap water of the Madrid region, and among the antibiotics, sulfamethoxazole was detected with the second-highest concentration (median: 326 ng L−1) compared to trimethoprim antibiotic (424 ng L−1).14 In Italian surface waters, 160 CECs have been identified over 15 years of continuous monitoring.15 In a nationwide survey of Brazil, caffeine was frequently detected in both drinking and surface waters.16 All these comprehensive national studies emphasize the persistence of various CECs at different trace levels in surface waters.
CEC concentrations were reported to decay from the point source of contamination, i.e. wastewater effluent discharges. This is due to various factors such as dilution, degradation, and partitioning, which also play a pivotal role in the spatial and temporal distribution of CECs in freshwater sources. Contaminant studies typically monitor a range of CECs in various surface water sources or drinking water treatment plants. However, there are limited data on the spatial and temporal distribution of CECs in drinking water sources at the watershed level.16,17 More importantly, data on the levels of CECs from the discharge of wastewater effluent until the intake of the drinking water plant are limited. Hence, continuous and multiple-site monitoring of CECs is needed to identify the origins of contamination to control the pollution at the source.18 In addition, the literature is limited about the transport of CECs under extreme weather conditions during the year, in particular in surface waters.19 In fact, temperature is an important parameter that affects the CEC's degradation20 and the microbial activity of surface waters. Hence, the current study will provide new data on the levels of CECs and their transport/movement from the wastewater effluent discharge station to the drinking water intake station. Moreover, this study covers a high diversity of sampling locations within the watershed, representing mainly wastewater effluent discharges, septic tank discharges, and the impact of tributaries. The results of this study will help to identify the crucial point sources that are contributing to CECs contamination within the watershed.
The current research is based on a multiple-site sampling program that was conducted for over a year. This study monitors seven CECs (acetaminophen (Acph), salicylic acid (SA), caffeine (Caf), ibuprofen (IBP), carbamazepine (CBZ), sulfamethoxazole (SMX), and drospirenone (DPN)) occurrence and demonstrates the importance of seasonal effects in accurately detecting the presence of these CEC in surface water. The objective of this study was to: (I) monitor the selected CECs in a watershed (lake and river), from the upstream (wastewater effluent discharge) to the intake of the drinking water treatment plant; (II) conduct sampling campaigns from the different sampling stations which are connected to the watershed over a year; (III) conduct spatial and temporal data analysis to understand the fate of CECs along the watershed. CECs were selected in this study based on the frequent detection of these contaminants at trace levels in the surface waters and their extreme persistence.
2 Methods
2.1. Study and sampling stations
Lake St-Charles (46° 55′ N, 71° 22′ W) is the primary drinking water reservoir for Québec City, Canada. The lake primarily flows out into the St-Charles River (46° 56′ 28′′ N, 71° 23′ 14′′ W) via a dam that separates the lake and the river. In addition to 34 small tributaries, the lake is fed by two main water sources: Delage Lake and Huron River.21 Delage Lake, Lake St-Charles, and Huron River receive wastewater effluents from wastewater treatment plants and septic installations/systems. In addition to Lake St-Charles discharge (42%), St-Charles River is also fed by Jaune River and Nelson River, which contribute approximately 23% and 35% of the total river water, respectively.22 The St-Charles River runs north about 33 km through Québec City and ends in the St-Lawrence River, which is among the world's largest river systems.
A total of 28 sampling campaigns were conducted in ten locations within the watershed, between the municipal wastewater discharge points and drinking water intake (Fig. 1). Sampling occurred twice each month between May 2016 and August 2017 (November and December of 2016 samples were not collected due to challenging weather conditions). The collected samples were integrated into the regular water quality monitoring program for the rivers and lakes of the St-Charles watershed. Due to the challenging conditions during winter, sampling is generally not conducted from November through April. Selection of sampling sites around the St-Charles Lake and along the St-Charles River focused on the points of discharge for the wastewater effluents from the two surrounding municipal treatment plants, and water bodies affected by domestic and industrial septic installations, public/private playgrounds, agricultural activities, and other tributaries. The sampling stations represent areas of potential pollutant discharge within the watershed.
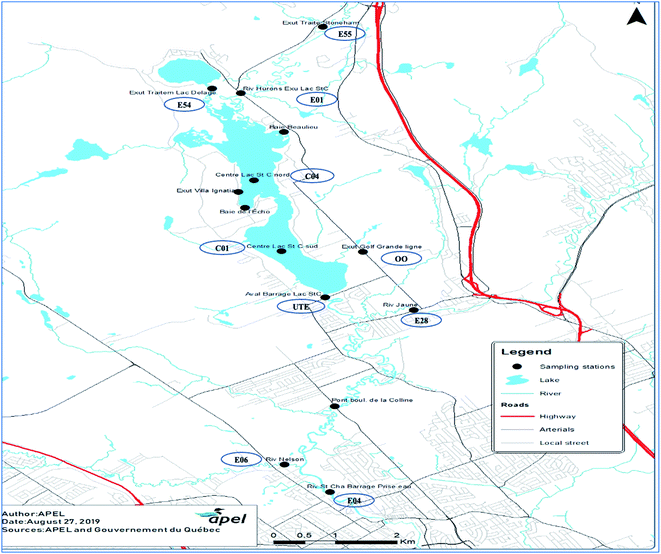 |
| Fig. 1 Map of study area and sampling sites across St-Charles lake and river. | |
This study is part of a long-term monitoring program that has been in progress for 13 years and includes many additional sampling stations (326 total) covering the St-Charles watershed. This program continues to evolve as new sampling sites are added to determine the potential point sources of wastewater discharges into river and lake sources. The sampling station names were assigned to be consistent with their names within the larger program and do not reflect the order of sample collection. Sampling station, E55, is located at the discharge of the wastewater effluent from the Stoneham municipality before the water is discharged into the Huron River. This site can sometimes be submerged into the Huron River. This can result in a slight dilution of the samples. Sampling station, E54, is located at the wastewater discharge of the wastewater treatment plant in the City of Lac Delage. There is no possibility of dilution associated with this station (E54). This effluent discharges into the northern marshes in the northern basin of Lake St-Charles. Samples from station E01, are representative of the water quality of the entire Huron River, a water body that receives the effluent from E55 and diffuses charges of nutrients from septic installations.18 Station C04 represents the Baie d'Écho section of the northern basin of Lake St-Charles. This bay is suspected to be heavily impacted by septic systems from private households and treatment centers for substance abuse. C01 station is situated in the southern basin of Lake St-Charles, at the outflow of the lake. Station E04 is located on the St-Charles River, and water collected from this site is representative of the water quality of the river downstream of St-Charles Lake. Station, OO, is located at the Lake St-Charles watershed that drains a golf course, a busy road, and several houses that are connected to a municipal sewer system. The tributary discharges into the southern basin of Lake St-Charles. However, the golf course has a septic system to handle wastewater, and the water at site OO is likely affected by this, especially during the summer and fall. Station E28, represents the water quality of Jaune River before it discharges into St-Charles River (downstream of Lake St-Charles). Samples from site E06 are representative of the water quality of the Nelson River (downstream from Lake St-Charles and Jaune River), and finally, site UTE samples are representative of the water quality of the St-Charles River just before the raw water intake at the drinking water treatment plant (Fig. 1). As mentioned, site names were established as part of a larger, ongoing study. Using consistent site names enables our results to be used for investigations of correlation in future research. All methods and materials used for sampling, except for those related to CECs, can be found in the 2017 AGIRO document.23
The study focuses on all the possible tributaries or rivers that are connected to the main drinking water source of Quebec City. This was the first study reported in the literature that investigated the occurrence and fate of different CECs in the St-Charles lake and river water from the upstream north to the intake of the drinking water treatment plant. The study was conducted over 14 months, but the sampling campaigns were interrupted during the period of extreme cold in winter. In this study, the dilution factor of river/lake was not considered while analyzing the temporal effect on these CECs.
2.2. Materials
Analytical standards of the selected CECs and internal standards were supplied from Sigma-Aldrich (Spruce St., St. Louis, MO, USA) and had >99% purity. Acetonitrile, methanol, acetic acid, formic acid, ascorbic acid, and isopropanol were purchased from Fisher Scientific (Ontario, Canada).
Sample preparation, extraction, and CEC analyses were carried out according to Pulicharla et al.24 Briefly, solid-phase extraction (SPE) cartridges (SiliCycle, Quebec) were used to extract the selected CECs. All extractions were stored in the dark at 4 °C until further analysis. The analyses were performed using an ultra-pressure liquid chromatography (UPLC) instrument (Waters Acquity I-Class (Milford, USA)) with a source mounted on a Xevo TQ-S Mass Spectrometer (Waters, Milford, USA). Chromatographic separations of selected CECs were performed with an Agilent Zorbax SB-C18 Rapid Resolution reverse-phase column (2.1 × 50 mm, 1.8 μm (Santa Clara, USA)). Three different gradient elution methods were developed for the same column and solvent composition to quantify the specific CEC being examined. The mass spectrometer was operated in Multiple Reaction Mode (MRM) to obtain analyte confirmation and quantification points.
3 Results and discussion
3.1. Occurrence of selected CECs
Overall, the detection frequencies of five contaminants, Acph, SA, Caf, IBP, and CBZ, were between 55% and 94% (Table 1). Detection frequencies of SMX were between 44% and 82% and DPN was between 22% and 47% at all sampling locations. The concentration of DPN was below the limit of quantification (LOQ) at all sampling stations throughout the sampling period. The detection frequencies of measured contaminants were consistent with other studies, including a two-year study of surface water in Denver, Colorado, where Caf and CBZ frequencies were between 57.5% and 77.8% and SBZ was detected slightly more often with a detection frequency of around 87.0%.6 However, the median and maximum concentrations of Caf, CBZ, and SMZ were higher (38.0–77.0 ng L−1) in Denver surface water compared to the values detected in our study (<LOQ-33.0 ng L−1).6 Similarly, a study by Fairbairn et al., 2016 reported >50% detection frequency of Acph, Caf, SMX, and CBZ. Still, at the wastewater effluent discharge point in Zumbro River, USA, the concentrations were similar for Acph (≈10.0 ng L−1) and higher for other contaminants.25
Table 1 The summary results of selected contaminants of emerging interest statistical analysis
Surface water sampling station |
Contaminants |
Frequency of detection (%) |
Frequency of quantification (%) |
Range (ng L−1) |
Median (ng L−1) |
Mean (ng L−1) |
The reported quantification frequency was calculated using the values above LOD, including the values between LOD and LOQ. LOD: limit of detection; LOQ: limit of quantification. |
Exit of Stoneham sewage effluent (E55) |
Acetaminophen |
66.67 |
44.40 |
2.7–400.0 |
10.1 |
50.5 |
Salicylic acid |
55.56 |
55.56 |
9.2–400.0 |
19.5 |
48.8 |
Caffeine |
51.85 |
51.85 |
12.6–110 000.0 |
170.0 |
13 682.0 |
Ibuprofen |
55.56 |
44.44 |
5.0–370.0 |
88.0 |
96.3 |
Carbamazepine |
55.56 |
55.56 |
44.0–190.0 |
61.0 |
86.3 |
Sulfamethoxazole |
44.44 |
44.44 |
14.0–93.0 |
51.0 |
49.6 |
Drospirenone |
22.22 |
0.00 |
<LOQ |
<LOQ |
<LOQ |
Hurons River discharges into St-Charles Lake (E01) |
Acetaminophen |
66.67 |
48.15 |
2.5–93.0 |
8.85 |
17.6 |
Salicylic acid |
59.26 |
59.26 |
9.2–670.0 |
22.2 |
74.0 |
Caffeine |
55.56 |
48.15 |
2.3–96.0 |
8.2 |
15.0 |
Ibuprofen |
59.26 |
3.70 |
5.0–11.9 |
<LOQ |
<LOQ |
Carbamazepine |
59.26a |
29.63a |
<LOQ |
<LOQ |
<LOQ |
Sulfamethoxazole |
48.15a |
3.70a |
<LOQ |
<LOQ |
<LOQ |
Drospirenone |
25.93a |
3.70a |
<LOQ |
<LOQ |
<LOQ |
Wastewater treatment plant effluent of the City of Lac Delage (E54) |
Acetaminophen |
85.19 |
74.07 |
5–33 000.0 |
15.8 |
2854.6 |
Salicylic acid |
74.07 |
74.07 |
9.2–490.0 |
19.5 |
48.8 |
Caffeine |
70.37 |
66.67 |
2.3–44 000.0 |
33.0 |
8272.7 |
Ibuprofen |
74.07 |
55.56 |
5.0–210.0 |
25.5 |
39.1 |
Carbamazepine |
74.07 |
74.07 |
58.0–450.0 |
230.0 |
240.0 |
Sulfamethoxazole |
66.67 |
51.85 |
1.2–210.0 |
<LOQ |
19.9 |
Drospirenone |
22.22 |
0.00 |
<LOQ |
<LOQ |
<LOQ |
Northern basin of St-Charles Lake (C04) |
Acetaminophen |
88.24 |
52.94 |
1.9–100.0 |
6.80 |
16.3 |
Salicylic acid |
88.24 |
88.24 |
9.2–980.0 |
32 |
119.9 |
Caffeine |
70.59 |
70.59 |
7.5–620.0 |
12.65 |
113.1 |
Ibuprofen |
82.35 |
17.64 |
5.0–40.0 |
<LOQ |
<LOQ |
Carbamazepine |
88.24 |
41.18 |
1.8–7.2 |
<LOQ |
<LOQ |
Sulfamethoxazole |
52.94a |
23.53a |
<LOQ |
<LOQ |
<LOQ |
Drospirenone |
47.06a |
5.89a |
<LOQ |
<LOQ |
<LOQ |
Southern basin of St-Charles Lake (CO1(E)) |
Acetaminophen |
94.11 |
52.94 |
4.0–34.0 |
6.3 |
10.1 |
Salicylic acid |
94.11 |
94.11 |
9.2–1000.0 |
26.5 |
128.6 |
Caffeine |
76.47 |
76.47 |
6.2–550.0 |
14.2 |
100.7 |
Ibuprofen |
64.07a |
11.76a |
<LOQ |
<LOQ |
<LOQ |
Carbamazepine |
58.82 |
35.29 |
1.8–6.2 |
<LOQ |
<LOQ |
Sulfamethoxazole |
82.35 |
35.29 |
1.2–4.6 |
<LOQ |
<LOQ |
Drospirenone |
11.76 |
0.00 |
<LOQ |
<LOQ |
<LOQ |
Exit of golf ground (OO) |
Acetaminophen |
62.96 |
44.4 |
3.1–1800.0 |
21.9 |
163.2 |
Salicylic acid |
55.56 |
55.56 |
9.2–290.0 |
35.0 |
60.5 |
Caffeine |
51.85 |
48.15 |
2.3–3200.0 |
22.1 |
265.8 |
Ibuprofen |
55.56 |
0.00 |
<LOQ |
<LOQ |
<LOQ |
Carbamazepine |
55.56 |
0.00 |
<LOQ |
<LOQ |
<LOQ |
Sulfamethoxazole |
44.44 |
3.70 |
<LOQ |
<LOQ |
<LOQ |
Drospirenone |
25.93 |
3.70 |
<LOQ |
<LOQ |
<LOQ |
Start of St-Charles River (E04) |
Acetaminophen |
85.19 |
51.85 |
1.6–62.0 |
7.4 |
39.4 |
Salicylic acid |
81.48 |
81.48 |
9.2–4000.0 |
23.8 |
224.3 |
Caffeine |
77.78 |
74.07 |
2.3–370.0 |
18.9 |
68.6 |
Ibuprofen |
81.48 |
7.41 |
5.0–27.0 |
<LOQ |
<LOQ |
Carbamazepine |
81.48a |
33.33a |
<LOQ |
<LOD |
<LOQ |
Sulfamethoxazole |
70.37a |
11.11a |
<LOQ |
<LOQ |
<LOQ |
Drospirenone |
29.63 |
3.70a |
<LOQ |
<LOQ |
<LOQ |
Jaune River (E28) |
Acetaminophen |
81.48 |
59.25 |
2.3–52.0 |
7.7 |
12.9 |
Salicylic acid |
74.04 |
74.04 |
9.2–350.0 |
16.8 |
45.1 |
Caffeine |
70.37 |
59.26 |
2.3–140.0 |
8.7 |
17.3 |
Ibuprofen |
77.78 |
11.11 |
5.0–34.0 |
<LOQ |
<LOQ |
Carbamazepine |
74.07 |
55.56 |
1.8–7.5 |
<LOQ |
<LOQ |
Sulfamethoxazole |
66.67 |
14.81 |
1.2–380.0 |
<LOQ |
24.2 |
Drospirenone |
25.96a |
7.41a |
<LOQ |
<LOQ |
<LOQ |
Nelson River (E06) |
Acetaminophen |
62.96 |
51.85 |
4.0–31.0 |
10.0 |
32.1 |
Salicylic acid |
55.56 |
55.56 |
9.2–2400.0 |
33.0 |
51.3 |
Caffeine |
51.85 |
48.15 |
2.3–49.0 |
11.6 |
16.2 |
Ibuprofen |
55.56 |
7.41 |
5.0–18.6 |
<LOQ |
<LOQ |
Carbamazepine |
55.56 |
0.00 |
<LOQ |
<LOQ |
<LOQ |
Sulfamethoxazole |
48.15a |
7.41a |
<LOQ |
<LOQ |
<LOQ |
Drospirenone |
25.93a |
3.70a |
<LOQ |
<LOQ |
<LOQ |
Exit of St-Charles Lake (UTE) |
Acetaminophen |
62.96 |
44.40 |
2.9–130.0 |
9.2 |
16.8 |
Salicylic acid |
55.56 |
55.56 |
9.2–190.0 |
21.6 |
35.9 |
Caffeine |
51.85 |
48.15 |
2.3–140.0 |
18.9 |
31.9 |
Ibuprofen |
55.56a |
3.70a |
<LOQ |
<LOQ |
<LOQ |
Carbamazepine |
55.56a |
22.22a |
<LOQ |
<LOQ |
<LOQ |
Sulfamethoxazole |
44.44a |
7.41a |
<LOQ |
<LOQ |
<LOQ |
Drospirenone |
25.93a |
3.70a |
<LOQ |
<LOQ |
<LOQ |
3.1.1 Stoneham sewage effluent (E55). Stoneham municipal plant uses alum-based coagulation treatment21 before discharges into Huron River water. Samples collected from this sewage effluent, where the water might mix with Huron River water indicated much higher median concentrations (Table 1), at about 10.5–170.0 ng L−1 for selected CECs, compared to other sampling stations. The mean (<LOQ-240.0 ng L−1) and the maximum range of concentrations (190.0–2400.0 ng L−1) of selected contaminants including SA, IBP, and CBZ were lower compared to 14 Canadian sewage plants (mean: 0.7–4.0 μg L−1).26 Conversely, levels of Acph, IBP, CBZ, SMX, and Caf were found to be higher than at wastewater effluents in Korea (range: 308–840 ng L−1) and Saudi Arabia (mean < 31.2 ng L−1).27,28 The relatively high concentrations indicate the poor removal efficiency of sewage treatment processes at the Stoneham municipal plant. Research has shown that the sedimentation process in sewage treatment is less efficient in removing trace levels of CECs than secondary and tertiary treatments.29
3.1.2 Huron River discharges into St-Charles Lake (E01). At site E01 on the Huron River, only four CECs were detected (values above LOQ): Acph, SA, Caf, and IBP. The median values (8.2–22.2 ng L−1) and the maximum range (11.9–670.0 ng L−1) of these CEC concentrations were lower in comparison to E55, except for SA. The relatively low concentration of contaminants is due to dilution caused by the river water, rainwater, and other tributaries that connect to the Huron River. The levels of the aforementioned CECs (Acph, SA, Caf, and IBP) in the Huron River are higher compared to the Yamaska River in Canada (1.0–34.7 ng L−1) and the Mississippi River in the USA (0–65.2 ng L−1).30,31 However, SMZ and CBZ were detected in higher concentrations in the Yamaska and Mississippi rivers, while in the St-Charles watershed at station E01, these compounds were not detected. At E01, higher median (22.2 ng L−1) and maximum (670.0 ng L−1) concentrations of SA were observed compared to station E55 median (19.5 ng L−1) and maximum (400.0 ng L−1) concentrations. The relatively high SA concentration in rivers is likely due to natural sources (plants), in addition to human activities.32
3.1.3 Wastewater treatment plant effluent in the City of Lac Delage (E54). The second highest source of CECs was found at the discharge point of the City of Lac Delage WWTP effluent, which feeds into the St-Charles Lake. This is because the municipality of Lac Delage discharges its wastewater effluent into the Delage Lake after minimal treatment, such as aerated lagoons (alum-based coagulation). Higher median (15.8 ng L−1 and 230 ng L−1) and mean (2854.6 ng L−1 and 240 ng L−1) concentrations of Acph and CBZ were observed at station E54 compared to the Stoneham municipality effluent, E55 (median: 10.1 ng L−1 and 61 ng L−1; mean: 50.5 ng L−1 and 86.3 ng L−1). The detected levels of CECs at station E54 were like those of other North American surface waters, such as the Yamaska River, Canada, and the Mississippi River, U.S.30,31
3.1.4 Across St-Charles Lake: northern basin (C04) and southern basin and (C01). Three out of seven contaminants were consistently detected above the limit of quantification (LOQ) at the northern basin and southern basin (the outflow) of Lake St-Charles (C04 and C01). Those contaminants and their median values are Acph (6.3–6.8 ng L−1), SA (26.5–32 ng L−1), and Caf (12.65–14.2 ng L−1). IBP and CBZ were detected in the northern basin of St-Charles Lake (C04) and CBZ and SMX were detected in the southern basin of St-Charles Lake (C01). Few significant differences in the concentrations of each contaminant were observed across the lake, consistent with a previous study of Yamaska River.31
3.1.5 Runoff from the golf course (OO). A golf course that treats wastewater using a septic system is located on the west side of Lake St-Charles and a tributary connected to this area discharges into the southern basin (the outflow) of Lake St-Charles (Fig. 1). The median concentrations of Acph (21.9 ng L−1), SA (35 ng L−1), and Caf (22.1 ng L−1) in the water samples collected at this site are relatively higher compared to the Lake St-Charles samples (Table 1). The median levels of the other contaminants were below the LOQ. The median concentrations of Acph and SA were higher than the discharge from the Stoneham WWTP (E55) and the Lake Delage WWTP effluent (E54). The treatment associated with septic systems involves conventional processes (sedimentation, natural filtration) that are not efficient at removing contaminants that remain at nano- and micro-concentrations, such as pharmaceuticals, personal care products, and pesticides.30 However, the reported concentrations of Acph, Caf, and SA were lower compared to other studies of these CEC concentrations (μg L−1) at septic effluents. This is likely because the septic effluent in our study mixed with surface water, and this sampling station (OO) was only influenced by one septic installation. The other studies investigated water affected by multiple septic installations.33,34
3.1.6 Along the St-Charles River (E04, E28, E06, UTE). Similar to Lake St-Charles, three to four selected contaminants were detected at levels above the quantification limits at sites along St-Charles, Jaune, and Nelson rivers. Water collected at station E04, located on the St-Charles River, is representative of the water quality of the river downstream of the Lake St-Charles outflow. At this station, the detected median values of the three to four CECs were similar to those found in lake samples. However, the ranges of concentration were higher compared to the lake. This might be related to the presence of septic installations near the exit of Lake St-Charles, including as part of the golf course (OO) (Fig. 1). The small tributaries connected to these septic installations might run into the St-Charles River, resulting in increased detection levels of CECs at the outflow of the lake. Many studies have proven that septic installations do not efficiently treat trace contaminants.35 In the case of SA, a continuous increase was observed from far upstream to the exit point of the lake. The Jaune and Nelson rivers run into the St-Charles River approximately 1.0 km and 10.0 km upstream of the St-Charles River, respectively. Nelson River (E06) had a slightly higher median concentration of contaminants, including Acph (10.0 ng L−1) and Caf (11.6 ng L−1), and twice the concentration of SA (33.0 ng L−1) compared to Jaune River (E28) (Acph: 7.7 ng L−1, SA: 16.8 ng L−1 and Caf: 8.7 ng L−1). Concentrations of CECs in both the Jaune and Nelson rivers are influenced by the few septic systems/installations that are still operating and are connected to both watersheds. The existence of septic installations close to Nelson River discharge into St-Charles River may explain the higher concentrations in Nelson River. Moreover, the flow of this river is slightly less than that of the Jaune River. Compared to site E04 at the start of St-Charles River, where values were 7.4 ng L−1 for Acph and 18.9 ng L−1 for Caf, the measured median concentrations of contaminants in both rivers were similar for Acph (7.7–10.0 ng L−1) and lower for Caf (8.7–11.6 ng L−1).Further, the samples collected from St-Charles River at the intake of the drinking water treatment plant have shown similar concentrations compared to the exit of Lake St-Charles (UTE). Even though the CECs are subject to dilution from the river water and other tributaries affected by surface runoff and rainwater, similar detection levels were observed upstream and downstream of the river sample stations, suggesting the persistence of CECs across the river water. While biological, chemical, or natural (sunlight) degradation/transformation of CECs may reduce CEC concentrations in river water, other water sources (surface runoff or other tributaries) that feed into the St-Charles River may increase CEC concentrations.
3.2. Temporal and spatial variations of targeted contaminants
3.2.1 Spatial variation. Only three contaminants including Acph, SA, and Caf have shown spatial variation across Lake St-Charles and St-Charles River (Fig. 2). And, no significant spatial variation was observed for the remaining four CECs (IBP, SMX, CBZ, and DPN). IBP, SMX, and CBZ were detected above quantification limits only at the Stoneham municipal effluent (E55) and the WWTP effluent of the City of Lac Delage (E54).
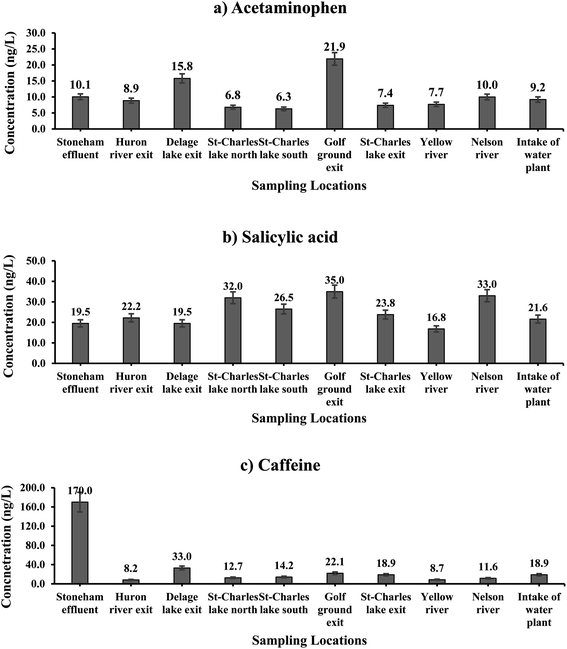 |
| Fig. 2 Spatial distribution of three major selected CEI across of SW; (a) acetaminophen; (b) salicylic acid; (c) caffeine. | |
3.2.1.1 Acetaminophen. Median concentrations of Acph varied from 6.0 ng L−1 to 10.0 ng L−1 across Lake St-Chares and St-Charles River during the 14 month sampling period. Our results showed that the highest loads of Acph that entered Lake St-Charles were mainly from the water discharge from the golf course (OO; 21.9 ng L−1), followed by the WWTP effluent of the City of Lac Delage (E54; 15.8 ng L−1), the Stoneham municipal sewage effluent (E55; 10.1 ng L−1) and the Nelson River (E06; 10.0 ng L−1). Depending on the location of entry of these sources into the lake and river, fluctuations in the concentration of contaminants were notable, as shown in Fig. 2a. Yet, while the population of Stoneham and the volume of treated wastewater in the municipality were relatively high, the median concentration of Acph was lower compared to septic tank effluents. During the 14 month sampling period, the standard deviation of Acph was between 1–12% at all sampling stations, except E54 (871%) and OO (40%) due to the outlier values. Many studies have shown that WWTPs can remove Acph by >90%.36,37 However, the conventional methods such as sedimentation and filtration, which are the main treatment processes used in septic systems and wastewater treatment plants in the City of Lac Delage, are unable to reliably remove trace amounts of contaminants.38
3.2.1.2 Salicylic acid. The highest median concentration of SA was measured at the exit of the golf course (OO) (35.0 ng L−1), followed by the Nelson River site (E06) (33.0 ng L−1) and the northern basin of Lake St-Charles (C04) (32.0 ng L−1), as shown in Fig. 2b. At the remaining sampling stations, median SA concentrations were 22.0 ng L−1 ± 3, on average. SA comes from multiple sources, including as a natural plant substance (phytohormone), a metabolite of acetylsalicylic acid, and food preservatives.39 The similar concentrations of SA at the exit of the Stoneham municipal sewage effluent (E55) and most of the lake and river samples can be explained by the different sources of SA. The high SA concentrations observed at the exit of the golf course (OO) could be from a combination of human activity and plant sources. In contrast, in the Nelson River and the northern basin of Lake St-Charles, plants are thought to be the main source of SA, along with the residual concentrations from wastewater effluents. In addition, the distribution of data at each station had standard deviations between 4–15% which suggests a continuous occurrence of SA across the watershed.
3.2.1.3 Caffeine. The trend in Caf concentrations along the lake and river was similar to that of Acph. The highest concentration of Caf was measured at the exit of the Stoneham sewage effluent (E55) (170.0 ng L−1), followed by the City of Lac Delage WWTP effluent (E54) (33.0 ng L−1), and at the golf course (OO) (22.0 ng L−1). Wide variations in Caf levels were observed across the lake and river samples, as shown in Fig. 2c. The high concentration of Caf in the area where the Stoneham sewage effluent mixes with the Huron River (E55) supports the use of Caf as a chemical marker for surface water pollution from domestic wastewater or wastewater effluents.40 Moreover, at each station, standard deviation values for Caf were within the acceptable range (below 15%).The concentration of the remaining contaminants (IBP, SMX, and CBZ) was below the quantification limit at all sampling stations except at the exit of the Stoneham sewage effluent (E55) and the City of Lac Delage WWTP effluent (E54). Compared to station E54, with 25.5 ng L−1 of IBP and 4.4 ng L−1 of SMX, station E55 had the highest measured concentrations of IBP and SMX, at 88.0 ng L−1 and 51.0 ng L−1, respectively. For CBZ, station E54 samples had higher levels at 230.0 ng L−1, compared to E55 municipal sewage effluent samples, at 61 ng L−1. The differences in CEC concentrations between the Stoneham municipality and Lac Delage municipality effluents may be associated with the differences in population and wastewater treatment processes. For instance, the Stoneham wastewater treatment plant uses alum-based coagulation followed by a tertiary treatment to treat sewage, whereas the Lac Delage municipality uses only the alum-based coagulation/precipitation. Regardless of the type of treatment, the wastewater treatment processes at both plants might be responsible for the high concentrations of CECs in Huron River, Lake St-Charles, and St-Charles River. Further, the acceptable range of standard deviation of CECs at all sampling stations indicates that the measurement of CECs is accurate and they are present throughout the year in the drinking water source.
3.2.2 Temporal variation. In general, at sampling stations, the selected contaminants except DPN were detected in high concentrations between May–July 2016 and February–July 2017 (ESI, Fig. 1†). To be specific, Acph, Caf, IBP, and SMX were detected in 2017 at higher levels and more frequently compared to 2016. And SA was observed at higher levels in 2016 than in 2017. Most of the contaminant concentrations were higher from the late winter to the summer season, in both years 2016 and 2017, because there may be a high consumption pattern of the compounds during the summer season. In addition to the high consumption, the occurrence of the selected CECs highly depends on the physico-chemical properties of the contaminants (ESI, Table 1†). Acph which is highly soluble in water has reported a degradation of around 60% by sunlight and a dominant contribution of degradation by microbes of around 80% in surface waters.41,42 Similar to Acph, Caf is also highly soluble in water and is persistent to photolysis but highly susceptible to microbial degradation.43,44 Hence, both Acph and Caf are present as they undergo higher microbial degradation compared to photolysis during summer and lower degradation due to less sunlight and less microbial activity during winter. On the other hand, SA which is soluble in water was efficiently degraded by both sunlight and microbes.45,46 The higher levels of SA detection throughout the year could be associated to the origins of this contaminant: both natural (plant substance as a metabolite of acetylsalicylic acid) and anthropogenic. Finally, IBP, SMX, and CBZ are not susceptible to both sunlight and microbial degradation which makes them persistent in surface waters.47 However, the concentrations of these contaminants are low in surface waters due to their slight solubility and high adsorption to organic matter. Continuous variations in the volume, flow rates, tributary volumes entering the lake and river systems during these seasons, and the differences in the removal by natural degradation of the CEC might be the reasons for not observing considerable trends in temporal variations of CECs during autumn, winter, or spring seasons.24 In addition, the partitioning/adsorption of the CECs between the water phase and sediment significantly affects the spatio-temporal variations.48
4 Conclusions
Seven CECs that cover a broad range of physico-chemical properties were monitored in surface and drinking water sources (lakes and rivers). The focus was placed on the major tributaries running into Lake St-Charles and St-Charles River and the wastewater effluents that are released into the watershed (wastewater plants and septic tanks). Out of the seven CECs investigated in this study, three, including Acph, Caf, and SA, were measured at levels above the quantification limits at all sampling stations. The results indicate that where conventional wastewater treatment processes are applied, trace levels of CECs are not efficiently eliminated. Across the lake and river, Acph, Caf, and SA levels did not show any significant variation (<5% standard deviation). Despite the continuous input of these contaminants via tributaries and wastewater effluents, no significant increase or bioaccumulation was observed. The accumulation of CECs in the watershed might be offset by the natural degradation (sunlight), biodegradation, and sorption potential of contaminants to the river sediments. Wastewater effluent samples mixed with river samples have a significantly higher concentration range for all the contaminants examined. The CEC concentrations in the lake and river were slightly higher at the point where the tributaries run into them. Higher levels were detected during the summer season across the sampling stations and there were no significant trends during the other seasons. The dataset for the selected CECs can play a valuable role in future research by supporting risk assessments associated with chronic exposure to ecological systems. The data generated can also be used to monitor the wastewater treatment plants' removal efficiency and contribute to identify pollution control strategies. Future studies should consider the monitoring of the CECs in sediment samples at sites corresponding to the sampling locations to understand the bioaccumulation/partitioning/persistence of the investigated CECs at trace levels. Moreover, the dilution factor of the watershed/surface water should be considered to better understand the fate patterns of CECs in the watershed.
List of abbreviations
Acph | Acetaminophen |
CBZ | Carbamazepine |
CECs | Contaminants of emerging concern |
Caf | Caffeine |
DPN | Drospirenone |
IBP | Ibuprofen |
SA | Salicylic acid |
SPE | Solid-phase extraction |
SMX | Sulfamethoxazole |
E55 | Exit of Stoneham sewage effluent |
E01 | Hurons River discharges into St-Charles Lake |
E54 | Wastewater treatment plant effluent of the City of Lac Delage |
C04 | Northern basin of St-Charles Lake |
CO1(E) | Southern basin of St-Charles Lake |
OO | Exit of golf ground |
E04 | Start of St-Charles River |
E28 | Jaune River |
E06 | Nelson River |
UTE | Exit of St-Charles Lake |
Funding
This research was funded by MITACS, grant number IT12226. This work is also a part of the research program of the NSERC (Natural Sciences and Engineering Research Council of Canada) Research Chair on Drinking Water of Laval University (CREPUL), Canada.
Conflicts of interest
There are no conflicts to declare.
Acknowledgements
Authors are thankful to Mitacs Accelerate Fellowship program for the scholarship provided to the first author. They also thank the staff of Agiro, Ville de Quebec and CREPUL for their help in sampling planning and water quality analysis, and Crayon-bleu/Blue-pencil for language editing.
References
- R. Guedes-Alonso, S. Montesdeoca-Esponda, J. A. Herrera-Melián, R. Rodríguez-Rodríguez, Z. Ojeda-González, V. Landívar-Andrade, Z. Sosa-Ferrera and J. J. Santana-Rodríguez, Sci. Total Environ., 2020, 703, 135596 CrossRef CAS PubMed.
- M. F. Meyer, S. M. Powers and S. E. Hampton, Environ. Sci. Technol., 2019, 53, 12961–12973 CrossRef CAS PubMed.
- C. Zhang, L. Barron and S. Sturzenbaum, Sci. Total Environ., 2021, 781, 146684 CrossRef CAS PubMed.
- K. O. K'oreje, M. Okoth, H. Van Langenhove and K. Demeestere, J. Environ. Manage., 2020, 254, 109752 CrossRef PubMed.
- A. Huff Chester, C. Gordon, H. A. Hartmann, S. E. Bartell, E. Ansah, T. Yan, B. Li, N. K. Dampha, P. L. Edmiston and P. J. Novak, Environ. Toxicol. Chem., 2022, 41, 369–381 CrossRef CAS PubMed.
- X. Bai, A. Lutz, R. Carroll, K. Keteles, K. Dahlin, M. Murphy and D. Nguyen, Chemosphere, 2018, 200, 133–142 CrossRef CAS PubMed.
- M. Česen, M. Ahel, S. Terzić, D. J. Heath and E. Heath, Sci. Total Environ., 2019, 650, 2446–2453 CrossRef PubMed.
- C. Fu, B. Xu, H. Chen, X. Zhao, G. Li, Y. Zheng, W. Qiu, C. Zheng, L. Duan and W. Wang, Sci. Total Environ., 2022, 807, 151011 CrossRef CAS PubMed.
- K. M. Gani and A. A. Kazmi, Contamination of emerging contaminants in Indian aquatic sources: first overview of the situation, J. Hazard., Toxic Radioact. Waste, 2017, 21, 04016026 CrossRef.
- M. L. Fuzzen, L. M. Bragg, G. R. Tetreault, P. A. Bahamonde, R. N. Tanna, C. J. Bennett, M. E. McMaster and M. R. Servos, PLoS One, 2016, 11, e0164879 CrossRef PubMed.
- Z. Tian, K. T. Peter, A. D. Gipe, H. Zhao, F. Hou, D. A. Wark, T. Khangaonkar, E. P. Kolodziej and C. A. James, Environ. Sci. Technol., 2019, 54, 889–901 CrossRef PubMed.
- J. M. Corrêa, A. L. Sanson, C. F. Machado, S. F. Aquino and R. J. Afonso, Environ. Sci. Pollut. Res., 2021, 28, 30242–30254 CrossRef PubMed.
- M. J. Focazio, D. W. Kolpin, K. K. Barnes, E. T. Furlong, M. T. Meyer and S. D. Zaugg, Sci. Total Environ., 2008, 402, 201–216 CrossRef CAS PubMed.
- Y. Valcárcel, S. González Alonso, J. L. Rodríguez-Gil, A. Gil and M. Catalá, Chemosphere, 2011, 84, 1336–1348 CrossRef PubMed.
- R. Meffe and I. de Bustamante, Sci. Total Environ., 2014, 481, 280–295 CrossRef CAS PubMed.
- K. C. Machado, M. T. Grassi, C. Vidal, I. C. Pescara, W. F. Jardim, A. N. Fernandes, F. F. Sodré, F. V. Almeida, J. S. Santana and M. C. Canela, Sci. Total Environ., 2016, 572, 138–146 CrossRef CAS PubMed.
- N. H. Tran, M. Reinhard, E. Khan, H. Chen, V. T. Nguyen, Y. Li, S. G. Goh, Q. B. Nguyen, N. Saeidi and K. Y.-H. Gin, Sci. Total Environ., 2019, 676, 252–267 CrossRef CAS PubMed.
- E. J. Tiedeken, A. Tahar, B. McHugh and N. J. Rowan, Sci. Total Environ., 2017, 574, 1140–1163 CrossRef CAS PubMed.
- S. M. Bard, Mar. Pollut. Bull., 1999, 38, 356–379 CrossRef CAS.
- S. Zhang, S. Gitungo, J. E. Dyksen, R. F. Raczko and L. Axe, Int. J. Environ. Res. Public Health, 2021, 18, 1288 CrossRef PubMed.
- B. Narancic, I. Laurion, B. B. Wolfe, S. Behmel and A. N. Rousseau, Canadian Water Resources Journal/Revue canadienne des ressources hydriques, 2020, 45, 125–143 CrossRef.
- V. Boulé, C. Vallières, V. Laflamme, M. Bouchard-Valentine, P. Jobin, C. Sauvageau, D. Plourde, P. Coté and N. Pagé, Final report to CMQ, Basel, Roche, 2010 Search PubMed.
- Agiro, 2017 Archives, https://agiro.org/annee/2017/, accessed June 28, 2022 Search PubMed.
- R. Pulicharla, F. Proulx, S. Behmel, J.-B. Sérodes and M. J. Rodriguez, Sci. Total Environ., 2021, 751, 141748 CrossRef CAS PubMed.
- D. J. Fairbairn, W. A. Arnold, B. L. Barber, E. F. Kaufenberg, W. C. Koskinen, P. J. Novak, P. J. Rice and D. L. Swackhamer, Environ. Sci. Technol., 2016, 50, 36–45 CrossRef CAS PubMed.
- C. D. Metcalfe, B. G. Koenig, D. T. Bennie, M. Servos, T. A. Ternes and R. Hirsch, Environ. Toxicol. Chem., 2003, 22, 2872–2880 CrossRef CAS PubMed.
- W.-J. Sim, J.-W. Lee and J.-E. Oh, Environ. Pollut., 2010, 158, 1938–1947 CrossRef CAS PubMed.
- A. Shraim, A. Diab, A. Alsuhaimi, E. Niazy, M. Metwally, M. Amad, S. Sioud and A. Dawoud, Arabian J. Chem., 2017, 10, S719–S729 CrossRef CAS.
- A. I. Shah, M. U. Din Dar, R. A. Bhat, J. P. Singh, K. Singh and S. A. Bhat, Ecol. Eng., 2020, 152, 105882 CrossRef.
- S. Zhang, Q. Zhang, S. Darisaw, O. Ehie and G. Wang, Chemosphere, 2007, 66, 1057–1069 CrossRef CAS PubMed.
- V. Yargeau, A. Lopata and C. Metcalfe, Water Qual. Res. J., 2007, 42, 231–239 CrossRef CAS.
- K. Drzewiecka and M. Mleczek, Acta Physiol. Plant., 2017, 39, 182 CrossRef.
- P. J. Phillips, C. Schubert, D. Argue, I. Fisher, E. T. Furlong, W. Foreman, J. Gray and A. Chalmers, Sci. Total Environ., 2015, 512–513, 43–54 CrossRef CAS PubMed.
- G. C. Richards, BMJ Evid. Based Med., 2021, 26, 65–68 CrossRef PubMed.
- L. Tan, C. Zhang, F. Liu, P. Chen, X. Wei, H. Li, G. Yi, Y. Xu and X. Zheng, J. Environ. Manage., 2021, 300, 113709 CrossRef PubMed.
- H. Al Qarni, P. Collier, J. O'Keeffe and J. Akunna, Environ. Sci. Pollut. Res., 2016, 23, 13003–13014 CrossRef CAS PubMed.
- K. M. Kanama, A. P. Daso, L. Mpenyana-Monyatsi and M. A. A. Coetzee, J. Toxicol., 2018, 2018, 3751930 Search PubMed.
- L. F. Angeles, R. A. Mullen, I. J. Huang, C. Wilson, W. Khunjar, H. I. Sirotkin, A. E. McElroy and D. S. Aga, Environ. Sci.: Water Res. Technol., 2019, 6, 62–77 RSC.
- J. Wang, A. C. Allan, W. Wang and X. Yin, N. Z. J. Crop Hortic. Sci., 2022, 50, 99–117 CrossRef CAS.
- G. Ebrahimzadeh, R. N. Nodehi, M. Alimohammadi, M. R. Rezaei Kahkah and A. H. Mahvi, J. Environ. Manage., 2021, 283, 111971 CrossRef CAS PubMed.
- S. Behravesh, N. Mirghaffari, A. A. Alemrajabi, F. Davar and M. Soleimani, Environ. Sci. Pollut. Res. Int., 2020, 27, 26929–26942 CrossRef CAS PubMed.
- C. Zhou, Q. Zhou and X. Zhang, Water Res., 2019, 148, 133–141 CrossRef CAS PubMed.
- S. N. Gummadi, B. Bhavya and N. Ashok, Appl. Microbiol. Biotechnol., 2012, 93, 545–554 CrossRef CAS PubMed.
- A. Raschitor, A. Romero, S. Sanches, V. J. Pereira, J. G. Crespo and J. Llanos, Molecules, 2021, 26, 7277 CrossRef CAS PubMed.
- M. Hasan, K. Alfredo, S. Murthy and R. Riffat, J. Environ. Manage., 2021, 295, 113071 CrossRef CAS PubMed.
- M. E. Carlotti, S. Sapino, D. Vione, C. Minero, E. Peira and M. Trotta, J. Dispersion Sci. Technol., 2007, 28, 805–818 CrossRef CAS.
- S. Poirier-Larabie, P. A. Segura and C. Gagnon, Sci. Total Environ., 2016, 557–558, 257–267 CrossRef CAS PubMed.
- S. Zhao, X. Liu, D. Cheng, G. Liu, B. Liang, B. Cui and J. Bai, Sci. Total Environ., 2026, 569–570, 1350–1358 Search PubMed.
|
This journal is © The Royal Society of Chemistry 2022 |
Click here to see how this site uses Cookies. View our privacy policy here.