DOI:
10.1039/D2RA02851D
(Paper)
RSC Adv., 2022,
12, 15440-15446
Environmentally friendly domino multicomponent strategy for the synthesis of pyrroloquinolinone hybrid heterocycles†
Received
5th May 2022
, Accepted 13th May 2022
First published on 25th May 2022
Abstract
An efficient and elegant assembly of pyrene/aryl fused pyrrolo[2,3-b]quinolinone and pyrrolizino[3,2-b]quinolinone hybrid heterocycles was achieved via a domino multicomponent reaction strategy using a solid state melt reaction (SSMR) condition. The 1,3-dipole component was generated in situ from N-methylgylcine/L-proline and isatin, while the Baylis–Hillman adduct prepared from pyrene-1-carbaldehyde and various benzaldehydes is used as the dipolarophile. The domino protocol comprises 1,3-dipolar cycloaddition and a consequent double annulation reaction process. The advantages of this cascade protocol include environmentally friendly conditions, the avoidance of toxic organic solvents, simple work-up and good to excellent product yields.
Introduction
Domino multicomponent reaction is an attractive synthetic methodology that creates several transformations with notable advantages such as high reaction efficiency, convergence, elegance, cost savings, simple automation and minimization of synthetic work up steps.1 This protocol obviates the isolation and purification steps which leads to an improvement in the overall yield compared to classical multistep synthetic transformations in a single pot manipulation and represents an attractive synthetic method for building hybrid heterocyclic architectures.2 Besides, this protocol is well suited for the production of structurally diverse hybrid heterocycles with multiple stereogenic centers and biologically relevant molecules.3
Domino multicomponent reactions involving 1,3-dipolar cycloadditions are attractive in this connection as this reaction allows for the efficient assembly of biologically relevant five-membered heterocycles. This synthetic protocol has recently received considerable attention in organic synthesis.4–7 In particular, the synthesis of the pyrrolidine unit by the reaction of alkenes with azomethine ylides gains importance because of their occurrence in numerous bioactive natural products.8–10 In this context, we recently reported the synthesis of structurally unusual hybrid heterocycles comprising spiropyrrolidine units11 and pyrroloquinolinone analogs prepared from Baylis–Hillman adducts via the multicomponent 1,3-dipolar cycloaddition and domino reaction sequence.12 Spiropyrrolidine analogues possess an interesting biological profiles including antimicrobial,13 anti-cancer14 and anti-Alzheimer15,16 activities. Pyrroloquinolinone analogs are found to be an essential component of many natural products such as melodinus alkaloids, (+) scandin, (+) meloscine, meloscandinone17,18 which are used in traditional Chinese folkmedicine to treat meningitis in children and rheumatic heart disease.
With an aim to construct novel heterocycles comprising pyrrolo [3,2-c]quinolino/pyrrolizino [2,3-c] quinolinone, we have now investigated the synthetic utility of Baylis–Hillman adducts as dipolarophiles through sustainable green domino protocol that involves a decarboxylative 1,3-dipolar cycloaddition19–21 followed by a double annulation via sequential lactonization and lactamization reactions. Our synthetic strategy employed for the synthesis of pyrroloquinoline heterocycles is shown in Fig. 1.
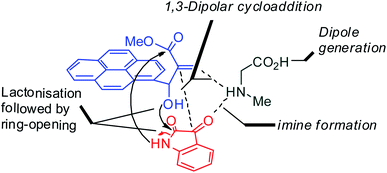 |
| Fig. 1 Synthetic strategy of pyrroloquinolinones. | |
Results and discussion
The structurally unexplored new class of highly functionalized starting precursors viz. 3-(1,8-dihydropyren-1-yl)-2-methylenebutanoate 3 and various substituted methyl 2-(hydroxy(phenyl)methyl)acrylate 5 were required for the synthesis of pyrroloquinolinones, which were synthesized from pyrene-1-carbaldehyde 2 and various substituted benzaldehydes 4 by the Baylis–Hillman reaction (Scheme 1). Incidentally, pyrene is important polycyclic aromatic ring system that has been shown diverse biological activity including cytotoxicity against human cancer cell lines.22
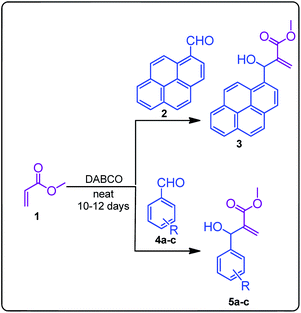 |
| Scheme 1 Synthesis of Baylis–Hillman adducts. | |
The domino multicomponent reaction was carried out by the reaction of isatin 6, sarcosine 7 and the Balylis–Hillman adduct 3 under solvent free melting conditions to furnish the unexpected pyrene tethered pyrroloquinolinone hybrids 9a–d in excellent yields (Scheme 2). Firstly, the domino reaction was examined with various solvent systems such as CH3CN, toluene, xylene and dioxane as summarized in Table 1. However, even after a longer reaction time under these solvent conditions, we did not obtain any fruitful results, but instead ended up with an inseparable mixture of the products. Ultimately, the domino reaction was carried out under solid state melt reaction conditions. Thus, in a typical reaction, the starting substrate 3, 6a and 7 in a round bottom flask at 180 °C under solvent free conditions afforded the desired product 9a in 93% yield without column purification. It was found that 180 °C was the optimum temperature for this solid-state melt reaction in terms of both yield and time. The reaction was also performed with Baylis–Hillman adduct 5a–c under the above optimized reaction conditions, which afforded 10 in good to excellent yields. It is pertinent to note that the compounds synthesized are racemates which has been indicated in Table 2.
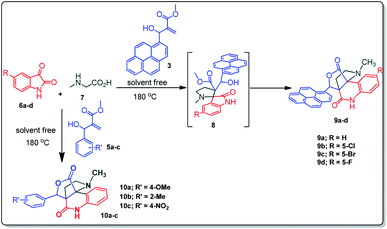 |
| Scheme 2 Synthesis of pyrroloquinolinone hybrids 9a–d and 10a–c. | |
Table 1 Optimization of reaction conditions for the synthesis of compound 9a
Entry |
Solvents |
T (°C) |
Time |
Yielda (%) |
Isolated yield of the products. Reactants melted at the mentioned temperature. |
1 |
Acetonitrile |
85 |
12 h |
— |
2 |
Toluene |
110 |
12 h |
— |
3 |
Xylene |
120 |
12 h |
— |
4 |
Dioxane |
100 |
12 h |
— |
5 |
Noneb |
100 |
1 h |
— |
6 |
Noneb |
130 |
1 h |
15 |
7 |
Noneb |
160 |
1 h |
63 |
8 |
Noneb |
180 |
10 min |
93 |
Table 2 Synthesis of aryl fused polycyclic pyrroloquinolinone derivatives
Entry |
Dipolarophile |
1,2-Diketone |
Sec-amino acid |
Mixture of stereoisomersa |
Yieldb |
Isolated products are mixture of stereoisomers. Yield of isolated product after column chromatography. |
1 |
3 |
 |
 |
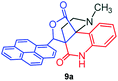 |
93 |
2 |
3 |
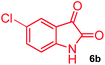 |
 |
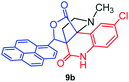 |
96 |
3 |
3 |
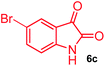 |
 |
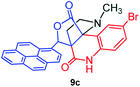 |
95 |
4 |
3 |
 |
 |
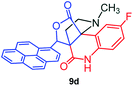 |
91 |
5 |
5a |
 |
 |
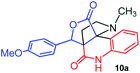 |
92 |
6 |
5b |
 |
 |
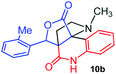 |
93 |
7 |
5c |
 |
 |
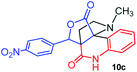 |
87 |
8 |
3 |
 |
 |
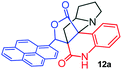 |
94 |
9 |
3 |
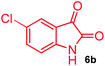 |
 |
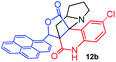 |
91 |
10 |
3 |
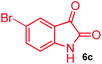 |
 |
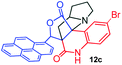 |
92 |
The formation of the pyrroloquinoline 9a was unambiguously determined by spectroscopic data. In the 1H and 13C NMR spectrum of 9a, interestingly, the absence of the methoxy(–OCH3) and the –OH groups confirm the formation of the unusual cyclized product. In the 1H NMR spectrum, the hydrogens of the –NCH3 unit and –NH of lactam resonated as singlet at δ 2.63 and 10.78 ppm respectively. The –NCH2 hydrogens of the pyrrolidine ring exhibited as multiplets at δ 2.84 and δ 3.07. In the 13C NMR spectra, the carbon signals at δ 178.9 and 173.2 ppm were ascribable to quinolinone and lactone ring carbonyl carbons, respectively. The carbon signal at δ 39.3 ppm was assignable to –NCH3 carbon and the two carbon signals at δ 64.3 and 78.5 ppm were due to two bridged quaternary carbons, respectively. The two methylene groups appeared in negative region at δ 30.9 and 57.3 ppm which was further confirmed by DEPT-135 NMR spectrum. The observed chemical shifts are in agreement with the structure of 9a. Finally, compound 9a was unambiguously determined by single crystal X-ray crystallographic analysis as shown in Fig. 2.23
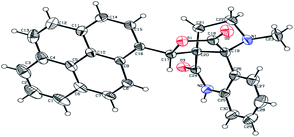 |
| Fig. 2 ORTEP diagram of compound 9a. | |
Further, we have extended the scope of this multicomponent cascade transformation, by using cyclic amino acid namely L-proline, as described in Scheme 3. Thus, the reaction of non-stabilized azomethine ylides generated in situ from isatin 6a–c and L-proline 11 with the Baylis–Hillman adduct 3 under the optimized conditions afforded pyrrolizinoquinolinone hybrids 12a–c in good yields. The structure of pyrrolizinoquinoline was elucidated by spectroscopic data.
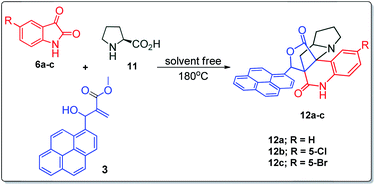 |
| Scheme 3 Synthesis of pyrene fused pyrrolizidinoquinolines | |
A likely mechanistic pathway for the assembly of pyrroloquinolinone hybrids via the multicomponent domino reaction is shown in Scheme 4. Initially, the reaction of isatin 6 and sarcosine 7 forms the non-stabilized 1,3-dipole component 13 through spontaneous dehydration and decarboxylation. Subsequently, the reaction of dipole component 13 with the dipolarophile 3 can take place through spiro intermediate 14 or 8 to furnish 9. Thus, the exclusive formation of spiro intermediate 8 in the cycloaddition process shows that compound 8 is favoured over compound 14. Subsequently, the hydroxyl group of spiro intermediate 8 underwent an intramolecular nucleophilic attack on the carbonyl of the adjacent oxindole ring, leading to the formation of the intermediate 15, which in turn led to an aniline intermediate 16 through stabilization of the oxygen anion. Finally, the amino group was cyclized to the final product 9 by nucleophilic attack on ester carbonyl followed by elimination of the methoxy group. Formation of an unusual product by two C–N, three C–C bonds that have three stereogenic center in single-pot synthetic operation.
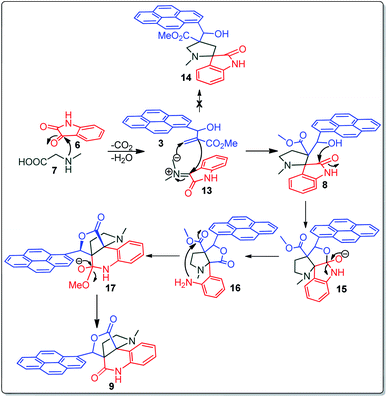 |
| Scheme 4 Plausible reaction pathway for the formation of 9. | |
Conclusions
In summary, we have successfully synthesized structurally interesting unexplored new class of pyrene/aryl derived pyrroloquinolinone and pyrene derived pyrrolizinoquinoline hybrid heterocycles employing domino multicomponent reaction strategy under eco-friendly solid-state melt reaction conditions. Besides, the starting precursor, the electron deficient pyrene alkene, was synthesized for the first time in excellent yield through Baylis–Hillman reaction. The domino reaction involves 1,3-dipolar cycloaddition followed by double annulation cascade reaction process, the product generates up to three stereocenter via two C–N and three C–C bonds. It is noteworthy to mention that this domino reaction was performed under solvent and catalyst free conditions, which is very attractive from green chemistry perspective.
Experimental
General considerations
1H and 13C NMR spectra were determined on a BRUKER 300 MHz NMR instrument in chloroform-d6 solvent with trimethyl silane (TMS) as a reference standard. High resolution mass spectra were obtained by ESI using Orbitrap elite mass spectrometer. C, H and N analyses were performed using PerkinElmer CHNS 2400B instrument. The X-ray diffraction analysis was determined on a Bruker (2008) SMART APEX II detector diffractometer. Silica gel (mesh size 100–200) was used for column chromatography and hexane and ethyl acetate as eluent. The reaction was monitoring by thin layer chromatography (TLC) developed on 25 mm silica gel-G (ACME) coated glass plates and monitoring by iodine.
General procedure for synthesis of BAH, 3/5
A mixture of methyl acrylate 1 (1.5 mmol), pyrene-1-carbaldehyde 2 (1.0 mmol)/substituted benzaldehydes 4 (1.0 mmol) and DABCO (0.1 mmol) were allowed to stand at ambient temperature for 12–15 days. Upon completion of the reaction as evidenced by TLC analysis, the reaction mixture was diluted with ethyl acetate (20 mL) and washed successively with 2 N HCl solution, H2O and aqueous sodium bicarbonate solution. Organic solvent was dried over anhydrous Na2SO4 and evaporated under vaccuo. The residue was purified using column chromatography using hexane
:
EtOAc (8
:
2 v/v) to afford the alcohol 3/5.
Methyl 2-(hydroxy(pyren-1-yl)methyl)acrylate 3. 1H NMR (300 MHz, CDCl3): δ 3.72 (s, –OCH3, 3H), 5.56 (s, 1H), 6.35 (s, 1H), 6.62 (s, 1H), 7.94–8.23 (m, 9H). 13C NMR (75 MHz, CDCl3): 52.11, 69.63, 123.0, 124.5, 124.7, 124.9, 125.2, 125.3, 125.9, 127.1, 127.4, 127.5, 127.8, 128.2, 130.6, 131.1, 131.3, 133.9, 142.2, 167.2 ppm. Mass: m/z 316.15 (M+).
General procedure for synthesis of fused pyrrolo[3,2-c] quinolinone hybrids, 9/10
A mixture of BHA 3/5 (1 mmol), isatin 6 (1.1 mmol) and sarcosine 7 (1.1 mmol) was placed in a flask and melted at 180 °C, the completion of the reaction as confirmed by TLC. After the disappearance of the starting materials, the crude product was recrystallized with mixture of 5 mL ethyl acetate and hexane (ratio 1
:
4), successfully gave the pure desired product 9/10 as a solid.
(3aR*,9bR*,12R*)-1-Methyl-12-(pyren-1-yl)-2,3-dihydro-1H-3a,9b-(methanooxymethano)pyrrolo[3,2-c]quinoline-4,10(5H)-dione, 9a. Colorless solid (93%), Mp: 246–248 °C, IR (KBr): 1709, 1742 cm−1; 1H NMR (300 MHz, CDCl3 + DMSO-d6): δ 2.37–2.47 (m, 2H), 2.63 (s, –NCH3, 3H), 2.78–2.90 (m, 1H), 3.07–3.09 (m, 1H), 6.53 (s, 1H), 7.16 (t, J = 7.5, 6.6 Hz, 1H), 7.36–7.41 (m, 1H), 7.80 (d, J = 7.8 Hz, 1H), 7.95–8.22 (m, 9H), 10.78 (s, 1H, N–H). 13C NMR (75 MHz, CDCl3 + DMSO-d6): 31.0, 39.3, 57.3, 64.3, 78.5, 118.9, 121.6, 127.1, 127.9, 129.2, 129.3, 129.4, 130.0, 130.4, 130.9, 131.6, 132.0, 132.4, 132.8, 133.3, 134.4, 135.1, 135.5, 135.9, 136.3, 142.7, 173.2, 178.9 ppm. Mass: m/z 458.50 (M+). Anal. calcd. For C30H22N2O3: C, 78.59, H, 4.84, N, 6.11%; found: C, 78.61, H, 4.81, N, 6.14%.
(3aR*,9bR*,12R*)-8-Chloro-1-methyl-12-(pyren-1-yl)-2,3-dihydro-1H-3a,9b-(methanooxymethano)pyrrolo[3,2-c]quinoline-4,10(5H)-dione, 9b. Colorless solid (96%), Mp: 252–254 °C, IR (KBr): 1710, 1739 cm−1; 1H NMR (300 MHz, CDCl3 + DMSO-d6): δ 2.14–2.22 (m, 1H), 2.34–2.40 (m, 1H), 2.56 (s, −NCH3, 3H), 2.72 (dd, J = 8.7, 9.0 Hz, 1H), 3.02 (dd, J = 6.0, 6.3 Hz, 1H), 6.73 (s, 1H), 7.30 (d, J = 8.4 Hz, 1H), 7.52 (d, J = 8.7 Hz, 1H), 7.66 (s, 1H), 8.03–8.37 (m, 9H), 11.32 (s, 1H, N–H). 13C NMR (75 MHz, CDCl3 + DMSO-d6): 26.0, 33.9, 52.0, 58.6, 72.6, 76.0, 115.7, 118.2, 122.5, 123.6, 123.8, 124.2, 124.5, 125.2, 125.5, 126.2, 126.5, 126.9, 127.0, 127.2, 127.9, 128.1, 128.4, 129.9, 130.4, 130.6, 131.0, 137.1, 167.5, 173.2 ppm. Mass: m/z 492.16 (M+). Anal. calcd. For C30H21ClN2O3: C, 73.09, H, 4.29, N, 5.68%; found: C, 73.11, H, 4.27, N, 5.70%.
(3aR*,9bR*,12R*)-1-Methyl-12-(pyren-1-yl)-2,3-dihydro-1H-3a,9b-(methanooxymethano)pyrrolo[3,2-c]quinoline-4,10(5H)-dione, 9c. Colorless solid (95%), Mp: 261–263 °C, IR (KBr): 1707, 1735 cm−1; 1H NMR (300 MHz, DMSO-d6): δ 2.04–2.12 (m, 1H), 2.28–2.37 (m, 1H), 2.50 (s, –NCH3, 3H), 2.68–2.77 (m, 1H), 2.99–3.04 (m, 1H), 6.87 (s, 1H), 7.23 (d, J = 8.4 Hz, 1H), 7.73 (d, J = 8.1 Hz, 2H), 8.04–8.42 (m, 9H), 11.34 (s, 1H, N–H). 13C NMR (75 MHz, DMSO-d6): 26.1, 33.9, 52.0, 58.6, 72.6, 76.0, 113.9, 116.2, 118.7, 122.9, 123.5, 123.7, 124.4, 124.8, 125.4, 125.7, 126.4, 127.1, 128.0, 128.2, 129.9, 130.7, 130.9, 131.1, 133.4, 137.6, 167.6, 173.5 ppm. Mass: m/z 536.12 (M+).Anal. calcd. For C30H21BrN2O3: C, 67.05, H, 3.94, N, 5.21%; found: C, 73.11, H, 4.27, N, 5.70%.
(3aR*,9bR*,12R*)8-Fluoro-1-methyl-12-(pyren-1-yl)-2,3-dihydro-1H-3a,9b-(methanooxymethano)pyrrolo[3,2-c]quinoline-4,10(5H)-dione, 9d. Colorless solid (91%), Mp: 242–244 °C, IR (KBr): 1712, 1741 cm−1; 1H NMR (300 MHz, CDCl3 + DMSO-d6): δ 2.16–2.24 (m, 1H), 2.33–2.40 (m, 1H), 2.52 (s, –NCH3, 3H), 2.73 (dd, J = 8.4, 9.0 Hz, 1H), 3.01–3.06 (m, 1H), 6.67 (s, 1H), 7.31 (d, J = 6.3 Hz, 2H), 7.43 (d, J = 8.7 Hz, 1H), 8.04–8.35 (m, 9H), 11.22 (s, 1H, N–H). 13C NMR (75 MHz, CDCl3 + DMSO-d6): 23.6, 31.6, 49.7, 56.1, 70.4, 73.7, 76.2, 112.8, 112.9, 113.0, 113.1, 114.9, 115.2, 115.6, 115.7, 120.1, 121.2, 121.5, 121.8, 122.1, 122.8, 123.1, 123.7, 124.5, 124.6, 124.8, 125.4, 125.8, 127.5, 128.3, 128.6, 132.2, 165.0, 170.9 ppm. Mass: m/z 476.18 (M+). Anal. calcd. For C30H21FN2O3: C, 75.62, H, 4.44, N, 5.88% found: C, 75.65, H, 4.46, N, 5.85%.
(3aR*,9bR*,12R*)-1-Methyl-12-(4-methoxyphenyl)-2,3-dihydro-1H-3a,9b-(methanooxymethano)pyrrolo[3,2-c]quinoline-4,10(5H)-dione, 10a. Colorless solid (92%), Mp: 220–222 °C, IR (KBr): 1709, 1744 cm−1; 1H NMR (300 MHz, CDCl3): δ 2.07–2.16 (m, 1H), 2.40–2.58 (m, 1H), 2.61 (s, –NCH3, 3H), 2.73 (q, J = 9.0, 18.0 Hz, 1H), 3.07–3.14 (m, 1H), 3.81 (s, –OCH3, 3H), 5.39 (s, 1H), 6.87–6.90 (m, 2H), 7.02 (d, J = 8.1 Hz, 1H), 7.18 (t, J = 7.2 Hz, 1H), 7.30 (d, J = 8.7 Hz, 2H), 7.39 (t, J = 7.2 Hz, 1H), 7.80 (d, J = 7.5 Hz, 1H), 9.94 (s, 1H, N–H). 13C NMR (75 MHz, CDCl3): 26.7, 34.3, 52.4, 55.2, 59.1, 73.0, 79.4, 113.7, 114.6, 116.5, 123.8, 126.0, 127.5, 129.9, 130.7, 136.7, 159.8, 170.2, 173.7 ppm. Mass: m/z 364.14 (M+). Anal. calcd. For C21H20N2O4: C, 69.22, H, 5.53, N, 7.69%; found: C, 69.27, H, 5.60, N, 7.63%.
(3aR*,9bR*,12R*)-1-Methyl-12-(o-tolyl)-2,3-dihydro-1H-3a,9b(methanooxymethano)pyrrolo[3,2-c]quinoline-4,10(5H)-dione, 10b. Colorless solid (93%), Mp: 268–270 °C, IR (KBr): 1709, 1744 cm−1; 1H NMR (300 MHz, CDCl3): δ 2.19 (s, –NCH3, 3H), 2.37–2.52 (m, 2H), 2.59 (s, 3H), 2.80 (q, J = 9.1, 17.7 Hz, 1H), 3.07–3.15 (m, 1H), 5.68 (s, 1H), 6.96 (d, J = 7.8 Hz, 1H), 7.13–7.29 (m, 4H) 7.41 (t, J = 7.8 Hz, 1H), 7.47–7.50 (m, 1H), 7.80 (d, J = 7.5 Hz, 1H), 9.39 (s, 1H, N–H). 13C NMR (75 MHz, CDCl3): 19.2, 26.0, 34.4, 52.5, 59.0, 73.6, 76.4, 114.7, 116.3, 123.9, 125.8, 127.1, 128.8, 130.1, 130.7, 131.0, 131.6, 135.8, 136.8, 169.7, 173.8 ppm. Mass: m/z 348.14 (M+). Anal. calcd. For C21H20N2O3: C, 72.40; H, 5.79; N, 8.04%; found: C, 72.45, H, 6.84, N, 7.99%.
(3aR*,9bR*,12R*)-1-Methyl-12-(4-nitrophenyl)-2,3-dihydro-1H-3a,9b-(methanooxymethano)pyrrolo[3,2-c]quinoline-4,10(5H)-dione, 10c. Colorless solid (87%), Mp: 250–252 °C, IR (KBr): 1720, 1745 cm−1; 1H NMR (300 MHz, CDCl3): δ 1.90–2.04 (m, 1H), 2.44–2.55 (m, 1H), 2.62 (s, –NCH3, 3H), 2.76 (q, J = 9.0, 17.7 Hz, 1H), 3.09–3.16 (m, 1H), 5.49 (s, 1H), 7.02 (d, J = 8.1 Hz, 1H), 7.24 (t, J = 7.5 Hz, 1H), 7.46 (t, J = 7.8 Hz, 1H), 7.61–7.64 (m, 2H), 7.79 (d, J = 7.8 Hz, 1H), 8.23–8.23 (m, 2H), 9.87 (s, 1H, N–H). 13C NMR (75 MHz, CDCl3): 26.9., 34.1, 52.2, 58.6, 73.0, 78.2, 114.2, 116.5, 123.5, 124.2, 127.3, 129.9, 131.1, 136.4, 141.3, 148.1, 169.6, 172.7 ppm. Mass: m/z 379.11 (M+): anal. calcd. For C20H17N3O5: C, 63.32, H, 4.52, N, 11.08%; found: C, 63.37, H, 4.55, N, 11.10%.
(6aR*,7aR*,11aR*,14R*)-14-(Pyren-1-yl)-7a,8,9,10-tetrahydro-5H-6a,11a-(methanooxymethano)pyrrolizino[2,3-c]quinoline-6,12(7H)-dione, 12a. Colorless solid (94%), Mp: 248–250 °C, IR (KBr): 1719, 1748 cm−1; 1H NMR (300 MHz, CDCl3 + DMSO-d6): δ 1.32–1.34 (m, 1H), 1.60–1.68 (m, 2H), 1.99–2.04 (m, 2H), 2.39–2.44 (m, 1H), 2.53–2.63 (m, 2H), 3.66–3.72 (m, 1H), 6.59 (s, 1H), 7.18–7.48 (m, 3H), 7.78 (d, J = 8.1 Hz, 1H), 8.05–8.32 (m, 9H), 11.17 (s, 1H, N–H). 13C NMR (75 MHz, CDCl3 + DMSO-d6): 29.9, 36.9, 39.5, 53.9, 64.3, 68.1, 81.9, 119.6, 121.5, 127.6, 128.5, 128.9, 129.2, 129.3, 129.5, 130.3, 130.7, 131.3, 132.2, 132.5, 132.7, 133.0, 133.3, 133.6, 135.1, 135.6, 135.9, 136.1, 143.8, 173.2, 180.5 ppm. Mass: m/z 484.19 (M+). Anal. calcd. For C32H24N2O3: C, 79.32, H, 4.99, N, 5.78% found: C, 79.35, H, 4.96, N, 5.75%.
(6aR*,7aR*,11aR*,14R*)-2-Chloro-14-(pyren-1-yl)-7a,8,9,10-tetrahydro-5H-6a,11a-(methanooxymethano)pyrrolizino[2,3-c]quinoline-6,12(7H)-dione, 12b. Colorless solid (91%), Mp: 256–258 °C, IR (KBr): 1719, 1748 cm−1; 1H NMR (300 MHz, CDCl3 + DMSO-d6): δ 1.32–1.36 (m, 1H), 1.67–1.71 (m, 2H), 1.96–2.11 (m, 2H), 2.42–2.68 (m, 3H), 3.67–3.72 (m, 1H), 6.65 (s, 1H), 7.27–8.34 (m, 12H), 11.29 (s, 1H, N–H). 13C NMR (75 MHz, CDCl3 + DMSO-d6): 24.5, 31.4, 34.6, 48.6, 58.8, 62.9 76.4, 116.0, 117.9, 122.3, 123.7, 123.9, 124.1, 124.2, 125.1, 125.5, 126.0, 126.5, 127.3, 127.5, 127.8, 127.9, 128.1, 128.4, 129.9, 130.4, 130.6, 131.0, 137.3, 167.8, 174.9 ppm. Mass: m/z 518.19 (M+). Anal. calcd. For C32H23ClN2O3: C, 74.06, H, 4.47, N, 5.40% found: C, 74.09, H, 4.45, N, 5.43%.
(6aR*,7aR*,11aR*,14R*)-2-Bromo-14-(pyren-1-yl)-7a,8,9,10-tetrahydro-5H-6a,11a-(methanooxymethano)pyrrolizino[2,3-c]quinoline-6,12(7H)-dione, 12c. Colorless solid (92%), Mp: 259–261 °C, IR (KBr): 1725, 1756 cm−1; 1H NMR (300 MHz, CDCl3 + DMSO-d6): δ 1.34–1.42 (m, 1H), 1.68–1.80 (m, 2H), 2.04–2.17 (m, 3H), 2.45–2.57 (m, 1H), 2.73–2.80 (m, 1H), 3.73–3.79 (m, 1H), 6.56 (s, 1H), 7.23 (s, 1H), 7.54 (s, 1H), 7.66 (s, 1H), 8.00–8.26 (m, 9H), 11.16 (s, 1H, N–H). 13C NMR (75 MHz, CDCl3 + DMSO-d6): 29.8, 36.7, 39.6, 54.0, 64.2, 68.2, 82.3, 121.4, 123.2, 127.1, 129.1, 129.3, 130.1, 130.6, 131.0, 132.0, 132.5, 132.9, 133.3, 135.1, 135.9, 136.3, 138.5, 173.2, 180.3 ppm. Mass: m/z 562.11 (M+). Anal. calcd. For C32H23BrN2O3: C, 68.21, H, 4.11, N, 4.97% found: C, 68.24, H, 4.08, N, 4.95%
Conflicts of interest
There is no conflict of interest.
Acknowledgements
Authors thank the Department of Science and Technology (DST), New Delhi for financial support. SM and RR thanks CSIR, New Delhi for SRF Fellowship. RR thanks UGC New Delhi for BSR Faculty Fellowship. The project was supported by Researchers Supporting Project number (RSP-2021/143), King Saud University, Riyadh, Saudi Arabia.
Notes and references
- B. Jiang, G. Zhang, N. Ma, F. Shi, S. J. Tu, P. Kaur and G. Li, Org. Biomol. Chem., 2011, 9, 3834 RSC.
- H. Jia, H. Feng and Z. Sun, Org. Biomol. Chem., 2015, 13, 8177 RSC.
-
(a) L. F. Tietze, Chem. Rev., 1996, 96, 115 CrossRef CAS PubMed;
(b) H. Pellissier, Tetrahedron, 2006, 62, 1619 CrossRef CAS;
(c) H. Pellissier, Tetrahedron, 2006, 62, 2143 CrossRef CAS;
(d) N. T. Dat, J. H. Lee, K. Lee, Y. S. Hong, Y. H. Kim and J. J. Lee, Nat. Prod., 2008, 71, 1696 CrossRef PubMed.
- S. Kobayashi and A. K. Jorgensen, Cycloaddition Reactions in Organic Synthesis, Wiley, Weinheim, Germany, 2002 Search PubMed.
- G. Kanagaraju and A. Thangamani, Orient. J. Chem., 2014, 30, 1619 CrossRef CAS.
- P. Dhanalakshmi, S. Sriram Babu, S. Thimmarayaperumal and S. Shanmugam, RSC Adv., 2015, 5, 33705 RSC.
- G. Bhaskar, Y. Arun, C. Balachandran, C. Saikumar and P. T. , Perumal, Eur. J. Med. Chem., 2012, 51, 79 CrossRef CAS PubMed.
- B. Yu, D. Q. Yu and H. M. Liu, Eur. J. Med. Chem., 2015, 97, 673 CrossRef CAS PubMed.
- N. Deppermann, H. Thomanek, A. H. Prenzel and W. Maison, J. Org. Chem., 2010, 75, 5994 CrossRef CAS PubMed.
-
(a) K. Bernauer, G. Englert and W. Vetter, Experientia, 1965, 21, 374 CrossRef CAS PubMed;
(b) K. Bernauer, G. Englert, W. Vetter and E. Weiss, Helv. Chim.Acta, 1969, 52, 1886 CrossRef CAS;
(c) J. R. Cannon, K. D. Croft, Y. Matsuki, V. A. Patrick, R. F. Toia and A. H. White, Aust. J. Chem., 1982, 35, 1655 CrossRef CAS.
- N. Arumugam, A. I. Almansour, R. Suresh Kumar, V. Siva Krishna, D. Sriram and R. Padmanaban, Arab. J. Chem., 2021, 14, 102938 CrossRef CAS.
- R. Rajesh and R. Raghunathan, Eur. J. Org.Chem., 2013, 13, 2597 CrossRef.
-
(a) N. Arumugam, R. Raghunathan, A. I. Almansour and U. Karama, Bioorg. Med. Chem. Lett., 2012, 22, 1375 CrossRef CAS PubMed;
(b) N. Arumugam, G. Periyasami, R. Raghunathan, S. Kamalraj and J. Muthumary, Eur. J. Med. Chem., 2011, 46, 600 CrossRef CAS PubMed.
-
(a) A. I. Almansour, N. Arumugam, R. Suresh Kumar, P. V. Subbarayan, A. A. Alshatwi and H. A. Ghabbour, Anticancer Compounds, US Pat., 9486444 B1, 2016 Search PubMed;
(b) I. A. Abdulrahman, N. Arumugam, R. Suresh Kumar, V. S. Periasamy, A. A. Ali and J. Athinarayanan, Anticancer compound, US Pat., 9873699B1, 2018 Search PubMed.
- N. Arumugam, R. Suresh Kumar, I. A. Abdulrahman, M. Altaf, R. Padmanaban, P. Sureshbabu, G. Angamuthu, D. Kotresha, T. S. Manohar and S. Venketesh, Bioorg. Chem., 2018, 79, 64 CrossRef CAS PubMed.
-
(a) R. Suresh Kumar, I. A. Abdulrahman, N. Arumugam, M. A. Dhaifellah, A. Basiri, D. Kotresha, T. S. Manohar, S. Venkesh and M. A. A. M. Asiri, Bioorg. Chem., 2018, 81, 134 CrossRef PubMed;
(b) R. Suresh Kumar, I. A. Abdulrahman, N. Arumugam, M. Q. A. Dhaifellah, M. Altaf, A. Basiri, D. Kotresha, T. S. Manohar and S. Venkesh, Bioorg. Chem., 2018, 77, 263 CrossRef PubMed.
- K. S. Feldman and J. F. Antoline, Tetrahedron, 2013, 69, 1434 CrossRef CAS PubMed.
-
(a) M. Plat, M. Hachem-Mehri, M. Koch, U. Scheidegger and P. Potier, Tetrahedron Lett., 1970, 11, 395 CrossRef;
(b) N. Rodier, Y. Mauguen, M. Hachem-Mehri and M. Plat, Acta. Crystallogr., Sect.B: Struct. Sci., 1978, 34, 232 CrossRef.
-
(a) S. Paul and Y. R. Lee, Green Chem., 2016, 18, 1488 RSC;
(b) M. Bakthadoss, G. Sivakumar and D. Kannan, Org. Lett., 2009, 11, 4466 CrossRef CAS PubMed;
(c) M. Bakthadoss, D. Kannan and R. Selvakumar, ChemComm, 2013, 49, 10947 RSC.
-
(a) N. Arumugam, A. I. Almansour, S. Kumar, V. S. Periasamy, J. Athinarayanan, A. A. Ali, G. Periyasami, M. Altaf and J. C. Menéndez, Tetrahedron, 2018, 74, 5358 CrossRef CAS;
(b) N. Arumugam, A. I. Almansour, R. Suresh Kumar, J. C. Menéndez, M. A. Sultan, U. Karama, H. A. Ghabbour and H. K. Fun, Molecules, 2015, 20, 16142 CrossRef CAS PubMed.
-
(a) N. Arumugam, A. I. Almansour, R. Suresh Kumar, S. Perumal, H. A. Ghabbour and H. K. Fun, Tetrahedron Lett., 2013, 54, 2515 CrossRef CAS;
(b) N. Arumugam, I. A. Abdulrahman, R. Suresh Kumar, G. Periyasami, M. A. Dhaifallah, R. Krishnamoorthy, V. S. Periasamy, A. A. Ali, S. M. Mahalingam, T. Shankar and J. C. Menéndez, Molecules, 2018, 23, 1094 CrossRef PubMed.
-
(a) Y. Sangoi, O. Sakuleko, S. Yuenyongsawad, A. Kanjana-opas, K. Ingkaninan, A. Plubrukan and K. M. Suwanborirux, Drugs, 2008, 6, 578 Search PubMed;
(b) P. W. Okanya, K. I. Mohr, K. Gerth, R. Jansen and R. Muller, J. Nat. Prod., 2011, 74, 603 CrossRef CAS PubMed;
(c) L. Ni, Z. Li, F. Wu, J. Xu, X. Wu, L. Kong and H. Yao, Tetrahedron Lett., 2012, 53, 1271 CrossRef CAS;
(d) A. J. Carroll, S. Duffy and V. M. Avery, J. Org. Chem., 2010, 75, 8291 CrossRef CAS PubMed;
(e) D. J. Panarese and C. W. Lindsley, Org. Lett., 2012, 14, 5808 CrossRef PubMed.
-
(a) Crystallographic data (including structure factors) for the compound 9a in this article have been deposited with the Cambridge Crystallographic Data Centre as supplementary publication number CCDC 885083.†;
(b) R. Raju, R. Raghunathan, N. Arumugam, A. I. Almansour, R. Suresh Kumar, R. Padmanaban, S. Yeswanthkumar, V. Viswanathan and K. Perumal, J. Mol. Struct., 2022, 1259, 1327 CrossRef.
|
This journal is © The Royal Society of Chemistry 2022 |