DOI:
10.1039/D2RA02465A
(Paper)
RSC Adv., 2022,
12, 16229-16234
An efficient route to the synthesis of novel zwitterionic pyridinium-cyanopropenides with 3-heteroaryl-substituted trimethinium salts†
Received
17th April 2022
, Accepted 24th May 2022
First published on 30th May 2022
Abstract
In this study, eight new zwitterionic derivatives were synthesized using a simple design method from the reaction of various 2-substituted 1,3-bis(dimethylamino)-trimethinium salts with malononitrile or ethyl 2-cyanoacetate in excellent yields in the presence of triethylamine in ethanol at reflux. The molecular structures of the new compounds were confirmed by IR, UV/vis, mass, 1H, and 13C NMR spectra as well as by elemental analyses.
Introduction
Polymethine dyes constitute an independent class of conjugated π systems different from polyenes and aromatics. Polymethinic π systems are conjugated planar open-chain or ring compounds of the general formula 1 with high polarizabilities and medium-sized delocalization energies, with equal π-bond orders but unequal (alternating) π-electron densities along the carbon chain as well as relatively high chemical reactivity, preferring substitution over addition reactions.1,2 Polymethine dyes have unique inherent properties include conjugated structure, relatively good stability, medium fluorescence intensities, high molar absorption coefficients (about 105 dm3 mol−1 cm−1), and narrow bandwidths invisible region.3,4
Cyanine dyes are compounds that have a wide range of applications in many fields of science, pharmacology, medicine, and technology engineering. Such as bactericidal and fungicidal, anti-cancer, acid–base indicators, laser technology, organic solar cells, dyes for polymers, and spectral sensitizers for silver halide emulsion, etc.5–14
According to formula 1, cyanine dyes are a conjugate chain of carbon atoms located between two nitrogen centers, which this polymethine bridge links an electron donor group at one end and an electron acceptor group at the other. Conjugation between electron donor and acceptor group leads to delocalization of π electrons and hence positive charge over the two nitrogen atoms. The dye with 3 methine groups (n = 1 in formula 1) is classified as carbocyanine dyes or trimethine cyanine dyes. The wavelength of the cyanine dye absorption/emission depends on the nature of the end groups and the length of the polymethine chain. Monomethine and trimethine dyes are usually absorbed in the visible region (500–600 nm) of the electronic spectrum, and with each added methine unit (CH
CH) cause a bathochromic shift of about 100 nm in the electronic spectrum, resulting in absorption on the wavelength of 700–800 nm for penta- and heptamethine cyanines.15–23
Due to the importance of cyanine dyes, researchers paid a lot of attention to provide different synthetic methods for the preparation of these structures. For example, in 2019, Naimi-Jamal obtained trimethine oxonol dyes from the reaction of 1,3-dimethylbarbituric acid with vinamidinium salts (Scheme 1A).4 They also produced other trimethine oxonol dyes from the same salts with 1,3-indandione (Scheme 1B).24 Mazières isolated trimethine benzothiazole cyanine dyes using the orthoester approach (Scheme 1C).25
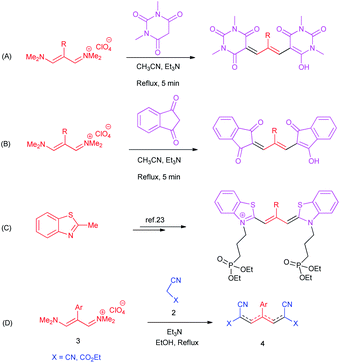 |
| Scheme 1 Representative condensation reactions towards cyanine dyes synthesis. | |
In continuation of our interest in the development of trimethinium salts in organic synthesis,26–30 herein we report a simple and highly efficient synthetic procedure for the preparation of new zwitterionic pyridinium-cyanopropenides from the reaction of trimethinium salts and malononitrile derivatives in the presence of triethylamine in ethanol as a green solvent in excellent yields without any by-products (Scheme 1D).
Results and discussion
The synthetic pathway for the synthesis of these new compounds is consisting of two steps. First, trimethinium salts 1 were prepared similar to the previous studies.26–33 Second, for the synthesis of novel zwitterionic pyridinium-cyanopropenides, trimethinium salts were isolated as perchlorate salts and reacted directly without additional purification were reacted with malononitrile derivatives in the presence of triethylamine in ethanol as a green solvent at reflux. Then, In order to optimize the reaction conditions for the synthesis of zwitterionic pyridinium-cyanopropenides derivatives, the model reaction between 1,3-bis(dimethylamino)-2-(isoquinolinium-2-yl)trimethinium bis(perchlorate) 3d and ethyl 2-cyanoacetate 2 was investigated in the presence of different bases and solvents. The results are summarized in Table 1. Different bases were evaluated in this study, such as NaH, Na2CO3, i-Pr2NEt, which lead to a low-yield product (Table 1, entries 1–3). Various solvents such as CH3CN, MeOH, and EtOH were studied, which CH3CN could not trigger this reaction (Table 1, entry 6), higher yield and shorter reaction time were obtained when the reaction was carried out in the presence of 1 mmol Et3N in ethanol as a solvent at reflux conditions (Table 1, entry 4). The control experiment confirmed that the reaction has not occurred under neutral and acid conditions (Table 1, entries 7 and 8).
Table 1 Optimization of the reaction conditions
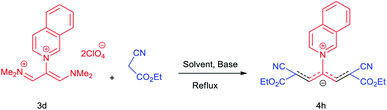
|
Entry |
Conditions |
Solvent |
Time (h) |
Yielda (%) |
Isolated yield. |
1 |
NaH |
EtOH |
5 |
40 |
2 |
Na2CO3 |
EtOH |
5 |
40 |
3 |
i-Pr2NEt |
EtOH |
5 |
80 |
4 |
Et3N |
EtOH |
5 |
95 |
5 |
Et3N |
MeOH |
24 |
25 |
6 |
Et3N |
CH3CN |
24 |
— |
7 |
— |
EtOH |
24 |
— |
8 |
AcOH |
EtOH |
24 |
— |
Then, the scope and efficiency of the process were explored under optimized conditions. For this purpose, 2-substituted trimethinium salts 3a–d were condensed with malononitrile or ethyl 2-cyanoacetate in the presence of Et3N (1 mmol) to produce corresponding products 4. The obtained products 4a–h are betaine derived from nitrogen-heteroaryl trimethinium salts 3a–d. “Betaines are zwitterionic compounds with a full negative and a full positive charge in the same molecule, according to trimethylammonio acetate, Me3N+–CH2–CO2−.33 The results are shown in Table 2.
Table 2 Synthesis of product 4 via the reaction of 2-substituted trimethinium salts 3 with malononitrile and ethyl cyanoacetate in the presence of Et3N in ethanol at reflux
The molecular structures of 4a–h were established by IR, UV/vis, mass, 1H and 13C NMR spectra as well as elemental analyses. The λmax values of 4a–h were determined in DMSO in the spectral range of 200–400 nm.
The proposed reaction mechanism of the formation of 4a–h in the presence of Et3N is shown in Scheme 2. In the initial step, the methylene group in structure 2 is deprotonated in the presence of Et3N, followed by intermediate A is formed by the nucleophilic attack of the carbanion group in malono to trimethinium salt 3. Then, removal of dimethylamine occurs and intermediate B is formed. In the next step, the nucleophilic attack of the second molecule of the carbanion group in malono on the obtained iminium salt C produce intermediate D. Finally, with the loss of the second molecule of dimethylamine and acidic hydrogen by base, the desired betaine products are created.
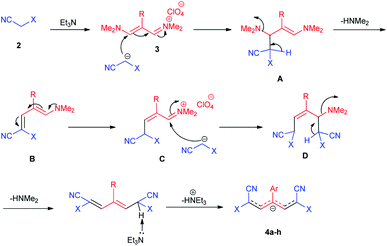 |
| Scheme 2 Proposed reaction mechanism for the synthesis of 4a–h. | |
Experimental
All chemicals were purchased from Merck or Fluka chemical companies. The 1H NMR (300 MHz) and 13C NMR spectra (75 MHz) were run on a Bruker Avance 400. Tetramethylsilane (TMS) was used as the internal standard for the NMR analysis. IR spectra were recorded using an FTIR apparatus. Melting points were recorded on a Stuart Scientific Apparatus SMP3 (UK) in open capillary tubes. Elemental C, H and N analyses, were performed using a Costech CHNS–O elemental analyzer. UV/vis absorption spectra were recorded at room temperature in DMSO using a PerkinElmer Lambda 25 spectrophotometer.
General procedure for the synthesis of pyridinium-cyanopropenides 4a–h
A solution of a 2-heteroraryl-substituted trimethinium salt 3a–d (1 mmol), malononitrile (2 mmol) or ethyl cyanoacetate (2 mmol) and triethylamine (1 mmol) in ethanol (10 mL) was heated under reflux for 5 h. Then, after cooling to room temperature, the precipitate formed was filtered off, washed with 2-propanol (3 × 3 mL), and dried under vacuum at 80 °C, to yield the desired betaine product 4a–h in excellent yields.
1,1,5,5-Tetracyano-3-(pyridinium-1-yl) penta propenides (4a)
Red powder, mp. > 260 °C, 1H NMR (DMSO-d6, 300 MHz), δ (ppm): 7.76 (s, 2H), 8.36 (t, J = 6.6 Hz, 2H), 8.81 (t, J = 7.6 Hz, 1H), 9.19 (d, J = 5.1 Hz, 2H). 13C NMR (DMSO-d6, 75 MHz), δ (ppm): 53.8, 114.4, 118.2, 120.9, 129.6, 148.9, 150.1, 150.2. Anal. calcd for C14H7N5: C, 68.57; H, 2.88; N, 28.56%. Found: C, 68.55; H, 2.85; N, 28.57%. λmax (DMSO) = 457 nm.
2,6-Dicyano-1,7-diethoxy-1,7-dioxo-4-(pyridinium-1-yl) penta-propenides (4b)
Orange powder, mp. > 260 °C, 1H NMR (DMSO-d6, 300 MHz), δ (ppm): 1.20 (t, J = 6.9 Hz, 6H), 4.13 (q, J = 6.9 Hz, 4H), 8.01 (s, 2H), 8.30 (t, J = 6.6 Hz, 2H), 8.75 (t, J = 7.5 Hz, 1H), 9.12 (d, J = 5.4 Hz, 2H). 13C NMR (DMSO-d6, 75 MHz) δ (ppm): 14.8, 60.7, 76.5, 116.7, 119.0, 129.2, 148.0, 153.1, 154.0, 165.5. Anal. calcd for C18H17N3O4: C, 68.71; H, 5.05; N, 12.38%. Found: C, 68.70; H, 5.05; N, 12.37%. λmax (DMSO) = 452 nm.
1,1,5,5-Tetracyano-3-(3,5-dimethylpyridinium-1-yl) penta-propenides (4c)
Violet powder, mp. > 260 °C, 1H NMR (DMSO-d6, 300 MHz), δ (ppm): 2.51 (s, 6H), 7.74 (s, 2H), 8.53 (s, 1H), 8.93 (s, 2H). 13C NMR (DMSO-d6, 75 MHz), δ (ppm): 18.2, 53.8, 114.5, 118.2, 120.9, 139.6, 146.2, 149.1, 149.9. Anal. calcd for C16H11N5: C, 70.32; H, 4.06; N, 25.63%. Found: C, 70.33; H, 4.08; N, 25.62%. λmax (DMSO) = 455 nm.
2,6-Dicyano-1,7-diethoxy-1,7-dioxo-4-(3,5-dimethylpyridinium-1-yl)-penta-propenides (4d)
Red powder, mp. > 260 °C, 1H NMR (DMSO-d6, 300 MHz), δ (ppm): 1.20 (t, J = 3.4 Hz, 6H), 2.51 (s, 6H), 4.11–4.16 (m, 4H), 7.99 (d, J = 3.6 Hz, 2H), 8.46 (s, 1H), 8.85 (s, 2H). 13C NMR (DMSO-d6, 75 MHz), δ (ppm): 14.8, 18.2, 60.6, 76.6, 116.7, 119.0, 139.1, 146.3, 147.7, 148.2, 165.5. Anal. calcd for C20H21N3O4: C, 65.38; H, 5.76; N, 11.44%. Found: C, 65.75; H, 5.77; N, 11.43%. λmax (DMSO) = 453 nm.
1-(1,1,5,5-Tetracyano-3-(quinolinium-1-yl)-penta-propenides) (4e)
Red powder, mp. > 260 °C, 1H NMR (DMSO-d6, 300 MHz), δ (ppm): 7.97 (s, 2H), 8.12 (t, J = 7.5 Hz, 1H), 8.20 (d, J = 8.7 Hz, 1H), 8.32–8.40 (m, 2H), 8.60 (d, J = 7.8 Hz, 1H), 9.54 (d, J = 8.1 Hz, 1H), 9.65 (dd, J = 1.2, 5.7 Hz, 1H). 13C NMR (DMSO-d6, 75 MHz), δ (ppm): 53.9, 114.5, 116.6, 118.1, 119.1, 123.0, 130.1, 131.2, 131.7, 138.1, 140.8, 151.0, 151.1, 153.9. Anal. calcd for C18H9N5: C, 73.21; H, 3.07; N, 23.72%. Found: C, 73.22; H, 3.05; N, 23.74%. λmax (DMSO) = 450 nm.
2,6-Dicyano-1,7-diethoxy-1,7-dioxo-4-(quinolinium-1-yl) penta-propenides (4f)
Red powder, mp. > 260 °C, 1H NMR (DMSO-d6, 300 MHz), δ (ppm): 1.16 (t, J = 6.9 Hz, 6H), 4.10 (q, J = 6.9 Hz, 4H), 8.07–8.12 (m, 2H), 8.21–8.34 (m, 4H), 8.57 (d, J = 7.8 Hz, 1H), 9.47 (d, J = 8.1 Hz, 1H), 9.58 (d, J = 5.4 Hz, 1H). 13C NMR (DMSO-d6, 75 MHz), δ (ppm): 14,8, 60.7, 76.7, 114.6, 116.8, 119.4, 122.9, 130.2, 130.7 131.5, 137.4, 141.2, 148.5, 150.2, 153.7, 165.4. Anal. calcd for C22H19N3O4: C, 67.86; H, 4.92; N, 10.79%. Found: C, 67.85; H, 4.90; N, 10.80%. λmax (DMSO) = 455 nm.
1,1,5,5-Tetracyano-3-(isoquinolinium-2-yl) penta-propenides (4g)
Red powder, mp. > 260 °C, 1H NMR (DMSO-d6, 300 MHz), δ (ppm): 7.86 (s, 2H), 8.10 (t, J = 7.5 Hz, 1H), 8.31 (t, J = 7.6 Hz, 1H), 8.41 (d, J = 8.1, 1H), 8.58 (d, J = 8.1 Hz, 1H), 8.78 (d, J = 6.6 Hz, 1H), 8.85 (dd, J = 0.9, 6.6 Hz, 1H), 10.28 (s, 1H). 13C NMR (DMSO-d6, 75 MHz), δ (ppm): 53.9, 114.7, 118.3, 121.1, 127.4, 127.9, 128.1, 131.6, 132.1, 138.7, 138.8, 139.7, 150.3, 155.1. υmax (kBr): 2224, 1611, 1541, 1248, 1056 cm−1. Anal. calcd for C18H9N5: C, 73.21; H, 3.07; N, 23.72%. Found: C, 73.22; H, 3.05; N, 23.74%. λmax (DMSO) = 450 nm.
2,6-Dicyano-1,7-diethoxy-4-(isoquinolinium-2-yl)-1,7-dioxo-penta-propenides (4h)
Red powder, mp. > 260 °C, 1H NMR (DMSO-d6, 300 MHz), δ (ppm): 1.18 (t, J = 6.9 Hz, 6H), 4.13 (q, J = 6.9 Hz, 4H), 8.06–8.15 (m, 3H), 8.29 (t, J = 7.2 Hz, 1H), 8.41 (d, J = 8.1 Hz, 1H), 8.57 (d, J = 8.1 Hz, 1H), 8.71–8.80 (m, 2H), 10.22 (s, 1H). 13C NMR (DMSO-d6, 75 MHz), δ (ppm): 14.8, 60.7, 76.7, 116.9, 119.2, 127.1, 127.8, 128.3, 131.4, 131.7, 138.1, 138.5, 140.1, 148.2, 154.9, 165.5. Anal. calcd for C22H19N3O4: C, 67.86; H, 4.92; N, 10.79%. Found: C, 67.85; H, 4.90; N, 10.80%. λmax (DMSO) = 455 nm.
Conclusions
In conclusion, we report on a highly efficient, one-pot method for the synthesis of new zwitterionic pyridinium-cyanopropenides by reaction of 2-heteroaryl-substituted trimethinium salts with malononitrile or ethyl cyanoacetate in ethanol solution under reflux.
A simple procedure with excellent yields, mild reaction conditions, easy purification of the products, and absence of by-products, are the main advantages of this method.
Conflicts of interest
There are no conflicts to declare.
Acknowledgements
The authors thank Persian Gulf University Research Councils for the financial support of this work.
References
- C. Reichardt, Chiral polymethine dyes: a remarkable but forgotten conjugated π system, J. Phys. Org. Chem., 1995, 8, 761, DOI:10.1002/poc.610081202.
- S. Dähne and D. Leupold, Kopplungsprinzipien organischer Farbstoffe, Angew. Chem., 1966, 78, 1029, DOI:10.1002/ange.19660782302.
- A. M. Mehranpour, S. Hashemnia and F. Azamifar, Synthesis and Characterization of γ-Heteroaryl-substituted Pentamethine Cyanine Dyes with Carboxy or Methoxycarbonyl Substituents at the Two Heterocyclic End Groups, J. Heterocycl. Chem., 2014, 51, 1457, DOI:10.1002/jhet.1816.
- F. Azamifara, M. R. Naimi-Jamal and O. M. Demchuk, Design, synthesis and characterization of new trimethine oxonol dyes from 1,3-indandione and 2-substituted vinamidinium salts, Dyes Pigm., 2019, 161, 438, DOI:10.1016/j.dyepig.2018.09.043.
- D. Komljenovic, M. Wiessler, W. Waldeck, V. Ehemann, R. Pipkorn, H. Shrenk, J. Debus and K. Braun, NIR-Cyanine Dye Linker: a Promising Candidate for Isochronic Fluorescence Imaging in Molecular Cancer Diagnostics and Therapy Monitoring, Theranostics, 2016, 6, 131, DOI:10.7150/thno.11460.
- M. Kawakami, K. Koya, T. Ukai, N. Tatsuta, A. Ikegawa and K. Ogawa, Structure-activity of novel rhodacyanine dyes as antitumor agents, J. Med. Chem., 1998, 41, 130, DOI:10.1021/jm970590k.
- E. Soriano, C. Holder, A. Levitz and M. Henary, Benz [c,d] indolium-containing monomethine cyanine dyes: snthesis and photophysical properties, Molecules, 2016, 21, 23, DOI:10.3390/molecules21010023.
- H. Chen, H. Sun, X. Zhang, X. Sun, Y. Shi, S. Xu and Y. Tang, A colorimetric and fluorometric dual-modal DNA logic gate based on the response of a cyanine dye supramolecule to G-quadruplexes, New J. Chem., 2016, 40, 1940, 10.1039/c5nj02652k.
- D. E. Lynch, M. Z. H. Chowdhury, N.-L. Luu, E. S. Wane, J. Heptinstall and M. J. Cox, Water soluble bis(indolenine)squaraine salts for use as fluorescent protein-sensitive probes, Dyes Pigm., 2013, 96, 116, DOI:10.1016/j.dyepig.2012.07.015.
- A. M. Lopez, E. Valero and M. Bradley, Synthesis and optimization of a reactive oxygen species responsive cellular delivery system, New J. Chem., 2017, 41, 2392, 10.1039/c6nj02985j.
- H. A. Shindy, Fundamentals in the Chemistry of Cyanine Dyes: A Review, Dyes Pigm., 2017, 145, 505, DOI:10.1016/j.dyepig.2017.06.029.
- M. E. Jung and W.-J. Kim, Practical syntheses of dyes for difference gel electrophoresis, Bioorg. Med. Chem., 2006, 14, 92, DOI:10.1016/j.bmc.2005.07.068.
- S. M. Usama, C.-M. Lin and K. Burgess, On the Mechanisms of Uptake of Tumor-Seeking Cyanine Dyes, Bioconjug Chem, 2018, 29, 3886, DOI:10.1021/acs.bioconjchem.8b00708.
- S. R. Mujumdar, R. B. Mujumdar, C. M. Grant and A. S. Waggoner, Cyanine-Labeling Reagents: Sulfobenzindocyanine Succinimidyl Esters, Bioconjugate Chem., 1996, 7, 356, DOI:10.1021/bc960021b.
- M. Mojzych and M. Henary, Synthesis of Cyanine Dyes, Top. Heterocycl. Chem., 2008, 14, 1, DOI:10.1007/7081_2008_119.
- A. Mishra, R. K. Behera, P. K. Behera, B. K. Mishra and G. B. Behera, Cyanines during the 1990s: a review, Chem. Rev., 2000, 100, 1973, DOI:10.1021/cr990402.
- G. B. Behera, P. K. Behera and B. K. Mishra, Cyanine dyes: self-aggregation and behaviour in surfactants a review, J. Surf. Sci. Technol., 2007, 23, 1, DOI:10.18311/jsst/2007/1958.
- M. Henary, S. Paranjpe and E. A. Owens, Synthesis and applications of benzothiazole containing cyanine dyes, Heterocycl. Commun., 2013, 19, 1, DOI:10.1515/hc-2013-0012.
- V. Buß, D. Ulbrich and C. Reichardt, Chiral Tri- and Pentamethinium Cyanine Dyes with 1,2,3,4-Tetrahydro-6-methylquinolyl
End Groups: UV/Vis and CD Spectroscopy and Structure Correlations, Liebigs Ann., 1996, 1996, 1823, DOI:10.1002/jlac.199619961116.
- R. M. El-Shishtawy and P. Almeida, A new Vilsmeier-type reaction for one-pot synthesis of pH sensitive fluorescent cyanine dyes, Tetrahedron, 2006, 62, 7793 CrossRef CAS.
- Y. Sato, S. Yajima, A. Taguchi, K. Baba, M. Nakagomi, Y. Aiba and S. Nishizawa, Trimethine cyanine dyes as deep-red fluorescent indicators with high selectivity to the internal loop of the bacterial A-site RNA, Chem. Commun., 2019, 55, 3183, 10.1039/c9cc00414a.
- Y. Gu, X. Fei, Y. Liu, Y. Wang and X. Yang, Trimethine cyanine dyes with an indole nucleus: synthesis and spectral properties studies, J. Lumin., 2013, 134, 184, DOI:10.1016/j.jlumin.2012.08.050.
- J. Gu, U. R. Anumala, R. Heyny-von Haußen, J. Hölzer, V. Goetschy-Meyer, G. Mall, I. Hilger, C. Czech and B. Schmidt, Design, Synthesis and Biological Evaluation of Trimethine Cyanine Dyes as Fluorescent Probes for the Detection of Tau Fibrils in Alzheimer's Disease Brain and Olfactory Epithelium, ChemMedChem, 2013, 8, 891, DOI:10.1002/cmdc.201300090.
- F. Azamifar, M. R. Naimi-Jamal, A. Rineh and M. J. Kelso, Synthesis, structural/photophysical characterization and theoretical investigations with new β-pyridinium/quinolinium and β-bromine substituted bis(1,3-dimethylbarbituric acid) trimethine oxonol dyes that display large Stokes shifts, Dyes Pigm., 2020, 172, 107758, DOI:10.1016/j.dyepig.2019.107758.
- M. R. Mazières, C. Duprat, J. Bellan and J. G. Wolf, Synthesis and characterisation of new phosphonate labelled cyanines, Dyes Pigm., 2007, 74, 404, DOI:10.1016/j.dyepig.2006.03.003.
- Z. Rafiee Samani and A. M. Mehranpour, Synthesis of new allylidene amino phenol-containing Schiff bases and metal complex formation using trimethinium salts, RSC Adv., 2021, 11, 21695, 10.1039/d1ra04214a.
- N. Golzar, A. M. Mehranpour and N. Nowrouzi, A facile and efficient route to one-pot synthesis of new cyclophanes using vinamidinium salts, RSC Adv., 2021, 11, 13666, 10.1039/d0ra10548a.
- Z. Rafiee Samani and A. M. Mehranpour, Synthesis of novel 5-substituted isophthalates from vinamidinium salts, Tetrahedron Lett., 2019, 60, 151002, DOI:10.1016/j.tetlet.2019.151002.
- A. M. Mehranpour and M. Zahiri, Synthesis and characterization of new benzimidazole derivatives using 2-substituted 1,3-bis(dimethylamino)-trimethinium salts, Tetrahedron Lett., 2014, 55, 3969, DOI:10.1016/j.tetlet.2014.05.038.
- A. M. Mehranpour, S. Hashemnia and F. Azamifar, Synthesis of new malonaldehyde derivatives using 2-heteroaryl-substituted trimethinium salts, Tetrahedron Lett., 2013, 54, 321, DOI:10.1016/j.tetlet.2012.11.046.
- A. M. Mehranpour, S. Hashemnia and R. Maghamifar, Synthesis and Characterization of New γ-Substituted Pentamethine Cyanine Dyes, Synth. Commun., 2010, 40, 3594, DOI:10.1080/00397910903457290.
- A. M. Mehranpour, S. Hashemnia and Z. Shayan, Synthesis and characterization of new derivatives of 1,4-diazepinium salts, Synth. Commun., 2011, 41, 3501, DOI:10.1080/00397911.2010.518332.
- T. H. H. Chen and N. Murata, Enhancement of tolerance of abiotic stress by metabolic engineering of betaines and other compatible solutes, Curr. Opin. Plant Biol., 2002, 5, 250, DOI:10.1016/s1369-5266(02)00255-8.
|
This journal is © The Royal Society of Chemistry 2022 |
Click here to see how this site uses Cookies. View our privacy policy here.