DOI:
10.1039/D2RA02314H
(Paper)
RSC Adv., 2022,
12, 19265-19269
Cs2CO3 catalyzed direct aza-Michael addition of azoles to α,β-unsaturated malonates†
Received
10th April 2022
, Accepted 27th June 2022
First published on 1st July 2022
Abstract
A highly efficient method for the synthesis of azole derivatives via a direct aza-Michael addition of azoles to α,β-unsaturated malonates using Cs2CO3 as a catalyst, has been successfully developed. A series of azole derivatives have been obtained in up to 94% yield and the reaction could be amplified to gram scale in excellent yield in the presence of 10 mol% of Cs2CO3.
Introduction
Azoles and their derivatives are important heterocyclic scaffolds which have been widely found in many natural products, bioactive compounds, and drug candidates.1,2 Particularly, the pyrazole constitutes the structural core featured in numerous pharmacologically active molecules.3 For example, the β-pyrazolyl acid A has activity toward human GPR40 G-protein coupled receptor (Fig. 1).4 A prominent example is the Janus kinase (JAK) inhibitor Ruxolitinib (INCB018424), which has been used in the treatment of myelofibrosis (Fig. 1).5 Therefore, in the past two decades, continuous efforts have been directed towards the development of efficient methods for accessing such pyrazole structures in medicinal chemistry and organic synthesis.6–11 To date, numerous concise and robust synthetic methods, mainly including N-nucleophilic substitutions,6 C–N cross-couplings7,8 and aza-Michael additions,9,10 have been established. Among them, the direct aza-Michael addition of pyrazole has attracted more attention as a highly efficient method for construction of pyrazole derivatives.10,11
 |
| Fig. 1 Biologically pyrazole compounds. | |
As we all know, the pyrazoles via N-deprotonation generating active N-nucleophiles under base-catalysis,12 could react with all kinds of Michael receptors to afford pyrazole derivatives. These Michael receptors in aza-Michael addition of pyrazole mainly include methyl acrylate,10c,d,j,k acrylonitrile,10c,j β,γ-unsaturated-α-keto esters,10f nitroalkenes,10e α,β-unsaturated ketones10a–c or imides10i and maleic or crotonic acid10g,h (Scheme 1a). Specially, several catalytic asymmetric aza-Michael additions of pyrazoles had been successfully realized in which the optically active pyrazole derivatives were obtained.11 Nevertheless, the development of alternative receptor in aza-Michael addition of azole will be remain as a highly desirable work, owing to their easy accessing other valuable pyrazole derivatives. To the best of our knowledge, the α,β-unsaturated malonates, which had been used as Michael receptors in numerous transformations, had their potential in the construction of azole derivatives via direct aza-Michael addition of azoles.13 Herein, we describe a Cs2CO3 catalyzed direct aza-Michael addition of azoles 2 to α,β-unsaturated malonates 1 to afford azole derivatives 3 (Scheme 1b).
 |
| Scheme 1 Commonly encountered aza-Michael addition of azoles. | |
Results and discussion
In the initial study, dimethyl 2-benzylidenemalonate 1a and pyrazole 2a were chosen as the model substrates for the synthesis of pyrazole derivatives via the direct aza-Michael addition. No product was observed without catalyst when stirring in THF at 25 °C for 24 h (Table 1, entry 1). Next, various bases as catalysts were surveyed in THF at 25 °C and trace amount of product 3aa was observed in the presence of 100 mol% of organic base Et3N (Table 1, entry 2). Meanwhile, DBU could afford pyrazole derivative 3aa in lower yield (31%, Table 1, entry 3). When the reaction was performed with 100 mol% of inorganic bases, the acceptable yields of 3aa were obtained (Table 1, entries 4–7). Comparatively, the Cs2CO3 exhibited a slight superiority in reactivity toward this aza-Michael addition compared with LiOH·H2O, K3PO4·7H2O, and K2CO3 (Table 1, entries 6 vs. 4, 5 and 7). Further optimization of the reaction conditions was then aimed at exploring the efficiency of solvent. Unfortunately, the yield of 3aa decreased slightly in other types of solvents (CH3OH, PhCH3, EtOAc, CH2Cl2, Table 1, entries 6 vs. 8–11), and the THF was still the most suitable solvent for this reaction. The efficiency of temperature was also examined (Table 1, entries 6 and 12–13), and it was found that increasing the temperature to 40 °C had nearly no effect on the yield of 3aa (Table 1, entry 12) but the yield of 3aa decreased when reducing the temperature to 0 °C (Table 1, entry 13). Increasing the amount of pyrazole 2a to 0.3 mmol could further improve the yield of 3aa to 80% (Table 1, entry 14). We were delighted to find that reducing the amount of Cs2CO3 to 10 mol% had no effect on the yield of 3aa (Table 1, entry 15), while the yield of 3aa decreased significantly when reducing the amount of Cs2CO3 to 1 mol% (Table 1, entry 16). Reducing the amount of solvent THF to 0.20 mL, the yield of 3aa increased slightly (Table 1, entry 17). The reaction was amplified to 0.50 mmol scale and also proceeded smoothly, affording 3a in 84% yield (Table 1, entry 18). Therefore, the optimal conditions were identified as 10 mol% of Cs2CO3 in THF at 25 °C for 24 h.
Table 1 Optimization of the reaction conditionsa

|
Entry |
Base |
Solvent |
T (°C) |
Yieldb (%) |
Reaction conditions: 1a (0.20 mmol), 2a (0.20 mmol), base (100 mol%), solvent (1.0 mL), 24 h. Isolated yield. 0.30 mmol of 2a was used. 10 mol% of Cs2CO3 was used. 1 mol% of Cs2CO3 was used. 0.2 mL of THF was used. 1a (0.50 mmol), 2a (0.75 mmol), Cs2CO3 (10 mol%), THF (0.5 mL), 24 h. |
1 |
— |
THF |
25 |
0 |
2 |
Et3N |
THF |
25 |
Trace |
3 |
DBU |
THF |
25 |
31 |
4 |
LiOH·H2O |
THF |
25 |
60 |
5 |
K3PO4·7H2O |
THF |
25 |
58 |
6 |
Cs2CO3 |
THF |
25 |
69 |
7 |
K2CO3 |
THF |
25 |
53 |
8 |
Cs2CO3 |
CH3OH |
25 |
— |
9 |
Cs2CO3 |
PhCH3 |
25 |
62 |
10 |
Cs2CO3 |
EtOAc |
25 |
48 |
11 |
Cs2CO3 |
CH2Cl2 |
25 |
61 |
12 |
Cs2CO3 |
THF |
40 |
67 |
13 |
Cs2CO3 |
THF |
0 |
50 |
14c |
Cs2CO3 |
THF |
25 |
80 |
15c,d |
Cs2CO3 |
THF |
25 |
79 |
16c,e |
Cs2CO3 |
THF |
25 |
55 |
17c,e,f |
Cs2CO3 |
THF |
25 |
83 |
18g |
Cs2CO3 |
THF |
25 |
84 |
Under the optimal conditions (Table 1, entry 17), various α,β-unsaturated malonates 1 were evaluated, affording the corresponding pyrazole derivatives 3 in moderate to excellent yields (up to 92%). As shown in Table 2, the reactivity of this direct aza-Michael addition was sensitive to the steric hindrance on the ester group of α,β-unsaturated malonates 1. The substrates 1 containing bulkier ester groups (–CO2Et, –CO2iPr, and –CO2tBu) gave lower yields than its with –CO2Me group (Table 2, entries 2–4 vs. 1). For the effects of substituents in the phenyl ring, the reactivity of the direct aza-Michael addition was sensitive to the steric hindrance rather than to the electronic property of α,β-unsaturated malonates 1. The substrates 1 with ortho-substituents gave lower yields than those with para or meta ones (Table 2, entries 7 vs. 5 and 6, 10 vs. 8 and 9, 13 vs. 11 and 12, 17 vs. 15 and 16, 20 vs. 18 and 19). The substrates with 2-F, 2-Cl, 2-Br, 2-Me or 2-OMe substituents on phenyl ring (1g, 1j, 1m, 1q and 1t) were transformed into pyrazole derivatives 3ga, 3ja, 3ma, 3qa and 3ja in moderate yields (Table 2, entries 7, 10, 13, 17 and 20). Meanwhile, the fused-ring substrates (1u and 1v) were also tolerable, giving the desired products with 75% and 88% yields, respectively (Table 2, entries 21 and 22). For the thienyl heteroaromatic substrates 1w and 1x, the reaction generated the desired products 3wa and 3xa in 84% and 88% yield (Table 2, entries 23 and 24), while the 2-furyl heteroaromatic substrate 1y afforded the desired product 3ya in 76% yield (Table 2, entry 25). At the same time, the alkyl substituted substrates 1z, 1α, 1β and 1γ also gave the corresponding pyrazole derivatives 3za, 3αa, 3βa and 3γa in good yields (60–92%, Table 2, entries 26–29).
Table 2 Substrate scope of α,β-unsaturated malonatesa

|
Entry |
R1 |
R2 |
3 |
Yieldb (%) |
Reaction conditions: 1 (0.50 mmol), 2 (0.75 mmol), Cs2CO3 (10 mol%), THF (0.5 mL), 25 °C, 24 h. Isolated yield. |
1 |
Ph |
Me |
3aa |
84 |
2 |
Ph |
Et |
3ba |
68 |
3 |
Ph |
iPr |
3ca |
67 |
4 |
Ph |
tBu |
3da |
69 |
5 |
4-FC6H4 |
Me |
3ea |
84 |
6 |
3-FC6H4 |
Me |
3fa |
73 |
7 |
2-FC6H4 |
Me |
3ga |
66 |
8 |
4-ClC6H4 |
Me |
3ha |
92 |
9 |
3-ClC6H4 |
Me |
3ia |
87 |
10 |
2-ClC6H4 |
Me |
3ja |
64 |
11 |
4-BrC6H4 |
Me |
3ka |
74 |
12 |
3-BrC6H4 |
Me |
3la |
71 |
13 |
2-BrC6H4 |
Me |
3ma |
52 |
14 |
4-F3CC6H4 |
Me |
3na |
81 |
15 |
4-MeC6H4 |
Me |
3oa |
63 |
16 |
3-MeC6H4 |
Me |
3pa |
91 |
17 |
2-MeC6H4 |
Me |
3qa |
55 |
18 |
4-MeOC6H4 |
Me |
3ra |
77 |
19 |
3-MeOC6H4 |
Me |
3sa |
87 |
20 |
2-MeOC6H4 |
Me |
3ta |
65 |
21 |
2-Naphthyl |
Me |
3ua |
75 |
22 |
1-Naphthyl |
Me |
3va |
88 |
23 |
3-Thienyl |
Me |
3wa |
84 |
24 |
2-Thienyl |
Me |
3xa |
81 |
25 |
2-Furyl |
Me |
3ya |
76 |
26 |
nPr |
Me |
3za |
85 |
27 |
iPr |
Me |
3αa |
92 |
28 |
iBu |
Me |
3βa |
60 |
29 |
nC9H19 |
Me |
3γa |
74 |
Next, the use of this catalytic system for aza-Michael addition of a variety of substituted pyrazoles 2 was explored, and the desired pyrazole derivatives 3 were obtained in moderate to excellent yields (up to 94%). As shown in Table 3, the electronic nature of the substituents in pyrazoles 2 had obvious effect on the efficiency of this reaction (Table 3, 3ab–3af). The substrates 2 with electron-donating Me group gave higher yields than those with electron-withdrawing (Cl or Br) substituents (Table 3, 3ae, 3af vs. 3ab, 3ac and 3ad). For indazole substrate 2g, the aza-Michael addition generated the desired product 3ag in 52% yield (Table 3, entry 7).14
Table 3 Substrate scope of azoles.a,b
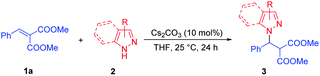
|
Reaction conditions: 1 (0.50 mmol), 2 (0.75 mmol), Cs2CO3 (10 mol%), THF (0.5 mL), 25 °C, 24 h. Isolated yield. |
 |
Then, the use of this catalytic system for the direct aza-Michael addition of triazoles 2 to dimethyl 2-benzylidenemalonate 1a was explored, and the desired N1-substituted triazole derivative 3ah was obtained in 71% yield for the 1,2,4-triazole 2h, while the N2-substituted triazole derivative 3ai was obtained in 61% yield for the 1,2,3-triazole 2i (Scheme 2). For the substrate 1H-benzotriazole 2j, the reaction generated triazole derivatives 3aj and 3aj′ in 57% and 18% yields, simultaneously (3aj/3aj′ = 3.2/1, based on the isolated yields, Scheme 3) under the optimal conditions.15 Besides, the direct aza-Michael additions of imidazole and pyrrole to dimethyl 2-benzylidene-malonate 1a were also explored, unfortunately, no desired products were observed under the optimal conditions.
 |
| Scheme 2 Direct aza-Michael addition of triazoles 2h and 2i to dimethyl 2-benzylidenemalonate 1a. | |
 |
| Scheme 3 Direct aza-Michael addition of benzotriazole 2j to dimethyl 2-benzylidenemalonate 1a. | |
On account of the synthetic potential of this method, the reaction was amplified to gram scale. As shown in Scheme 4, the direct aza-Michael addition of pyrazole 2a (1.02 g, 15.0 mmol) to methyl dimethyl 2-benzylidenemalonate 1a (2.20 g, 10.0 mmol) proceeded smoothly under the optimal conditions, affording the pyrazole derivative 3aa in 75% yield (Scheme 4a). Delightly, the yield of 3aa could be improved to 94% when the reaction concentration was increased twice as much in the gram scale synthesis (Scheme 4b).
 |
| Scheme 4 Preparative scale synthesises of selected compound. | |
According to the previous studies on the reactive properties of azoles in literatures,9,12 a reasonable catalytic cycle was proposed in Fig. 2. Because the pKa value of N1–H in azole is less that of H2CO3 [pKa (N1–H) = 2.49, pKa1 (H2CO3) = 6.37], the N1-deprotonation of azoles 2 could be promoted by the conjugated base CO32−, which had been from the ionization of Cs2CO3. First, the active N-nucleophiles I and HCO3− were generated via the N1-deprotonation of azoles 2. Then the N-nucleophiles I attacked the α,β-unsaturated malonates 1 at β-positions, forming the enolate intermediates II. Next, the HCO3− transferred the H+ to the enolate oxygen of intermediates II due to that the pKa value of HCO3− is less than that of enolates, providing the enol type azole derivatives 3′. Meanwhile, the CO32− could regenerate and participate in the next round of catalytic cycle. Finaly, the azole derivatives 3 were obtained via the tautomerism of the enol type azole derivatives 3′.
 |
| Fig. 2 Proposed catalytic cycle. | |
Conclusions
We have developed a highly efficient method for the synthesis of azole derivatives via a direct aza-Michael addition of azoles to α,β-unsaturated malonates using Cs2CO3 as catalyst. A series of azole derivatives (38 examples) have been obtained in up to 94% yield. The reaction could be amplified to gram scale in excellent yield (94%) in the presence of 10 mol% of Cs2CO3, which had shown the potential value of the catalytic system for practical synthesis. Further study on an enantioselective version of this direct aza-Michael addition is still in progress.
Conflicts of interest
There are no conflicts to declare.
Acknowledgements
We are grateful to the National Natural Science Foundation of China (No. 22001219), the Natural Science Foundation of Sichuan Province (No. 2022NSFSC1189) and the Fundamental Research Funds for the Central Universities, Southwest Minzu University (No. 2021PTJS25) for financial supports.
Notes and references
- For selected reviews, see:
(a) S. D. Roughley and A. M. Jordan, J. Med. Chem., 2011, 54, 3451 CrossRef CAS PubMed;
(b) E. Vitaku, D. T. Smith and J. T. Njardarson, J. Med. Chem., 2015, 57, 10257 CrossRef;
(c) R. J. D. Hatley, S. J. F. Macdonald, R. J. Slack, J. Le, S. B. Ludbrook and P. T. Lukey, Angew. Chem., Int. Ed., 2018, 57, 3298 CrossRef CAS PubMed.
-
(a) D. Patel, M. Jain, S. R. Shah, R. Bahekar, P. Jadav, B. Darji, Y. Siriki, D. Bandyopadhyay, A. Joharapurkar, S. Kshirsagar, H. Patel, M. Shaikh, K. V. V. M. Sairam and P. Patel, ChemMedChem, 2011, 6, 1011 CrossRef CAS PubMed;
(b) G. Venkatesan, P. Paira, S. L. Cheong, K. Vamsikrishna, S. Federico, K.-N. Klotz, G. Spalluto and G. Pastorin, Bioorg. Med. Chem., 2014, 22, 1751 CrossRef CAS PubMed;
(c) M. Xin, X. Zhao, W. Huang, Q. Jin, G. Wu, Y. Wang, F. Tang and H. Xiang, Bioorg. Med. Chem., 2015, 23, 6250 CrossRef CAS PubMed;
(d) G. Venkatesan, P. Paira, S. L. Cheong, S. Federico, K. N. Klotz, G. Spalluto and G. Pastorin, Eur. J. Med. Chem., 2015, 92, 784 CrossRef CAS;
(e) Z. S. Cheruvallath, S. L. Gwaltney, M. Sabat, M. Tang, H. Wang, A. Jennings, D. Hosfield, B. Lee, Y. Wu, P. Halkowycz and C. E. Grimshaw, Bioorg. Med. Chem. Lett., 2017, 27, 2678 CrossRef CAS PubMed;
(f) M. Dawidowski, V. C. Kalel, V. Napolitano, R. Fino, K. Schorpp, L. Emmanouilidis, D. Lenhart, M. Ostertag, M. Kaiser, M. Kolonko, B. Tippler, W. Schliebs, G. Dubin, P. Mäser, I. Tetko, K. Hadian, O. Plettenburg, R. Erdmann, M. Sattler and G. M. Popowicz, J. Med. Chem., 2020, 63, 847 CrossRef CAS PubMed.
- For selected reports, see:
(a) J. J. Cui, M. Tran-Dube, H. Shen, M. Nambu, P.-P. Kung, M. Pairish, L. Jia, J. Meng, L. Funk, I. Botrous, M. McTigue, N. Grodsky, K. Ryan, E. Padrique, G. Alton, S. Timofeevski, Y. S. amazaki, Q. Li, H. Zou and J. Christensen, J. Med. Chem., 2011, 54, 6342 CrossRef CAS PubMed;
(b) M. Andrés, M. Bravo, M. A. Buil, M. Calbet, J. Castro, T. Domènech, P. Eichhorn, M. Ferrer, E. Gómez, M. D. Lehner, I. Moreno, R. S. Roberts and S. Sevilla, Bioorg. Med. Chem. Lett., 2013, 23, 3349 CrossRef PubMed;
(c) S. Fuse, T. Morita, K. Johmoto, H. Uekusa and H. Tanaka, Chem.–Eur. J., 2015, 21, 14370 CrossRef CAS PubMed;
(d) B. Gopula, Y.-F. Tsai, T.-S. Kuo, P.-Y. Wu, J. P. Henschke and H.-L. Wu, Org. Lett., 2015, 17, 1142 CrossRef CAS PubMed;
(e) S. Patel, S. F. Harris, P. Gibbons, G. Deshmukh, A. Gustafson, T. Kellar, H. Lin, X. Liu, Y. Liu, Y. Liu, C. Ma, K. Scearce-Levie, A. S. Ghosh, Y. G. Shin, H. Solanoy, J. Wang, B. Wang, J. Yin, M. Siu and J. W. Lewcock, J. Med. Chem., 2015, 58, 8182 CrossRef CAS PubMed;
(f) F. A. Romero, J. M. Murray, K. W. Lai, V. Tsui, B. K. Albrecht, L. An, M. H. Beresini, G. de L. Boenig, S. M. Bronner, E. W. Chan, K. X. Chen, Z. Chen, E. F. Choo, K. Clagg, K. Clark, T. D. Crawford, P. Cyr, D. D. A. Nagata, K. E. Gascoigne, J. L. Grogan, G. Hatzivassiliou, W. Huang, T. L. Hunsaker, S. Kaufman, S. G. Koenig, R. Li, Y. Li, X. Liang, J. Liao, W. Ly, J. Q. Liu, J. Maher, C. Masui, M. Merchant, Y. Ran, A. M. Taylor, J. S. Wai, F. Wang, X. Wei, D. Yu, B.-Y. Zhu, X. Zhu and S. R. Magnuson, J. Med. Chem., 2017, 60, 9162 CrossRef CAS PubMed;
(g) S. Varghese, R. Rahmani, S. Russell, G. S. Deora, L. Ferrins, A. Toynton, A. Jones, M. Sykes, A. Kessler, A. Eufrasio, A. T. Cordeiro, J. Sherman, A. Rodriguez, V. M. Avery, M. Piggott and J. B. Baell, ACS Med. Chem. Lett., 2020, 11, 278 CrossRef CAS PubMed.
- S. P. Brown, P. Dransfield, J. B. Houze, J. Liu, J. Liu, Z. Ma, J. C. Medina, V. Pattaropond, M. J. Schmitt, R. Sharma and Y. Wang, US Pat. 7687526B2, 2010.
- R. A. Mesa, U. Yasothan and P. Kirkpatrick, Nat. Rev. Drug Discovery, 2012, 11, 103 CrossRef CAS PubMed.
- For selected reports, see:
(a) A. Huang, K. Wo, S. Y. C. Lee, N. Kneitschel, J. Chang, K. Zhu, T. Mello, L. Bancroft, N. Norman and S.-L. Zheng, J. Org. Chem., 2017, 82, 8864 CrossRef CAS;
(b) C. Pezzetta, D. Bonifazi and R. W. M. Davidson, Org. Lett., 2019, 21, 8957 CrossRef CAS PubMed;
(c) D. Xu, L. Frank, T. Nguyen, A. Stumpf, D. Russell, R. Angelaud and F. Gosselin, Synlett, 2020, 31, 595 CrossRef CAS.
- For selected reports, see:
(a) A. Correa and C. Bolm, Angew. Chem., Int. Ed., 2007, 46, 8862 CrossRef CAS PubMed;
(b) Y.-C. Teo, F.-F. Yong, C.-Y. Poh, Y.-K. Yana and G.-L. Chua, Chem. Commun., 2009, 6258 RSC;
(c) H. W. Lee, A. S. C. Chan and F. Y. Kwong, Tetrahedron Lett., 2009, 50, 5868 CrossRef CAS;
(d) Q. Yang, Y. Wang, D. Lin and M. Zhang, Tetrahedron Lett., 2013, 54, 1994 CrossRef CAS;
(e) A. M. Haydl, K. Xu and B. Breit, Angew. Chem., Int. Ed., 2015, 54, 7149 CrossRef CAS PubMed;
(f) F. Damkaci, A. Alawaed and E. Vik, Tetrahedron Lett., 2016, 57, 2197 CrossRef CAS;
(g) C. Yuan, Y. Zhao and L. Zheng, Synlett, 2019, 30, 2173 CrossRef CAS.
- For selected reports, see:
(a) M. L. Kantam, T. Ramani and L. Chakrapani, Synth. Commun., 2008, 38, 626 CrossRef CAS;
(b) Y.-C. Teo and G.-L. Chua, Chem.–Eur. J., 2009, 15, 3072 CrossRef CAS PubMed;
(c) Y.-S. Liu, Y. Liu, X.-W. Ma, P. Liu, J.-W. Xie and B. Dai, Chin. Chem. Lett., 2014, 25, 775 CrossRef CAS;
(d) T. Niwa, H. Ochiai, Y. Watanabe and T. Hosoya, J. Am. Chem. Soc., 2015, 137, 14313 CrossRef CAS PubMed;
(e) Q. Zhou, F. Du, Y. Chen, Y. Fu, W. Sun, Y. Wu and G. Chen, J. Org. Chem., 2019, 84, 8160 CrossRef CAS PubMed;
(f) A. Y. Jiu, H. S. Slocumb, C. S. Yeung, X.-H. Yang and V. M. Dong, Angew. Chem., Int. Ed., 2021, 60, 19660 CrossRef CAS PubMed.
- For selected review, see: M. G. Vinogradov, O. V. Turova and S. G. Zlotin, Org. Biomol. Chem., 2019, 17, 3670 RSC.
- For selected reports, see:
(a) L. Yang, L.-W. Xu, W. Zhou, L. Li and C.-G. Xia, Tetrahedron Lett., 2006, 47, 7723 CrossRef CAS;
(b) Y.-J. Wu, Tetrahedron Lett., 2006, 47, 8459 CrossRef CAS;
(c) J.-M. Xu, C. Qian, B.-K. Liu, Q. Wu and X.-F. Lin, Tetrahedron, 2007, 63, 986 CrossRef CAS;
(d) C. Qian, J.-M. Xu, Q. Wu, D.-S. Lv and X.-F. Lin, Tetrahedron Lett., 2007, 48, 6100 CrossRef CAS;
(e) Y. Wu, J. Wang, P. Li and F. Y. Kwong, Synlett, 2012, 23, 788 CrossRef CAS;
(f) J. Wang, P.-F. Li, S. H. Chan, A. S. C. Chan and F. Y. Kwong, Tetrahedron Lett., 2012, 53, 2887 CrossRef CAS;
(g) H. N. Khachatryana, S. S. Hayotsyana, K. S. Badalyana, H. S. Attaryana and G. V. Hasratyan, Russ. J. Gen. Chem., 2015, 85, 1982 CrossRef;
(h) H. N. Khachatryan, Russ. J. Gen. Chem., 2017, 87, 572 CrossRef CAS;
(i) H. Zhou, X. Xiang, B. Ma, G. Wang, Z. Zhang and J. Yang, Synthesis, 2019, 51, 3142 CrossRef CAS;
(j) K. Kodolitsch, F. Gobec and C. Slugovc, Eur. J. Org. Chem., 2020, 2020, 2973 CrossRef CAS;
(k) A. Gupta and M. L. Condakes, J. Org. Chem., 2021, 86, 17523 CrossRef CAS PubMed;
(l) V. Srinivasulu, M. A. A. Khanfar, H. A. Omar, R. ElAwady, S. M. Sieburth, A. Sebastian, D. M. Zaher, F. A. Marzooq, F. H and T. H. Al-Tel, J. Org. Chem., 2019, 84, 14476 CrossRef CAS PubMed;
(m) V. Srinivasulu, I. Shehadi, M. A. Khanfar, O. G. Malik, H. Tarazi, I. A. Abu-Yousef, A. Sebastian, N. Baniodeh, M. J. O'Connor and T. H. Al-Tel, J. Org. Chem., 2019, 84, 934 CrossRef CAS PubMed;
(n) V. Srinivasulu, P. Schilf, S. Ibrahim, M. A. Khanfar, S. M. Sieburth, H. Omar, A. Sebastian, R. A. AlQawasmeh, M. J. O'Connor and T. H. Al-Tel, Nat. Commun., 2018, 9, 4989 CrossRef PubMed.
- For selected reports, see:
(a) P. Diner, M. Nielsen, M. Marigo and K. A. Jørgensen, Angew. Chem., Int. Ed., 2007, 46, 1983 CrossRef CAS PubMed;
(b) Q. Lin, D. Meloni, Y. Pan, M. Xia, J. Rodgers, S. Shepard, M. Li, L. Galya, B. Metcalf, T.-Y. Yue, P. Liu and J. Zhou, Org. Lett., 2009, 11, 1999 CrossRef CAS PubMed;
(c) J. Zhang, Y. Zhang, X. Liu, J. Guo, W. Cao, L. Lin and X. M Feng, Adv. Synth. Catal., 2014, 356, 3545 CrossRef CAS;
(d) P. Li, F. Fang, J. Chen and J. Wang, Tetrahedron: Asymmetry, 2014, 25, 98 CrossRef CAS;
(e) S.-J. Lee, J.-Y. Bae and C.-W. Cho, Eur. J. Org. Chem., 2015, 2015, 6495 CrossRef CAS.
- F. Chevallier, Y. S. Halauko, C. Pecceu, I. F. Nassar, T. U. Dam, T. Roisnel, V. E. Matulis, O. A. Ivashkevichd and F. Mongin, Org. Biomol. Chem., 2011, 9, 4671 RSC.
- For selected reports, see:
(a) I. Meskini, L. Toupet, M. Daoudi, A. Kerbal, B. Bennani, P. H. Dixneuf, Z. H. Chohan, A. C. L. Leite and T. B. Hadda, J. Braz. Chem. Soc., 2010, 21, 1129 CrossRef CAS;
(b) I. Meskini, L. Toupet, M. Daoudi, A. Kerbal, M. Akkurt, Z. H. Chohan and T. B. Hadda, J. Chem. Crystallogr., 2010, 40, 812 CrossRef CAS;
(c) L. Patalag, J. A. Ulrichs, P. G. Jones and D. B. Werz, Org. Lett., 2017, 19, 2090 CrossRef CAS PubMed.
- For selected reports, see:
(a) F. Zigheimat, M. R. Islami and F. Nourmohammadian, Synlett, 2014, 25, 229 CAS;
(b) J. Yang, Y. Bao, H. Zhou, T. Li, N. Li and Z. Li, Synthesis, 2016, 48, 1139 CrossRef CAS.
- For selected reports on the aza-Michael addition of 1H-benzotriazole, see:
(a) G. Luo, S. Zhang, W. Duan and W. Wang, Synthesis, 2009, 9, 1564 Search PubMed;
(b) J. Lv, H. Wu and Y. Wang, Eur. J. Org. Chem., 2010, 2010, 2073 CrossRef;
(c) J. Wang, W. Wang, X. Liu, Z. Hou, L. Lin and X. M. Feng, Eur. J. Org. Chem., 2011, 2011, 2039 CrossRef;
(d) S.-L. Xie, Y.-H. Hui, X.-J. Long, C.-C. Wang and Z.-F. Xie, Chin. Chem. Lett., 2013, 24, 28 CrossRef CAS;
(e) S.-W. Chen, G.-C. Zhang, Q.-X. Lou, W. Cui, S.-S. Zhang, W.-H. Hu and J.-L. Zhao, ChemCatChem, 2015, 7, 1935 CrossRef CAS;
(f) Z. Li, T. Li, R. Fu and J. Yang, Heterocycl. Commun., 2017, 23, 287 CrossRef CAS.
|
This journal is © The Royal Society of Chemistry 2022 |
Click here to see how this site uses Cookies. View our privacy policy here.