DOI:
10.1039/D2RA01987F
(Paper)
RSC Adv., 2022,
12, 12932-12937
Synthesis of renewable diesel and jet fuel range alkanes using 2-methylfuran and cyclohexanone†
Received
28th March 2022
, Accepted 21st April 2022
First published on 28th April 2022
Abstract
New solid acid catalysts were prepared by bisphenol A, paraformaldehyde and chlorosulfonic acid, and applied to hydroxylalkylation/alkylation (HAA) of 2-methylfuran (2-MF) and cyclohexanone. After optimizing the reaction conditions, the conversion of 2-MF reached 99% and the yield of 5,5′-(cyclohexane-1,1-diyl)bis(2-methylfuran) acquired 98%. The activity and catalytic efficiency were higher than those of Amberlyst 15 and Amberlyst 36 resins, which could be rationalized by high acid strength. At the same time, the catalysts were characterized by acid–base titration and FTIR. Hydrodeoxygenation (HDO) of HAA products of 2-methylfuran and cyclopentanone were processed on the Ni/SiO2 catalyst prepared by wet impregnation method to further convert into aviation kerosene, and the yield reached 93%.
Introduction
Entering the 21st century, the aviation transportation industry has developed rapidly and occupies an important position in economic development and national security. Therefore, the safe and stable supply of high-quality aviation fuel is crucial. However, the substitutability of aviation fuel is poor. Aviation fuel is mainly derived from petrochemical-based fossil resources. Then, the combustion of petrochemical-based fuel also brings serious air pollution. So the development of renewable and environmentally friendly aviation fuel is the key to promote the sustainable development of the aviation industry.
In recent years, the use of renewable biomass resources to produce fuels1–3 and chemicals4–7 has attracted wide attention. Many works have confirmed the high potential of using lignocellulose to produce platform compounds. However, the carbon content of lignocellulose materials is generally C5–C6, which is far lower than the minimum carbon number of diesel fuel. Oxygenated compounds with long carbon chain of diesel or jet fuel are prepared by C–C coupling reaction of platform chemicals derived from lignocellulose. Subsequently, these oxygenated compounds were further hydrodeoxygenated to alkanes with high energy density.
2-Methylfuran (2-MF) is obtained the selective hydrogenation product of furfural, which is produced on an industrial scale through the hydrolysis and dehydration of hemicellulose in agricultural wastes and forest residues.8–10 High density biofuel precursors were generated by hydroxyl alkylation/alkylation of 2-methylfuran and furfural, and hydrodeoxidation. Alonso D. M.11 and YuJia Luo et al.11,12 used Lewis acid Zr/SBA-15 catalyst to catalyze the hydroxylalkylation/alkylation of furfural and methylfuran. Methylfuran was hydroxylated (alkylated) with acetone, hydroxyacetone, mesityl oxide and butyraldehyde, and hydrodeoxygenated to synthesize renewable biomass diesel oil. High-density biofuel was synthetized using hydroxyl alkylation/alkylation of cyclopentanone/cyclohexanone and furfural. Wei Wang, et al.,13–17 reported renewable diesel were obtained with 2-methylfuran and angelica lactone under catalysts for the reaction include Nafion212, Amberlyst-36 and Amberlyst-15 resin. However, the high cost of Nafion212 resins may limit their use in industrial scale. In order to meet the needs of practical application, it is still necessary to develop cheaper and more accessible solid acid catalysts.
In this work, A novel sulfonated resin solid acid catalyst (SR180) was prepared using bisphenol A,18 paraformaldehyde and chlorosulfonic acid for the first time. Hydroxyalkylation/alkylation reaction of for cyclohexanone and 2-MF was implemented on the SR180 as catalyst for the solvent-free. The effects of calcination temperature, reaction temperature, reaction time and catalyst dosage on HAA reaction were studied. As the final purpose of this experiment, HAA products of 2-MF and cyclohexanone were further hydrodeoxygenated on Ni/SiO2 catalyst to obtain alkanes in the range of diesel and jet fuel. In order to understand the catalytic performance of the catalyst, a series of methods were used to characterize the catalyst.
Experimental
Material
p-Toluene sulfonic acid was purchased from Tianjin Damao Chemical Reagent Factory. Paraformaldehyde was provided from Tianjin Kemiou Chemical Reagent Factory. Bisphenol A was purchased from Tianjin Institute of Photoing Fine Chemistry. A total of 3.05 g bisphenol A was weighed, and 10 mL of n-butanol was placed in a round-bottom flask with a condenser tube and a thermometer, and heated in a constant temperature oil bath at 80 °C. When bisphenol A was completely dissolved, the solution was transparent. Then 1.88 g paraformaldehyde and 0.28 g p-toluene sulfonic acid were added, and the reaction mixture was heated to 90 °C for 6 h. After the reaction was completed, the n-butanol solvent was evaporated by a rotary evaporator, and then calcined at 150 °C for two hours. The carrier was obtained by grinding, sieving and calcination. Then 1.00 g of solid acid catalyst carrier was weighed and placed in a round-bottom flask. 1 mL of chlorosulfonic acid was added and stirred evenly. The mixture was stirred in an oil bath at 50 °C for 4 h. After the reaction was complete, it was quenched with ice water and filtered. The deionized water was washed until the pH of the washing solution was neutral. The mixture was dried overnight at 80 °C in an oven and packaged for use.
H-Y (SiO2/Al2O3 = 4), Hβ (SiO2/Al2O3 = 4), H-ZSM-5 (SiO2/Al2O3 = 50) were purchased from Nankai University, nano-SiO2 was supplied from The Aladdin reagent factory. Hydrodeoxygenation catalysts of Ni/H-ZSM-5, Ni/Hβ, Ni/HY, Ni/SiO2 were prepared. The saturated water absorption of H-ZSM-5, Hβ, HY, SiO2 molecular sieves were 1.5, 1.6, 1.65, 2.5 mL g−1, respectively. A certain concentration of nickel nitrate solution was prepared, and it was impregnated on different molecular sieves (molecular sieves need to be calcined at 773 K for 4 h before impregnation), stirring evenly, standing at room temperature for 24 h, 393 K overnight drying. Then the catalyst was calcined at 773 K for 4 h in air. The catalyst was reduced under condition of 120 mL min−1 H2, at 773 K during 2 h. The loading of Ni is 5 wt%.
Instrument measurement
Accurately weigh 2 mg of SR resin to be tested and 200 mg of vacuum-dried KBr, mix them uniformly in a 2 mL sample tube, and then pour them into an agate mortar and repeatedly grind and press them into tablets.
Measuring the number of –SO3H sites on the catalyst surface. Firstly, 0.1 g catalyst was dispersed in 20 mL 2 mol L−1 NaCl solution and ultrasonicated for 1.5 h, then centrifuged. The obtained liquid was titrated with 8 mmol L−1 NaOH solution (pre-calibrated with standard potassium hydrogen phthalate solution) and phenolphthalein was used as indicator. The –SO3H molar amount per gram catalyst is calculated according to the consumption of NaOH solution.
Catalytic tests
Hydroxyalkylation/alkylation reaction (HAA) reaction of 2-methylfuran (2-MF) with furfural was carried out in a 35 mL pressure tube. The reaction temperature was controlled by oil bath method. 2-MF and cyclohexanone are purchased in https://www.Aladdin-E.com and used directly without further purification. 0.98 g cyclohexanone (10 mmol), 1.68 g 2-MF (20 mmol) and 0.10 g catalyst were placed in a 35 mL pressure tube and stirred for 2 h in solvent-free setting temperature for HAA reaction of 2-MF and cyclohexanone. The product analysis showed that the HAA product mainly contained C16 intermediate by NMR analysis, indicating that 2-MF and cyclohexanone could be effectively transferred to the target product in the presence of sulfonic acid solid acid catalyst. After the reaction, the sample was centrifuged and quantitatively determined by gas chromatography (Agilent 7890 B). The gas chromatography analysis of liquid products was carried out on Agilent 7890 B chromatograph under the meteorological condition, using HP-INNOWAX capillary column (30 m, 0.25 mm ID, 0.5 mm membrane) and FID detector. The column temperature was maintained at 40 °C for 2 min, and rose to 280 °C at 15 °C∙ min−1 for 5 min. The injection port and FID were set at 250 °C and 320 °C, respectively. Hydrogen flow 30 mL min−1, air flow 400 mL min−1 and tail blowing 25 mL min−1.
The catalytic reaction was carried out in a 100 mL stainless steel autoclave equipped with a mechanical agitator, a pressure gauge and an automatic temperature control device. In this experiment, 500 mg HAA product, 200 mg hydrodeoxygenation catalyst and 10 mL cyclohexane were added to the reactor. After sealing, the reactor was purged with hydrogen 4–5 times and pressurized to 4 MPa. Then the reactor was heated to the required temperature and stirred continuously at 800 rpm. After the reaction was completed, the samples were cooled to room temperature and centrifuged for quantitative determination by Agilent 7890 B.
Results and discussion
Catalyst characterization
The calcination temperature of the catalyst precursor was investigated, and the volatile components were removed to obtain a stable solid acid precursor. The determination results of solid acid content obtained by sulfonation of precursor chlorosulfonic acid at different calcination temperatures are shown in Table 1. It can be seen from Table 1 that when the calcination temperature of the solid acid precursor is from 100 to 300 °C, the acid content of the prepared solid acid increases first and then decreases. At 150 °C and 180 °C, the acid content of catalysts of SR150 and SR180 were 2.74 mmol g−1 and 2.79 mmol g−1. The acid amount of SR150 is equivalent to that of SR180.
Table 1 Acid values of the catalysts different calcination temperature used in HAA of 2-MF and cyclohexanone
Calcination |
Temperature (°C) |
Acid value (mmol g−1) |
SR100 |
100 |
2.16 |
SR150 |
150 |
2.74 |
SR180 |
180 |
2.79 |
SR200 |
200 |
2.06 |
SR300 |
300 |
1.98 |
Infrared spectra of the prepared solid acid catalysts of SR100, SR150, SR180, SR200 and SR300 resin were shown in Fig. 1. It can be seen that the material has many functional groups. The broad peak at 3420 cm−1 can be attributed to OH groups adjacent to oxygen atoms or SO3H groups via hydrogen bonds. According to ref. 19, the peaks at 2847 cm−1 and 2939 cm−1 can be attributed to the stretching vibration of –CH2– in catalysts. The bands at 1218 cm−1 and 1034 cm−1 can be attributed to the symmetric stretching of O
S
O and SO3–H in the SO3H group, respectively.20 Peaks at 1425 cm−1, 1500 cm−1 and 1612 cm−1 can be attributed to the characteristic vibrations of benzene rings.21,22
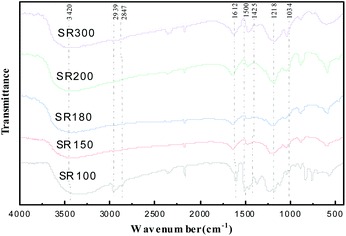 |
| Fig. 1 IR spectra of solid acid catalysts over SR180. However, the 5,5′-(cyclohexane-1,1-diyl)bis(2-methylfuran) yield decreases over SR200 and SR300 with the increase of calcination temperature. When the calcination temperature of 300 °C, the 5,5′-(cyclohexane-1,1-diyl)bis(2-methylfuran) yield was decreased to 78% over SR300. | |
Hydroxyalkylation/alkylation
The hydroxyalkylation/alkylation of 2-methylfuran with cyclohexanone is carried out over a series solid acid catalysts. The hydroxyalkylation/alkylation reaction between 2-methylfuran and cyclohexanone is shown in Scheme 1. The NMR spectra of hydroxylation/alkylation product of 2-methylfuran with cyclohexanone are shown as p. S1 and p. S2 in ESI† respectively. The NMR spectrum of a (p. S1 in ESI†) shows the following peaks 1H NMR (CDCl3-d), δ: 5.99 (s, 2H), 5.96 (s, 2H), 2.30 (s, 6H), 2.09 (d, 4H, J = 12), 1.51 (m, 6H); 13C NMR (CDCl3-d), δ: 154.8 (2C), 150 (2C), 106.2 (2C), 105.6 (2C), 45.7 (1C), 36.1 (2C), 25.1 (1C), 18 (2C), 13.7 (2C). Based on 1HNMR and 13CNMR spectra analysis, 5,5′-(cyclohexane-1,1-diyl)bis(2-methylfuran) (a) was identified as the major product.
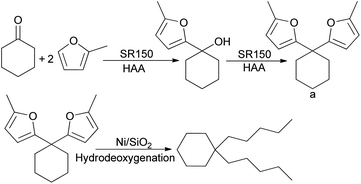 |
| Scheme 1 Reaction route of alkanes in the range of synthetic diesel or aviation kerosene fuels using 2-MF and cyclohexanone. | |
HAA of 2-MF and cyclohexanone solvent-free were carried out on acidic catalysts by acidification of phenolic condensation products of bisphenol A and paraformaldehyde. 5,5′-(Cyclohexane-1,1-diyl)bis(2-methylfuran) is the main product.
The effect of catalysts at different calcination temperatures on the hydroxylalkylation/alkylation reaction of 2-methylfuran and cyclohexanone was shown Fig. 2. It can be seen from the Fig. 2 that with the increased in calcination temperature, the product yield first increased and then decreased, which is consistent with the acid amount of catalysts of the acid–base titration. When the calcination temperature is 150 °C, the 5,5′-(cyclohexane-1,1-diyl)bis(2-methylfuran) yield is 93.95% over SR150. When the calcination temperature is 180 °C, the yield of 5,5′-(cyclohexane-1,1-diyl)bis(2-methylfuran) is 94.26%.
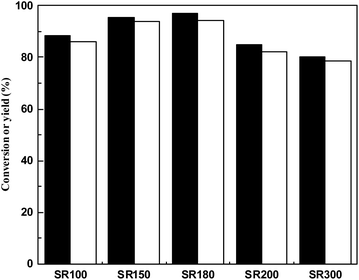 |
| Fig. 2 Changes of conversion of 2-MF (black bar) and carbon yield of a (white bar) under different calcination temperatures. Reaction conditions: 60 °C, 2 hours; 0.98 g cyclohexanone and 1.68 g 2-MF, different SR catalysts 0.10 g. | |
It can be seen from Fig. 3 that the activity of acidic resins decreased in the order of SR150 > Nafion212 > Amberlyst15 > Amberlyst36. This can be explained by the chemical structure of these acidic resins. According to literature22,23 only –SO3H is the active centre catalyzing the HAA reaction of 2-methylfuran and cyclohexanone, and the number of –SO3H acidic sites for Amberlyst15, Amberlyst36 (ref. 24) and Nafion212 are 4.43, 3.52 and 1.08 mmolg−1. Water is formed during HAA of 2-MF and cyclohexanone. Under the catalysis of acidic sites, the water produced by the HAA reaction can further react with the furan functional groups in the HAA product, and this continuous HAA-hydrolysis-HAA reaction (or excessive condensation) will lead to the production of large polymer molecules, which will block Holes in Amberlyst resin. The order of activity is therefore as above. The HAA yields of 2-MF and cyclohexanone catalyzed by Nafion212 and amberlyst15 were consistent with the results reported in the literature.25
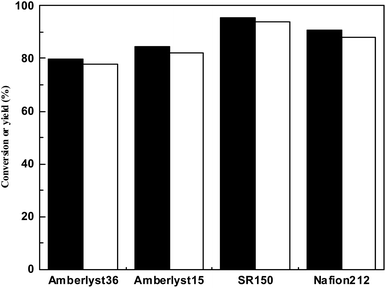 |
| Fig. 3 Conversion of 2-MF (black bar) and carbon yield of a (white bar) catalyzed by different acidic resins. Reaction conditions: 60 °C, 2 h; 0.10 g SR150, 0.98 g cyclohexanone and 1.68 g 2-MF. | |
According to ref. 26–30, the HAA reaction of 2-MF was very sensitive to the acidity of the catalyst. In the HAA reaction of 2-MF, strong acid was more active than weak acid. The HAA reaction of 2-MF and cyclohexanone was easier due to the electrophilicity of cyclohexanone.
The activity of different acidic resins in 2-MF and cyclohexanone HAA is related to the acid strength of these catalysts. The acid strength of different resins can be rationalized by the chemical environment of –SO3H groups. According to ref. 30, Nafion resin is a perfluorosulfonic acid resin, commonly known as superacid. Amberlyst resin is a kind of sulfonic acid functionalized crosslinked polystyrene resin. We can also attribute the excellent performance of SR150 in the HAA reaction of 2-MF and cyclohexanone to its relatively high acid strength.
It can be seen from Fig. 4 that the catalytic efficiency increases with the increase of catalyst dosage. When the catalyst dosage of 0.01 g, the 5,5′-(cyclohexane-1,1-diyl)bis(2-methylfuran) yield is 32.86%, and the catalytic effect increased with the increase of catalyst dosage. When the catalyst dosage increased 0.01 g, the 5,5′-(cyclohexane-1,1-diyl)bis (2-methylfuran) yield increases slowly. Therefore, in the follow-up study, the amount of catalyst is 0.01 g.
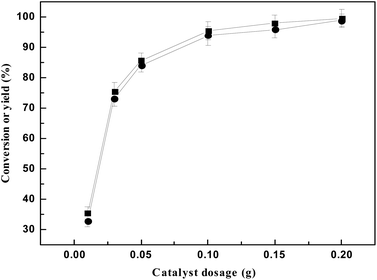 |
| Fig. 4 The conversion of 2-MF (■) and the carbon yield of a (●) varied with the amount of catalyst. Reaction conditions: 60 °C, 2 hours; 0.98 g cyclohexanone and 1.68 g 2-MF. | |
The effect of reaction temperature on HAA reaction of 2 MF and cyclohexanone is shown in Fig. 5. Fig. 5 showed that when the reaction temperature is from 40 °C to 60 °C, the catalytic efficiency of is improved. While the reaction temperature is 40 °C, the yield of the 5,5′-(cyclohexane-1,1-diyl)bis(2-methylfuran) is 61%, and when the temperature is higher than 60 °C, the yield of the 5,5′-(cyclohexane-1,1-diyl)bis(2-methylfuran) yield increased only from 93% to 96%. Based on green energy saving, 60 °C is the best reaction temperature.
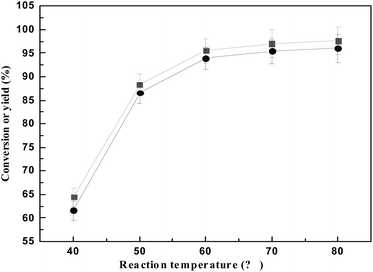 |
| Fig. 5 The conversion of 2-MF (■) and the carbon yield of a (●) varied with reaction temperature. Reaction conditions: 2 h, 0.10 g SR150; 0.98 g cyclohexanone and 1.68 g 2-MF. | |
The effect of reaction time on HAA reaction of 2 MF and cyclohexanone is shown in Fig. 6. It can be seen from Fig. 6 that the yield of the 5,5′-(cyclohexane-1,1-diyl)bis(2-methylfuran) is 40% when the reaction time is 15 min, the yield of the 5,5′-(cyclohexane-1,1-diyl)bis(2-methylfuran) increased significantly to 93% with the extension of reaction time up to 2 h. Then the yield of 5,5′-(cyclohexane-1,1-diyl)bis(2-methylfuran) changes gently with the extension of reaction time. The yield of 5,5′-(cyclohexane-1,1-diyl)bis(2-methylfuran) is 98% when the reaction time of 6 h.
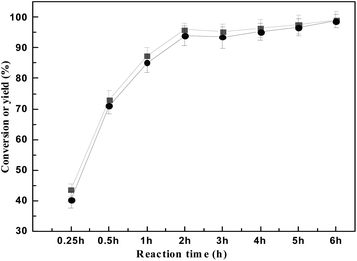 |
| Fig. 6 The conversion of 2-MF (■) and the carbon yield of a (●) varied with reaction time. Reaction conditions: 60 °C, 0.10 g SR150; 0.98 g cyclohexanone and 1.68 g 2-MF. | |
We also study the reusability of SR150 for HAA of 2-MF and cyclohexanone. Therefore, SR150 was reused under optimal conditions (0.10 g catalyst, 60 °C, 2 h). In order to eliminate the influence of residues (including HAA products, unreacted 2-MF and cyclohexanone) on the used catalysts, the catalysts were fully washed and soaked with methanol and dried at 60 °C for 2 h. From the results shown in Fig. 7, SR150 is very stable under the conditions studied, the catalyst is reused four times.
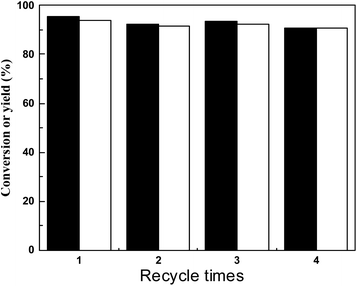 |
| Fig. 7 Number of catalyst recycles, conversion of 2-MF (black bar) and carbon yield of a (white bar). Reaction conditions: 60 °C; 0.10 g SR150; 0.98 g cyclohexanone and 1.68 g 2-MF. | |
Hydrodeoxygenation reaction
As the ultimate goal of this work, alkanes in the range of renewable biomass diesel or aviation fuel were produced. We also studied the hydrodeoxygenation (HDO) of HAA products of 2-MF and cyclohexanone. HDO was reacted in a 100 mL stainless steel axe reactor with cyclohexane as solvent. After the reaction, the samples were cooled to room temperature and centrifuged for quantitative determination by GC (p. S3 in ESI†) and GC-MS (p. S4 in ESI†). According to GC and GC-MS analysis, 5,5'-(cyclohexane-1,1-diyl)bis(2-methylfuran) was completely converted to alkanes on non-noble metal supported molecular sieves, with 1,1-dipentylcyclohexane as the main product.
Hydrodeoxygenation of HAA product of 2-MF and cyclohexanone was implemented over molecular sieve loaded non-noble metal catalysts. For comparison, the loading amount of metal nickel in the catalyst is 5%. It can be seen from Fig. 8 that the carbon yield (80.97%) of C8–C16 alkanes in diesel and jet fuels over Ni/SiO2 was higher than that over the catalysts of Ni/HY (73.51%), Ni/HZSM5 (60.13%) and Ni/Hβ (35.35%). Under this reaction condition, Ni/HZSM5 was not completely hydrodeoxygenated, and the product of Ni/Hβ had 45% naphtha.
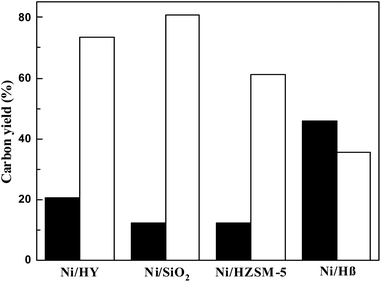 |
| Fig. 8 Carbon yields of C8–C16 diesel range alkanes (white bars), C5–C7 gasoline range alkanes (black bars), over molecular sieve loaded non-noble metal catalysts. Reaction conditions: 500 mg HAA product, 200 mg catalyst, 280 °C, 24 h. | |
After hydrodeoxygenation mixture of 5,5′-(cyclohexane-1,1-diyl)bis(2-methylfuran) was distilled under reduced pressure, the obtained biofuel has a density of 0.83 g mL−1.
Conclusions
In this work, a new solid acid resin catalyst was prepared by bisphenol A, paraformaldehyde and chlorosulfonic acid, and applied to the hydroxyalkylation/alkylation of 2-methylfuran and cyclohexanone. The conversion of 2-MF reached 99% and the yield of 5,5′-(cyclohexane-1,1-diyl)bis(2-methylfuran) acquired 98%, under optimizing the reaction conditions. At the same time, hydrodeoxygenation of HAA products of 2-MF and cyclohexanone were executed over non-noble metal-supported molecular sieve catalysts. 5,5′-(Cyclohexane-1,1-diyl)bis(2-methylfuran) was completely converted to alkanes over Ni/SiO2, with 1,1-dipentylcyclohexane as the main product. The carbon yield of C8–C16 alkanes in diesel and jet fuels reached 80.97%. Acquired cycloalkanes has 0.83 g mL−1. It can be used as high-density fuel or additive in other diesel or aviation kerosene fuels.
Conflicts of interest
There are no conflicts to declare.
Acknowledgements
This work was supported by Key Scientific Research Plan of Shaanxi Provincial Education Department in 2020 (20JS013), and Shaanxi Natural Science Basic Research Program (S2020-JC-WT-0001 and 2020JM-598) and the Open Project of Shaanxi Key Laboratory of Catalysis (SLGPT2019KF01-24).
Notes and references
- X. Wang and R. Rinaldi, A Route for Lignin and Bio-Oil Conversion: Dehydroxylation of Phenols into Arenes by Catalytic Tandem Reactions, Angew. Chem., 2013, 125(44), 11499–11503 CrossRef PubMed.
- K. Barta and P. C. Ford, Catalytic conversion of nonfood woody biomass solids to organic liquids, Acc. Chem. Res., 2014, 45(5), 1503–1512 CrossRef PubMed.
- S. Shylesh, A. A. Gokhale, C. R. Ho and A. T. Bell, Novel Strategies for the Production of Fuels, Lubricants, and Chemicals from Biomass, Acc. Chem. Res., 2017, 50, 2589–2597 CrossRef CAS PubMed.
- A. Corma, S. Iborra and A. Velty, Chemical routes for the transformation of biomass into chemicals, Chem. Rev., 2007, 107(6), 2411–2502 CrossRef CAS PubMed.
- M. Zheng, J. Pang and A. Wang, et al., One-pot catalytic conversion of cellulose to ethylene glycol and other chemicals: From fundamental discovery to potential commercialization, Chin. J. Catal., 2014, 35(5), 602–613 CrossRef CAS.
- Y. Zan, G. Miao and H. Wang, et al., Revealing the roles of components in glucose selective hydrogenation into 1,2-propanediol and ethylene glycol over Ni-MnO_x-ZnO catalysts, J. Energy Chem., 2019, 38, 15–19 CrossRef.
- A. Corma, S. Iborra and A. Velty, Chemical routes for the transformation of biomass into chemicals, Chem. Rev., 2007, 107(6), 2411–2502 CrossRef CAS PubMed.
- M. J. Gilkey, et al., Mechanistic Insights into Metal Lewis Acid-Mediated Catalytic Transfer Hydrogenation of Furfural to 2-Methylfuran, ACS Catal., 2015, 5(7), 3988–3994 CrossRef CAS.
- L. Grazia, et al., Gas-phase cascade upgrading of furfural to 2-methylfuran using methanol as a H-transfer reactant and MgO based catalysts, Catal. Sci. Technol., 2016, 6(12), 4418–4427 RSC.
- M. Kalong, et al., Hydrogen-free hydrogenation of furfural to furfuryl alcohol and 2-methylfuran over Ni and Co-promoted Cu/γ-Al2O3 catalysts, Fuel Process. Technol., 2021, 214, 106721 CrossRef CAS.
- D. M. Alonso, J. Q. Bond and J. A. Dumesic, Catalytic conversion of biomass to biofuels, Green Chem., 2010, 12(9), 1493–1513 RSC.
- Y. J. Luo, Y. H. Zhou and Y. B. Huang, A New Lewis Acidic Zr Catalyst for the Synthesis of Furanic Diesel Precursor from Biomass Derived Furfural and 2-Methylfuran, Catal. Lett., 2018, 149 Search PubMed.
- Q. Deng and J. Xu, et al., Efficient synthesis of high-density aviation biofuel via solvent-free aldol condensation of cyclic ketones and furanic aldehydes, Fuel Process. Technol., 2016, 148, 361–366 CrossRef CAS.
- W. Wang, S. Sun, F. Han, G. Li, X. Shao and L. Ning, Synthesis of Diesel and Jet Fuel Range Cycloalkanes with Cyclopentanone and Furfural, Catalysts, 2019, 9, 886 CrossRef CAS.
- A. Corma, et al., Production of high-quality diesel from biomass waste products, Angew. Chem., Int. Ed., 2011, 50(10), 2375–2378 CrossRef CAS PubMed.
- O. d. l. T. a. M. R. Avelino Corma, Production of high quality diesel from cellulose and hemicellulose by the Sylvan process: catalysts and process variables, Energy Environ. Sci., 2012, 5, 6328–6344 RSC.
- W. Wang, et al., Synthesis of renewable high-density fuel with isophorone, Sci. Rep., 2017, 7(1), 6111 CrossRef PubMed.
- F. Liguori, C. Moreno-Marrodan and P. Barbaro, Biomass-derived chemical substitutes for bisphenol A: recent advancements in catalytic synthesis, Chem Soc Rev., 2020, 49(17), 6329–6363 RSC.
- X. Zhang, Z. Zhang and F. Wang, et al., Lignosulfonate-based heterogeneous sulfonic acid catalyst for hydrolyzing glycosidic bonds of polysaccharides, J. Mol. Catal. Chem., 2013, 377, 102–107 CrossRef CAS.
- D. Lee, Preparation of a Sulfonated Carbonaceous Material from Lignosulfonate and Its Usefulness as an Esterification Catalyst, Molecules, 2013, 18(7), 8168–8180 CrossRef CAS PubMed.
- X. Ouyang, L. Ke, X. Qiu, Y. Guo and Y. Pang, Sulfonation of Alkali Lignin and Its Potential Use in Dispersant for Cement, J. Dispersion Sci. Technol., 2009, 30(1), 1–6 CrossRef CAS.
- S. Li and N. Li, et al., Lignosulfonate-based acidic resin for the synthesis
of renewable diesel and jet fuel range alkanes with 2-methylfuran and furfural, Green Chem., 2015, 17, 3644–3652 RSC.
- G. Ren and G. Li, et al., Cycloalkanes with 2-methylfuran and benzaldehyde, Sustain. Energy Fuels, 2022,(6), 1156–1163 RSC.
- G. Li, N. Li and J. Yang, et al., Synthesis of renewable diesel with the 2-methylfuran, butanal and acetone derived from lignocellulose, Bioresour. Technol., 2013, 134, 66–72 CrossRef CAS PubMed.
- Q. Deng, P. Han and J. Xu, et al., Highly controllable and selective hydroxyalkylation/alkylation of 2-methylfuran with cyclohexanone for synthesis of high-density biofuel, Chem. Eng. Sci., 2015, 138, 239–243 CrossRef CAS.
- G. Li, N. Li, Z. Wang, C. Li, A. Wang, X. Wang, Y. Cong and T. Zhang, Synthesis of High-Quality Diesel with Furfural and 2-Methylfuran from Hemicellulose, ChemSusChem, 2012, 5(10), 1958–1966 CrossRef CAS PubMed.
- L. Guang, L. Ning and J. Yang, et al, Synthesis of renewable diesel with the 2-methylfuran, butanal and acetone derived from lignocellulose, Bioresour. Technol., 2013, 134(Complete), 66–72 Search PubMed.
- Li Guang, Li Ning and S. Li, et al., Synthesis of renewable diesel with hydroxyacetone and 2-methyl-furan, Chem. Commun., 2013, 49(51), 5727–5729 RSC.
- Li Guang, Li Ning and X. Wang, et al., Synthesis of Diesel or Jet Fuel Range Cycloalkanes with 2-Methylfuran and Cyclopentanone from Lignocellulose, Energy Fuels, 2014, 28, 5112–5118 CrossRef.
- B. G. Harvey, M. E. Wright and R. L. Quintana, High-Density Renewable Fuels Based on the Selective Dimerization of Pinenes, Chem. Commun., 2012, 24, 267–273 Search PubMed.
|
This journal is © The Royal Society of Chemistry 2022 |
Click here to see how this site uses Cookies. View our privacy policy here.