DOI:
10.1039/D2RA01185A
(Paper)
RSC Adv., 2022,
12, 30817-30828
A novel expansive soil hardener: performance and mechanism of immersion stability
Received
22nd February 2022
, Accepted 11th October 2022
First published on 27th October 2022
Abstract
Aiming at the existing problems of poor treatment effect and immersion stability of expansive soils, a slag soil hardener (SSH, developed by Wuhan University, China) was combined with different additives to dispose in this study. The free expansion rate, compressive strength, and immersion stability of samples were compared, and the influences of different additives, curing age, and dry density on the process and mechanism of improvement were discussed. The experimental results indicated that SSH combined with quicklime had the best improvement effect on expansive soils, in which the mass ratio of raw materials was: expansive soil/SSH/quicklime = 92/4/4, and the free expansion rate decreased from 45.90% to 4.4%, compressive strength increased from 2.53 MPa to 6.69 MPa, and there was no splitting after immersion under this ratio. FTIR spectroscopy, XPS and SEM were performed to analyze the characteristic functional groups, structural forms, and morphology of samples to study the mechanism of improvement, which showed that SSH greatly reduced the proportion of montmorillonite in the whole system and further enhanced the mechanism of ion exchange, soil particle connection, and coating protection. The research can provide theoretical reference for engineering the application of expansive soil area in rainy climate and has dual economic and environmental benefits.
1. Introduction
Expansive soils are a kind of highly plastic clay with obvious expansion and contraction, as their volume changes with the environment.1 Its basic components are hydrophilic minerals mainly including montmorillonite and illite,2 which are greatly affected by environmental humidity.3 According to statistics, expansive soils have been found in more than 40 countries and are widely distributed in six continents with a large number.4 Expansive soils are widely distributed in China, accumulating in more than 20 provinces and regions.5 Because expansive soils have softened cracking caused by moisture change around bad engineering properties,6 such as in China or even worldwide, the expansive soil area engineering problems and frequent geological disasters7 have become key technical problems and one of major issues in construction, mainly including the road construction and other kinds of shallow engineering constructions.8
In order to meet the requirements of engineering fillers, the chemical improvement method with simple operation and short time limit is widely adopted in the world to improve the free expansion rate, strength, and immersion stability of expansive soils.9 The more common chemical improvers mainly include fly ash,10 cement,11 sodium silicate,12 organic matter,13 other new improvers,14 and quicklime improvement.15 Pradhan P. K. et al.16 showed that the fly ash can improve the early strength of expansive soils and achieve waste treatment with waste, but the stability after immersion is insufficient. Du J. et al.17 found that cement can reduce the hydrophilicity of expansive soil particles and improve the stability after immersion,18 but the application scope is limited because of the large shrinkage coefficient. Murmu A. L. et al.,19 through the improvement of sodium silicate, found that the gel effect could effectively reinforce expansive soils and enhance the cohesive force of soil particles, resulting in an aggregation phenomenon. However, the material itself has a large viscosity and complicated practical operation, and the strength is difficult to reach the actual engineering application standards.
Quicklime has gradually become the most widely used improver for expansive soils due to its advantages of easy availability of raw materials and simple operation, but there are still problems such as insufficient stability.20 According to the study of Shi B. et al.,21 after the addition of 5–10% quicklime into expansive soils for improvement, their free expansion rate and strength can meet the requirements of engineering use, but the immersion stability of quicklime soils is obviously insufficient, which cannot meet the actual engineering application in rainy climate areas and lead to engineering problems.22 The slag soil hardener (hereinafter referred to as “SSH”) is a silicon aluminum-based environmental material developed by Wuhan University, China, which contains a variety of high-state cations, has good hydrorigid cementitious properties, and can effectively solidify sands, soils and other construction waste materials to recycle in actual engineering construction. Therefore, SSH was tried to be applied to the research of expansive soils to explore the solution of the problem of insufficient immersion stability.
In order to solve the problem of insufficient immersion stability of expansive soils and further improve their free expansion rate and compressive strength to expand the application scope of expansive soils as engineering fillers, especially in the expansive soil area with rainy climate, in this study, SSH combined with different additives was used to modify the typical expansive soil. The immersion stability, free expansion rate and compressive strength of samples were compared, and the effects of the different additives, curing age, and dry density on the improvement were discussed to select the best additives and ratio. FTIR spectroscopy, XPS and SEM were used to characterize the functional group characteristics, composition and morphology of expansive soils before and after modification to study the improvement process and mechanism.
2. Materials and methods
2.1 Materials
2.1.1 Expansive soils. The expansive soil used in the research was taken from Guangxi Province, China and was gray and white. Weak expansive soils are common in the local, while medium and strong expansive soils are rarely distributed. In order to remove some impurities that were not part of the soil particles and to ensure the accuracy of the results, the expansive soil was sampled in the local field and air-dried at room temperature for 48 h, crushed and screened through a soil sieve (2 mm) for use as a research material.
2.1.2 Slag soil hardeners (SSHs). SSHs are mainly composed of slag or slag composition, which is mixed with an appropriate ratio of activators and surfactants and ground to a Brewer ratio area of 400–900 m2 kg−1. The SSH used in the research was taken from Wuhan University of China. In addition, in order to make it fully contact the soil particles, the reaction area was increased, which facilitates the exploration of the improvement effect; then, the SSH was used as a research material after drying, grinding and passing standard sieve (0.5 mm).
2.1.3 Additives. Based on the current research results, quicklime is one of the best modified materials to improve the basic physical properties of expansive soils, so it was used as one of the additives in this study. Steel slag itself, as a cementitious material, contains a variety of high-valence cations, which can promote the ion exchange of the expansive soil system and reduce the hydrophilicity of soil particles. In addition, a small amount of sand was added to reduce the water dispersion of expansive soil particles, so as to explore the applicability of different additives to the improvement of expansive soils.The quicklime used in the research was powdered and 65% pure. The specific surface area of steel slag used was 0.32 m2 g−1, the average porosity was 5.3 nm, the grindability index was 0.96, and the density was in the range of 3.1–3.6 g cm−3. The sand used was fine sand for ordinary concrete, and its fineness modulus was within the range of 2.2–1.6.
2.2 Methods
2.2.1 Characterization methods. The moisture content, liquid-plastic limit, particle size distribution and chemical composition were determined according to the “Standard for Geotechnical Test Methods of China” (GB/50123-2019), and a digital display soil liquid plastic limit combined tester (LP-100D, resolution 0.1 mm) was used to measure the liquid-plastic limit of expansive soils. The moisture content was measured using a moisture meter (Dsh-50a-1), the particle size distribution was determined using a laser particle size analyzer (Microtrac S3500), and the chemical composition was analyzed using an XRF (ARL Perform-X).
2.2.2 Preparation and curing. The preparation and curing process is shown in Fig. 1. Different ratios of materials were added to the same ratio of deionized water (Table 1 for specific ratios) and stirred in a mortar mixer (YDT712-4/8) for 30 min, and a plastic film was used to seal and store the mixture. Water was evenly distributed in the mixture. After sealing for 3 days, the material was poured into a φ 5 × 5 mold, placed in a universal material testing machine, and pressed and formed under 40 kN pressure. After demolding, the sample was cured for 7 d and 28 d at room temperature, respectively, for subsequent determination and characterization.
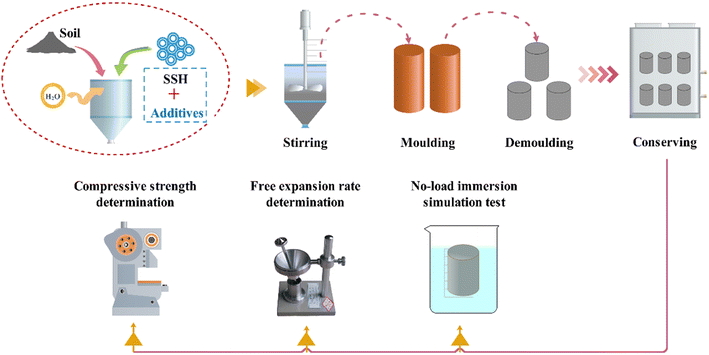 |
| Fig. 1 Flow chart of the experiment. | |
Table 1 Experimental results for different raw material ratios
Samples |
Materials (mass fraction, %) |
Expansive soil |
SSH hardener |
Additives |
Quicklime |
Steel slag |
Sand |
S0 |
100 |
0 |
0 |
0 |
0 |
S1 |
92 |
0 |
8 |
0 |
0 |
S2 |
92 |
4 |
4 |
0 |
0 |
S3 |
92 |
4 |
0 |
4 |
0 |
S4 |
89 |
3 |
0 |
3 |
5 |
S5 |
89 |
3 |
3 |
0 |
5 |
2.2.3 Determination of free expansion rate. According to the “Technical Code for Building in Expansive Soil Area of China” (GB/50112-2013), the free expansion rates of the original expansive soil and the samples after curing for 28 days were measured using a free expansion rate tester (WX-2000).
2.2.4 Determination of compressive strength. According to the “Test Method for Physical and Mechanical Properties of Concrete of China” (GB/50081-2019) determination of the compressive strength of solidified body, this test was conducted using a universal material testing machine (WE-300S, accuracy grade 1), and calculations were done as P = FN/A, where P is the compressive strength, unit MPa; FN is the failure load, unit N; A is the loading area, unit mm2.
2.2.5 No-load water immersion simulation test. After curing for 28 days, the samples were immersed in water without loading, and the dispersion degree was recorded at 3, 5, 10, 30, 60, 120, 180, and 24 × 60 min. The residual volume of the corresponding cured body was measured, and the water-immersion stability of different samples was compared.
2.2.6 Determination methods. The main mineral compositions of expansive soils were preliminarily confirmed by XRD (Rigaku Miniflex 600). In addition, the original expansive soil and the samples with a curing age of 28 days were selected for characterization. An FTIR spectrometer (Thermo Nicolet 5700) was used to analyze the characteristic functional groups. The main compositions and structural forms were characterized by XPS (Thermo ESCALAB 250XI) and the morphology was analyzed by SEM (TESCAN MIRA3 LMH).
2.2.7 Differential analysis of samples. The raw material ratios of the samples provided in Table 1 are the original data obtained from the test. In order to build a unified measurement standard, the Z-score standardized calculation formula (eqn (1)) was used in this study to standardize and transform the test parameters. The standardized parameters of the obtained samples are shown in Table 2. |
 | (1) |
Table 2 Standardized parameters of samples
Samples |
Expansive soil (χ1) |
SSH hardener (χ2) |
Quicklime (χ3) |
Steel slag (χ4) |
Sand (χ5) |
S0 |
2.0823 |
−1.3728 |
−0.8533 |
−0.6965 |
−0.7071 |
S1 |
−0.0905 |
−1.3728 |
1.8773 |
−0.6965 |
−0.7071 |
S2 |
−0.0905 |
0.9806 |
0.5120 |
−0.6965 |
−0.7071 |
S3 |
−0.0905 |
0.9806 |
−0.8533 |
1.6916 |
−0.7071 |
S4 |
−0.9054 |
0.3922 |
−0.8533 |
1.0945 |
1.4142 |
The correlation matrix RL of the samples obtained from the transformation of standardized parameters is shown in eqn (2).
|
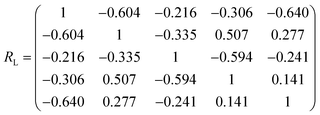 | (2) |
The characteristic equation of RL can be obtained from the correlation matrix of samples, as shown in eqn (3):
The five non-negative eigenvalues and variance contribution rates calculated according to the characteristic equation are shown in Table 3, and the parameters with eigenvalues > 1 were taken as principal components. It can be seen that the variance contribution rates of expansive soil and SSH hardener were α1 = 48.196% and α2 = 28.458%, respectively, and the sum of them was 76.654%, while the sum of variance contribution rates of quicklime, steel slag and sand was only α3 + α4 + α5 = 23.346%. It was much smaller than α1 + α2 = 76.654%. Therefore, it can be considered that expansive soils and SSH hardeners have a large weight on the ratio index of sample raw materials.
Table 3 Eigenvalue and variance contribution rate of RL
Parameters |
Initial eigenvalue |
Principal components extraction |
Eigenvalue |
Variance contribution rate (αi, %) |
Cumulative value (%) |
Eigenvalue |
Variance contribution rate (αi, %) |
Cumulative value (%) |
χ1 |
2.410 |
48.196 |
48.196 |
2.410 |
48.196 |
48.196 |
χ2 |
1.423 |
28.458 |
76.654 |
1.423 |
28.458 |
76.654 |
χ3 |
3.728 × 10−4 |
0.008 |
76.662 |
|
|
|
χ4 |
0.399 |
7.979 |
84.641 |
|
|
|
χ5 |
0.768 |
15.359 |
100.000 |
|
|
|
3. Results and discussion
3.1 Properties of expansive soils
The basic physical properties and chemical composition of the expansive soil are shown in Tables 4 and 5. It can be seen that the free expansion rate of the expansive soil was Fs > 40% and the plasticity index IP > 15%, which indicated that the expansive soil was the weak expansive soil and mainly composed of Si, Al, Fe and other elements, belonging to the SiO2–Al2O3–Fe2O3 system. The Si element composition was obviously large, and usually existed in the form of aluminosilicate.23
Table 4 Basic physical properties of expansive soils
Moisture content (%) |
Maximum dry density (g cm−3) |
Liquid limit/WL (%) |
Plastic limit/WP (%) |
Plastic index/IP (%) |
Free expansion rate/Fs (%) |
13.50 |
1.88 |
43.98 |
20.68 |
23.30 |
45.90 |
Table 5 Chemical compositions of expansive soils (mass fraction, %)
Compounds |
SiO2 |
Al2O3 |
Fe2O3 |
K2O |
CaO |
MgO |
TiO2 |
Others |
Wt (%) |
69.28 |
17.13 |
6.21 |
2.33 |
1.99 |
1.33 |
1.01 |
0.72 |
XRD was performed to determine the main mineral components in expansive soils, and the results are shown in Fig. 2. It can be preliminarily determined that the main mineral components were montmorillonite, illite, kaolinite, quartz, illite/montmorillonite mixed layer and kaolinite/montmorillonite mixed layer. In order to further determine the types and morphology of minerals contained in expansive soil, more accurate detection should be carried out by XPS, see “3.6.1 XPS results” for details.
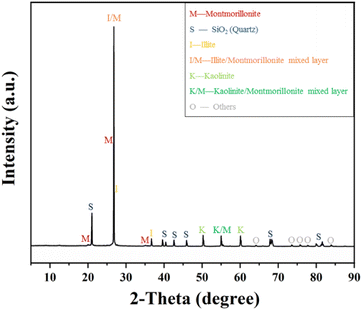 |
| Fig. 2 XRD characterization of the expansive soil. | |
The free expansion rate of expansive soil was mainly determined by the exposed area of expansive minerals such as montmorillonite and illite. The soil particle size determines the exposed surface area of corresponding substances and the combination effect with a hardener to a certain extent, which greatly affects the soil expansion potential and stability improvement.24 The particle size distribution of expansive soils used in the research is shown in Fig. 3. It can be seen that the particle size of <5.0 μm accounted for 26.54%, the particle size of 5.0–20.0 μm accounted for 49.95%, and the particle size of >20.0 μm accounted for 23.51%, mainly distributed in the range of 1–20 μm, which indicated the presence of a certain degree of exposed area with the corresponding expansion potential and ability to combine with the hardener in the soil.
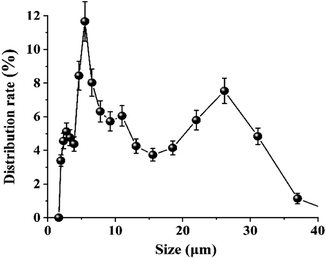 |
| Fig. 3 Particle size distribution of the expansive soil. | |
3.2 Properties of the SSH
The main chemical composition of the SSH used in the research is shown in Table 6. It can be seen that it was mainly composed of Si, Al, Ca and other elements, belonging to the SiO2–Al2O3–CaO system. Its powder usually existed in the form of oxides, and the corresponding salts were formed after mixing with water, with strong curing performance.25
Table 6 Chemical compositions of SSH (mass fraction, %)
Compounds |
SiO2 |
Al2O3 |
CaO |
MgO |
Fe2O3 |
SO3 |
Wt (%) |
26.4–43.2 |
7.9–23.6 |
20.2–39.3 |
1.2–10.1 |
0.4–4.8 |
0.1–0.8 |
In order to ensure the high surface activity of SSH, it is necessary to grind it to a small size in the research. The particle size distribution of SSH is shown in Fig. 4. It can be seen that the particle size < 5.0 μm accounted for 45.29%, the particle size between 5.0 and 10.0 μm accounted for 30.25%, and the particle size > 10.0 μm accounted for 24.46%, mainly distributed in the range of 1–10 μm, which indicated that it can have a large contact area with other substances.
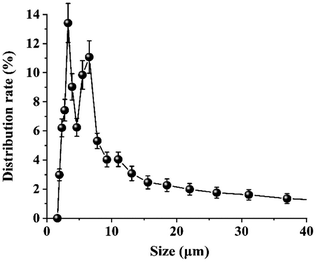 |
| Fig. 4 Particle size distribution of the SSH. | |
3.3 Free expansion rate
According to the corresponding classification requirements of expansive soils mentioned in the “Technical Code for Highway Subgrade Construction of China” (JTG/3610-2019), the expansive soil can be identified only when its free expansion rate reaches over 40%,26 and the free expansion rate of the expansive soil after chemical improvement determines its grade as a construction filler.27
In the research, the free expansion rate of the samples with a curing age of 28 d, different raw materials and ratios was measured, and the results are shown in Fig. 5. It can be seen that the free expansion rate of the original expansive soil (S0 sample) was 45.90%. After the incorporation of different materials and ratios, the free expansion rates of S3 and S4 samples were little different from that of the original expansive soil, which only slightly decreased. However, the free expansion rate of S1, S2 and S5 samples decreased significantly. Comparing the effects of the three samples, it can be seen that the free expansion rate of addition of the SSH decreased more than that of the use of quicklime alone, and the improvement effect was about 1.5 times. In addition, the incorporation effect of the sand was poor and the free expansion rate increased. In conclusion, improvement by SSH combined with quicklime had the best effect in the free expansion rate of expansive soil, which was only 4.4%.
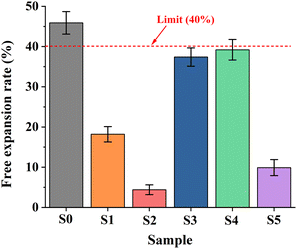 |
| Fig. 5 Free expansion rate of samples. | |
3.4 Compressive strength and dry density
When the expansive soil is used as a building filler, it needs to have a certain compressive strength to ensure that it is not easily damaged by external forces, so as to maintain the stability of buildings in engineering construction.28 The compressive strength and dry density of samples with different raw materials and ratios were measured after curing to 7 d and 28 d, and the results are shown in Fig. 6 and 7.
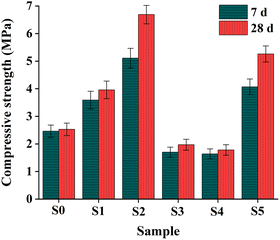 |
| Fig. 6 Compressive strength of samples. | |
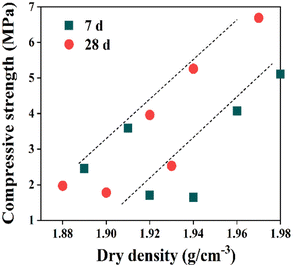 |
| Fig. 7 Dry density – compressive strength graph. | |
It can be seen from Fig. 6 that the strength of original expansive soil (S0 sample) in the research at 7 d and 28 d was 2.46 and 2.53 MPa respectively. After the addition of raw materials with different ratios, the strength of S3 and S4 samples decreased slightly, while the strength of S1, S2 and S5 samples at each age increased significantly. The strength of S2 sample cured by the SSH combined with quicklime was the highest, which was 2.08–2.64 times higher than that of the original expansive soil and 1.42–1.69 times that of using quicklime alone.
Fig. 7 shows that the dry density of samples at different ages was in the range of 1.88–1.98 g cm−3, and the compressive strength of samples fluctuated slightly with the change in dry density within this range. Moreover, as the dry density increased, the compressive strength of samples showed an upward trend. In addition, it can be seen from Fig. 6 and 7 that the curing age increased from 7 d to 28 d, which had a significant influence on the strength of S2 and S5 samples. The compressive strength of S2 samples with the best effect increased by 31% and 29% from 7 d to 28 d, respectively, which indicated that the combined effect of the SSH and quicklime to improve the expansive soil was greatly affected by the curing age.
Therefore, according to the analysis of free expansion rate, compressive strength and dry density indexes, the S1, S2 and S5 samples with good effects were all mixed with quicklime, which confirmed that quicklime played a certain role in the improvement of expansive soils.29 By comparing S1 and S2 samples, the incorporation of the SSH can reduce the free expansion rate and increase the compressive strength clearly. By comparing S2 and S5, the compressive strength decreased obviously and free expansion rate increased slightly after the addition of sand. In conclusion, the steel slag and sand as additives have no obvious effect on expansive soil improvement, and quicklime had a certain effect, while the free expansion rate and compressive strength of the expansive soil can be improved more significantly by adding SSHs compared with using quicklime alone.
3.5 No-load water immersion simulation test
After curing for 28 d, a water immersion simulation test without loading was carried out for the samples with different raw materials and ratios. The test process is shown in Fig. 8; the samples were immersed in water without loading, and the dispersion phenomenon at different time points was observed and the residual volume of the cured body was measured. In addition, the dispersion degree in soaking of the samples at main time points was compared, and the results are shown in Fig. 9 and 10.
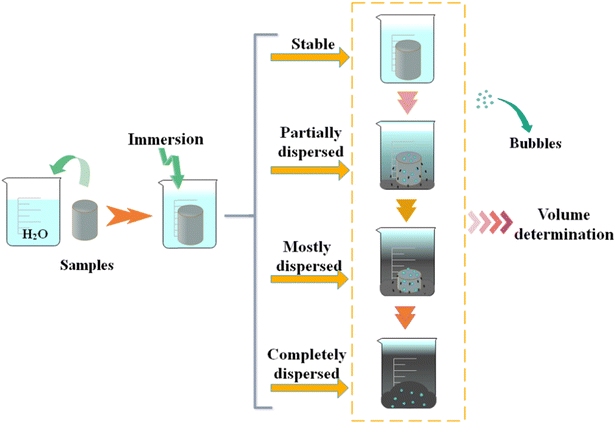 |
| Fig. 8 Flow chart of the no-load immersion simulation experiment. | |
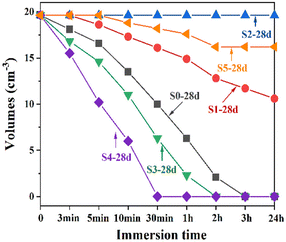 |
| Fig. 9 Trend graph of volume change of samples. | |
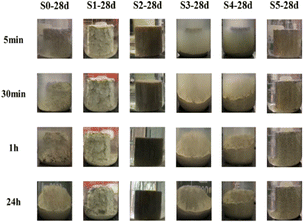 |
| Fig. 10 Main changes of samples. | |
It can be seen from Fig. 9 and 10 that there were significant differences in the soaking results of the samples with different raw materials and ratios. Except the S2 sample, other samples showed different degrees of dispersion or even completely collapsed after soaking. Among them, the S4 sample dispersed fastest and completely dispersed within 30 min, followed by the S3 and S0 samples, which completely dispersed within 2 h and 3 h, respectively. In addition, the S1 and S5 samples with general effects showed different degrees of dispersion after immersion, and the dispersion rate of the S1 sample was faster than that of the S5 sample. However, the S2 sample with the best effect did not show any dispersion or collapse phenomenon after soaking in water, and its compressive strength was still as high as 5.83 MPa after soaking in water for 24 h, which only slightly decreased compared with that before soaking in water. Therefore, it can be seen that the expansive soil improved by quicklime alone was obviously less stable in soaking water, which confirmed the previous studies and cannot meet the requirements of engineering fillers in expansive soil area in the rainy climate. However, the incorporation of SSHs greatly improved the immersion stability of expansive soils, and the compressive strength difference after soaking was not obvious, which can be applied to the construction of expansive soil areas in the rainy climate.
Based on the above-mentioned results of the free expansion rate, compressive strength and no-load water immersion simulation test of the samples with different raw materials and ratios, it can be seen that the effects of various indexes of S2 sample were the best, indicating that the addition of 4% SSH and 4% quicklime had the best effect on the improvement of expansive soils. Among them, the use of quicklime alone had a certain effect on the improvement of expansive soils, but the immersion stability was obviously insufficient. The incorporation of SSHs can significantly enhance the immersion stability, meanwhile improving the free expansion rate and compressive strength of expansive soils, which solved the problem of engineering fillers in expansive soil areas in the rainy climate.
3.6 Mechanism study
Combined with the chemical composition of materials of the samples and the high content of Si in the expansive soil (about 70%), the original expansive soil (S0-0 d) and the samples with a curing age of 28 days were selected. By studying the existing forms of main element Si, surface characteristic functional groups and microstructure changes in the samples of expansive soils before and after improving, the improvement process and mechanism were analyzed.
3.6.1 XPS results. XPS was performed to study the existence forms of the main element Si in the above-mentioned samples, and the results are shown in Fig. 11. As can be seen from it, Si elements mainly exist in the form of montmorillonite [(Na,Ca)Al2Mg(Si4O10)(OH)2·4H2O] and quartz (SiOx) in the original expansive soil (S0-0 d) and after curing for 28 days (S0 sample), and the binding energy of SiOx increased after curing, which indicated that the properties of the expansive soil become stabler after water gain and loss. In the S1 sample with quicklime alone, a large amount of C–S–H gels such as CaSiO3 were formed after the hydration reaction, and the ratio of montmorillonite to SiO2 decreased. In the S2 sample with SSH and quicklime, Ca6(Si6O17)(OH)2 and Al2SiO5 were the main forms, and the percentage of montmorillonite decreased obviously. Montmorillonite still existed in the main mineral form in the S3 and S4 samples, and some crystals such as Ca6(Si6O17)(OH)2 were also formed, but the form of Ca6(Si6O17)(OH)2 mainly existed in the S5 sample. Combined with the previous research results that the free expansion rate of expansive soil increased with the proportion of montmorillonite.30 It can be seen that the incorporation of SSH can significantly reduce the proportion of montmorillonite in the expansive soil, the degree of reduction was more obvious than using quicklime alone. And a large number of hardness larger compound salts were generated, which reduced the expansion potential and close-grained solidification structure through certain packages and protection mechanism. Therefore, the free expansion rate decreased more significantly, while the compressive strength improved.
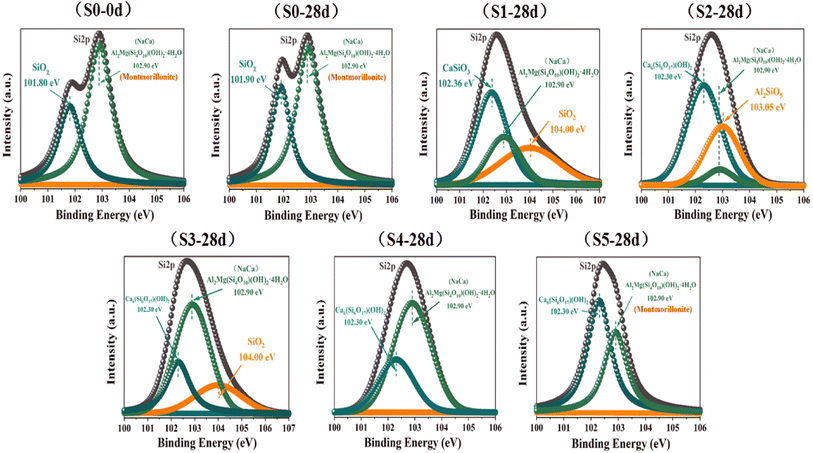 |
| Fig. 11 XPS characterization of samples. | |
3.6.2 FTIR results. The surface functional groups of the above-mentioned samples were characterized by FTIR spectroscopy, and the results are shown in Fig. 12. As can be seen from it, there were a large number of free hydroxyl groups in the whole reaction system. Compared with the original expansive soil (S0-0 d) and after curing for 28 days (S0 sample), the improved samples all generated the calcium silicate complex salts. In addition, the diffraction peak of the Si–O–Si bond in the samples after improvement was redshifted, the wave number became smaller, and the bond energy became weaker. Combined with quicklime, the expansive soil itself was mainly composed of montmorillonite and SiOx, the properties of components were stabler after improvement, and the stability was of the order of S2 > S5 > S1 > S4 > S3 > S0 > S0-0 d, which further confirmed that the properties of the expansive soil became stabler after water gain and loss, and quicklime can improve the properties of expansive soils to a certain extent, while the incorporation of SSHs had a more significant enhancement effect on the improvement of expansive soils than the use of quicklime alone.
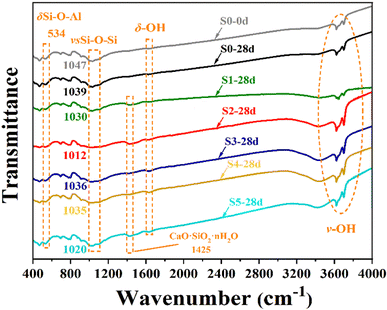 |
| Fig. 12 FTIR characterization of samples. | |
3.6.3 SEM results. The surface morphology changes of the above-mentioned samples were characterized by SEM, and the results are shown in Fig. 13. As can be seen from it, due to the presence of the main mineral component montmorillonite, the surface of the original expansive soil (S0-0 d) and after curing for 28 days (S0 sample) before improvement had a flaky structure, poor intergranular bonding ability, and overall dispersion, so it had a high expansion potential. Compared with the S0 sample, the S1 sample improved by using quicklime alone had better intergranular binding ability, and some particles aggregated into clusters, but the overall microstructure still showed a certain degree of dispersion. Compared with the S0 and S1 samples, the S2 sample improved by using SSH and quicklime had enhanced intergranular bonding ability, and the particles basically aggregated and became massive, which significantly restrained the expansion ability of montmorillonite. Compared with the S0 sample, the S3 and S4 samples had a slightly stronger intergranular binding ability, and a small number of particles aggregated and formed partial clumps, but the effect was not as obvious as for the S1, S2 and S5 samples. Moreover, the aggregation degree of soil particles in S5 was between that of the S1 and S2 samples.
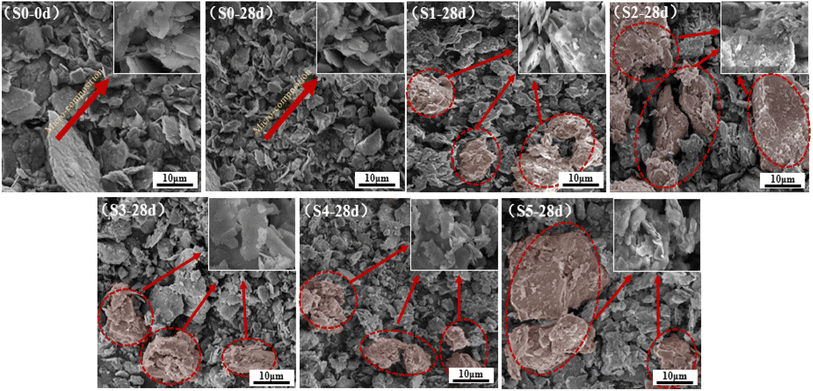 |
| Fig. 13 SEM characterization of samples (red area surrounded by circle indicated aggregates of soil particles and arrows point to indicated morphological features of the aggregates when magnified). | |
From the above-mentioned analysis, it can be seen that quicklime had a certain promotion effect on the binding ability between the particles of expansive soils, while the aggregation ability between the particles of expansive soil was further significantly improved after the incorporation of SSHs, and the surface morphology basically changes from dispersed flake to block, which had a good inhibition effect on the expansion potential of expansive soil particles and was beneficial to improve the compressive strength, and the effect was more significant than that of using quicklime alone.
3.6.4 Mechanism analysis. Combined with the above-mentioned test and characterization results, the process and mechanism of SSHs combined with quicklime to improve expansive soil performance were analyzed, and a comparison was made between using quicklime alone. The analysis results are shown in Fig. 14. According to the research of Nishimura S. et al.,31 the swelling, adhesion and strength of expansive soil particles were mainly determined by the thickness of the water film binding between soil particles. The thinner the water film is, the smaller the swelling is, the stronger the adhesion between particles is, and the higher the strength is.32 In addition, the type of cation adsorbed by soil particles has a great influence on the thickness of the colloidal double electric layer, and the ion exchange capacity of the system is in descending order of Fe3+, Fe2+, Ca2+, Mg2+, K+, H+ and Na+, while the high-value cation tends to make particles aggregate more easily than the low-value cation.33
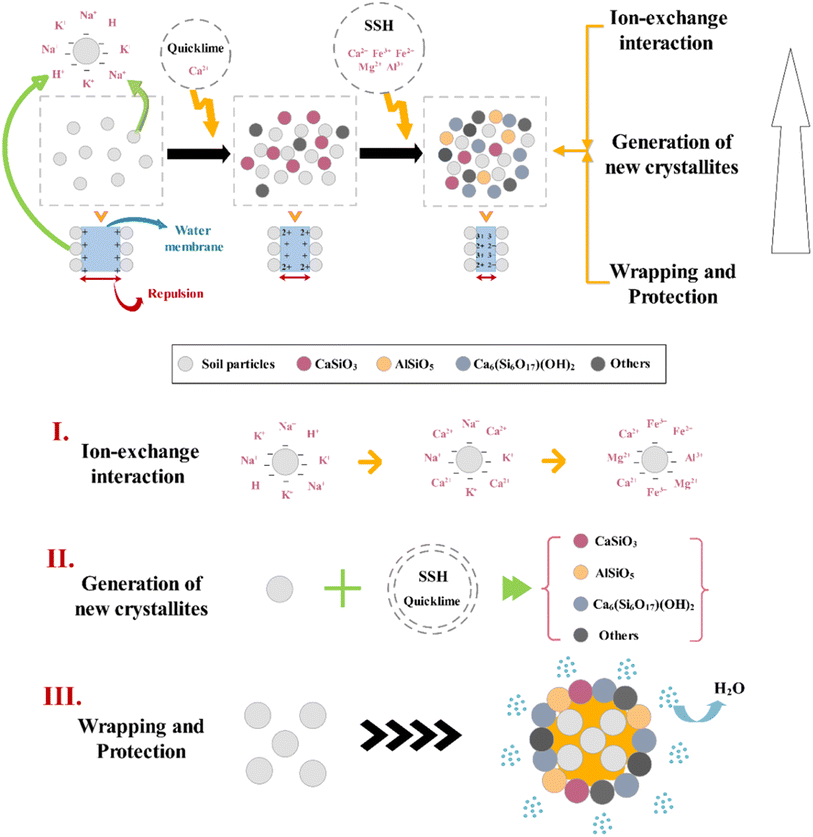 |
| Fig. 14 Mechanism analysis. | |
Fig. 14 shows that Ca2+ contained in quicklime can reduce the thickness of the water film to a certain extent, and gel materials such as CaSiO3 generated via hydration reactions can fill the structure of soil particles and form a certain wrapping and protection effect. The SSH contained a large number of Ca2+, Fe3+, Fe2+, Mg2+, Al3+ and other high-value cations, which further enhanced the exchange of Na+, K+, H+ and other low-value cations in montmorillonite, the main component of the expansive soil, so that the colloidal double electric layer was thinner and repulsion between soil particles was significantly reduced. Thus, the thickness of the soil-particle-bonded water film was less. The general formula of the reaction is as follows:34
|
[L]+ − Al2(Si4O10)(OH)2·nH2O + [H]n+ → [H]n+ − Al2(Si4O10)(OH)2·nH2O + [L]+
| (4) |
In eqn (4), [L]+ represents low-valent cations, and [H]n+ represents high-valent cations. The exchange of cations made the colloidal double electric layer thinner, thus causing changes in the charged properties of the surface of soil particles,35 which accelerated the agglomeration of soil particles and greatly enhanced the aggregation ability between particles, thus significantly promoting the cohesive force and compressive strength of the expansive soil, and improving the free expansion rate and immersion stability.
In addition, the incorporation of the SSH made the whole reaction system produce a large number of crystalline compounds such as Ca6(Si6O17)(OH)2 and Al2SiO5. These substances had significant bonding properties and strength, which can further enhance the binding ability of expansive soil particles. A large number of dispersed soil particles were connected to form a spatial network of large particles, which filled the pores between soil particles and made the overall structure compact. In addition, the composite salt substance formed can form a good wrapping effect on soil particles, thus reducing the soil expansion potential and playing a protective role of water isolation.
Therefore, compared with the use of quicklime alone, the incorporation of the SSH can further enhance the mechanism of ion exchange, soil particle connection and envelope protection to solve the problem of insufficient immersion stability of the expansive soil, meanwhile further improving the free expansion rate and compressive strength.
4. Implication
(1) The slag soil hardener (SSH) solved the defect of insufficient immersion stability of expansive soils in the original improvement methods. Combined with this research applied to the industrial scene, the research can be applied to the actual engineering, especially in the rainy climate region, through the actual operation of spraying, rotary tillage, sealing, roller compaction and regional maintenance on the expansive soil area. In addition, the process is simple and raw materials are easy to obtain, which has the characteristics of green, environmental and efficient treatment.
(2) According to statistics, the cover area of the expansive soil in China is more than 60
000 km2 and the amount is as high as 1.6 × 1011 t, which caused annual economic losses of more than $15 billion.36 Therefore, this research can significantly improve the economic benefit, and the slag soil hardener (SSH) is made of solid wastes, which can achieve the double benefit of treating waste with waste.
5. Conclusions
(1) The research solved the problem of expansive soils in a green and efficient way, and the optimal technological conditions were expansive soil/SSH/quicklime = 92/4/4 (mass ratio). Through spraying, rotary tillage, sealing, compaction and regional conservation, the free expansion rate was reduced from 45.90% to 4.4%, compressive strength was enhanced from 2.53 MPa to 6.69 MPa, and no splitting after immersion under the ratio.
(2) The proportion and hydrophilicity of montmorillonite in the whole system were greatly reduced in the incorporation of SSHs, and the mechanisms of ion exchange, soil particle connection and wrapping protection were further enhanced; meanwhile, composite salt crystalline compounds such as Ca6(Si6O17)(OH)2 were generated.
(3) The research can be applied to the practical engineering application of the expansive soil in the rainy climate, which can recover the economic losses caused by it of about $15 billion based on the existing volume and realize the treatment of waste with waste, which has dual economic and environmental benefits.
Author contributions
Tao Mei: conceptualization, formal analysis, investigation, data curation, writing – original draft. Jirong Lan: writing – review & editing, funding acquisition. Yiqie Dong: resources, writing – review & editing. Shanshan Zhang: investigation. Huiting Tao: validation, supervision. Haobo Hou: funding acquisition, resources, writing – review & editing.
Conflicts of interest
The authors declare that they have no known competing financial interests or personal relationships that could have appeared to influence the work reported in this paper.
Acknowledgements
This work was financially supported by the National Key Research and Development Program of China (2018YFC1801703, 2020YFC1806401) which is greatly appreciated.
References
- S. Arefin, H. Al-Dakheeli and R. Bulut, Stabilization of expansive soils using ionic stabilizer, Bull. Eng. Geol. Environ., 2021,(80), 4025–4033 CrossRef CAS.
- A. Moghal, V. Vydehi and M. B. Moghal, et al., Effect of calcium-based derivatives on consolidation, strength, and lime-leach ability behavior of expansive soil, J. Mater. Civ. Eng., 2020, 32(4), 04020048 CrossRef CAS.
- S. Sudhakar, E. Duraisekaran and G. D. Vignesh, et al., Performance evaluation of quarry dust treated expansive clay for road foundations, Iran. J. Sci. Technol., 2021, 45(4), 2637–2649 Search PubMed.
- M. Malekzadeh and H. Bilsel, Hydro-mechanical behavior of polypropylene fiber reinforced expansive soils, KSCE J. Civ. Eng., 2014, 18(7), 2028–2033 CrossRef.
- Y. Du, S. Li and S. Hayashi, Swelling–shrinkage properties and soil improvement of compacted expansive soil, Ning-Liang Highway, China, Eng. Geol., 1999, 53(3–4), 351–358 CrossRef.
- C. C. Ikeagwuani and D. C. Nwonu, Emerging trends in expansive soil stabilization: a review, Journal of Rock Mechanics and Geotechnical Engineering, 2019, 11(2), 423–440 CrossRef.
- A. Al-Rawas and A. Ali, Microfabric and mineralogical studies on the stabilization of an expansive soil using cement by-pass dust and some types of slags, Can. Geotech. J., 2002, 39(5), 1150–1167 CrossRef CAS.
- S. Selvakumar and B. Soundara, Swelling behaviour of expansive soils with recycled geofoam granules, Geotextiles and Geomembranes, 2019, 47(1), 1–11 CrossRef.
- R. Thirumalai, S. S. Babu and V. Naveennayak, et al., A review on stabilization of expansive soil using industrial solid wastes, Engineering, 2017, 9(12), 1008–1017 CrossRef CAS.
- B. Cetin, M. Dayioglu and S. Nam, Stabilization of expansive Belle Fourche shale clay with different chemical additives, Appl. Clay Sci., 2017,(146), 56–69 Search PubMed.
- N. Nuansrithong and P. Jamsawang, et al., Laboratory investigations on the swelling behavior of composite expansive clays stabilized with shallow and deep clay-cement mixing methods, Appl. Clay Sci., 2017,(148), 83–94 Search PubMed.
- Y. Shang and Y. Fu, Experimental study of the mechanical properties of expansive soil with added nanomaterials, Arabian J. Geosci., 2018, 11(8), 180 CrossRef.
- O. Buzzi and S. Fityus, et al., Use of expanding polyurethane resin to remediate expansive soil foundations, Can. Geotech. J., 2010, 47(6), 623–634 CrossRef CAS.
- N. Ijaz, F. Dai and L. C. Meng, et al., Integrating lignosulphonate and hydrated lime for the amelioration of expansive soil: a sustainable waste solution, J. Cleaner Prod., 2020, 254, 119985 CrossRef CAS.
- W. Ogila, Effectiveness of fresh cement kiln dust as a soil stabilizer and stabilization mechanism of high swelling clays, Environ. Earth Sci., 2021, 80(7), 283 CrossRef CAS.
- P. K. Pradhan, S. K. Mohanty and C. R. Mohanty, et al., Stabilization of expansive soil using industrial wastes, Geomechanics and Engineering, 2017, 12(01), 111–125 CrossRef.
- J. Du, A. Zhou and X. Lin, et al., Revealing expansion mechanism of cement-stabilized expansive soil with different interlayer cations through molecular dynamics simulations, J. Phys. Chem. C, 2020, 124(27), 14672–14684 CrossRef CAS.
- Y. Y. Zhou, F. H. Zhao and W. C. Shi, et al., A general discussion of soil-water interaction in expansive soil, Appl. Mech. Mater., 2013, 368–370(3), 1591–1595 Search PubMed.
- A. L. Murmu, A. Jain and A. Patel, Mechanical properties of alkali activated fly ash geopolymer stabilized expansive clay, KSCE J. Civ. Eng., 2019, 23(9), 3875–3888 CrossRef.
- G. Stoltz, O. Cuisinier and F. Masrouri, Multi-scale analysis of the swelling and shrinkage of a lime-treated expansive clayey soil, Appl. Clay Sci., 2012, 61(61), 44–51 CrossRef CAS.
- B. Shi, H. T. Jiang and Z. B. Liu, et al., Engineering geological characteristics of expansive soils in China, Eng. Geol., 2002, 67(12), 63–71 CrossRef.
- J. Hernandez, S. Vargas and M. Estevez, et al., Hydrophobic modification of an expansive soil using polymers and organic compounds: a comparative study with lime, Geotechnique, 2005, 55(8), 613–616 CrossRef.
- B. V. Reddy, S. M. Rao and M. Muttharam, et al., The impact of cyclic wetting and drying on the swelling behavior of stabilized expansive soils, Eng. Geol., 2001, 60(1), 223–233 Search PubMed.
- D. Yang, Y. Shang and P. Xiang, Study on dynamic elastic modulus and damping ratio of lime-modified expansive soil, Sci. Adv. Mater., 2019, 11(7), 1052–1058 CrossRef CAS.
- H. Haobo, A soil curing agent, China Pat., 98113594.3[ZL], 2001-05-09, 2005 Search PubMed.
- L. Miao, S. Liu and Y. Lai, Research of soil–water characteristics and shear strength features of Nanyang expansive soil, Eng. Geol., 2002, 65(4), 261–267 CrossRef.
- S. H. Liu, Y. S. Wang and K. S. Zhu, et al., Experimental study on strength characteristics of Nanyang expansive soil under loading and its application, J. Hydraul. Eng., 2010, 41(3), 361–367 Search PubMed.
- T. Schanz and M. Elsawy, Swelling characteristics and shear strength of highly expansive clay–lime mixtures: a comparative study, Arabian J. Geosci., 2015, 8(10), 7919–7927 CrossRef CAS.
- S. Khattab, M. Al-Mukhtar and J. M. Fleureau, Long-term stability characteristics of a lime-treated plastic soil, J. Mater. Civ. Eng., 2007, 19(4), 358–366 CrossRef CAS.
- J. Wu, S. Wu and F. Zheng, Features study of impact on fiber expansive soil with wetting-drying cycles, Geotechnical Investigation and Surveying, 2011, 39(9), 14–18 Search PubMed.
- S. Nishimura, S. Por and S. Likitlersuang, et al., Deformation characteristics and stress responses of cement-treated expansive clay under confined one-dimensional swelling, Appl. Clay Sci., 2017,(146), 316–324 Search PubMed.
- U. Chaduvula, B. V. S. Viswanadham and J. Kodikara, A study on desiccation cracking behavior of polyester fiber-reinforced expansive clay, Appl. Clay Sci., 2017, 142(6), 163–172 CrossRef CAS.
- P. V. Sivapullaiah and B. S. N. Prakash, Electroosmotic flow behaviour of metal contaminated expansive soil, J. Hazard. Mater., 2007, 143(3), 682–689 CrossRef CAS PubMed.
- F. H. Chen, Foundations on expansive soils, Developments in Geotechnical Engineering, 1975, 125(3), 29–30 Search PubMed.
- M. Ghasemi and M. Sharifi, Effects of layer-charge distribution on swelling behavior of mixed-layer illite-montmorillonite clays: a molecular dynamics simulation study, J. Mol. Liq., 2021, 335(5–6), 116188 CrossRef CAS.
- J. L. Zheng and H. P. Yang, Treatment theory, technology and practice of expansive soil, People's Communications Press, Beijing, 2004, pp. 3–23 Search PubMed.
|
This journal is © The Royal Society of Chemistry 2022 |
Click here to see how this site uses Cookies. View our privacy policy here.