DOI:
10.1039/D2RA01146H
(Paper)
RSC Adv., 2022,
12, 11443-11453
Waste to white light: a sustainable method for converting biohazardous waste to broadband white LEDs†
Received
21st February 2022
, Accepted 3rd April 2022
First published on 13th April 2022
Abstract
The Covid-19 pandemic has generated a lot of non-degradable biohazardous plastic waste across the globe in the form of disposable surgical and N95 masks, gloves, face shields, syringes, bottles and plastic storage containers. In the present work we address this problem by recycling plastic waste to single system white light emitting carbon dots (CDs) using a pyrolytic method. The synthesized CDs have been embedded into a transparent polymer to form a carbon dot phosphor. This CD phosphor has a broad emission bandwidth of 205 nm and is stable against photo degradation for about a year. A white LED with CRI ∼70 and CIE co-ordinates of (0.25, 0.32) using the fabricated CD phosphor is reported. Further our phosphor is scalable and is environmentally sustainable, and will find wide application in next generation artificial lighting systems.
Introduction
The recent Covid-19 pandemic has impacted the way in which waste is generated across the globe. Due to increased hospitalization and increased use of PPE kits a lot of biohazardous plastic waste is generated that finally ends up as micro-plastics in soil.1 Although PPE made of plastic plays a great role in saving human lives, there is a growing concern about the increase of one time use plastics in the form of disposable surgical face masks, N95 face masks, gloves, face shields, medical kits including syringes, food storage containers (for packaged take-out meals), bottles etc. Sadly this pandemic has halted the war against one time use plastics.2–5 The vast usage of PPE during Covid-19 created a massive disruption in the plastic waste disposal system and has worsened the plastic pollution across the globe. Reports state that hospitals in Wuhan generated ∼240 tons of one time plastic based medical waste per day,6 the 8 week lockdown in Singapore produced ∼1400 tons of plastic waste in the form of plastic containers for packaged meals and home delivered groceries7 accompanied by a 40% escalation in the production of PPE. China has raised its medical mask production to 14.8 million per day and Japan requires ∼600 million masks per month. In the UK alone, 24.37 billion masks per day are required. Based on a report, an overall 1.6 × 105 tons per day of plastic waste has been generated across the globe during this pandemic.8 Due to wide usage of one-time plastics, the global plastic packaging market has projected a growth from USD 902.2 billion in 2019 to USD 1012.6 billion in 2021.9 At the global level, prioritization of human health over environmental pollution has led to a reversal of policies on banning one-time plastics,10 which would have serious effects on human and aquatic life. So it is the demand of the hour to provide alternate waste treatment processes.
Plastic is made of hydrocarbon chains (chemical compounds made of carbon and hydrogen) with abundance of 62–92% carbon content.11,12 In the past, there have been reports on recycling/up cycling of plastic waste. Zhang et al. reported up cycling of polyethylene bags into biodegradable surfactants by a catalytic method using Pt/γ-Al2O3 as catalyst.13 Backstrom et al. reported selective conversion of high-density polyethylene (HDPE) into plasticizers for polylactic acid (PLA) processing. The synthesis mechanism involves microwave assisted degradation of plastic waste in the presence of nitric acid.14 Mahardika et al. reported up cycling of plastic waste into photoluminescence carbon polymer using a heating process at around polyethylene melting point temperature (200–300 °C) in the presence of ethanol solvent.15 Savita et al. reported upcycling of plastic waste into fluorescent carbon dots for copper metal ion sensing in aqueous media. The synthesis route involves thermal calcination of plastic waste in a muffle furnace at 400 °C in the presence of inert atmosphere.16 Wala et al. reported conversion of plastic waste into flash graphene using direct/alternating current flash joule heating technique that relies on electricity to induce flash joule heating in plastic waste.17 Xueying Mu et al. reported direct catalytic carbonization (MgO/Co(acac)3 – hybrid catalyst) of plastic waste in an autoclave at 700 °C into three-dimensional porous carbon nanosheets for high performance capacitors.18 Haoqiang Song et al. produced photo luminescent carbon dots from polystyrene foam using solvothermal method in the presence of dichloromethane (DCE) and nitric acid (HNO3).19 In this synthesis route DCE is used to dissolve polystyrene waste and by varying the quantity of HNO3 in the reaction mixture different color remitting CDs are fabricated. Extreme care need to be taken due to high toxicity of HNO3 and DCE. Also the carbon dots fabricated by Song et al. show dilution dependent behaviour and their emission alters when the concentration of CD is changed which could be a boon to fabricate different light emitting diodes with these dots but has its own drawbacks in fabricating stable white light emitting diodes. Vadivel Ramanan et al. reported synthesis of nitrogen doped carbon dots from polystyrene waste using solvothermal route in the presence of CHCl3 and ethylenediamine for fluorimetric detection of Au3+ ions in aqueous media.20 Noha et al. reported synthesis for carbon-based nanomaterials from polyethylene terephthalate waste by thermal decomposition of plastic waste at 800 °C in an autoclave.21 Archana et al. reported synthesis of green fluorescent carbon quantum dots using polyolefin waste residue for the detection of Cu2+ ions and live cell imaging. The synthesis technique involves pyrolysis of plastic waste using ultrasonic-assisted chemical oxidation approach and use H2SO4 and HNO3 during syntheis.22 Most of the plastic waste recycling techniques reported use harmful chemicals such as H2SO4, HNO3, HCl etc.
In this paper we demonstrate a simple, environment friendly one step recycling process based on pyrolysis of plastic waste into white light emitting carbon dots. This method has potential to transform the current approach for handling plastic waste. Optimization of the synthesis process is carried out to obtain high quality CDs. Further the CDs are embedded in a transparent poly dimethyl siloxane (PDMS) polymer to form solid state white light emitting CD phosphor. Furthermore, CD white LED is fabricated using carbon dots from plastic waste. It has been reported in the recent energy consumption statistics that artificial lighting sources consume ∼40% of total energy production. Since we plan to generate light from waste, this work would have high/immense impact on reducing environmental plastic pollution and address the problem of global energy crisis.
Fabrication section
Recycling of plastic waste to white LEDs (WLEDs) is carried out in 4. Steps involving: (1) plastic waste is collected and sanitized using soap water followed by drying to get rid of excess moisture. (2) Pyrolytic conversion of plastic waste to CDs (3) embedding CDs into transparent PDMS matrix to form CD phosphor (4) use of CD phosphor as active emissive layer in CD WLEDs. The entire process is depicted schematically in Fig. 1. For the synthesis of CDs from PPE plastic waste, used disposable gloves, face shields, syringes, food storage containers and bottles are collected and sanitized. Recycling of plastic waste to CDs is done using heat up method. Heat up method involves steady heating of precursors that result in the formation of nuclei followed by growth of nuclei. Fabrication of CDs from plastic waste involves breakdown of long carbon chains at high temperatures forming carbon dot nuclei accompanied by growth of CD nuclei. It is one of the most efficient single pot synthesis of CDs with poly-disperse particle size distribution as nucleation happens over a period of time. Different plastic wastes (1 g each) are heated separately at 250 °C using octadecene (30 ml) as a non – coordinating solvent. Carbon dot formation is indicated by color change of solution in reaction flask from colorless to dark brown. Further, the fabricated CDs are purified using ethanol as purifying agent and dispersed in chloroform for in depth analysis. Further CDs are incorporated in PDMS polymer. For the fabrication of plastic CD-PDMS polymer phosphor, PDMS and SYLGARD 184 curing agent are mixed in a vial in 10
:
1 ratio and stir continuously for a span of ∼20 min until the mixture gets clear without air bubbles. Further the mixture is degassed in a vacuum desiccator for a span of 20 min. To this mixture 2 ml of plastic CDs in chloroform are added, stirred well and the entire solution is heated at 90 °C until chloroform evaporates from the mixture. In the next step a part of the mixture is coated over a UV LED and the left over mixture is transferred to a pre-fabricated mold and allowed to cure for a span of 24 hours at ambient conditions. After 24 hours the fabricated plastic CD-polymer phosphor is taken out from mold for further studies.
 |
| Fig. 1 Schematic showing (a) recycling of plastic waste to white light emitting CDs using a pyrolytic route at temperatures as high as ∼250 °C. (b) Images of plastic CDs in day light and under UV illumination emitting bright white light. (c) Embedding CDs into transparent PDMS polymer to form CD phosphor. (d) Images of CD phosphor in day light and under UV illumination. (e) CD WLED fabricated using CD phosphor coated over UV LED chip. (f) Image of fabricated plastic CD WLED that emit bright light under driving voltages of 2.6 to 9 V. | |
Fabrication of white LEDs using as fabricated CDs phosphor is carried out successfully (Fig. 1) and elaborate investigation of the CD-WLED is carried out.
Results and discussion
Morphology and structural characterization of plastic CDs
Morphology of fabricated plastic CDs are analysed using high resolution transmission electron microscopy (HRTEM) images as shown in Figure (2a and b) (for morphology studies plastic CDs synthesized using storage container waste are used as they yielded white light with highest quantum yield that will be discussed in optical section). HRTEM images show crystalline spherical nano particle formation with planar lattice fringes of inter planar spacing values 0.17 nm, 0.19 nm, 0.21 nm, 0.34 nm that correspond to (004), (110), (100), (002) planes of graphitic crystal structure. Further, highly magnified images (Fig. 2b) clearly show hexagonal-shaped crystal structure with ABAB stacking of atoms confirming graphitic crystal structure in synthesized plastic CDs. Selected area electron diffraction (SAED) images reveal poly crystalline nature of fabricated plastic CDs (Fig. 2a inset). dhkl values deduced from the SAED pattern are in conformity with the values obtained from HRTEM images, further supporting/corroborating the graphitic crystal structure of the CDs. Furthermore, X-ray diffraction (XRD) patterns of plastic CDs (Fig. 2c) show peaks centered at around 19–21° that corresponds to (002) plane of graphitic crystal structure. Broad diffraction peak observed in XRD is due to partial graphitization, small size of nano particles and wide particle size distribution in the fabricated CDs.23–27 Multiple peaks observed in XRD are impurity peaks due to incomplete carbonization of plastic waste.28 Most of the CDs reported in literature show spherical nano particle morphology based on TEM and HRTEM images with graphitic crystal structure based on XRD data29–33 and our data match with the ones reported. Further, quality of graphitic structure in fabricated plastic CDs is explored using Raman spectra (Fig. 2d). D and G bands in Raman spectra are a typical signature of graphitic structure of CDs. In the Raman spectra of plastic CDs, peaks at 1348 cm−1 and 1581 cm−1 correspond to D and G bands of graphitic structure. D band represent vibrations of disordered sp3 hybridized carbon and G band represents vibration of ordered sp2 hybridized carbon. Furthermore, degree of graphitization is estimated from intensity ratio of ID/IG. An intensity ratio ID/IG of ∼0.85 is estimated from the Raman spectra which proves/demonstrates ordered graphitic structure with less defects. A Fourier transform infrared spectrum (FTIR) is employed to explore the composition and functional groups attached to plastic CD surface (Fig. 3a). Absorption peak at 1716 cm−1 correspond to C
O stretching vibrations. Absorption bands at 1202 cm−1 and 995 cm−1 correspond to C–O stretching vibrations.
 |
| Fig. 2 Showing morphological and structural characterization of fabricated plastic CDs supporting graphitic structure. (a) HRTEM images of plastic CDs showing spherical nano particles with d spacing of 0.17 nm, 0.19 nm, 0.21 nm, 0.34 nm that correspond to (004), (110), (100), (002) planes of graphitic crystal structure. Inset showing SAED image of plastic CDs supporting crystalline behaviour in fabricated plastic CDs. (b) HRTEM image of plastic CDs at 2 nm showing hexagonal crystallite structure with ABAB stacking of atoms. (c) XRD of plastic CDs with peak centered at around 19–21° that corresponds to (002) plane of graphitic crystal structure (d) Raman spectra of plastic CDs with D and G bands at 1348 cm−1 and 1581 cm−1. | |
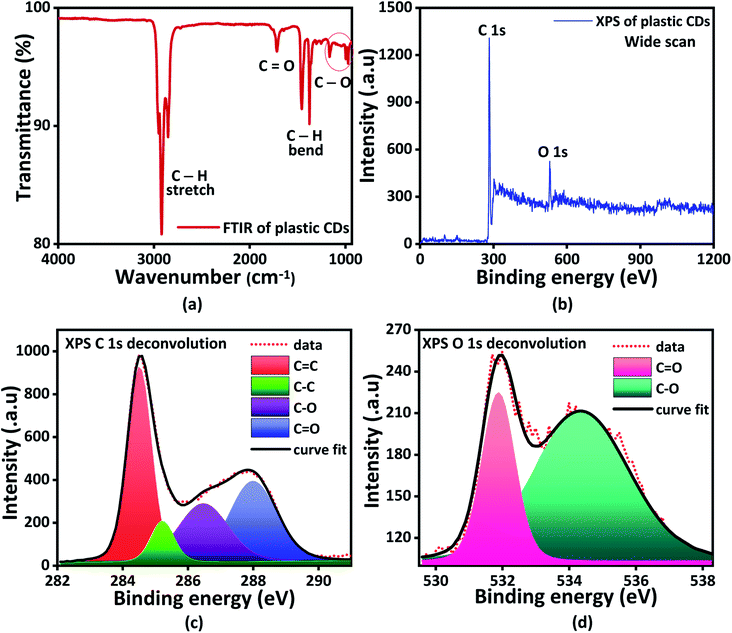 |
| Fig. 3 Showing FTIR and XPS images of plastic CDs elucidating the functional groups attached to CD surface and elemental composition in plastic CDs. (a) FTIR spectra of plastic CDs showing absorption peak at 1716 cm−1 and bands at 1202 cm−1 and 995 cm−1 that correspond to C O and C–O stretching vibrations respectively confirming the presence of C O and C–O groups on CD surface. (b) Wide scan XPS spectra of plastic CDs inferring that CDs are made of carbon and oxygen compounds with C 1s and O 1s bands at 281 eV and 531 eV respectively. De-convolution of (c) C 1s bands reveal C C, C–C, C–O and C O bonds in CDs with peak at 284.5 eV, 285.2 eV, 286.4 eV and 287.3 eV respectively. Further de-convolution of (d) O 1s bands reveal C O and C–O bonds in plastic CDs with peaks centred 531.8 eV and 534.2 eV respectively. | |
Peaks at 1454 cm−1 and 1376 cm−1 correspond to C–H bending modes and C
C stretching modes respectively. Bands at 2951 cm−1, 2918 cm−1 and 2852 cm−1 correspond to C–H stretching vibrations. Presence of C
O and C–O groups in FTIR spectra confirm surface oxygenation of CD surface.
In order to further analyse the elemental composition, bonding and surface groups, X-ray photoelectron spectroscopy (XPS) of fabricated plastic CDs is acquired. Wide scan XPS spectra of plastic CDs show C 1s and O 1s bands at 281 eV and 531 eV respectively which corroborates the inference from FTIR spectra, that fabricated plastic CDs mainly consist of two elements C and O (Fig. 3b). De-convolution of high resolution C 1s spectrum of plastic CDs reveal four peaks at 284.5 eV, 285.2 eV, 286.4 eV and 287.3 eV that correspond to C
C, C–C, C–O and C
O bonds respectively (Fig. 3c). Furthermore de-convolution of high resolution O 1s spectrum of plastic CDs reveal two peaks centred 531.8 eV and 534.2 eV that correspond to C
O and C–O bonds (Fig. 3d). The concentration of C
C bonds is 50.9%, C–C bonds is 11.4%, C–O bonds is 15.9% and C
O bonds comprised of 21.8%. The high intensity of C
C bonds indicate that the structure of fabricated plastic CDs are graphene dominated. Fabricated plastic CDs are carbon composition dominant with 71% carbon and oxygen elemental composition to be 29%. XPS analysis supports FTIR data obtained confirming the presence of C
O and C–O groups on plastic CD surface.
Optical characterization of plastic CDs and CD phosphor
Most of the CD based white LEDs reported so far use multi color (red, green, blue) emitting CDs to generate white light.34–36 However the so fabricated WLEDs suffer from poor stability, self-absorption losses, color aging, poor reproducibility37 etc., one way to address this issue is to use single system white light emitting CDs as active emitters in WLEDs. Also fabricated CDs should be highly luminescent with non-toxic/least toxic nature to use them in commercial lighting devices. In literature single component white light emission from QD is achieved by the creation of short wavelength and long wavelength excited states inside the system.38–42 So far single component CDs doped with nitrogen reported a highest quantum yield of ∼41% with absorption peaks at 280 and 380 nm and emission peaks at 436 and 495 nm. To further explore the optical properties of our fabricated nano structures, UV absorption and emission spectra of plastic CDs synthesized using gloves, bottle, syringe, face shield and storage container plastic waste is recorded. Storage container plastic, syringe, face shield is made of high density polyethylene and bottles are made of polyethylene tetrapthalate (PET/PETE), medical gloves are made of latex/poly vinyl chloride (thermoplastic)/nitrile compounds. CDs synthesized from all these plastic waste show a broad absorption window ranging from 300–400 nm (Fig. 4a). As observed from XPS, FTIR spectra, plastic CDs are made of carbon core with sp2 hybridized carbon and CD surface with sp3 hybridised carbon that are attached to C
O and C–O groups. The broad absorption of plastic CDs corresponds to electronic transitions between energy levels from intrinsic carbon core (π–π* electronic transitions) and surface states (n–π* electronic transitions). This broad absorption of plastic CDs leads to a broad emission spectra as observed in Fig. 4. The emission spectrum of these plastic CDs is recorded at different excitation energies ranging from 320 to 500 nm to explore the core carbon and surface energy states (Fig. 4b–h). Blue shifted emission mostly corresponds to electronic transitions between core carbon energy states (π–π* electronic transitions) and with increase in excitation energy a red shift in emission is observed that corresponds to electronic transitions between surface states on plastic CD surface (n–π* electronic transitions). At 320 nm and 350 nm excitation wavelengths, plastic CDs show a highest emission bandwidth of 159 nm and 140 nm respectively in colloidal state. This unique broad emission achieved using our synthesis technique favours white light emission from plastic CDs and under UV lamp at ∼350 nm (Fig. 4i).
 |
| Fig. 4 Showing (a) absorption spectra of CDs made using syringe, surgical mask, N95 mask, bottle, face shield, gloves and storage container plastic waste generated during Covid-19. Broad absorption is observed that favours broad emission. Emission spectra of CDs from (b) storage container plastic waste (c) face shield (d) gloves (e) bottle (f) syringe (g) surgical face mask (h) N95 face mask. Broad emission is observed that favours white light emission. (i) CDs from plastic waste in day light and under UV illumination, under UV illumination these dots emit bright white light. | |
Further the quantum yield studies of fabricated plastic CDs yield a QY of 6%, 5%, 5%, 5%, 4%, 4% and 3% from CDs fabricated using storage container, gloves, face shield, bottle, surgical mask, N95 mask and syringe plastic waste. QY of CDs can be enhanced by surface functionalization/passivation techniques. More details on QY enhancement of CDs is reported in our previous works.43,44 Henceforth from emission studies, we conclude fabrication of single system white light emitting nano structures from plastic waste and these dots can be used in the fabrication of white light emitting device.
In order to explore the application of plastic CDs in white LEDs it is favourable to make these plastic CDs in solid form or embed them in a solid matrix. CDs obtained from storage container and gloves waste are chosen to fabricate CD-PDMS polymer phosphor (plastic CDs are embedded in PDMS matrix) as they yielded highest QY with broad emission bandwidth. PDMS is chosen as polymer material to embed CDs due to its good dispersity, flexibility properties and its transparency in visible light region as CDs emit white light and any absorption losses occurring by polymer absorption is unwanted. Further UV absorption and emission studies are performed on plastic CD-PDMS polymer phosphor. Plastic CD-PDMS polymer phosphor show broad absorption ranging from 300–800 nm as observed in Fig. 5a with peak centred at around ∼570 nm. Broadened absorption and appearance of peak at 570 nm in CDs embedded in PDMS matrix unlike CDs in colloidal state (CDs dispersed in CHCl3) as shown in Fig. 5d is due to enhanced surface passivation of CDs in a more confined environment minimizing the agglomeration and self-absorption loses. CD–CD interactions and agglomeration of CDs is minimized by dispersing them in PDMS matrix. Also, PDMS passivates the non-emissive surface states/dangling bonds on plastic CD surface broadening the absorption spectra. This broad absorption of CD phosphor leads to a broad emission as observed in Fig. 5b, c, e and g. CD phosphor made using gloves and storage container plastic show broad emission with bandwidth of 177 nm, 205 nm and 171 nm, 197 nm at 320 nm and 350 nm excitations. Similar to plastic CDs in colloidal states CDs embedded in PDMS matrix (CD PDMS polymer phosphor) when excited at different energies ranging from 320 nm to 500 nm show a red shifted emission that corresponds to size and surface effects. Different sized dots and different surface groups on CD surface excite at different energies at different energies and emit correspondingly. CD polymer phosphor fabricated using gloves and storage container plastic emit bright white light under UV illumination as shown in Fig. 5h. Also solid state emission of plastic CDs in no dispersing/embedding media is observed by drop casting plastic CDs on a filter paper. Unlike most semiconductor quantum dots that need a dispersing solvent to emit as shown in Fig. 5h, plastic CDs drop casted on filter paper show bright white emission under UV lamp. This solid state emission of plastic CDs enables their application in fabricating electroluminescent white LEDs.
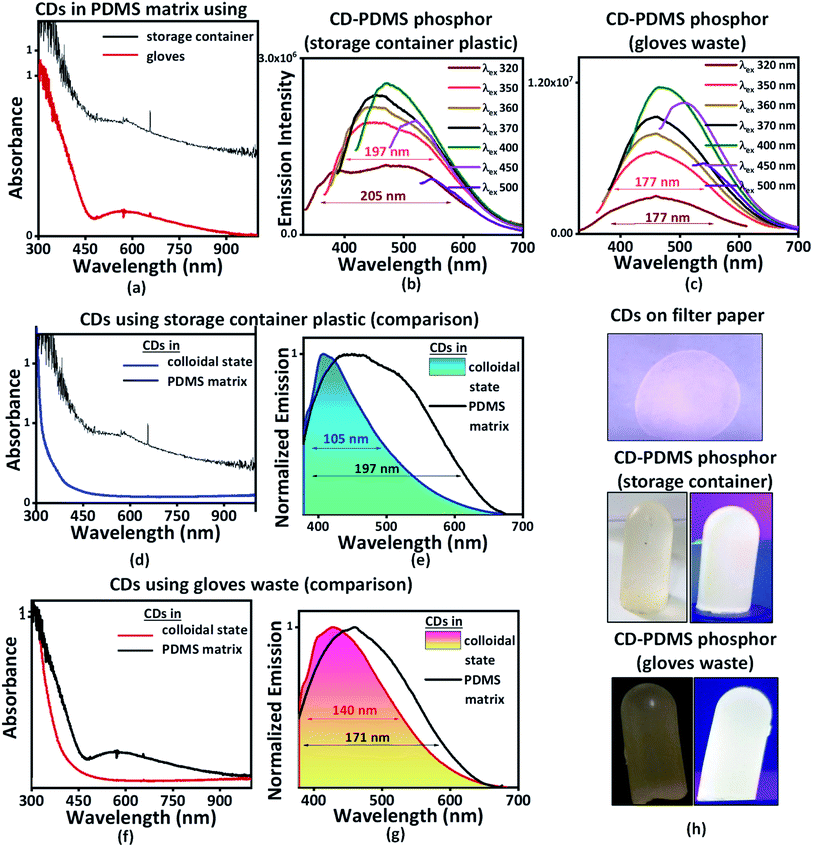 |
| Fig. 5 Showing (a) absorption (b and c) emission spectra of plastic CDs in PDMS matrix. (d) Comparison between (d and f) absorption (e and g) emission spectra of plastic CDs in CHCl3 and in PDMS matrix. (h) Images (from top to bottom) of plastic CDs on filter paper under UV illumination, plastic CD PDMS phosphor (plastic CDs in PDMS matrix). | |
To find the potential application of fabricated plastic CDs in white LEDs it is essential to find out the stability/durability of these dots45–48 i.e., if their emission degrades with time on exposure to ambient atmospheric conditions and moisture. There have been reports discussing the thermal stability of CD phosphor for CD WLED.49 In our work fabricated CD phosphor is placed in open atmosphere (under day light – ambient atmospheric conditions) for a span of 300 days and the emission spectra is recorded. Plastic CD phosphor is chosen for stability studies unlike plastic CDs in colloidal state to rule out the concentration effects on emission. It is observed that emission spectra and emission intensity of plastic CD phosphor is unchanged as observed in Fig. 6a and b. This shows that the fabricated plastic CDs don't photo bleach/photo degrade with time and their emission is unaffected on exposure to open atmosphere.
 |
| Fig. 6 Stability studies of fabricated CD-PDMS phosphor (CDs in PDMS matrix) to open atmosphere. Graphs showing emission spectra of CD-PDMS phosphor fabricated using CDs made from (a) storage container plastic waste and (b) gloves waste on exposure to open atmosphere for a span of 300 days. | |
White LED fabrication using plastic CDs
White LED using plastic CDs as phosphor is fabricated as shown in figure with plastic CDs coated over a 395 nm UV chip (Fig. 7a inset). The fabricated plastic CD-WLED emit bright white light under a forward bias voltage of ∼2.6 V to 9 V. Electroluminescence of plastic CD WLED is recorded and shown in Fig. 7a at a forward bias of 2.6 V. Fabricated plastic CD WLEDs exhibit a good color rendering index (CRI) of 70 with CRI co-ordinates of (0.25, 0.32) (Fig. 7b). The fabricated plastic CD WLEDs exhibit a low emission threshold voltage of 2.6 V which is the lowest driving voltage for carbon dot based white LEDs.50
 |
| Fig. 7 (a) Electroluminescence of plastic CD WLED at a forward bias of 2.6 V. Inset showing white LED using plastic CDs as phosphor is fabricated with plastic CDs coated over a 395 nm blue emitting UV chip. (b) Fabricated plastic CD WLEDs exhibit a good color rendering index (CRI) of 70 with CIE co-ordinates of (0.25, 0.32). | |
Conclusions
In summary, we have recycled PPE (gloves, face shield, face mask) and other plastic waste (syringe, bottle, storage container plastic) generated during Covid-19 into white LEDs. With our unique synthesis technique we were able to synthesize highly luminescent single system white light emitting carbon dots. Further synthesized plastic CDs are embedded in PDMS matrix to develop CD-PDMS phosphor for white LEDs. Finally white LED using plastic CDs is reported with CRI of ∼70 and CIE co-ordinates of (0.25, 0.32). This work of generating light from waste will reduce environmental plastic pollution (raised beyond limited due to Covid-19) and provides an economical and sustainable white LEDs.
Conflicts of interest
There are no conflicts to declare.
Acknowledgements
This work was supported by Seed grant from IISc, SERB through grant EEQ/2017/00655, STC through grant ISTC/PIA/AB/460 and Infosys research grant. The author is thankful for the characterization facilities provided by CeNSe, IISc Bangalore.
References
- J. Hopewell, R. Dvorak and E. Kosior, Plastics recycling: challenges and opportunities, Philos. Trans. R. Soc. London, Ser. B, 2009, 364(1526), 2115–2126 CrossRef CAS PubMed.
- D. Cressey, Bottles, bags, ropes and toothbrushes: the struggle to track ocean plastics, Nature, 2016, 536, 263–265 CrossRef CAS PubMed.
- G. Lopez, M. Artetxe, M. Amutio, J. Bilbao and M. Olazar, Thermochemical routes for the valorization of waste polyolefinic plastics to produce fuels and chemicals. A review, Renew. Sustain. Energy Rev., 2017, 73, 346–368 CrossRef CAS.
- A. Rahimi and J. M. Garcia, Chemical recycling of waste plastics for new materials production, Nat. Rev. Chem, 2017, 1(0046), 1–13 Search PubMed.
- R. Leblanc, The Decomposition of Waste in Landfills, The balance small business. 2021 Search PubMed.
- M. Zuo, Coronavirus leaves China with mountains of medical waste, South China Morning Post. 2020 Search PubMed.
- S. Bengali, The COVID-19 pandemic is unleashing a tidal wave of plastic waste, The Los Angeles Times. 2020 Search PubMed.
- N. U. Benson, D. E. Bassey and T. Palanisami, COVID pollution: impact of COVID-19 pandemic on global plastic waste footprint, Heliyon, 2021, 7(2), 1–9 CrossRef PubMed.
- COVID-19 impact on packaging market by material type, application and region—global forecast to 2021, Business Insider, 2020.
- A. L. P. Silva, J. C. Prata, T. R. Walker, D. Campos, A. C. Duarte, A. M. V. M. Soares, D. Barcelo and T. R. Santos, Increased plastic pollution due to COVID-19 pandemic: Challenges and recommendations, Sci. Total Environ., 2020, 742(140565), 1–9 Search PubMed.
- C. Zhuo and Y. A. Levendis, Upcycling Waste Plastics into Carbon Nanomaterials: A Review, J. Appl. Polym. Sci., 2014, 131(4), 1–14 CrossRef.
- O. I. Nkwachukwu, C. H. Chima, A. O. Ikenna and L. Albert, Focus on potential environmental issues on plastic world towards a sustainable plastic recycling in developing countries, Int. J. Ind. Chem., 2013, 4(34), 1–13 Search PubMed.
- F. Zhang, M. Zeng, R. D. Yappert, J. Sun, Y. H. Lee, A. M. L. Pointe, B. Peters, M. M. A. Omar and S. L. Scott, Polyethylene upcycling to long-chain alkylaromatics by tandem hydrogenolysis/aromatization, Science, 2020, 370(6515), 437–441 CrossRef CAS PubMed.
- B. Eva, O. Karin and H. Minna, Designed from Recycled: Turning Polyethylene Waste to Covalently Attached Polylactide Plasticizers, ACS Sustainable Chem. Eng., 2019, 7(12), 11004–11013 CrossRef.
- M. P. Aji, A. L. Wati, A. Priyanto, J. Karunawan, B. W. Nuryadin, E. Wibowo, P. Marwoto and Sulhadi, Polymer carbon dots from plastics waste upcycling, Environ. Nanotechnol. Monit. Manag., 2018, 9, 136–140 Search PubMed.
- S. Chaudhary, M. Kumari, P. Chauhan and G. R. Chaudhary, Upcycling of plastic waste into fluorescent carbon dots: An environmentally viable transformation to biocompatible C-dots with potential prospective in analytical applications, Waste Manag., 2021, 120, 675–686 CrossRef CAS PubMed.
- A. A. Wala, P. E. Savas, D. X. Luong, W. Chen, C. Kittrell, M. Bhat, R. Shahsavari and J. M. Tour, Flash Graphene from Plastic Waste, ACS Nano, 2020, 14(11), 15595–15604 CrossRef PubMed.
- X. Mu, Y. Li, X. Liu, C. Ma, H. Jiang, J. Zhu, X. Chen, T. Tang and E. Mijowska, Controllable Carbonization of Plastic Waste into Three-Dimensional Porous Carbon Nanosheets by Combined Catalyst for High Performance Capacitor, Nanomaterials, 2020, 10(1097), 2–13 Search PubMed.
- H. Song, X. Liu, B. Wang, Z. Tang and S. Lu, High production-yield solid-state carbon dots with tunable photoluminescence for white/multi-color light-emitting diodes, Sci. Bull., 2019, 64(23), 1788–1794 CrossRef CAS.
- V. Ramanan, B. Siddaiah, K. Raji and P. Ramamurthy, Green Synthesis of Multifunctionalized, Nitrogen-Doped, Highly Fluorescent Carbon Dots from Waste Expanded Polystyrene and Its Application in the Fluorimetric Detection of Au3+ Ions in Aqueous Media, ACS Sustainable Chem. Eng., 2018, 6(2), 1627–1638 CrossRef CAS.
- N. A. E. Essawy, A. H. Konsowa, M. Elnouby and H. A. Farag, A novel one-step synthesis for carbon-based nanomaterials from polyethylene terephthalate (PET) bottles waste, J. Air Waste Manage. Assoc., 2017, 67(3), 358–370 CrossRef PubMed.
- A. Kumari, A. Kumar, S. K. Sahu and S. Kumar, Synthesis of green fluorescent carbon quantum dots using waste polyolefins residue for Cu2+ ion sensing and live cell imaging, Sens. Actuators, B, 2018, 254, 197–205 CrossRef CAS.
- H. Yang, S. Jiang, H. Fang, X. Hu, G. Duan and H. Hou, Molecular orientation in aligned electrospun polyimide nanofibers by polarized FT-IR spectroscopy, Spectrochim. Acta, Part A, 2018, 200, 339–344 CrossRef CAS PubMed.
- S. Jiang, D. Han, C. Huang, G. Duan and H. Hou, Temperature-induced molecular orientation and mechanical properties of single electrospun polyimide nanofiber, Mater. Lett., 2018, 216, 81–83 CrossRef CAS.
- W. Xu, Y. Ding, Y. Yu, S. Jiang, L. Chen and H. Hou, Highly foldable PANi@CNTs/PU dielectric composites toward thin-film capacitor application, Mater. Lett., 2017, 192, 25–28 CrossRef CAS.
- S. Zhou, G. Zhou, S. Jiang, P. Fan and H. Hou, Flexible and refractory tantalum carbide-carbon electrospun nanofibers with high modulus and electric conductivity, Mater. Lett., 2017, 200, 97–100 CrossRef CAS.
- J. Ge, Q. Jia, W. Liu, M. Lan, B. Zhou, L. Guo, H. Zhou, H. Zhang, Y. Wang, Y. Gu and X. Meng, Carbon Dots with Intrinsic Theranostic Properties for Bioimaging, Red-Light-Triggered Photodynamic/Photothermal Simultaneous Therapy In Vitro and In Vivo, Adv. Healthcare Mater., 2015, 5, 665–675 CrossRef PubMed.
- X. M. Wei, Y. Xu, Y. H. Li, X. B. Yin and X. W. He, Ultrafast synthesis of nitrogen-doped carbon dots via neutralization heat for bioimaging and sensing applications, RSC Adv., 2014, 4(84), 44504–44508 RSC.
- Z. Wang, Y. Liu, S. Zhen, X. Li, W. Zhang, X. Sun, B. Xu, X. Wang, Z. Gao and X. Meng, Gram-Scale Synthesis of 41% Efficient Single-Component White-Light-Emissive Carbonized Polymer Dots with Hybrid Fluorescence/Phosphorescence for White Light-Emitting Diodes, Adv. Sci., 2020, 7, 1902688 CrossRef CAS PubMed.
- T. Yuan, F. Yuan, X. Li, Y. Li, L. Fan and S. Yang, Fluorescence–phosphorescence dual emissive carbon nitride quantum dots show 25% white emission efficiency enabling single-component WLEDs, Chem. Sci., 2019, 10, 9801 RSC.
- H. J. Wang, W. Y. Hou, T. T. Yu, H. Li. Chen and Q. Q. Zhang, Facile microwave synthesis of carbon dots powder with enhanced solid-state fluorescence and its applications in rapid fingerprints detection and white-light-emitting diodes, Dyes Pigm., 2019, 170, 107623 CrossRef CAS.
- F. Yan, Y. Jiang, X. Sun, J. Wei, L. Chen and Y. Zhang, Multicolor carbon dots with concentration-tunable fluorescence and solvent-affected aggregation states for white light-emitting diodes, Nano Res., 2020, 13(1), 52–60 CrossRef CAS.
- M. Jagannathan, D. Dhinasekaran, P. Soundharraj, S. Rajendran, D. V. N. Vo, A. Prakasarao and S. Ganesan, Green synthesis of white light emitting carbon quantum dots: Fabrication of white fluorescent film and optical sensor applications, J. Hazard. Mater., 2021, 416, 125091 CrossRef CAS PubMed.
- X. Da, Z. Han, Z. Yang, D. Zhang, R. Hong, C. Tao, H. Lin and Y. Huang, Preparation of multicolor carbon dots with high fluorescence quantum yield and application in white LED, Chem. Phys. Lett., 2022, 794, 139497 CrossRef CAS.
- X. Lei, D. Li, Y. Chen, Q. Liu, Q. Yan, J. Wang, B. Han, G. He and B. An, RGB-multicolor fluorescent carbon dots by changing the reaction solvent type for white light-emitting diodes, New J. Chem., 2022, 46, 4979 RSC.
- T. Zhang, F. Zhao, L. Li, B. Qi, D. Zhu, J. Lü and C. Lü, Tricolor White-Light-Emitting Carbon Dots with Multiple-Cores@Shell Structure for WLED Application, ACS Appl. Mater. Interfaces, 2018, 10(23), 19796–19805 CrossRef CAS PubMed.
- Y. Liu, M. Nishiura, Y. Wang and Z. Hou, π-Conjugated Aromatic Enynes as a Single-Emitting Component for White Electroluminescence, J. Am. Chem. Soc., 2006, 128, 5592–5593 CrossRef CAS PubMed.
- H. Yan, D. Tu, P. Leong, S. Guo, C. Lu and Q. Zhao, Highly Emissive Organic Single-Molecule White Emitters by Engineering o-Carborane-Based Luminophores, Angew. Chem., Int. Ed., 2017, 56(38), 11370–11374 CrossRef PubMed.
- Q. Y. Yang and J. M. Lehn, Bright white-light emission from a single organic compound in the solid state, Angew. Chem., Int. Ed., 2014, 53(18), 4572–4577 CrossRef CAS PubMed.
- Y. J. Yang, M. Lowry, C. M. Schowalter, S. O. Fakayode, J. O. Escobedo, X. Y. Xu, H. T. Zhang, T. J. Jensen, F. R. Fronczek, I. M. Warner and R. M. Strongin, An Organic White Light-Emitting Fluorophore, J. Am. Chem. Soc., 2006, 128(43), 14081–14092 CrossRef CAS PubMed.
- Z. Y. Zhang, Y. S. Wu, K. C. Tang, C. L. Chen, J. W. Ho, J. H. Su, H. Tian and P. T. Chou, Excited-State Conformational/Electronic Responses of Saddle-Shaped N,N′-Disubstituted-Dihydrodibenzo[a,c]phenazines: Wide-Tuning Emission from Red to Deep Blue and White Light Combination, J. Am. Chem. Soc., 2015, 137(26), 8509–8520 CrossRef CAS PubMed.
- C. J. Zhou, S. T. Zhang, Y. Gao, H. C. Liu, T. Shan, X. M. Liang, B. Yang and Y. G. Ma, Ternary Emission of Fluorescence and Dual Phosphorescence at Room Temperature: A Single-Molecule White Light Emitter Based on Pure Organic Aza-Aromatic Material, Adv. Funct. Mater., 2018, 28, 1802407 CrossRef.
- M. Perikala and A. Bhardwaj, Engineering photo-luminescent centers of carbon dots to achieve higher quantum yields, ACS Appl. Electron. Mater., 2020, 2(8), 2470–2478 CrossRef CAS.
- M. Perikala and A. Bhardwaj, Polymer Carbon Dots for Next Generation White Light Emitting Devices, IEEE 21st International Conference on Nanotechnology (NANO), 2021, pp. 356–359 Search PubMed.
- M. Perikala and A. Bhardwaj, Highly stable white-light-emitting carbon dot synthesis using a non-coordinating solvent, ACS Omega, 2019, 4(25), 21223–21229 CrossRef CAS PubMed.
- M. Perikala and A. Bhardwaj, Synthesis of highly stable carbon dots, AIP Conf. Proc., 2020, 2276(1), 020020 CrossRef.
- M. Perikala and A. Bhardwaj, Excellent color rendering index single system white light emitting carbon dots for next generation lighting devices, Sci. Rep., 2021, 11(1), 1–11 CrossRef PubMed.
- M. Perikala and A. Bhardwaj, Design and development of electroluminescent white light emitting diodes using carbon dots as active emitters, Bulletin of the American Physical Society, 2022 Search PubMed.
- Y. Yan, L. Yin, H. Guo, L. Wang and J. Zhang, High Stability Carbon Dots Phosphor and Ultra-High Color Rendering Index White Light-Emitting Diodes, IEEE Photonics J., 2022, 14(1), 1–6 Search PubMed.
- X. Mo, Q. Lu, T. Li, X. Tao, C. Qi, Y. Zhou, Q. Jiang and Y. Ouyang, Low-voltage multicolor electroluminescence from all-inorganic carbon dots/Si-heterostructured light-emitting diodes, J. Mater. Sci., 2019, 54, 8492–8503 CrossRef CAS.
|
This journal is © The Royal Society of Chemistry 2022 |
Click here to see how this site uses Cookies. View our privacy policy here.