DOI:
10.1039/D2RA00594H
(Paper)
RSC Adv., 2022,
12, 7262-7267
Chemoselective and one-pot synthesis of novel coumarin-based cyclopenta[c]pyrans via base-mediated reaction of α,β-unsaturated coumarins and β-ketodinitriles†
Received
28th January 2022
, Accepted 18th February 2022
First published on 4th March 2022
Abstract
In this paper, the base-mediated cascade reactions of 4-chloro-3-vinyl coumarins with β-ketodinitriles were demonstrated, allowing the efficient synthesis of coumarin-based cyclopenta[c]pyran-7-carbonitriles with interesting chemoselectivity. These transformations include the domino-style formation of C–C/C–C/C–O bonds through a base-mediated nucleophilic substitution, Michael addition, tautomerization, O-cyclization, elimination, and aromatization. The presented synthetic strategy has many advantages such as simple and readily available starting materials, green solvent, highly chemoselective route, synthetically useful yields, and easy purification of products by washing them with EtOH (96%), described as GAP (Group-Assistant-Purification) chemistry.
Introduction
It is highly of interest to efficiently prepare various heterocyclic structures based on privileged frameworks from the point of view of synthetic organic chemistry and drug discovery. Coumarins are important privileged heterocycles because their derivatives are valuable structures for the discovery of novel pharmaceutically active molecules and therapeutic agents.1–9 For instance, hypocrolide A is a natural antibiotic,10 derived from the fungus Hypocrea sp, and contains the coumarin core (Fig. 1). Furthermore, some substituted coumarins are very favorable to use in perfumes, cosmetics, lasers, radiometric chemosensors, bio-sensors, and living cells imaging.11–14 Accordingly, the synthesis of molecules containing the coumarin motif is highly desirable.
 |
| Fig. 1 Structures of coumarin and hypocrolide A. | |
Based on the literature and our previous reports,15–19 4-chloro-3-vinyl coumarin has three potential electrophilic active sites, which can selectively be attacked by various nucleophiles. Considering these active sites, we decided to investigate the base-mediated reaction of 4-chloro-3-vinyl coumarin 2a and β-ketodinitrile20 1a as a bisnucleophilic synthon. Indeed, we envisioned that this designed reaction offers an efficient pathway for the structural unity between coumarin and cyclopenta[c]pyran21–23 moieties (Fig. 2).
 |
| Fig. 2 The potential active sites of the starting materials and the proposed strategy for the construction of coumarin-based cyclopenta[c]pyrans. | |
It is noteworthy that the cyclopenta[c]pyran scaffold is a privileged heterocyclic system that serves as the structural core in various functionalized natural products. For example, iridoids24–28 are an expansive family of natural monoterpenoids, which are characterized by their cyclopenta[c]pyran ring systems. The structures of some natural iridoids are shown in Fig. 3.
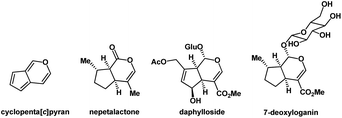 |
| Fig. 3 The core structure of cyclopenta[c]pyran and three natural iridoids possessing this substructure. | |
Members of this family have marine and terrestrial origins29–31 and they have attracted broad attention owing to their various pharmaceutical activities. In this context, developing straightforward methods for the preparation of such structures is worth exploring.
Results and discussion
We started our investigation with the preparation of β-ketodinitrile 1a by the base-mediated condensation of phenacyl bromide, and malononitrile in absolute EtOH at room temperature. After 2 h the reaction was completed, and compound 1a was formed. Then, without further purification within a one-pot sequential process, 4-chloro-3-vinyl coumarin 2a, and two equiv. KOH were added to the reaction tube. The reaction was stirred magnetically at room temperature for 24 h, but no specific product was formed. Pleasingly, a moderate yield of the expected coumarin-based cyclopenta[c]pyran 3a (55% yield) was obtained when the reaction mixture was refluxed at 80 °C for 15 hours (Scheme 1).
 |
| Scheme 1 Synthesis of coumarin-based cyclopenta[c]pyran 3a. | |
Encouraged by this very interesting result, the above reaction was chosen as the model reaction, and various bases were screened to optimize the reaction conditions. The desired product 3a was formed with moderate yields (49–60%) when inorganic bases such as NaOH, K2CO3, and Cs2CO3 were used (Table 1, entries 8–10). Then, piperidine (with relatively more nucleophilicity), DBU, and Et3N were tested for this process. The reaction promoted via piperidine didn't give 3a at all, probably owing to the nucleophilic substitution of piperidine with the chlorine atom of the substrate 2a (Table 1, entry 5). Moreover, product 3a was found with a negligible yield when DBU was used as base, and a mixture of overlapping spots were observed (Table 1, entry 6). Our examinations showed that Et3N is the best base for this transformation, affording the product 3a in a yield of 79% (Table 1, entry 1). In continue, different solvents were tested to obtain the optimal reaction solvent, and the best result was gained in absolute EtOH (Table 1, entries 1–4). It should be mentioned that the yield was remarkably decreased in non-anhydrous solvents. Furthermore, at temperatures below the reflux temperature, the reaction was completed over longer periods of time. We established the optimal reaction conditions for the preparation of coumarin-based cyclopenta[c]pyran derivatives as follows: use of 2.0 equiv. Et3N as the base and absolute EtOH as the solvent to perform the reaction at 80 °C (Table 1).
Table 1 Survey on conditions for the synthesis of 3aa
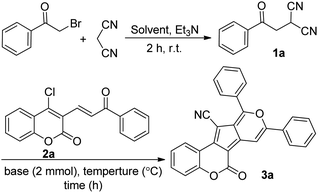
|
Entry |
Base |
Solvent |
Temp. (°C) |
Time (h) |
Yield (%) |
To a magnetically stirred solution of phenacyl bromide (1 mmol, 199 mg), and malononitrile (1 mmol, 66 mg), was added Et3N (1 mmol, 101 mg) in the mentioned absolute solvent. After 2 h, substrate 2a (1 mmol, 310 mg), and base (2 mmol) were added to the reaction mixture. The reaction was carried out at mentioned temperature, and it was monitored by TLC. After above mentioned time a brilliant orange product was isolated by filtration, and purified by washing with EtOH (96%). |
1 |
Et3N |
EtOH |
80 |
12 |
79 |
2 |
Et3N |
DMF |
80 |
13 |
43 |
3 |
Et3N |
MeCN |
80 |
17 |
65 |
4 |
Et3N |
THF |
65 |
24 |
20 |
5 |
Piperidne |
EtOH |
80 |
— |
— |
6 |
DBU |
EtOH |
80 |
11 |
10 |
7 |
KOH |
EtOH |
80 |
15 |
55 |
8 |
NaOH |
EtOH |
80 |
16 |
49 |
9 |
Cs2CO3 |
EtOH |
80 |
10 |
60 |
10 |
K2CO3 |
EtOH |
80 |
13 |
57 |
With the optimized reaction conditions for the synthesis of coumarin-based cyclopenta[c]pyrans in hand, we set out to investigate the generality of this cascade transformation using differently substituted α,β-unsaturated coumarins. Electron-donating and – withdrawing substitutes on aromatic rings of substrate 2 were all tolerated, affording the expected coumarin-based cyclopenta[c]pyrans (Scheme 2) in satisfactory yields (75–91%, 3b–3f). It is worth mentioning here that the nature of the substituent on α,β-unsaturated coumarins had a slight impact on the yields. Next, the influence of different substituents (either electron-withdrawing or electron-donating) of β-ketodinitrile onto this process was investigated, and products 3g–3k were obtained in very good yields (72–83%). Notably, for substrate 1 with a NO2 substituent attached on the benzene ring, no expected product was detected. With the aim of exploring the synthetic utility of this novel domino process, a gram-scale experiment was performed with phenacyl bromide (3.5 mmol, 0.696 g), malononitrile (3.5 mmol, 0.233 g), and 4-chloro-3-3-viyl coumarin (3.5 mmol, 1.08 g), yielding the desired compound 3a in 71% yield without a remarkable loss of efficiency compared to small scale (79%).
 |
| Scheme 2 Synthesis of coumarin-based cyclopenta[c]pyrans. | |
The structures of the synthesized products were characterized by Fourier transform infrared (FTIR), mass spectrometry, elemental analysis, and 1H NMR. Notably, the solubility of the products was too low, and except for 3d which was more soluble than the others, we couldn't record 13C{H} NMR spectra for them. The molecular structures of the synthesized compounds were undeniably confirmed by X-ray crystallographic analysis of product 3d (Fig. 4).
 |
| Fig. 4 ORTEP diagram of 3d (CCDC 1955485). | |
The mechanism of the first step including β-ketodinitriles formation is known.32–38 Based on experimental observations, a plausible mechanism for the formation of coumarin-based cyclopenta[c]pyrans is depicted in Scheme 3. Iinitially, Et3N deprotonates β-ketodinitrile 1 to form anion intermediate 4. Nucleophilic addition of the intermediate 4 to substrate 2, followed by base-mediated liberation of HCl afford intermediate 5. After that, a domino base-assisted intramolecular Michael addition and subsequent O-cyclization occurs and intermediate 6 forms. The final aromatic product 3 is generated by the base-mediated elimination of H2O and HCN.
 |
| Scheme 3 Mechanistic rationalization for the preparation of 3. | |
Conclusions
In summary, we presented a novel domino-type process for the synthesis of substituted coumarin-based cyclopenta[c]pyrans through a base-mediated nucleophilic substitution/Michael addition/tautomerization/O-cyclization/elimination/aromatization reaction of 4-chloro-3-vinyl coumarins with β-ketodinitriles. Notably, two new carbon–carbon bonds and one carbon–oxygen bond were formed in these reactions, and highly stable polycyclic aromatic products were obtained. This unprecedented strategy was carried out under relatively green conditions, providing an efficient approach to structural unity between two valuable organic moieties.
Experimental
General
Two melting points were measured on an Electrothermal 9100 apparatus. IR spectra were recorded as KBr pellets on a Nicolet FTIR 100 spectrophotometer. 1H NMR (500 MHz, 300 MHz) and 13C NMR (75 MHz) spectra were obtained using Bruker DRX-500 Avance and Bruker DRX-300 Avance spectrometers. All NMR spectra were recorded at r.t. in DMSO-d6 and CDCl3. Chemical shifts are reported in parts per million (δ) downfield from an internal TMS reference. Coupling constants (J values) are reported in hertz (Hz), and standard abbreviations were used to indicate spin multiplicities. Elemental analyses for C, H, and N were performed using a Heraeus CHN-O-Rapid analyzer. Mass spectra were recorded on a Finnigan-MATT 8430 mass spectrometer operating at an ionization potential of 70 eV. All chemicals and solvents were purchased from Merck or Aldrich and were used without further purification. Starting materials were synthesized according to the procedures reported in the literature.15–20 Single crystals of compounds 3d were formed in CH2Cl2.
General procedure for the preparation of compounds 3a–3k
To a magnetically stirred solution of phenacyl bromide (1 mmol, 199 mg), and malononitrile (1 mmol, 66 mg), was added Et3N (1 mmol, 101 mg) in the absolute EtOH (5 ml). After 2 h, α,β-unsaturated coumarin 2 (1 mmol, 310 mg), and Et3N (2 mmol) were added to the reaction mixture. The reaction was carried out at 80 °C, and it was monitored by TLC. After 12 h a brilliant orange product was isolated by filtration, and purified by washing with EtOH (96%).
6-Oxo-8,10-diphenyl-6H-pyrano[3′,4′:4,5]cyclopenta[1,2-c]chromene-11-carbonitrile (3a). Orange powder, dec. point = 310–312 °C, 0.32 g, yield: 79%. IR (KBr): 2201 (C
N), 1720 (C
O), 1643, 1601, and 1542 (Ar), 1225, 1177, 1114, and 999 (C–O) cm−1. Anal. calcd for C28H15NO3 (413.42): C, 81.35; H, 3.66, N, 3.39%. Found: C, 81.34; H, 3.62, N, 3.36%. 1H NMR (500 MHz, DMSO-d6): δ = 7.46 (1H, t, 3JHH = 8.5 Hz, CH2 of coumarin), 7.47 (1H, d, 3JHH = 8.3 Hz, CH4 of coumarin), 7.63–7.66 (4H, m, 4CH of Ph), 7.77 (2H, t, 3JHH = 7.2 Hz, 2CHpara of Ph), 7.83 (1H, t, 3JHH = 7.7 Hz, CH2 of coumarin), 8.15 (2H, t, 3JHH = 7.2 Hz, 2CHortho of Ph), 8.17 (2H, t, 3JHH = 7.2 Hz, 2CHortho of Ph), 8.55 (1H, d, 3JHH = 7.8 Hz, CH1 of coumarin), 8.62 (1H, s, CH7). MS (ESI, 70 eV): m/z (%) = 414 (M+, 100), 384 (12), 356 (24), 327 (24), 251 (22), 206 (18), 105 (13), 77 (16).
2-Chloro-6-oxo-8,10-diphenyl-6H-pyrano[3′,4′:4,5]cyclopenta[1,2-c]chromene-11-carbonitrile (3b). Orange powder, dec. point = 318–320 °C, 0.37 g, yield: 84%. IR (KBr): 2202 (C
N), 1730 (C
O), 1615, 1555, 1541, and 1468 (Ar), 1228, 1176, and 1005 (C–O) cm−1. Anal. calcd for C28H14ClNO3 (447.87): C, 75.09; H, 3.15, N, 3.13%. Found C, 75.07; H, 3.12, N, 3.12%. 1H NMR (300 MHz, CDCl3), δ = 7.35 (1H, d, 3JHH = 8.8 Hz, CH2 of coumarin), 7.49 (1H, dd, 3JHH = 8.8 Hz, 2JHH = 2.5 Hz, CH4 of coumarin), 7.56–7.60 (3H, m, 3CH of Ph), 7.73–7.76 (3H, m, 3CH of Ph), 8.07 (2H, t, 3JHH = 6.2 Hz, 2CHortho of Ph), 8.09 (2H, t, 3JHH = 6.2 Hz, 2CHortho of Ph), 8.66 (1H, d, 3JHH = 2.6 Hz, CH1 of coumarin), 8.74 (1H, s, CH7). MS (ESI, 70 eV): m/z (%) = 447 (M+, 100), 427 (3), 390 (6), 327 (13), 251 (9), 105 (11), 77 (14).
3-Methoxy-6-oxo-8,10-diphenyl-6H-pyrano[3′,4′:4,5]cyclopenta[1,2-c]chromene-11-carbonitrile (3c). Orange powder, dec. point = 343–345 °C, 0.34 g, yield: 77%. IR (KBr): 2198 (C
N), 1727 (C
O), 1614, 1556, 1460, and 1421 (Ar), 1205, 1166, 1117, and 1035 (C–O) cm−1. Anal. calcd for C29H17NO4 (443.45): C, 78.55; H, 3.86, N, 3.16%. Found: C, 78.54; H, 3.85, N, 3.14%. 1H NMR (500 MHz, CDCl3): δ = 3.92 (3H, s, OCH3), 6.92 (1H, d, 3JHH = 2.5 Hz, CH4 of coumarin), 6.95 (1H, dd, 3JHH = 8.5 Hz, 2JHH = 2.5 Hz, CH2 of coumarin), 7.55–7.60 (3H, m, 3CH of Ph), 7.72–7.75 (3H, m, 3CH of Ph), 8.07 (4H, d, 3JHH = 8.7 Hz 4CHortho of Ph), 8.66 (1H, d, 3JHH = 8.5 Hz, CH1 of coumarin), 8.74 (1H, s, CH7). MS (ESI, 70 eV): m/z (%) = 443 (M+, 100), 400 (20), 372 (10), 314 (18), 221 (23), 105 (38), 77 (24).
8-(2-Chlorophenyl)-6-oxo-10-phenyl-6H-pyrano[3′,4′:4,5]cyclopenta[1,2-c]chromene-11-carbonitrile (3d). Orange powder, dec. point = 255–257 °C, 0.38 g, yield: 86%. IR (KBr): 2204 (C
N), 1706 (C
O), 1605, 1548, and 1468 (Ar), 1176, 1115, 1045, and 1029 (C–O) cm−1. Anal. calcd for C28H14ClNO3 (447.87): C, 75.09; H, 3.15, N, 3.13%. Found: C, 75.06; H, 3.12, N, 3.12%. 1H NMR (500 MHz, DMSO-d6): δ = 7.42 (1H, t, 3JHH = 7.4 Hz, CH2 of coumarin), 7.47 (1H, d, 3JHH = 8.0 Hz, CH4 of coumarin), 7.59–7.66 (3H, m, 3CH of Ar), 7.70–7.74 (3H, m, 3CH of Ph), 7.78 (1H, t, 3JHH = 7.4 Hz, CH3 of coumarin), 7.98 (1H, d, 3JHH = 7.0 Hz, CH of Ar), 8.11 (2H, d, 3JHH = 7.2 Hz, 2CH of Ar), 8.50 (1H, d, 3JHH = 7.8 Hz, CH1 of coumarin), 8.51 (1H, s, CH7). 13C NMR (75 MHz, CDCl3): δ = 80.76, 111.48, 117.00, 117.70, 117.94, 123.74, 124.72, 125.59, 127.49, 128.86, 129.77, 130.77, 131.01, 131.09, 131.10, 131.47, 131.97, 132.20, 133.07, 139.31, 148.03, 153.31, 155.44, 158.49, 162.77. MS (ESI, 70 eV): m/z (%) = 447 (M+, 7), 308 (13), 251 (11), 139 (100), 105 (46), 77 (34). Crystal data for 3d C28H14ClNO3 (CCDC 1955485): MW = 575.55, monoclinic, P121/n1, a = 7.4936(15) Å, b = 24.222(5) Å, c = 13.519(3) Å, α = 90, β = 101.90(3), γ = 90, V = 2401.1(9) Å3, Z = 4, Dc = 1.474 mg m−3, F(000) = 1088, crystal dimension 0.50 × 0.30 × 0.20 mm, radiation, Mo Kα (λ = 0.71073 Å), 2.280 ≤ 2θ ≤ 24.499, intensity data were collected at 293(2) K with a Bruker APEX area-detector diffractometer, and employing ω/2θ scanning technique, in the range of −8 ≤ h ≤ 8, 0 ≤ k ≤ 28, 0 ≤ l ≤ 15; the structure was solved by a direct method, all non-hydrogen atoms were positioned and anisotropic thermal parameters refined from 3827 observed reflections with R (into) = 0.0286 by a full-matrix least-squares technique converged to R1 = 0.0695, and wR2 = 0.1724 [I > 2sigma(I)].
6-Oxo-8-phenyl-10-(p-tolyl)-6H-pyrano[3′,4′:4,5]cyclopenta[1,2-c]chromene-11-carbonitrile (3e). Orange powder, dec. point = 342–345 °C, 0.32 g, yield: 75%. IR (KBr): 2198 (C
N), 1730 (C
O), 1605, 1560, 1543, and 1511 (Ar), 1176, 1112, 1052, and 1036 (C–O) cm−1. Anal. calcd for C29H17NO3 (427.45): C, 81.49; H, 4.01, N, 3.28%. Found: C, 81.44; H, 4.02, N, 3.26%. 1H NMR (300 MHz, DMSO-d6): δ = 2.41 (3H, s, CH3), 7.44 (2H, d, 3JHH = 8.0 Hz, 2CH of Ar), 7.45 (1H, t, 3JHH = 7.8 Hz, CH2 of coumarin), 7.49 (1H, d, 3JHH = 7.8 Hz, CH4 of coumarin), 7.67 (1H, t, 3JHH = 7.4 Hz, CH3 of coumarin), 7.74 (1H, t, 3JHH = 8.5 Hz, CHpara of Ph), 7.77 (2H, t, 3JHH = 8.5 Hz, 2CHmeta of Ph), 8.05 (2H, d, 3JHH = 8.0 Hz, 2CH of Ar), 8.15 (2H, d, 3JHH = 8.4 Hz, 2CHortho of Ph), 8.58 (1H, d, 3JHH = 7.7 Hz, CH1 of coumarin), 8.62 (1H, s, CH7). MS (ESI, 70 eV): m/z (%) = 427 (M+, 100), 370 (6), 354 (8), 340 (10), 327 (14), 264 (12), 105 (55), 77 (21).
8-(4-Nitrophenyl)-6-oxo-10-phenyl-6H-pyrano[3′,4′:4,5]cyclopenta[1,2-c]chromene-11-carbonitrile (3f). Orange powder, dec. point = 367–369 °C, 0.41 g, yield: 91%. IR (KBr): 2204 (C
N), 1720 (C
O), 1594, 1542, 1521, and 1424 (Ar), 1175, 1111, 1051, and 1000 (C–O) cm−1. Anal. calcd for C28H14N2O5 (458.09): C, 73.36; H, 3.08, N, 6.11%. Found: C, 73.34; H, 3.10, N, 6.13%. 1H NMR (500 MHz, DMSO-d6): δ = 7.43 (1H, t, 3JHH = 8.4 Hz, CH2 of coumarin), 7.46 (1H, d, 3JHH = 8.6 Hz, CH4 of coumarin), 7.63 (1H, t, 3JHH = 8.0 Hz, CH3 of coumarin), 7.76 (2H, t, 3JHH = 7.6 Hz, 2CHmeta of Ph), 7.82 (1H, t, 3JHH = 7.4 Hz, CHpara of Ph), 8.17 (2H, d, 3JHH = 7.6 Hz, 2CHortho of Ph), 8.38 (2H, d, 3JHH = 8.6 Hz, 2CH of Ar), 8.41 (2H, d, 3JHH = 8.6 Hz, 2CH of Ar), 8.55 (1H, d, 3JHH = 7.9 Hz, CH1 of coumarin), 8.80 (1H, s, CH7). MS (ESI, 70 eV): m/z (%) = 458 (M+, 100), 412 (23), 354 (30), 327 (62), 251 (28), 105 (71), 77 (46).
6-Oxo-8,10-di-p-tolyl-6H-pyrano[3′,4′:4,5]cyclopenta[1,2-c]chromene-11-carbonitrile (3g). Orange powder, dec. point = 348–350 °C, 0.31 g, yield: 72%. IR (KBr): 2198 (C
N), 1730 (C
O), 1604, 1563, 1484, and 1422 (Ar), 1175, 1112, 1051, and 998 (C–O) cm−1. Anal. calcd for C30H19NO3 (441.14): C, 81.62; H, 4.34, N, 3.17%. Found: C, 81.63; H, 4.36, N, 3.15%. 1H NMR (500 MHz, DMSO-d6): δ = 2.47 (3H, s, CH3), 2.55 (3H, s, CH3), 7.37 (2H, d, 3JHH = 7.7 Hz, 2CH of Ar), 7.38 (1H, t, 3JHH = 7.2 Hz, CH2 of coumarin), 7.43 (1H, d, 3JHH = 8.2 Hz, CH4 of coumarin), 7.53 (2H, d, 3JHH = 7.7 Hz, 2CH of Ar), 7.56 (1H, t, 3JHH = 7.7 Hz, CH3 of coumarin), 7.96 (2H, d, 3JHH = 7.8 Hz, 2CH of Ar), 7.99 (2H, d, 3JHH = 7.9 Hz, 2CH of Ar), 8.72 (1H, s, CH7), 8.77 (1H, d, 3JHH = 7.9 Hz, CH1 of coumarin). MS (ESI, 70 eV): m/z (%) = 441 (M+, 100), 354 (16), 264 (19), 220 (29), 119 (25), 91 (57).
10-(4-Bromophenyl)-6-oxo-8-phenyl-6H-pyrano[3′,4′:4,5]cyclopenta[1,2-c]chromene-11-carbonitrile (3h). Orange powder, dec. point = 357–359 °C, 0.40 g, yield: 83%. IR (KBr): 2204 (C
N), 1729 (C
O), 1602, 1563, 1541, and 1425 (Ar), 1175, 1113, 1051, and 1005 (C–O) cm−1. Anal. calcd for C28H14BrNO3 (491.02): C, 68.31; H, 2.87, N, 2.85%. Found: C, 68.33; H, 2.85, N, 2.86%. 1H NMR (500 MHz, DMSO-d6): δ = 7.48 (1H, t, 3JHH = 8.2 Hz, CH2 of coumarin), 7.49 (1H, d, 3JHH = 8.4 Hz, CH4 of coumarin), 7.63 (2H, d, 3JHH = 7.0 Hz, 2CH of Ar), 7.64 (1H, t, 3JHH = 8.2 Hz, CHpara of Ph), 7.67 (1H, t, 7.2 Hz, CH3 of coumarin), 7.98 (2H, d, 3JHH = 8.2 Hz, 2CHmeta of Ph), 8.11 (2H, d, 3JHH = 8.2 Hz, 2CHortho of Ph), 8.16 (2H, d, 3JHH = 7.0 Hz, 2CH of Ar), 8.56 (1H, d, 3JHH = 7.0 Hz, CH1 of coumarin), 8.66 (1H, s, CH7). MS (ESI, 70 eV): m/z (%) = 493 (M+ + 1, 100), 491 (M+, 98), 327 (26), 251 (30), 105 (27), 77 (41).
10-(4-Chlorophenyl)-6-oxo-8-phenyl-6H-pyrano[3′,4′:4,5]cyclopenta[1,2-c]chromene-11-carbonitrile (3i). Orange powder, dec. point = 370–373 °C, 0.36 g, yield: 81%. IR (KBr): 2200 (C
N), 1729 (C
O), 1602, 1563, 1468, and 1423 (Ar), 1225, 1175, 1112, and 1051 (C–O) cm−1. Anal. calcd for C28H14ClNO3 (447.87): C, 75.09; H, 3.15, N, 3.13%. Found: C, 75.06; H, 3.13, N, 3.12%. 1H NMR (500 MHz, CDCl3): δ = 7.42 (1H, t, 3JHH = 7.3 Hz, CH2 of coumarin), 7.46 (1H, d, 3JHH = 8.2 Hz, CH4 of coumarin), 7.50–7.64 (4H, m, 2CHmeta of Ph, CHpara of Ph, and CH3 of coumarin), 7.74 (2H, d, 3JHH = 8.5 Hz, 2CH of Ar), 8.06 (2H, d, 3JHH = 8.5 Hz, 2CH of Ar), 8.09 (2H, d, 3JHH = 8.0 Hz, 2CHmeta of Ph), 8.78 (1H, d, 3JHH = 7.9 Hz, CH1 of coumarin), 8.81 (1H, s, CH7). MS (ESI, 70 eV): m/z (%) = 447 (M+, 2), 327 (10), 308 (9), 251 (18), 138 (100), 105 (31), 77 (28).
8-(4-Methoxyphenyl)-6-oxo-10-phenyl-6H-pyrano[3′,4′:4,5]cyclopenta[1,2-c]chromene-11-carbonitrile (3j). Orange powder, dec. point = 318–320 °C, 0.35 g, yield: 80%. IR (KBr): 2198 (C
N), 1735 (C
O), 1603, 1559, 1547, and 1470 (Ar), 1000 (C–O) cm−1. Anal. calcd for C29H17NO4 (443.45): C, 78.55; H, 3.86, N, 3.16%. Found: C, 78.54; H, 3.84, N, 3.15%. 1H NMR (500 MHz, DMSO-d6): δ = 3.95 (3H, s, OCH3), 7.28 (2H, d, 3JHH = 8.7 Hz, 2CH of Ar), 7.40 (1H, t, 3JHH = 7.1 Hz, CH4 of coumarin), 7.41 (1H, d, 3JHH = 7.1 Hz, CH2 of coumarin), 7.50–7.60 (3H, m, 3CH of Ph), 7.60 (1H, t, 3JHH = 6.8 Hz, CH3 of coumarin), 8.07 (2H, d, 3JHH = 7.3 Hz, 2CHortho of Ph), 8.11 (2H, d, 3JHH = 8.6 Hz, 2CH of Ar), 8.48 (1H, s, CH7), 8.51 (1H, d, 3JHH = 7.0 Hz, CH1 of coumarin). MS (ESI), 70 eV: m/z (%) = 443 (M+, 100), 428 (2), 372 (7), 344 (7), 314 (9), 221 (16), 105 (20), 77 (13).
6-Oxo-8-phenyl-10-(p-tolyl)-6H-pyrano[3′,4′:4,5]cyclopenta[1,2-c]chromene-11-carbonitrile (3k). Orange powder, dec. point = 301–305 °C, 0.33 g, yield: 78%. IR (KBr): 2181 (C
N), 1680 (C
O), 1652, 1616, 1598, and 1486 (Ar), 1218, 1201, 1169, and 1105 (C–O) cm−1. Anal. calcd for C29H17NO3 (427.12): C, 81.49; H, 4.01, N, 3.28%. Found: C, 81.51; H, 4.02, N, 3.28%. 1H NMR (500 MHz, DMSO-d6): δ = 2.56 (3H, s, CH3), 7.38 (1H, t, 3JHH = 7.6 Hz, CH2 of coumarin), 7.43 (1H, d, 3JHH = 8.3 Hz, CH2 of coumarin), 7.52–7.60 (6H, m, 6CH of Ar), 8.00 (2H, d, 3JHH = 7.7 Hz, 2CHortho of Ph), 8.07 (2H, d, 3JHH = 7.5 Hz, 2CH of Ar), 8.77 (1H, s, CH7), 8.78 (1H, d, 3JHH = 7.9 Hz, CH1 of coumarin). MS (ESI), 70 eV: m/z (%) = 427 (M+, 100), 370 (4), 340 (5), 327 (4), 213 (9).
Conflicts of interest
There are no conflicts to declare.
Acknowledgements
We gratefully thank Tarbiat Modares University for financial support. We cordially thank Dr Morteza Shiri and Abdolreza Hashemi for helping us to provide some of the required starting materials.
References
- H. Wei, J. Ruan and X. Zhang, RSC Adv., 2016, 6, 10846 RSC.
- R. Pingaew, A. Saekee, P. Mandi, C. Nantasenamat, S. Prachayasittikul, S. Ruchirawat and V. Prachayasittikul, Eur. J. Med. Chem., 2014, 85, 65 CrossRef CAS PubMed.
- R. Nagamallu and K. Kariyappa, Bioorg. Med. Chem. Lett., 2013, 23, 6406 CrossRef PubMed.
- D. B. Petitti, B. L. Strom, M. P. Kenneth and L. Melmon, Am. J. Med., 1986, 81, 255 CrossRef CAS.
- M. Pacholec, J. Tao and C. T. Walsh, Biochemistry, 2005, 44, 14969 CrossRef CAS PubMed.
- L. Zhang and Z. Xu, Eur. J. Med. Chem., 2019, 181, 111587 CrossRef CAS PubMed.
- T. Nasr, S. Bondock, H. M. Rashed, W. Fayad, M. Youns and T. M. Sakr, Eur. J. Med. Chem., 2018, 151, 723 CrossRef CAS.
- M. Z. Hassan, H. Osman, M. A. Ali and M. J. Ahsan, Eur. J. Med. Chem., 2016, 123, 236 CrossRef CAS.
- A. Gliszczynska and P. E. Brodelius, Phytochem. Rev., 2012, 11, 77 CrossRef CAS.
- Y. Yuan, Y. Feng, F. Ren, S. Niu, X. Liu and Y. Che, Org. Lett., 2013, 15, 6050 CrossRef CAS PubMed.
- C. Wu, Y. Sun, Y. Wang, W. Duan, J. Hu, L. Zhou and Q. Pu, Talanta, 2019, 201, 16 CrossRef CAS PubMed.
- S. Zhu, W. Lin and L. Yuan, Dyes Pigm., 2013, 99, 465 CrossRef CAS.
- C. Li, Y. Cai, M. Pang, X. Zhou, X. Luo and Z. Xiao, Biosens. Bioelectron., 2020, 147, 111779 CrossRef CAS PubMed.
- C.-G. Chen, N. Vijay, N. Thirumalaivasan, S. Velmathi and S.-P. Wu, Spectrochim. Acta, Part A, 2019, 219, 135 CrossRef CAS PubMed.
-
(a) A. Alizadeh, B. Farajpour, S. S. Mohammadi, M. Sedghi, H. Naderi-Manesh, C. Janiak and T.-O. Knedel, ChemistrySelect, 2020, 5, 9362 CrossRef CAS;
(b) A. Alizadeh, B. Farajpour, K. Amir Ashjaee Asalemi and S. Taghipour, ChemistrySelect, 2020, 5, 9834 CrossRef CAS.
-
(a) D. Heber, I. C. Ivanov and S. K. Karagiosov, J. Heterocycl. Chem., 1995, 32, 505 CrossRef CAS;
(b) A. Y. Bochkov, I. O. Akchurin, O. A. Dyachenko and V. F. Traven, Chem. Commun., 2013, 49, 11653 RSC.
- A. A. Patel, H. B. Lad, K. R. Pandya, C. V. Patel and D. I. Brahmbhatt, Med. Chem. Res., 2013, 22, 4745 CrossRef CAS.
- R. Bera, G. Dhananjaya, S. Nath, R. Kumar, K. Mukkanti and M. Pal, Tetrahedron, 2009, 65, 1300 CrossRef CAS.
-
(a) A. Alizadeh, B. Farajpour and M. Khanpour, Synlett, 2021, 32, 697 CrossRef CAS;
(b) A. Alizadeh and B. Farajpour, ChemistrySelect, 2021, 6, 6150 CrossRef CAS;
(c) A. Alizadeh and B. Farajpour, ChemistrySelect, 2021, 6, 11925 CrossRef CAS.
- S. Alishetty, H.-P. Shih and C.-C. Han, Org. Lett., 2018, 20, 2513 CrossRef CAS PubMed.
- S. Bera, C. G. Daniliuc and A. Studer, Org. Lett., 2015, 17, 4940 CrossRef CAS PubMed.
- A. Pal and A. Bhattacharjya, J. Org. Chem., 2001, 66, 9071 CrossRef CAS.
- A. Bartholomaeus and J. Ahokas, Toxicol. Lett., 1995, 80, 75 CrossRef CAS.
- H. Franzyk, S. R. Jensen, C. E. Olsen and J. M. Quiroga, Org. Lett., 2000, 2, 699 CrossRef CAS.
- L. Candish and D. W. Lupton, Org. Lett., 2010, 12, 4836 CrossRef CAS PubMed.
- S. Lee, S.-M. Paek, H. Yun, N.-J. Kim and Y.-G. Suh, Org. Lett., 2011, 13, 3344 CrossRef CAS PubMed.
- J. S. Beckett, J. D. Beckett and J. E. A. Hofferberth, Org. Lett., 2010, 12, 1408 CrossRef CAS PubMed.
- M. Soltau, M. Gowert and P. Margaretha, Org. Lett., 2005, 7, 5159 CrossRef CAS PubMed.
- B. Dinda, S. Debnath and Y. Harigaya, Chem. Pharm. Bull., 2007, 55, 159 CrossRef CAS PubMed.
- B. Dinda, S. Debnath and Y. Harigaya, Chem. Pharm. Bull., 2007, 55, 689 CrossRef CAS.
- B. Dinda, S. Debnath and Y. Harigaya, Chem. Pharm. Bull., 2009, 57, 765 CrossRef CAS.
- S. Ahadi, Z. Naghdiani, S. Balalaie and F. Rominger, Tetrahedron, 2015, 71, 6860 CrossRef CAS.
- A. Rakshit, P. Kumar, T. Alam, H. Dhara and B. K. Patel, J. Org. Chem., 2020, 85, 12482 CrossRef CAS.
- N. K. Vaishanv, S. P. Chandrasekharan, M. K. Zaheer, R. Kant and K. Mohanan, Chem. Commun., 2020, 56, 11054 RSC.
- M. Adib, E. Sheikhi, P. Haghshenas, S. Rajai-Daryasarei, H. R. Bijanzadeh and L. G. Zhu, Tetrahedron Lett., 2014, 55, 4983 CrossRef CAS.
- M. Adib, E. Sheikhi, P. Haghshenas and H. R. Bijanzadeh, Synlett, 2010, 18, 2775 CrossRef.
- I. Yavari and O. Khaledian, Synthesis, 2020, 52, 1379 CrossRef CAS.
- J. Sun, R.-Y. Yang and C.-G. Yan, ChemistrySelect, 2017, 2, 304 CrossRef CAS.
Footnote |
† Electronic supplementary information (ESI) available. CCDC 1955485. For ESI and crystallographic data in CIF or other electronic format see DOI: 10.1039/d2ra00594h |
|
This journal is © The Royal Society of Chemistry 2022 |
Click here to see how this site uses Cookies. View our privacy policy here.